Spatial and temporal opportunities for forest resilience promoted by burn severity attenuation across a productivity gradient in north western Patagonia
Florencia Tiribelli
A
B Present address:
Abstract
Fire regimes in many biomass-rich ecosystems worldwide are dominated by high-severity fires. Many of these systems lack fire-resistant traits or post-fire regeneration strategies. Understanding under which environmental and weather conditions they experience less severe fire is crucial for maintaining their persistence in the landscape.
Understand the spatial and temporal conditions that allow burn severity attenuation across Patagonia’s productivity gradient.
We modelled burn severity as a function of topography, weather, vegetation and productivity.
Low severity was a rare phenomenon, affecting only 8% of the areas burned. The probability of burning with high severity followed a hump-shaped relationship with productivity. Low severity occurred in fires that burned under cool and wet summer conditions in areas with sparser fuels or in wetter and more productive environments but with discontinuous and wet fuels.
Across the regional gradient, ecosystems of intermediate productivity generally lack conditions for low burn severity. Temporally, low burn severity occurs in smaller fires burning in productive ecosystems during cool and wet summers.
Future climate scenarios of increasing aridity and temperature in the region will disfavour conditions for low burn severity, thus promoting fire-mediated transitions from forests to alternative states dominated by more fire-adapted flammable species (e.g. shrublands).
Keywords: burn severity, ecosystems: temperate, fire severity, forest resilience, north west Patagonia, obligate seeders, productivity gradient, resproutes, shrublands.
Introduction
Fire regimes in many fuel-rich ecosystems worldwide are dominated by high-severity canopy-consuming (or canopy-killing) fires where low severity fires rarely take place. Although many of these systems have fire-adapted species highly resistant or resilient to severe fires (Jayen et al. 2006; Murphy et al. 2013; Palmer et al. 2018; Owen et al. 2020), others show high vulnerability to these disturbances due to the lack of fire-resistant traits or post-fire regeneration strategies (Holz et al. 2015; Kitzberger et al. 2016b; Landesmann et al. 2021). In these latter systems, it is critical to understand biophysical (bottom–up) and fire weather (top–down) conditions that lead to the attenuation of fire severity. These spatial and temporal drivers could help predict particular locations (e.g. fire refugia) or temporal opportunities (e.g. seasons or periods of particular mild fire weather conditions) where and when high burn severity is attenuated and fire-sensitive ecosystems are less vulnerable to fire, and produce an overall more resilient landscape response. Resilience here may be attained through regeneration either from surviving individuals in fire refugia, or from individuals that may have survived low-severity fires. Thus, it is imperative to understand under which environmental and weather conditions different communities have less severe responses to fire.
Vegetation burn severity, a measure of the immediate impacts of fire on the environment through aerial biomass consumption (Keeley 2009), largely depends on a complex interplay between bottom–up and top–down factors that interact at different scales. Fuel characteristics (amount, continuity and condition to burn) modified by local factors such as radiation, nearness to heat sinks and topography are examples of bottom–up controls of fire severity (Gill 1975; Keeley 2009; Bradstock et al. 2010; Murphy et al. 2013). Worldwide, it is still unclear under which fuel amounts and structures different vegetation types have less severe responses to fire (Parks et al. 2014, 2018; Lindenmayer et al. 2020, 2022; Bowman et al. 2021). In some systems, burn severity is believed to increase with fuel amount up to an asymptote, beyond which it is not possible for fires to burn more severely despite biomass increases (Murphy et al. 2013). However, the vegetation structure and fuel characteristics of highly productive systems such as broadleaf temperate forest or rain forest vegetation may lead to lower burn severity than less productive ecosystems, as happens with fire occurrence (Pausas and Paula 2012). In this case, severity peaks when the combined effects of fuel build-up and drought are maximised, and is reduced where shady environments of highly productive ecosystems generate moister and darker microclimatic conditions (He et al. 2019). Here, burn severity may not be a function of the amount of fuels available, but of the environmental conditions that these fuels experience, such as the structure of the vegetation, topography and weather. However, fuel characteristics are difficult to measure, and these data are not available across different vegetation types and fire seasons. Vegetation productivity is a proxy of fuel characteristics such as amount and condition where high temporal resolution fuel maps are not available. Understanding the relationship between burn severity and productivity across different vegetation types may be the first step towards understanding under which conditions different vegetation types experience less severe fires.
