Increasing fire danger in the Netherlands due to climate change
Hugo A. Lambrechts
A
B
Abstract
Temperatures and extreme weather events in Northwestern Europe are expected to increase due to climate change. As a result, longer and more intense water deficits are expected, resulting in weather conditions conducive to wildfires.
We assessed the impact of recent and future climate change on fire danger in the Netherlands.
Historical weather data and climate scenarios in combination with the Canadian Fire Weather Index (FWI) and Fine Fuel Moisture Code (FFMC) were used to assess historical and future trends in fire danger.
Our analyses showed that, especially during the last decade (2011–2020), the number of days at elevated fire danger has increased. The number of days with elevated fire danger is projected to double by mid-century compared to the reference period 1981–2010 for high emission scenarios. The days at elevated fire danger during the last decade were already comparable to predictions for 2085, indicating that the climate change scenarios may underestimate future fire danger.
Days at elevated fire danger increased over the last four decades and will continue to do so under future climate scenarios.
The Netherlands needs to prepare for more days with weather conducive to wildfires.
Keywords: adaptation, Canadian fire weather index, climate change, fire danger, fire management, Netherlands, Northwestern Europe, wildfire risk.
Introduction
Globally, there is a trend of planetary warming due to human-induced climate change (Masson-Delmotte et al. 2021). With anthropogenic climate change, there has been increased fire activity in many parts of the world (Ellis et al. 2022). Climate change could lead to changes in fire regimes, fire season length and increase fire risk across most of the planet over the 21st century (Jolly et al. 2015; Abatzoglou et al. 2018). The frequency of heatwaves has increased since 1950, leading to more droughts in southern Europe (IPCC 2023). These heatwaves are predicted to increase all over Europe (Kovats et al. 2014). Also, drought frequency and severity are expected to increase in the future (Grillakis 2019). Climatic change is expected to increase the fire danger across Europe (Ayanz et al. 2011; Karali et al. 2013; Donkers 2018; Giannaros et al. 2019; Dupuy et al. 2020; Stoof 2020). This is the case for both fire-prone Mediterranean countries (Giannakopoulos et al. 2011; San-Miguel-Ayanz et al. 2013; 2020) and emerging fire-prone countries in the Northwestern of Europe (Kovats et al. 2014; De Rigo et al. 2017; Arnell et al. 2021; Perry et al. 2022). Temperate Europe is not usually associated with wildfires, but recently fires have occurred more frequently in this region (Gazzard et al. 2016; Belcher et al. 2021; Stoof et al. 2024). In the Netherlands, a fire in the Deurnese Peel burned an area of 710 hectares on 20 April 2020. This was the biggest recorded recent fire in the Netherlands that overwhelmed the Dutch fire services capacity (Stoof et al. 2020). At the same time, another large wildfire occurred on the Dutch–German border, further challenging the capacity of the firefighting services. In the future, temperatures in the Netherlands are expected to increase, resulting in drought and heatwaves together with more extreme and intense precipitation events (Ligtvoet et al. 2015). These changes in temperature and precipitation will have a direct effect on the fire danger as they influence the fuel moisture conditions of the vegetation. Favourable weather and fuel conditions could create conditions where the potential for wildfires to occur is high. This is called a fire danger day (Merrill and Alexander 1987; De Groot 1998). This increased fire danger does not necessarily mean increased risk for humans i.e. wildfires in remote and unpopulated areas do not directly affect human safety. However, the Netherlands have a high population density and ease of access to natural areas resulting in a high human ignition potential. Human activities cause almost all fires in the Netherlands (Stoof et al. 2024). Wildfires are highly sensitive to changing weather condition caused by climate change as fuel moisture has a profound effect on fire behaviour (Moriondo et al. 2006; Legg et al. 2007). Fuel moisture together with the weather conditions are a good indicator of wildfire occurrence. This means that the number of days with elevated fire danger may increase in the future.
We determined historical and future fire danger in the Netherlands using the Canadian Fire Weather Index (Van Wagner 1987), a commonly used tool to estimate the forest fire danger (Taylor and Alexander 2006; Lawson and Armitage 2008) based on weather data (Bedia et al. 2018; Vitolo et al. 2019). It is often used to assess trends in fire danger, e.g. by Karali et al. 2014 and it is used for establishing relationships between the FWI System components and the fuel moisture/fire behaviour observed in local fuels (de Groot et al. 2006; Palheiro et al. 2006; Taylor and Alexander 2006). It is also applied for identifying periods of high fire activity (Dimitrakopoulos et al. 2011; Holsten et al. 2013; Karali et al. 2013; Bedia et al. 2018; Rovithakis et al. 2022).
In addition to the FWI, we specifically focused on the Fine Fuel Moisture Code (FFMC). The FFMC is a numeric rating of the moisture content of litter and other cured fine fuels, and is considered to be an indicator of the relative ease of ignition and flammability of fine fuels (Stocks et al. 1989). The FFMC can be derived using input data for the relative humidity, temperature, precipitation and windspeed. Van Wagner (1987) suggested that FFMC or Initial Spread Index (ISI) may best reflect fire danger in non-forested landscapes, because these indices primarily focus on the moisture content of fine fuels like grass, leaves and other surface materials. In non-forested landscapes, such as grasslands, shrublands or open fields, fine fuels are the primary sources of flammable material. In the United Kingdom, the FFMC and Initial Spread Index (ISI) were found to represent fire danger in heather and moorland fuels more accurately than the FWI (Legg et al. 2007; Davies et al. 2008; Davies and Legg 2016). Thus, FFMC might better explain fire danger in the open landscapes of the Netherlands.
Together with future projections, understanding how fire danger has changed over time and space helps the scientific community, forest service, land management agencies, civil protection and policymakers to better understand the impact of climate change on fire danger in emerging fire regions such as the Netherlands. According to Galizia et al. (2021), ‘wildfire risk management needs more efficient approaches to better understand fire activity at different spatio-temporal scales (Johnston et al. 2020; Taylor 2020)’. Therefore, we determined the historical and future spatio-temporal variation of elevated fire danger days in the Netherlands. Spatio-temporal knowledge of fire danger can help to identify present and future fire-vulnerable areas and help raising awareness and develop adaptation strategies. This is important for reducing losses through deployment of efficient wildfire prevention and suppression strategies (Giannaros et al. 2021). The key objective of this study was to analyse past and future trends in fire danger in the Netherlands, as an example of a temperate country with emerging fire risk.
Data and methodology
Elevated fire danger for the past and future was assessed using meteorological data, climate projections, Canadian Fire Weather Index analysis (FWI), FFMC and wildfire occurrences. To establish what constitutes elevated fire danger, we (1) explored two different methods to identify a threshold for fire danger; (2) calculated and mapped the historical annual elevated fire danger days (per decade) between 1981 and 2020; and (3) determined the potential future elevated fire danger days annually in the Netherlands for the periods 2036–2065 and 2071–2100 (hereafter referred to as 2050 and 2085, respectively). For this, 5 years of wildfire statistics of wildfire occurrences from Stoof et al. (2024) were matched with FWI and FFMC values calculated for 13 meteorological stations across the country. Trends in fire danger were then assessed for the historical and future periods according to chosen thresholds.
Study area characteristics
The Netherlands is situated in Northwestern Europe and between 50° and 53° northern latitude and 3° and 7° eastern longitude. It occupies an area of 41,865 km2 (Nabielek et al. 2016). The Netherlands is highly urbanised and most of the population lives in small to medium-sized towns. In 2022, the population was 17.6 million and it is one of the most densely populated countries in the world.