Fire weather is a top–down control of fire severity that can turn less flammable vegetation into highly flammable vegetation. However, it is unclear how variability in fire weather affects burn severity, a critical aspect in the current context of climate change (Hessl 2011). The effect of weather on burn severity depends on the vegetation involved, and the spatial and temporal scale at which the pattern is observed (Cansler and Mckenzie 2014; Abatzoglou et al. 2017; Bowman et al. 2021; Lindenmayer et al. 2021). Warmer and drier conditions can increase burn severity by producing environments conducive to high-intensity fires both between seasons and in individual fires in temperate forests (Abatzoglou et al. 2017; Tran et al. 2020; Bowman et al. 2021; Collins et al. 2022). When looking at the pattern across vegetation types, vegetation characteristics start to be more important than fire weather in driving fire severity patterns (Walker et al. 2020). Thus, the effect of weather on burn severity depends on fuels structure, loading and moisture, and the environmental conditions. In order to understand the role of weather on burn severity patterns, it is important to study several contrasting fire seasons along a representative geographic range of fuel characteristics and vegetation types. We propose that wetter and cooler fire weather conditions would promote lower burn severity in highly productive vegetation types where moister fuel condition and structure conditions act as fire retardants. In contrast, drier and hotter fire seasons would homogenise the landscape, creating conditions for high burn severity across all vegetation types. Finally, sites of intermediate productivity would be less sensitive to weather as fuel amount and conditions create fire-prone systems under any weather scenario.
Patagonia has one of the most striking productivity gradients on Earth, which provides a unique opportunity to test the importance of bottom–up and top–down controls of burn severity across contrasting vegetation types. At the most productive and fuel-rich end of the gradient grow temperate broadleaf forests dominated by fire-sensitive obligate seeders (e.g. Nothofagus dombeyi, N. pumilio) (Veblen et al. 2003; Kitzberger et al. 2005). These forests are most vulnerable to fire the first decades after fire owing to the high amount of continuous fine fuels exposed to desiccation. Once the trees overtop the understorey, vegetation fuel continuity and amount in this lower stratum decrease, increasing the chances of these forests burning with low severity (Paritsis et al. 2015; Tiribelli et al. 2018). After high-severity fires, tree species are unable to regenerate owing to lack of seed sources and/or adverse weather for seedling establishment, and forests shift to shrublands or grasslands (Landesmann et al. 2021). At intermediate portions of the gradient, mixed shrublands coexist in the landscape with dry forests. Shrublands are prone to crown-consuming severe fires, but owing to their resprouting capacity rapidly recover their initial structure (Paritsis et al. 2015; Tiribelli et al. 2018; Landesmann et al. 2021). Finally, dry forests are dominated by the fire-sensitive conifer Austrocedrus chilensis. Despite all this understanding of the interaction between flammability and vegetation, burn severity has only been assessed for individual fires under specific weather conditions (Assal et al. 2018; Landesmann et al. 2021; Franco et al. 2022).
Here, we seek to understand what are the spatial (bottom–up) and temporal (top–down) conditions that allow the fire-sensitive vegetation of Patagonia to burn with lower severity both at the landscape scale and for the main vegetation types in the region. Specifically, we ask: (i) how does the probability of burning with high severity change with climate, topography and distance to water bodies, and between vegetation types; (ii) how does burn severity change across Patagonian productivity gradients and does the pattern hold between vegetation types, and (iii) under different weather conditions? First, we propose that at the landscape scale, changes in productivity largely influence burn severity and we expect maximum burn severity at intermediate portions of the productivity gradient (dry forests and shrublands). Under lower productivity conditions (dry conditions, rocky/sandy sites), burn severity is attenuated owing to sparser fuel loads whereas in highly productive fuel-rich forest ecosystems, burn severity is attenuated owing to the dampening effect of microclimatic conditions on fuel moisture produced by denser tree canopies. Second, we propose that the effects of interannual fire weather variability on burn severity will be more pronounced in high-productivity ecosystems by modifying the attenuation effect of fuel moisture conditions. We expect that under dry/warm weather conditions, highly productive fuel-rich forest ecosystems will increase burn severity compared with wet/cool conditions more than dry forests or shrublands.