The Netherlands has a maritime climate with relatively mild winters, mild summers and rainfall all year round (Kottek et al. 2006). Rainfall is spread throughout the year and the annual precipitation ranges from 700 to 900 mm. The driest months are in spring. The average winter and summer temperatures are 3 and 17°C, respectively. The country’s fire regime is understudied, partly because the collection of wildfire statistics was stopped in 1994 due to low wildfire occurrence (Stoof et al. 2024). Data collection started again in 2017 and the fire season is concentrated in spring during average years, with summer fires being abundant during severe drought years such as 2018. Annual wildfire numbers range between 200 and 1000, with an average of 442 hectares burned each year (between 2017 and 2021). Mean fire size is under 1.5 ha, and the most affected vegetation types are heathland and other open habitat and forest (Stoof et al. 2024).
Matching wildfire occurrences with weather stations
A dataset of fire reports from Stoof et al. (2024) provided a total of 2760 wildfire occurrences for the period 2017–2021. These are the newest wildfire statistics since 1994. The definition of a wildfire is included in Box 1. Based on the wildfire registrations from 2017 until 2021, an elevated fire danger threshold of one fire per day and percentage-based methods were determined. Despite this period being relatively short, it captures a large diversity of weather years including normal, wet and dry years. As such, the dataset includes wildfires that happened under a wide range of weather conditions in the Netherlands. The wildfire incidents were registered to the nearest street name and municipality, and therefore, had to be converted to coordinates in ArcGIS Pro. Subsequently, each fire’s location was matched with the nearest meteorological station that had sufficiently long data available (from 1981 to 2021, Fig. 1), for which the FWI and FFMC parameters were calculated. This resulted in a dataset with FWI and FFMC information for each wildfire event.
Locations of the 13 Dutch Royal Meteorological Institute (KNMI) weather stations (white circles) and wildfire events (coloured circles) for the period 2017–2021. Colours indicate which weather station in each region was used for the threshold assessment.
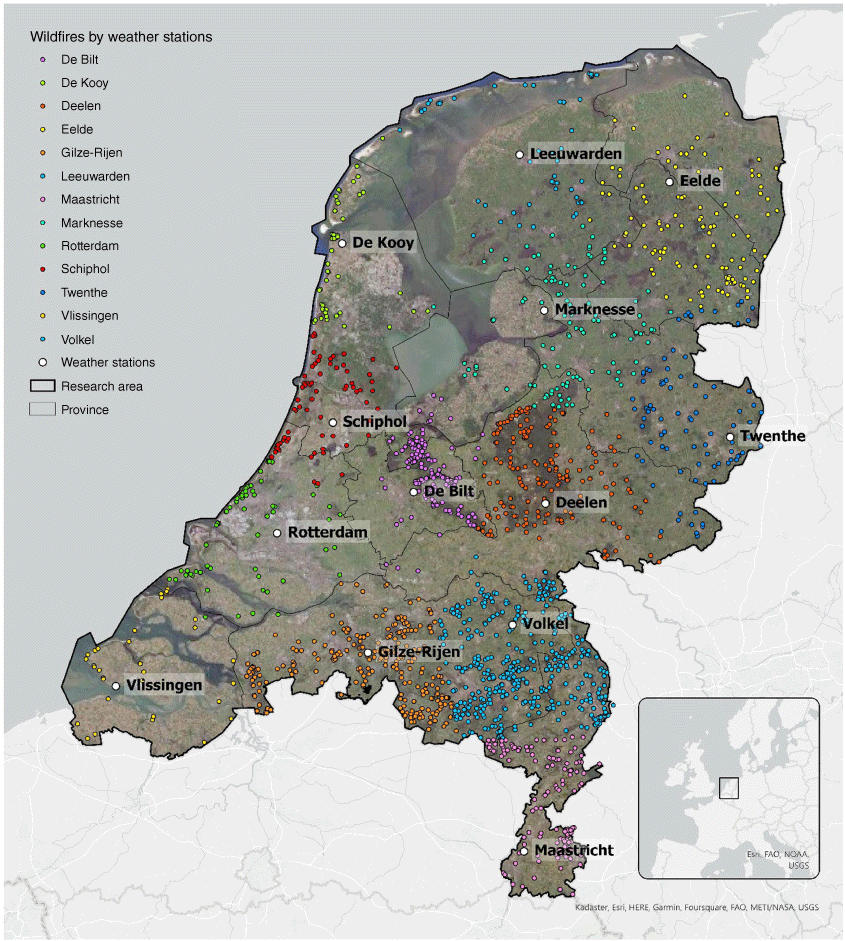
Box 1.Description of fire occurrence reports |
Wildfires are included in the dataset when they meet either of the following two criteria (Stoof et al. 2024):
|
Meteorological input data, historical trends and future climate projections
Meteorological data were obtained from the Dutch Royal Meteorological Institute (KNMI). The historical elevated fire danger days over the past four decades (1981–2020) were calculated using weather data from the KNMI. The dataset from the KNMI provided weather data from 1906 to 2022. Thirteen KNMI weather stations were selected for this study. In case of missing values (although limited to 1 or 2 months for single variables), gaps were filled with average values from the closest weather stations that provided daily weather observations. For our analyses we used average daily value for each of the variables, similar to previous studies in the United Kingdom (e.g. Arnell et al. 2021; Perry et al. 2022).
For the future climate change assessment, we used the KNMI’14 scenarios (Attema et al. 2014). These scenarios are developed for the same 13 weather station used for the historical assessment. The climate change scenarios are developed for mid-century 2050 (2036–2065) and end-century 2085 (2071–2100) periods. The reference period used for the scenarios is 1981–2010.
The KNMI’14 scenarios consist of four different scenarios based on future global warming and possible air circulation pattern changes. For this study, we selected two KNMI’14 scenarios as input for our climate change assessment: GL and WH. The GL scenario considers moderate temperature change based on low future emission and warming scenarios (+1°C for 2050 and +1.5°C for 2085) and the WH scenario considers high temperature change based on high emission and warming scenarios (+2°C for 2050 and +3.5°C for 2085). These scenarios were chosen to show extremes in the range of possible outcomes (the GL scenario with the least amount of change and WH with the highest degree of change). The climate scenarios used here are not based on downscaled climate model output, but on transformations of observed historical timeseries. Based on the analyses of regional and global climate models, transformation functions were developed. These functions were then used to transform the observations from different weather stations to develop the future climate scenarios. More detailed information about the KNMI’14 scenarios can be found in van den Attema et al. (2014).
The KNMI’14 scenarios do not include all weather variables for all seasons. Precipitation and temperature are provided for all four seasons, wind speed for the winter months, while relative humidity is missing altogether. In these cases, values of historical weather variables were applied from the reference period.
FWI and FFMC calculations, and thresholds for elevated fire danger
In this study, we focused on the Fire Weather Index (FWI) and the Fine Fuel Moisture Code (FFMC) component of the Canadian Fire Weather Index (CFWI). FWI and FFMC is calculated based on 24-h average wind speed, humidity, temperature and precipitation. This deviated from the standard procedure for calculating CFWI, but followed the procedure described by Arnell et al. (2021) and Perry et al. (2022). To initialise the calculations for FWI and FFMC for the period 1981–2020, we followed the procedure outlined by Lawson and Armitage (2008). The year 1980 was used to initialise the FWI system values, and the results from this year were excluded from further analysis. For subsequent years, fuel moisture codes for 31 December were used as the input for fuel moisture code calculation on 1 January. We calculated the first FWI and FFMC values by adopting the starting values from Lawson and Armitage (2008). However, the starting values are bound to seasons. In contrast, our study calculated daily FWI values for whole years.
This analysis resulted in a time series of daily FWI and FFMC values for the period 1981-2020 for each of the 13 meteorological stations (Fig. 1). For pragmatism, the period will still be referred to as 1981–2020. The 2017–2021 subset of this time series was subsequently matched with fire occurrences to classify what constitutes an elevated fire danger day for each of the 13 meteorological stations. We used two approaches to determine thresholds for elevated fire danger days: (1) the one-fire-a-day threshold following Karali et al. (2013), considering the FWI or FFMC threshold when at least one wildfire a day occurs, and (2) the percentage-based threshold following Andrews et al. 2003, considering the FWI or FFMC threshold for which a high percentage of wildfires occurred (80% in the case of De Jong et al. 2016). We considered not one but three percentages, namely 70, 80 and 90% of fires occurring above a given FWI or FFMC threshold. As such, this sensitivity analysis considered four fire danger day thresholds (the one-fire-a-day threshold, and three percentage-based thresholds) allowing analysis of the impact of the threshold chosen on the outcome of our study and increasing robustness of this work. We determined thresholds for the spring (March, April and May) and summer season (June, July and August) when fire danger was expected to be the highest. Finally, the chosen threshold was applied to the historical and future time series of FWI and FFMC to quantify the number of fire danger days. Root mean square errors were calculated comparing the elevated fire danger days for reference period and the future scenarios (Supplementary material Tables S4 and S5).
Results
Wildfire occurrences and thresholds for the period 2017–2021
Firstly, we determined thresholds at which elevated fire danger conditions occurred for the FWI and FFMC. During the 2017–2021 period, 70% of wildfires occurred at FFMC ≥81.3 (Fig. 2a) and FWI ≤3.23 (Fig. 2b). Considering the minimum and maximum number of fires that occur for the range in FWI and FFMC values, a different picture emerges. Fig. 3a and b show that fires occur at nearly all FWI and FFMC values, also the very low ones, but days with FWI ≥9 and FFMC ≥85 always have more than one fire a day, with the minimum number of fires per day increasing above those thresholds for both FFMC (Fig. 3a) and FWI (Fig. 3b). When comparing this for the FFMC for the spring and summer period, the threshold is at 83 and 84, respectively. For the FWI, the threshold is consistently at nine for wildfire occurrences recorded between 2017 and 2021 for the total, spring and summer periods (see Fig. 4).
Percentage of wildfires that occurred versus (a) FFMC, and (b) FWI index values. The total number of wildfire occurrences over this period were 2760. The number of wildfires is on the y-axis, the accumulated percentage of wildfires on the secondary axis and (a) FFMC, and (b) FWI on the x-axis.
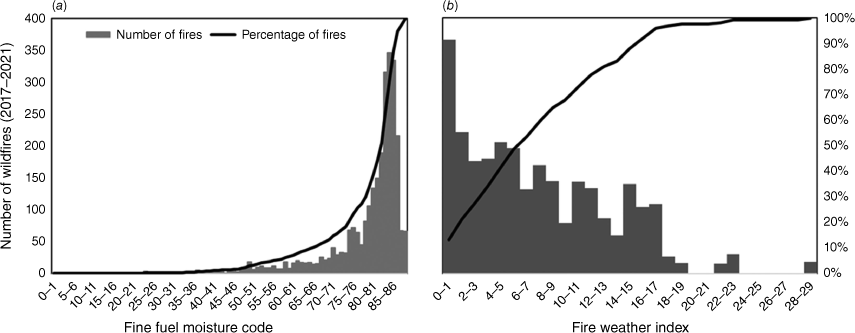
Graphs of the one-fire a day method for the average threshold over the Netherlands according to (a) FFMC, and (b) FWI from fire occurrences for the period 2017–2020. The average number of fires per day and minimum and maximum number of fires per day at each threshold is shown. Where both a maximum and minimum occurs, it means that there is always more than one fire at that threshold. Fig. 3a shows the full range of FFMC values and also the focussed range of 81–89 where most of the wildfires occurred.
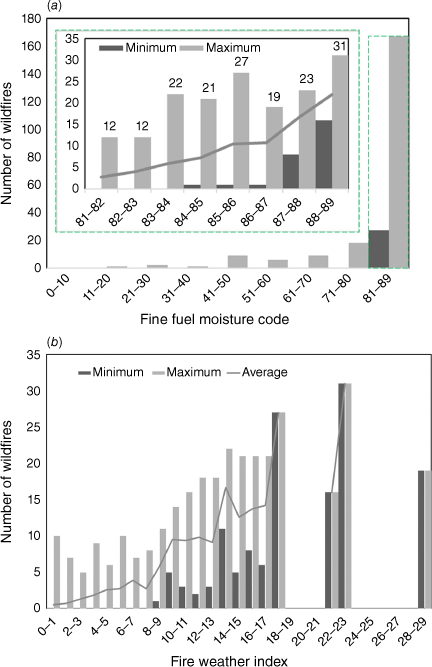
Graphs of seasonal analysis of the one-fire a day method for the average threshold over the Netherlands. It is shown for the FFMC for (a) Spring, and (b) Summer, and FWI for (c) Spring, and (d) Summer from fire occurrences for the period 2017–2020. The average number of fires per day and minimum and maximum number of fires per day at each threshold is shown. Where both a maximum and minimum occurs, it means that there is always more than one fire at that threshold.
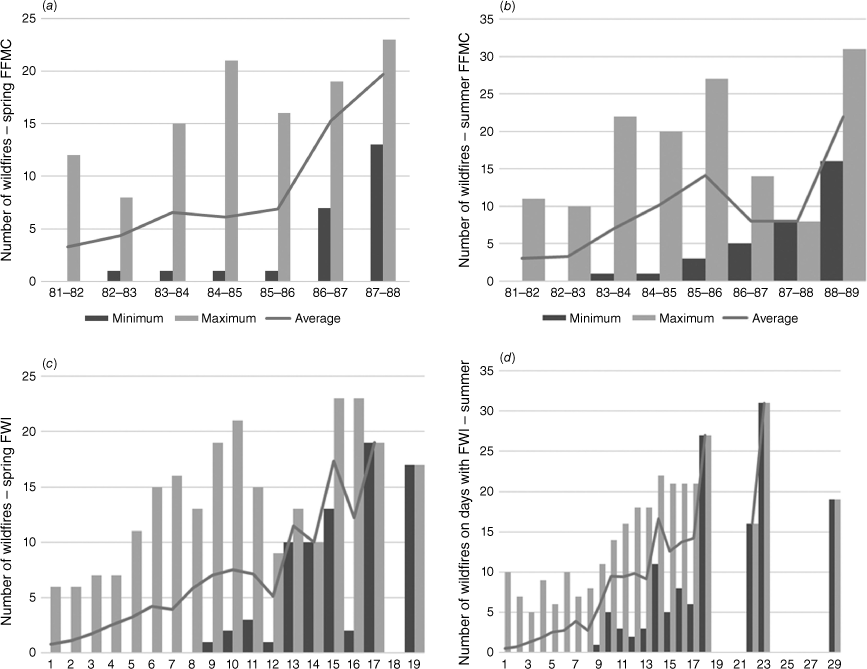
The threshold for an elevated fire danger day was determined for both the FWI and FFMC using the one-fire-a-day and percentage-based approaches. We focus our analysis on the FFMC. The threshold selection and the historical and future fire danger days results are shown for both the FWI and FFMC.
The FWI threshold was found to be at FWI ≥9 for the one-fire-a-day method and at FWI 3.23, 1.75, and 0.79 (70, 80 and 90%), respectively, for the percentage-based method (Fig. 2b, Table 1). The thresholds for the one-fire-a-day method for FFMC was found to be at FFMC ≥85 and 81.3, 77.2, and 66.4 (70, 80 and 90%), respectively, for the percentage-based method (Fig. 2a, Table 1). A threshold of FWI ≥9 and FFMC ≥85 were used for elevated fire danger threshold.
Historical elevated fire danger days according to FFMC
Fig. 4 shows the variability of the annual elevated fire danger days for the period 1981–2020 for each weather station. Fig. 5 presents results for the historical period (1981–2020) in average annual days at elevated fire danger days for each weather station of the Netherlands for four decades (1981–2020) according to the FFMC. Weather stations in the south saw the greatest increase in number of days at elevated fire danger while the northern weather stations experienced the smallest increase in the number of elevated fire danger days over the past four decades for both indices. The northern and coastal weather stations of De Kooy, Rotterdam, Leeuwarden, Schiphol and Vlissingen are the only weather stations that experienced a greater increase in the number of days at elevated fire danger for the FWI than the FFMC.