To answer these questions, we modelled burn severity as a function of biophysical predictors to find spatial opportunities for lower burn severity in the landscape. To understand burn severity patterns across the productivity gradient and under different vegetation types, we used vegetation cover and NDVI (Normalized difference vegetation index) as proxies for fuel characteristics and productivity. Finally, to find temporal opportunities for lower burn severity, we analysed burn severity–productivity patterns under contrasting fire weather conditions.
Methods
Study area
The study area extends 39–44°S along the Patagonian Andes, Argentina (Fig. 1). In this area, the vegetation varies along a steep precipitation gradient that extends from west to east (3000–800 mm year−1) and less markedly along a temperature gradient associated with elevation and slope exposure (Veblen et al. 1992). Here, we focus on six different community types: (1) wet closed canopy forest dominated by Nothofagus dombeyi with an understorey of the bamboo Chusquea culeou; (2) subalpine forests dominated by the deciduous Nothofagus pumilio; (3) dry forests dominated by Austrocedrus chilensis and small trees such as Lomatia hirsuta and Maytenus boaria; (4) alpine vegetation dominated by cushion shrubs; (5) shrublands of resprouting shrubs and small trees; and (6) forest dominated by exotic conifers (Pinus spp. and Pseudotsuga menziesii).
Extent of the study area in north-western Argentine Patagonia. Left: study area and fires scars by year. Right: location of the study area in Patagonia and Argentina.
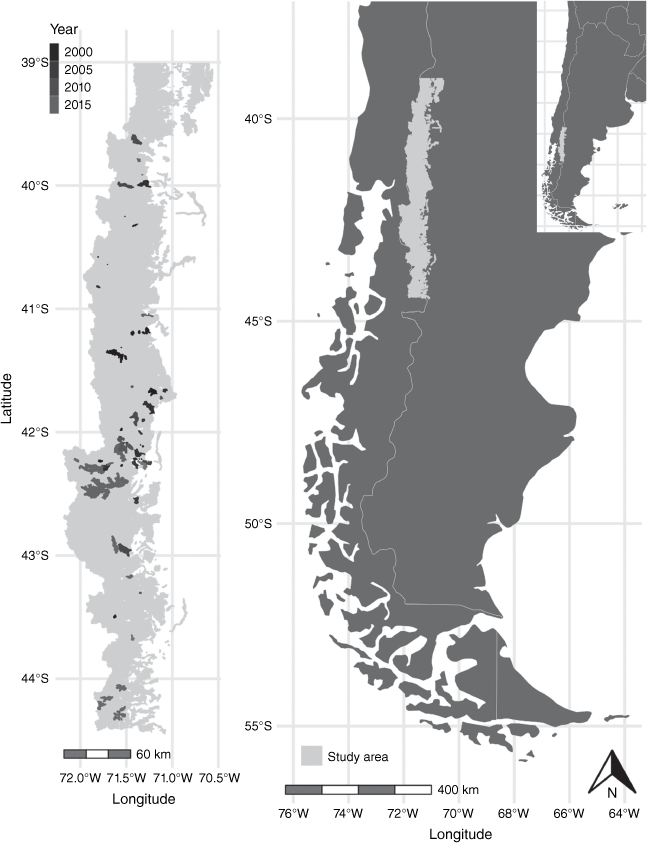
Nothofagus spp. have thin bark and are easily killed by fire even at medium severity (González et al. 2010; Arroyo-Vargas et al. 2019; Landesmann et al. 2021; Franco et al. 2022), although large trees may survive low-intensity surface fires (Kitzberger et al. 2005). All Nothofagus trees in the study area are non-resprouters, and do not have a persistent seed bank or fire-induced germination and thus can only regenerate from unburnt patches that provide seeds. These trees are also heliophilous, have a short post-fire regeneration window and their seeds are wind-dispersed with short dispersal distances – typically no further than 50–100 m. Trees fail to regenerate after severe large fires and forests are replaced by shrublands of resprouting species that often persist in the forest understorey. The conifer A. chilensis is an intermediate shade-tolerant tree able to regenerate even in dense shrublands (Landesmann et al. 2015) and capable of long-distance seed dispersal, having a better chance to recolonise terrain (Landesmann and Morales 2018). With long periods without fire, Austrocedrus eventually overtops and suppresses shrubs (Landesmann et al. 2016). At relatively drier sites and/or after severe fires, shrublands develop dominated by resprouting short trees (e.g. Nothofagus antarctica, L. hirsuta, M. boaria), tall shrubs (e.g. Embothrium coccineum, Diostea juncea) and the bamboo C. culeou (Veblen et