The annual number of days above thresholds at 13 KNMI weather stations in the Netherlands for the period 1981–2020 with average days above threshold for the period 1981–2010 shown in orange line.
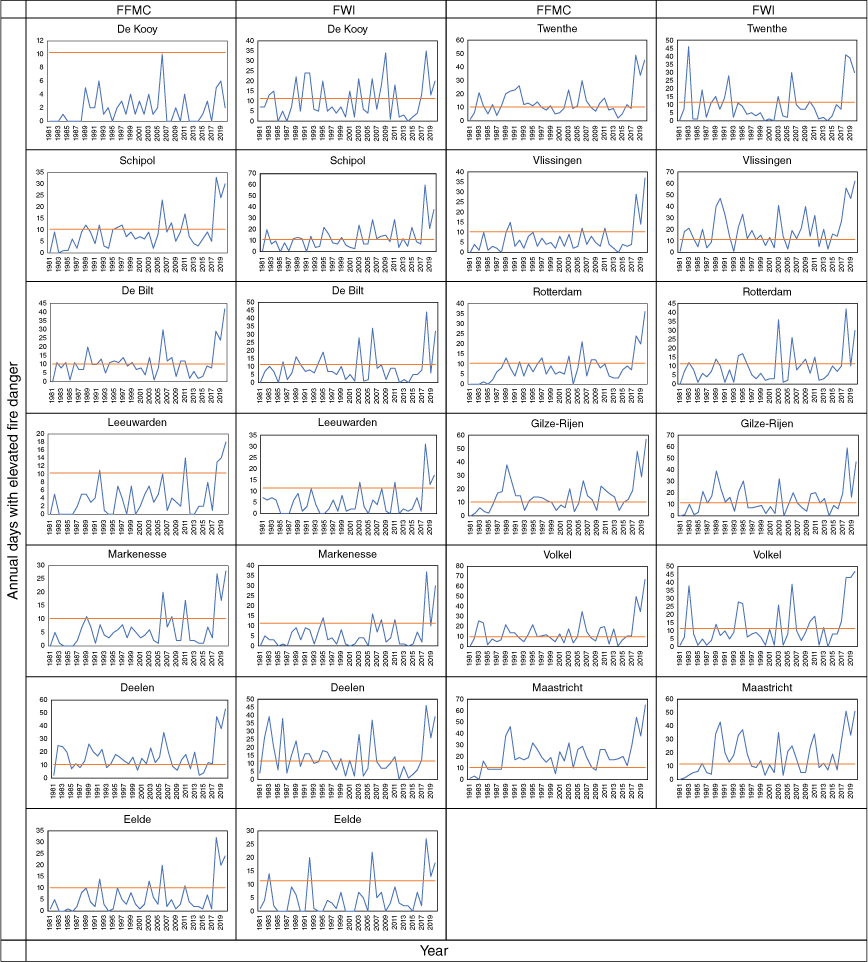
Maastricht, Schiphol, Leeuwarden, experienced a doubling in the number of days at elevated fire danger from 1981–1990 to 2011–2020. Gilze-Rijen and Volkel in the south nearly doubled in the number of elevated fire danger days, while this number of days nearly tripled at the Markenesse weather station from the first to last decade considered in the historical period (1981–2020).
In general, the FFMC showed more days at elevated fire danger than for the FWI. Deelen, Twenthe and Vlissingen had a higher number of days at elevated fire danger for the 1981–1990 decade than the following decades. From the 1981–1990 decade until the 2011–2020 decade, the number of days with elevated fire danger has steadily increased for all weather stations considering both indices (excluding for FWI for the stations mentioned earlier). The decade of 2011–2020 saw the greatest increase in the number of days at elevated fire danger.
Future annual elevated fire danger days according to FFMC
Fig. 6 depicts the changes in the future days at elevated fire danger according to the FFMC compared to the historical averages (period 1981–2020). Under the limited climate change - GL scenario, there is no substantial change in future evelated fire danger days. For the rapid climate change - WH scenarios, the days with elevated fire danger does substantially increase, almost doubling towards the end of the century. The number of days with elevated fire danger increased more for the summer period than for the spring period.
The average annual elevated fire danger days per decade (a) 1981–1990, (b) 1991–2000, (c) 2001–2010, and (d) 2011–2020 for the historical period, based on FFMC.
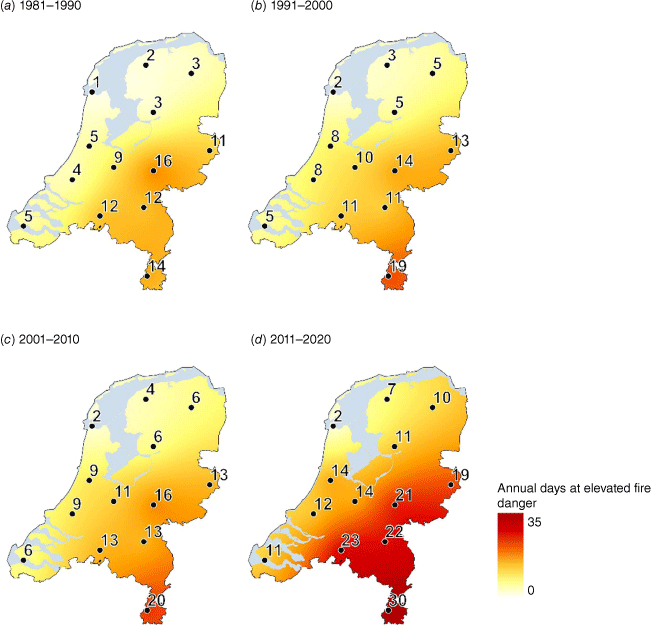
The average annual elevated fire danger days per decade according to the FFMC for the future KMNI scenarios: (a) Reference period 1981–2010, (b) GL 2036–2065, (c) GL 2071–2100, (d) WH 2036–2065, and (e) WH 2071–2100.