al. 2003). These communities are highly prone to burning owing to site and fuel conditions. Moreover, the decurrent multi-stemmed growth forms provide connected fuel ladders, making them highly flammable. Rapid fuel recovery mediated by vigorous post-fire resprouting results in short fire return intervals (Veblen et al. 1992). Many of these shrublands are early stages of post-fire succession that eventually result in closed-canopy forest, but this is often delayed by repeated burning and heavy livestock pressure (Blackhall et al. 2008, 2017; Paritsis et al. 2015).
Fire data and field metrics of burn severity
In order to understand patterns of burn severity between 1999 and 2019, we gathered fire perimeter data for 74 fires from previous studies (Paritsis et al. 2013; Tiribelli et al. 2019), the National Parks Administration (Administración de Parques Nacionales, APN), Lanín National Park (LNP) (Mermoz et al. 2005; Orellana 2013) and the Forest Management Department of Chubut Province (Mohr Bell 2015). Additional fires that were mapped by the present study group using Landsat imagery for other projects (Barberá I., Kitzberger, T., unpubl. data) were also included. For every fire, we had the year of occurrence and the burned area (ha) along with a shape layer with the limits of the fire (fire perimeters).
Data analysis
To understand how productivity, climate, topography and distance to water bodies changed the probability of high burn severity, we fitted a multivariate hierarchical regression model. We first trained a Bayesian logistic model as regional burn severity classification algorithm with field reference data and Landsat-based burn severity indexes to classify historic fires (see Supplementary Material S1.1). The model output can either be the pixel probability of burning with high severity (the posterior mean) or maps classified into low and high burn severity with the chosen probability cut-off (Supplementary Fig. S1). As a proxy for productivity, we used the mean NDVI value of the previous fire season to represent the productivity of the vegetation before burning. For this, we calculated NDVI values for every valid pixel between December and February of the season previous to the fire and then calculated the average in Google Earth Engine (Supplementary Table S7). We chose two variables to account for climatic conditions, annual mean temperature and annual precipitation, which correspond to the BIO1 and BIO12 variables of the WorldClim2.0 database (Fick and Hijmans 2017). To represent topography, we calculated aspect and slope in Google Earth Engine from the NASA SRTM (Shuttle Radar Topography Mission) Digital Elevation of the Google Earth Engine Data Catalog. We calculated northerliness and easterliness as the cosine and sine of the aspect in R with the REdaS package (Supplementary Table S5) (Maier 2015). We also calculated the Euclidean distance from every pixel to the nearest fire edge (Supplementary Table S5), and to the nearest water body (lake or river) with QGIS (QGIS Development Team 2021).
The probability of burning with high burn severity was modelled with a beta distribution and a logit link for the mean, which could vary steadily (linear model) or have a maximum or a minimum (quadratic model) as a function of the covariates. To do this, we randomly sampled 10% of all the pixels (116 713) that burned between 1999 and 2019 in the 74 fires, stratified by vegetation type, fire size and year. Details on the model’s structure, hierarchies and prior distributions for all model parameters can be found in Supplementary Material S1.2. For all model parameters, we calculated the mean of posterior distributions as point estimates and the 95% Highest Posterior Density (HPD) intervals as measures of uncertainty around these estimates (Gelman and Hill 2006). The effective sample size from each Monte Carlo Markov Chain (MCMC) can be found in Supplementary Material S1.2. We fitted the model in JAGS (Just Another Gibbs Sampler; Plummer 2003)
To understand how the probability of burning with high burn severities changed with different vegetation types along the productivity gradient, we chose 16 fires from the fire inventory that varied in year, size and geographic location. We sought a vegetation map that represented the pre-fire vegetation at smaller scale, especially the small forest patches, to assess how burn severity affects each vegetation type (Supplementary Table S5). To do that, we visually mapped in Google Earth the pre-fire vegetation using pre-fire images (Google Earth Pro 7.1 2020, Google Earth Pro 7.1 (2020) Patagonia, Argentina. 41-43° S, 72-71° W, Eye alt 0.5-2.5 km. Borders and labels, NOAA, DigitalGlobe 2020.). Vegetation classes were alpine vegetation (AV), dry forests (DV), shrublands (SH), exotic forests (EF), subalpine forests (SF) and wet forests (WF). All the polygons built in Google Earth were rasterised in R using the raster package with the same extent and resolution as the severity maps. This resulted in 16 vegetation maps that corresponded to 606 187 pixels. With this categorical data, we sampled 10% of the pixels and fitted a hierarchical regression model with a beta distribution with JAGS (Plummer 2003).
To understand how native forest and shrubland burn severity patterns changed with productivity and fire weather at the landscape scale, we plotted the estimated high burn severity probability as a function of these covariates for every burnt pixel. Here, we used NDVI as a proxy of productivity (Supplementary Table S7) and standardised anomalies of summer temperature and Palmer Drought Severity Index (PDSI) as proxies for fire weather for every fire year. For this, we processed the TerraClimate monthly climate database (Abatzoglou et al. 2018) using Google Earth Engine (Gorelick et al. 2017). Standardised anomalies for each variable were calculated using the 1981–2018 mean and standard deviation of each cell (Supplementary Table S7; and see Kitzberger et al. 2022). In order to be able to use every fire, we used the World Wildlife Fund (WWF) Valdivian Ecoregion Vegetation assessment (Lara et al. 1999) to derive four condensed vegetation types (Supplementary Table S5). Wet forests (WF) include original classes 01, 03, 05, 06, 07 and 11, dry forests (DF) original classes 02 and 04, subalpine forests (SF) the original class 08, shrubland (SH) the original class 09. We did this to be able to use all the fires in our inventory and not just the 16 fires for which we developed vegetation maps at the risk of losing finer-resolution patterns such as unburned islands of forests.
To assess if the overall pattern of burn severity changed with fire weather, we plotted the predicted high burn severity probability against NDVI values before the fire event as a proxy of fuel load before burning at 0.02 discrete NDVI intervals (Supplementary Table S7) and also discriminated by above and below average summer temperature and summer PDSI. To understand whether the overall pattern of burn severity against the productivity gradient applied to all vegetation types and all climatic scenarios or was just the result of the combination of all scenarios, we plotted the median high burn severity probability discriminated by vegetation type and the different fire weather scenarios. Finally, we plotted the predicted high burn severity probability against NDVI values at 0.02 discrete intervals under different climatic scenarios but discriminated by vegetation type.