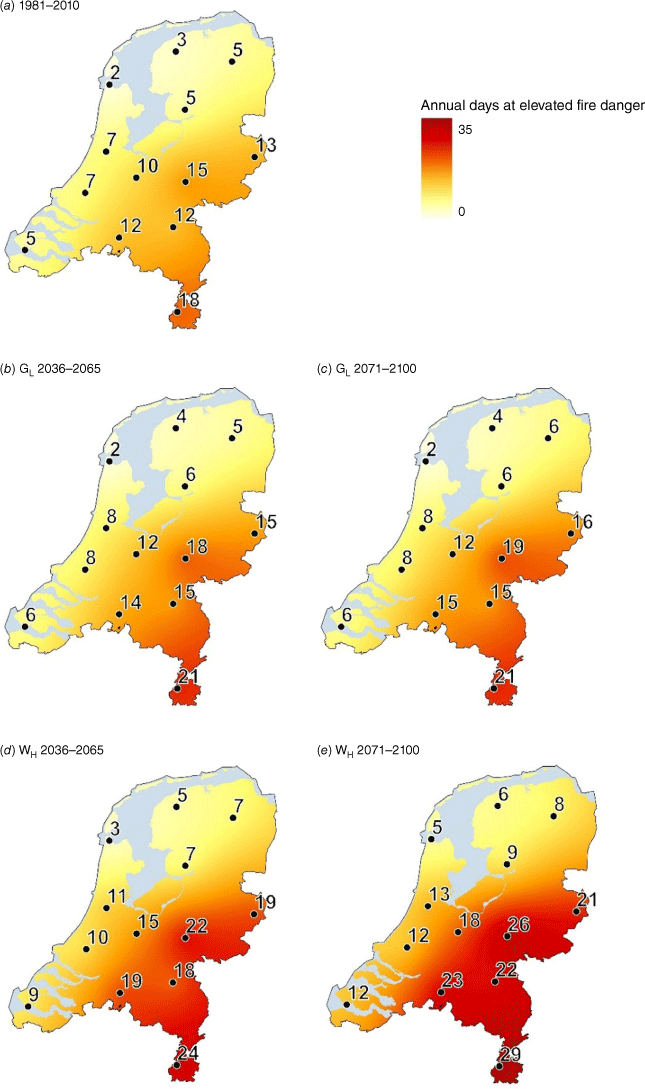
The biggest increase in elevated fire danger days according to the high scenario (WH) will be in the southern and central areas when compared to the historical elevated fire danger days (1981–2020). In the northern part of the county, there is only a small increase in the number of days with elevated fire danger with most weather stations doubling or almost doubling in the number of days at elevated fire danger. The southern part of the country, however, shows a much greater increase in the days with elevated fire danger. The number of elevated fire danger days almost doubled for most of the southern weather stations under the WH scenarios. When the WH scenarios are considered, the biggest increases are found in Maastricht changing from 17–24 to 28, Gilze-Rijen from 12–19 to 23 and for Volkel from 12–18 to 22 and Gelderland from 15–22 to 25 for the historical, 2050 and 2085 scenarios, respectively. The root mean squared errors when comparing the days at elevated fire danger for the historical period (1981–2020) to the KMNI scenarios for each of the weather stations, ranged between 0.8375–4.5432 for FFMC and 0.2205–1.7079 2085 for FWI.
When considering the seasonal breakdown of annual days at elevated fire danger, the increase in days at elevated fire danger does not change much for spring when compared to the historical period for both the FFMC (Table 2) and FWI (Table 3). It is striking that for both the FFMC and FWI the days at elevated fire danger increases considerably more during the summer period than during spring. The southern weather stations saw a greater increase in the number of elevated fire danger days during summer for both the FFMC and FWI for all scenarios when compared to the historical period.
Weather station | Historical period (1981–2020) | Scenario | |||||||||
---|---|---|---|---|---|---|---|---|---|---|---|
GL 2050 | WH 2050 | GL 2085 | WH 2085 | ||||||||
Spring | Summer | Spring | Summer | Spring | Summer | Spring | Summer | Spring | Summer | ||
De Kooy, 235 | 1 | 1 | 1 | 1 | 1 | 2 | 1 | 1 | 1 | 4 | |
Schiphol, 240 | 4 | 4 | 4 | 4 | 5 | 6 | 4 | 4 | 5 | 7 | |
De Bilt, 260 | 4 | 5 | 5 | 7 | 5 | 9 | 5 | 7 | 6 | 12 | |
Leeuwarden, 270 | 2 | 2 | 2 | 2 | 2 | 3 | 2 | 2 | 3 | 4 | |
Markenesse, 273 | 3 | 2 | 3 | 3 | 3 | 4 | 3 | 3 | 4 | 5 | |
Deelen, 275 | 6 | 9 | 7 | 10 | 8 | 14 | 8 | 11 | 8 | 17 | |
Eelde, 280 | 2 | 2 | 3 | 3 | 3 | 4 | 3 | 3 | 3 | 4 | |
Twenthe, 290 | 5 | 7 | 7 | 8 | 7 | 12 | 7 | 9 | 7 | 14 | |
Vlissingen, 310 | 2 | 3 | 2 | 4 | 2 | 7 | 2 | 4 | 3 | 9 | |
Rotterdam, 344 | 3 | 4 | 3 | 4 | 3 | 6 | 3 | 4 | 4 | 8 | |
Gilze-Rijen, 350 | 5 | 7 | 5 | 9 | 6 | 14 | 5 | 9 | 7 | 16 | |
Volkel, 375 | 5 | 7 | 5 | 9 | 5 | 12 | 5 | 9 | 6 | 16 | |
Maastricht, 380 | 6 | 11 | 7 | 13 | 7 | 16 | 7 | 13 | 8 | 19 |
Weather station | Historical period (1981–2020) | Scenarios | |||||||||
---|---|---|---|---|---|---|---|---|---|---|---|
GL 2050 | WH 2050 | GL 2085 | WH 2085 | ||||||||
Spring | Summer | Spring | Summer | Spring | Summer | Spring | Summer | Spring | Summer | ||
De Kooy, 235 | 2 | 7 | 3 | 8 | 3 | 14 | 3 | 8 | 3 | 18 | |
Schiphol, 240 | 4 | 6 | 5 | 11 | 5 | 12 | 5 | 11 | 5 | 17 | |
De Bilt, 260 | 2 | 6 | 7 | 15 | 3 | 12 | 7 | 15 | 4 | 15 | |
Leeuwarden, 270 | 2 | 2 | 3 | 5 | 3 | 6 | 3 | 5 | 3 | 9 | |
Markenesse, 273 | 2 | 2 | 5 | 7 | 3 | 7 | 5 | 7 | 4 | 9 | |
Deelen, 275 | 4 | 10 | 7 | 17 | 5 | 16 | 7 | 17 | 6 | 19 | |
Eelde, 280 | 2 | 2 | 4 | 6 | 3 | 5 | 4 | 6 | 3 | 7 | |
Twenthe, 290 | 2 | 7 | 7 | 16 | 3 | 12 | 7 | 16 | 4 | 15 | |
Vlissingen, 310 | 3 | 12 | 4 | 13 | 4 | 22 | 4 | 13 | 5 | 26 | |
Rotterdam, 344 | 2 | 6 | 5 | 12 | 3 | 12 | 5 | 12 | 3 | 16 | |
Gilze-Rijen, 350 | 2 | 9 | 6 | 17 | 4 | 15 | 6 | 17 | 4 | 19 | |
Volkel, 375 | 3 | 8 | 6 | 18 | 3 | 14 | 6 | 18 | 4 | 17 | |
Maastricht, 380 | 4 | 10 | 7 | 18 | 5 | 16 | 7 | 19 | 5 | 20 |
Further, it is noteworthy that the days with elevated fire danger days for the last decade of the historical period (2011–2020) already exceeds the projected elevated fire danger days, e.g. the elevated fire danger days for the 2050 WH scenario for Maastricht are 28, whereas it was 30 days for the last decade of the historical period (2011–2020, Fig. 6). This is the case for most weather stations, but especially for those in the southeast of the Netherland.
In contrast to this, the future scenarios for the FWI gives higher number of days with elevated fire danger at most stations or seasons when compared to the historical period (Fig. 6). This is especially the case for the coastal weather stations. The number of days at elevated fire danger increased under the GL scenarios for both time periods, seasons at all weather stations. A similar trend was observed for the WH scenario. The number of days with elevated fire danger increases more for the summer than the spring under both scenarios. The biggest increases were observed in the southern part of the Netherlands, although also the north of the Netherlands showed increased number of days at elevated fire danger compared to the FFMC. The Vlissingen weather station showed the highest increase in days at elevated fire danger changing from 16.9–28.9 to 34.5 from historical average to end of the century under the WH scenario. The other coastal weather stations also have higher increases in days with elevated fire danger for all scenarios and periods.