Results
Burn severity classification algorithm
With a probability cut-off of 0.62, the burn severity classification model had an accuracy of 0.86 and kappa of 0.70, with high sensitivity (0.90) and specificity (0.84) (Supplementary Fig. S1). The average proportion of pixels classified with high burn severity among the 74 fires was 0.875 (s.d. 0.181, range 0.245–1). Shrublands had the highest average proportion of pixels classified as high severity (mean 0.903 s.d. 0.173, range 0.206–1), followed by dry forests (mean 0.837, s.d. 0.191, range 0.304–1), subalpine forests (mean 0.824, s.d. 0.208, range 0–1) and exotic conifer forests (mean 0.809, s.d. 0.269, range 0.138–1). Wet forests had the lowest average proportion of pixels classified as high severity (mean 0.75, s.d. 0.26, range 0.046–1) (Supplementary Table S6).
General drivers of burn severity
During the 20-fire-season period of this study, 74 fires burned 113 521.1 ha. These fires burned mostly as high fire severity (high 104 667.4 ha (92%) vs low 8853.66 ha (8%). High burn severity probability (HBSP) on average was far above 0.5 for almost every covariate, and in general, their effects were small and uncertain (Fig. 2). HBSP peaked at the intermediate portion of the NDVI gradient, reaching a probability of 0.9 of burning with low severity (0.5–0.9; Fig. 2a). The lowest probability was reached at the lowest portion of the gradient (0.77, NDVI < 0.3). The distance to water bodies, although different from zero (Supplementary Table S8), had a negligible effect (Fig. 2b). Intermediate slopes showed lower HBSP than flat terrain or steep slopes (Fig. 2c), and there was also some edge effect, given that near the fire edge, HBSP was lower than further away from it (Fig. 2d). South-facing mountain slopes had lower HBSP than north-facing slopes, and west-facing slopes lower HBSP than east-facing slopes although the difference was very low (Fig. 2e, f). Annual mean temperature showed a hump-shape with HBSP, where high and low annual mean temperature had the lowest HBSPs (Fig. 2g). Annual precipitation had the inverse pattern to temperature; pixels with mid-high annual precipitations had lower HBSP than the ones with low precipitation (Fig. 2h). Shrublands, exotic species plantations and dry forest had the highest HBSP, while wet forest and subalpine forest had the lowest HBSP (Fig. 3).
High burn severity probability (HBSP) relationship with (a) productivity (NDVI), (b) distance to water bodies (lakes and rivers), (c) slope, (d) distance to the nearest fire edge, (e) northerliness, (f) easterliness, (g) annual mean temperature and (h) annual precipitation. Black lines correspond to the predicted global mean partial effects from a hierarchical regression (fixing the remaining covariates at their means), and grey shading to the 95% highest posterior density interval. This figure relates to the 74 fires that occurred between 1999 and 2019.