Discussion
Threshold selection
To determine the number of days at elevated fire danger for the historical period and future scenarios, a threshold at which this occurs had to be determined. The threshold is based on 5 years of data from 2017 until 2021. Regardless of the threshold that is used and the scenario, the number of future elevated fire danger days increased compared to the historical period (Supplementary Tables S2, S3). While acknowledging the sensitivity of the threshold chosen in defining the number of days with elevated fire danger, we selected the one-fire-a-day threshold as a basis for the historic and future elevated fire danger projections. This method was utilised to avoid overestimation of the days at elevated fire danger. This means that a day with elevated fire danger was defined for FFMC ≥85 and FWI ≥9, and these thresholds were used to calculate the number of days at elevated fire danger. Both these determined thresholds are in line with thresholds found in previous studies. The threshold for the FFMC at 85 is in line with the threshold identified in other studies 84.9 (Dimitrakopoulos et al. 2011) and 86.3 (Giannaros et al. 2021). This is also in line with findings from Taylor et al. (2021) who found most fires in Scotland, the UK and northern Europe occurred at an FFMC that was classified by European Forest Fire Information System (EFFIS) as being ‘Very low’ (<82.7) and ‘Low’ (82.7–86.1) risk. Most fires occurred at an FFMC of less than 86 in late winter and above 86 in late spring/summer period according to their findings. Thresholds are well aligned to that utilised by the Met Office for England and Wales for the FWI and FFMC (Perry et al. 2022). Days with values exceeding these thresholds will be at high fire danger and thus have an elevated fire danger. The thresholds at 9.38 and 84 marked the lowest end of the high fire danger classes for FWI and FFMC, respectively. In a study of the effect of climate change on the fire danger in the UK, Arnell et al. (2021) used thresholds for FFMC of 86.6–88.5 for May to June and 86.8–90.4 for July to August and for FWI at 15.3–25.4 for May to June and 17.0–35.7 for July to August. The thresholds chosen represent moderately high fire danger, but some other studies have used more extreme thresholds. The thresholds identified in this study are consistently lower than thresholds identified by Arnell et al. (2021). This suggests that fires in The Netherlands occur at lower FFMC and FWI threshold than in the UK. Giannaros et al. (2021) considered FWI >11.2 to be elevated fire danger. The threshold determined for FWI in this study is consistently lower than that of the studies by Arnell et al. (2021), Giannaros et al. (2021) and Perry et al. (2022), suggesting that fire occurs at a lower threshold in the Netherlands.
Changes in elevated fire danger days over the last 40 years
Considering the period 1981–2020, there is a clear increasing trend of elevated fire danger days from the first to the last decade. This was the case for both the FWI and FFMC. Trends were consistent across all the weather stations used. After four decades, the central part of the Netherlands is experiencing the same number of elevated fire danger days as the southernmost province of Limburg did at the beginning of the historical period. This points to a potential increase of the length of the fire season and northwards shift of higher fire danger. This is in line with projections that fire regimes will shift north with climate change (De Rigo et al. 2017; Galizia et al. 2021) and that eleavted fire danger days will increase for the region (De Rigo et al. 2017). The changes over the historical period are also in line with Jolly et al. (2015) who found that globally the fire season length increased over the period 1979–2013. De Rigo et al. (2017) also found that areas experiencing lower moisture conditions would shift further northwards from the Mediterranean to Northern Europe. Over the period 1981–2020 all the stations saw an increase in elevated fire danger days for all the decades. Especially during the last decade (2011–2020) there has been a large increase in the number of days with elevated fire danger. This is in line with findings from De Rigo et al. (2017) and Jolly et al. (2015). The fire danger has changed with a northward shift of fire weather over the country over the historical period in this study as a result of climate change.
Future change in days with elevated fire danger
In the future, the number of days with elevated fire danger will increase under both KNMI scenarios used in this study. Under the most extreme WH scenario, the number of days will increase more than for the GL scenario. The number of days at elevated fire danger will increase more towards the end of the century. According to the scenarios, a limitation of future greenhouse gas emissions (GL scenarios) will limit the increase in days with elevated fire danger.
In the future, all regions of the Netherlands are expected to experience an increase in the number of elevated fire danger days compared to the average for the historical period. The lowest increase in fire danger days will be in the north of the country and the greatest increase in regions in the central and southern regions of the Netherlands. There is a clear increasing trend in the number of fire danger days for the Netherlands in the future, especially in the southeast side of the country that borders Belgium and Germany.
It is also notable that the elevated fire danger days for the 2085 KNMI’14 WH scenario are expected to have doubled in most regions in the Netherlands when compared to the historical reference period (1981–2010). In the northern provinces, this increase in elevated fire danger days means that under the future scenarios, it will experience the same number of fire danger days as North-Brabant experienced in the historical reference period (1981–2010). Following our analysis, irrespective of of the scenario used, fire danger is expected to increase in the future. A study in Germany found a prolonging of the potential fire season under a 2 and 3°C increase in temperatures in future (Holsten et al. 2013).
When the elevated fire danger days for the future scenarios are compared to the 2011–2020 period, the values are remarkably similar. Already from the past four decades, the steady increase in elevated fire danger days (Fig. 4) can be seen as a result of climate change. Considering the limitations in data and methods, the thresholds are underestimated and thus days with elevated fire danger could increase more in the future than presented here. Root square mean errors for FWI and FFMC showed that the scenarios are a good predictor of future days at elevated fire danger.
It is noteworthy that when comparing the FFMC for the last decade (2011–2020) in Fig. 5 with the future scenarios (Fig. 6), the days at elevated fire danger for FFMC look similar to the 2050 KNMI GL, 2050 KNMI WH and 2085 KNMI GL scenarios. The last decade has been one of the driest decades on record for the Netherlands (Vautard et al. 2020). The results showed that the past decade (2011–2020) already surpassed the figures predicted for the GL scenarios. This could indicate that future climate scenarios are underestimating future changes and that climate change might be progressing more rapidly than anticipated by the KNMI’14 scenarios. van Oldenborgh et al. (2009) and Vautard et al. (2020) observed that trends in temperature increase are much larger in Western Europe than those in current climate models. The datasets and scenarios of the KNMI are easy to use and readily available, but underestimates future days at elevated fire danger.
When considering the seasonal breakdown of future days with elevated fire danger over the springtime, FWI generally shows less days and FFMC more days with elevated fire danger. The inverse is true for summertime where there is generally more annual days with elevated fire danger for the FWI and less for the FFMC. Studies focusing on the United Kingdom (De Jong et al. 2016; Arnell et al. 2021) report FFMC to be a better index for spring wildfires, and FWI better for summer wildfires. Our results indicate that this also applies to the Netherlands.
It can be seen from our results that there is a general increase in the number of elevated fire danger days in the past 40 years and also with future scenarios until the end of the century. This is in line with other studies globally, and in Europe that points towards increased fire danger. Forest fires are to increase in the future (Bowman et al. 2017; Moritz et al. 2014). Increase in elavted fire danger days in future are in line with Moriondo et al. (2006). de Dios et al. (2021) finding that Pyrenean forests may be on the brink of a major change in fire regime. They further hypothesised that their findings are likely applicable to the Alps of Europe and forest areas of Central Europe.
Limitations of the current study
Our results clearly show that climate change is expected to increase fire danger in the Netherlands. Given the methodology that was followed, the number of days at elevated fire danger presented here for the future period are probably an underestimation of the actual number of elevated fire danger days.
Firstly, inputs for the current research consisted of weather information, wildfire registrations and KNMI’14 scenarios. Regarding the KNMI’14 scenarios, it should be noted that: first, these scenarios do not include all weather variables. While precipitation and temperature are provided for all four seasons, windspeed is not provided for summer periods and the relative humidity data are not at all available. In these cases, historical weather variables’ values from reference period (1981–2010) were applied if the information was not available. Relative humidity is a good predictor of fire weather in Germany (Wittich 1998; Holsten et al. 2013), and a study by White and Silva (2021) found that the variable with the greatest influence on the FFMC was the relative humidity. The exclusion of a change in the variable relative humidity in KNMI’14 scenarios may currently point to an underestimation of the fire danger as relative humidity could reduce in the future, especially during warm and dry periods. To more accurately determine the number of days with elevated danger, inclusion of year-round relative humidity and windspeed variables in climate scenarios can mean that future fire weather and resulting elevated fire danger days can be more accurately modelled for each season, allowing for better planning and preparation for future wildfire conditions.
Secondly, the lack of wildfire registrations on which the elevated fire danger threshold of at least one fire a day is based on only 5 years (2017–2021) of wildfire occurrence data was available for our analyses. A longer period could have potentially yielded a more robust threshold and a better estimation of the number of elevated fire danger days. A study in the United Kingdom by De Jong et al. (2016) based their results on only 3 years of data. Also, the distribution of different weather conditions over the 5 years makes our data set diverse in weather conditions. However, the discussion about threshold selection underscores the main conclusion of this study, that the number of days with elevated fire danger is increasing due to recent and future climate change, is robust and not sensitive to the exact determination of the thresholds.
Thirdly, the use of average instead of 12 pm temperatures as input for the FWI and FFMC mean that the temperature might be underestimated. The values for the average temperature may be lower than for maximum temperature values.
Practical implications and future directions
Results suggest that the last decade may serve as a preview of future fire danger conditions in the Netherlands when compared to scenarios. Although all scenarios showed an increase in days with elevated fire danger under reduced emissions (+1°C for 2050 and +1.5°C for 2085), there will be limited increases in the number of days with elevated fire danger, while no reduction in emissions (+2°C for 2050 and +3.5°C for 2085) may result in a greater number of days with elevated fire danger in the Netherlands. This assessment, however, does not account for all potential extreme weather days in the future. Further, KNMI scenarios need to be adapted to include the humidity and windspeed all year round.
While future fire weather conditions may not reach the severity experienced to date in Mediterranean Europe, considering fuel conditions and population density in the Netherlands, conditions conducive to wildfire occurrence are likely to increase. Disaster risk management efforts should prioritise actions such as landscape management, wildfire risk awareness and education (Lambrechts et al. 2023). Drawing insights from the Dutch experience in water management can inform governance strategies for wildfires in the context of climate change (Lambrechts et al. 2023). This proactive approach extends beyond the Netherlands; countries in Northwestern Europe should also prepare for a rise in future elevated fire danger days.
Conclusion
In conclusion, our analysis, taking all variables into account, indicates a consistent trend of increasing days with elevated fire danger over the past four decades in the Netherlands, with FFMC better reflecting elevated fire danger than the FWI code. This upward trajectory is anticipated to persist in the future under human-induced climate change. Future scenarios project a further increase in fire danger, particularly towards the end of the century. Notably, the central and southern regions of the Netherlands are expected to experience a more pronounced increase in annual days at elevated fire danger.
Crucially, our findings indicate that the current KNMI'14 scenarios may be underestimating future fire danger in the Netherlands as end of century results are similar to what has occurred in the last decade (2011–2020). This underscores the necessity for heightened preparedness in the Netherlands and Northwestern Europe. There is an urgent need for the development of strategies and policies to adapt to and mitigate the risks and impacts of future wildfires on the environment, recreational areas, and civil society. In the light of these findings, it is imperative for stakeholders and policymakers to collaboratively work towards enhancing wildfire prevention, preparedness and response measures. By anticipating and addressing the challenges posed by increasing fire danger, the Netherlands and Northwestern Europe can proactively safeguard their communities and natural landscapes. This underscores the importance of ongoing research, adaptive policies and international cooperation to effectively manage the evolving threat of wildfires in the face of climate change.
Data availability
The data that support this study will be shared upon reasonable request to the corresponding author.
Declaration of funding
This research received funding from the PyroLife project, funded by the European Union’s Horizon 2020 research and innovation programme MSCA-ITN-2019 – Innovative Training Networks, under grant agreement No. 860787.
Acknowledgements
We would like to acknowledge the contribution of various colleagues within the Water Systems and Global Change group at Wageningen University, Jelmer Dam and Edwin Kok from the Netherlands Institute for Public Safety. Marc Castellnou from the Catalan Fire and Rescue Service and Kerryn Little within the PyroLife network for their ideas and discussions about the topic that contributed to this manuscript.
References
Abatzoglou JT, Williams AP, Boschetti L, Zubkova M, Kolden CA (2018) Global patterns of interannual climate–fire relationships. Global Change Biology 24(11), 5164-5175.
| Crossref | Google Scholar | PubMed |
Andrews PL, Loftsgaarden DO, Bradshaw LS (2003) Evaluation of fire danger rating indexes using logistic regression and percentile analysis. International Journal of Wildland Fire 12(2), 213-226.
| Crossref | Google Scholar |
Arnell N, Freeman A, Gazzard R (2021) The effect of climate change on indicators of fire danger in the UK. Environmental Research Letters 16(4), 044027.
| Crossref | Google Scholar |
Bedia J, Golding N, Casanueva A, Iturbide M, Buontempo C, Gutiérrez JM (2018) Seasonal predictions of Fire Weather Index: paving the way for their operational applicability in Mediterranean Europe. Climate Services 9, 101-110.
| Crossref | Google Scholar |
Belcher C, Brown I, Clay G, Doerr S, Elliott A, Gazzard R, Kettridge N, Morison J, Perry M, Santin C (2021) UK wildfires and their climate challenges: Expert Led Report Prepared for the third Climate Change Risk Assessment. Available at https://www.ukclimaterisk.org/wp-content/uploads/2021/06/UK-Wildfires-and-their-Climate-Challenges.pdf
Bowman DMJS, Williamson GJ, Abatzoglou JT, Kolden CA, Cochrane MA, Smith AMS (2017) Human exposure and sensitivity to globally extreme wildfire events. Nature Ecology & Evolution 1(3), 0058.
| Crossref | Google Scholar | PubMed |
Davies GM, Legg CJ (2016) Regional variation in fire weather controls the reported occurrence of Scottish wildfires. PeerJ 4, e2649.
| Crossref | Google Scholar | PubMed |
Davies MG, Gray A, Hamilton A, Legg CJ (2008) The future of fire management in the British uplands. The International Journal of Biodiversity Science and Management 4(3), 127-147.
| Crossref | Google Scholar |
de Dios VR, Hedo J, Camprubí ÀC, Thapa P, Del Castillo EM, de Aragón JM, Bonet JA, Balaguer-Romano R, Díaz-Sierra R, Yebra M (2021) Climate change induced declines in fuel moisture may turn currently fire-free Pyrenean mountain forests into fire-prone ecosystems. Science of The Total Environment 797, 149104.
| Crossref | Google Scholar |
de Groot WJ, Goldammer JG, Keenan T, Brady MA, Lynham TJ, Justice CO, Csiszar IA, O’Loughlin K (2006) Developing a global early warning system for wildland fire. Forest Ecology and Management 234(1), S10.
| Google Scholar |
De Jong MC, Wooster MJ, Kitchen K, Manley C, Gazzard R, McCall FF (2016) Calibration and evaluation of the Canadian Forest Fire Weather Index (FWI) System for improved wildland fire danger rating in the United Kingdom. Natural Hazards and Earth System Sciences 16(5), 1217-1237.
| Crossref | Google Scholar |
Dimitrakopoulos A, Bemmerzouk A, Mitsopoulos I (2011) Evaluation of the Canadian fire weather index system in an eastern Mediterranean environment. Meteorological Applications 18(1), 83-93.
| Crossref | Google Scholar |
Donkers H (2018) Vuur en natuur. Succesvolle bestrijding leidt tot meer bosbranden. Available at https://repository.ubn.ru.nl/bitstream/handle/2066/183897/183897.pdf
Dupuy J-l, Fargeon H, Martin-StPaul N, Pimont F, Ruffault J, Guijarro M, Hernando C, Madrigal J, Fernandes P (2020) Climate change impact on future wildfire danger and activity in southern Europe: a review. Annals of Forest Science 77(2), 35.
| Crossref | Google Scholar |
Ellis TM, Bowman DM, Jain P, Flannigan MD, Williamson GJ (2022) Global increase in wildfire risk due to climate‐driven declines in fuel moisture. Global Change Biology 28(4), 1544-1559.
| Crossref | Google Scholar | PubMed |
Galizia LF, Curt T, Barbero R, Rodrigues M (2021) Understanding fire regimes in Europe. International Journal of Wildland Fire 31(1), 56-66.
| Crossref | Google Scholar |
Gazzard R, McMorrow J, Aylen J (2016) Wildfire policy and management in England: an evolving response from Fire and Rescue Services, forestry and cross-sector groups. Philosophical Transactions of the Royal Society B: Biological Sciences 371(1696), 20150341.
| Crossref | Google Scholar | PubMed |
Giannakopoulos C, Karali A, Roussos A, Hatzaki M, Xanthopoulos G, Kaoukis K (2011) Evaluating present and future fire risk in Greece. Advances in Remote Sensing and GIS Applications in Forest Fire Management From Local to Global Assessments 181. https://op.europa.eu/en/publication-detail/-/publication/5e90be79-efe8-43dd-8670-2518a43155f4.
| Google Scholar |
Giannaros C, Melas D, Giannaros TM (2019) On the short-term simulation of heat waves in the Southeast Mediterranean: sensitivity of the WRF model to various physics schemes. Atmospheric Research 218, 99-116.
| Crossref | Google Scholar |
Giannaros TM, Kotroni V, Lagouvardos K (2021) Climatology and trend analysis (1987–2016) of fire weather in the Euro‐Mediterranean. International Journal of Climatology 41, E491-E508.
| Crossref | Google Scholar |
Grillakis MG (2019) Increase in severe and extreme soil moisture droughts for Europe under climate change. Science of The Total Environment 660, 1245-1255.
| Crossref | Google Scholar | PubMed |
Holsten A, Dominic AR, Costa L, Kropp JP (2013) Evaluation of the performance of meteorological forest fire indices for German federal states. Forest Ecology and Management 287, 123-131.
| Crossref | Google Scholar |
Johnston LM, Wang X, Erni S, Taylor SW, McFayden CB, Oliver JA, Stockdale C, Christianson A, Boulanger Y, Gauthier S (2020) Wildland fire risk research in Canada. Environmental Reviews 28(2), 164-186.
| Crossref | Google Scholar |
Jolly W, Cochrane M, Freeborn P, Holden Z, Brown T, Williamson G, Bowman D (2015) Climateinduced variations in global wildfire danger from 1979 to 2013. Nature Communications 6(1), 7537.
| Crossref | Google Scholar |
Karali A, Hatzaki M, Giannakopoulos C, Roussos A, Xanthopoulos G, Tenentes V (2014) Sensitivity and evaluation of current fire risk and future projections due to climate change: the case study of Greece. Natural Hazards and Earth System Sciences 14(1), 143-153.
| Crossref | Google Scholar |
Kottek M, Grieser J, Beck C, Rudolf B, Rubel F (2006) World map of the Köppen-Geiger climate classification updated. Meteorologische Zeitschrift 15(3), 259-263.
| Crossref | Google Scholar |
Kovats R, Valentini R, Bouwer L, Georgopoulou E, Jacob D, Martin E, Rounsevell M (2014) Europe. In ‘Climate Change 2014: Impacts, Adaptation, and Vulnerability. Part B: Regional Aspects. Contribution of Working Group II to the Fifth Assessment Report of the Intergovernmental Panel on Climate Change’. (Eds VR Barros, CB Field, DJ Dokken, MD Mastrandrea, KJ Mach, TE Bilir, M Chatterjee, KL Ebi, YO Estrada, RC Genova, B Girma, ES Kissel, AN Levy, S MacCracken, PR Mastrandrea and LL White) (Cambridge University Press: Cambridge, UK and New York)
Lambrechts HA, Paparrizos S, Brongersma R, Kroeze C, Ludwig F, Stoof CR (2023) Governing wildfire in a global change context: lessons from water management in the Netherlands. Fire Ecology 19(1), 6.
| Crossref | Google Scholar |
Moriondo M, Good P, Durao R, Bindi M, Giannakopoulos C, Corte-Real J (2006) Potential impact of climate change on fire risk in the Mediterranean area. Climate Research 31(1), 85-95.
| Crossref | Google Scholar |
Moritz MA, Batllori E, Bradstock RA, Gill AM, Handmer J, Hessburg PF, Leonard J, McCaffrey S, Odion DC, Schoennagel T, Syphard AD (2014) Learning to coexist with wildfire. Nature 515(7525), 58-66.
| Crossref | Google Scholar | PubMed |
Palheiro PM, Fernandes P, Cruz MG (2006) A fire behaviour-based fire danger classification for maritime pine stands: comparison of two approaches. Forest Ecology and Management 234, S54.
| Crossref | Google Scholar |
Perry MC, Vanvyve E, Betts RA, Palin EJ (2022) Past and future trends in fire weather for the UK. Natural Hazards and Earth System Sciences 22(2), 559-575.
| Crossref | Google Scholar |
Rovithakis A, Grillakis MG, Seiradakis KD, Giannakopoulos C, Karali A, Field R, Lazaridis M, Voulgarakis A (2022) Future climate change impact on wildfire danger over the Mediterranean: the case of Greece. Environmental Research Letters 17(4), 045022.
| Crossref | Google Scholar |
San-Miguel-Ayanz J, Moreno JM, Camia A (2013) Analysis of large fires in European Mediterranean landscapes: lessons learned and perspectives. Forest Ecology and Management 294, 11-22.
| Crossref | Google Scholar |
Stocks BJ, Lawson B, Alexander M, Wagner CV, McAlpine R, Lynham T, Dube D (1989) The Canadian forest fire danger rating system: an overview. The Forestry Chronicle 65(6), 450-457.
| Crossref | Google Scholar |
Stoof C (2020) Nederland moet leren leven met vuur. (Wageningen University & Research) Available at https://www.naturetoday.com/intl/nl/nature-reports/message/?msg=26471
Stoof C, Kok E, Cardil A, Van Marle M (2024) In temperate Europe, fire is already here: the case of The Netherlands. AMBIO 53, 604-623.
| Crossref | Google Scholar |
Taylor S (2020) Atmospheric cascades shape wildfire activity and fire management decision spaces across scales− A conceptual framework for fire prediction. Frontiers in Environmental Science 8, 527278.
| Crossref | Google Scholar |
Taylor SW, Alexander ME (2006) Science, technology, and human factors in fire danger rating: the Canadian experience. International Journal of Wildland Fire 15(1), 121-135.
| Crossref | Google Scholar |
van Oldenborgh GJ, Drijfhout S, Van Ulden A, Haarsma R, Sterl A, Severijns C, Hazeleger W, Dijkstra H (2009) Western Europe is warming much faster than expected. Climate of the Past 5(1), 1-12.
| Crossref | Google Scholar |
Vautard R, van Aalst M, Boucher O, Drouin A, Haustein K, Kreienkamp F, Van Oldenborgh GJ, Otto FE, Ribes A, Robin Y, Schneider M (2020) Human contribution to the record-breaking June and July 2019 heatwaves in Western Europe. Environmental Research Letters 15(9), 094077.
| Crossref | Google Scholar |
Vitolo C, Di Giuseppe F, Krzeminski B, San-Miguel-Ayanz J (2019) A 1980–2018 global fire danger re-analysis dataset for the Canadian Fire Weather Indices. Scientific Data 6(1), 190032.
| Crossref | Google Scholar |
White BLA, Silva MFA (2021) Estimating fine dead fuel moisture content under equatorial CLIMATE conditions. FLORESTA 51(3), 696-702.
| Crossref | Google Scholar |
Wittich K (1998) Waldbrandgefahren-Vorhersage des Deutschen Wetterdienstes. AFZ/Der Wald 53(6), 321-324.
| Google Scholar |