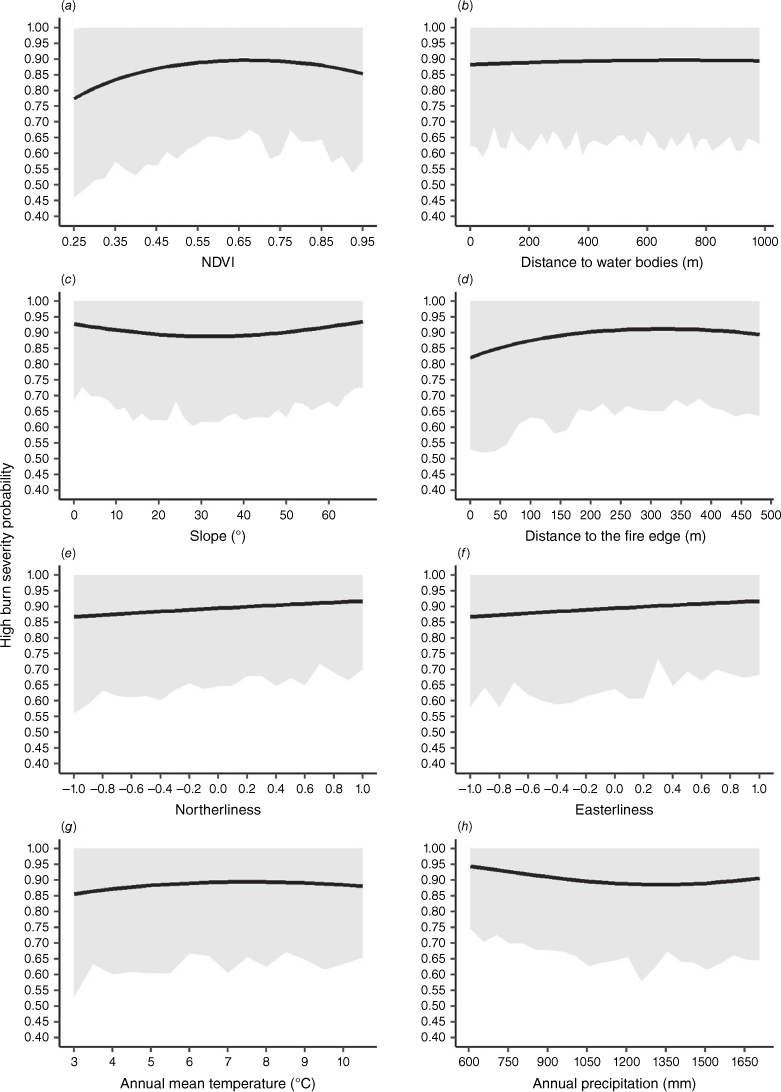
High burn severity probability (HBSP) for different vegetation types across the productivity gradient. AV, alpine vegetation; DF, dry forest; SH, shrubland; EF, exotic forest; SF, subalpine forest; WF, wet forest. Vegetation types are sorted from low to high by their NDVI range. The box represents 50% of the data, lower and upper boundaries correspond to the first and third quartiles (25th and 75th percentiles), and the lower and upper whiskers extend from the boundary to the largest or lowest value no further than (IQR, inter-quartile range) of the predicted probability (posterior mean). Different letters indicate low overlap between posterior distributions of the severity class (overlap < 5%). This figure relates to the 16 fires that have detailed (fine-scale) vegetation cover maps.
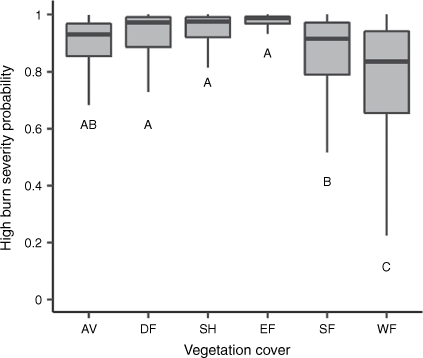
Burn severity patterns in native forests and shrublands in relation to productivity and fire weather
Out of the 20 years analysed, 5 years had above-average summer PDSI conditions and 5 below-average summer temperature, and only 2 years had both conditions. In native forests (dry, subalpine and wet forests) and shrublands, HBSP showed little variation at low or intermediate productivity (NDVI between 0.5 and 0.8), and decreased sharply at high productivity (NDVI > 0.8, Fig. 4a). When fire years were stratified between dry (PDSI below average) and wet (PDSI above average) summers and warm vs. cool summers (temperature above and below average, respectively), divergent patterns were found. HBSP dropped by 0.1–0.2 at NDVIs > ~0.65 during cool summers (Fig. 4b) as a well as during wet summers (Fig. 4c), whereas the HBSP-NDVI variation during warm and dry summers largely resembled that of all fires taken together (Fig. 4).
High burn severity probability patterns for pixels of burned native forests and shrublands as a function of productivity (NDVI) during: (a) all fires regardless of the summer weather condition; (b) fires that occurred in summers with below (blue bars) and above (red bars) average temperature; and (c) fires that occurred during summers with below average (green bars, wet) and above average (orange bars, dry) summer Palmer Drought Severity Index (PDSI). Points correspond to median values and the lower and upper whiskers extend to (IQR, inter-quartile range) of the predicted high burn severity probability (posterior mean) for all pixels of the 74 fires that occurred between 1999 and 2019.
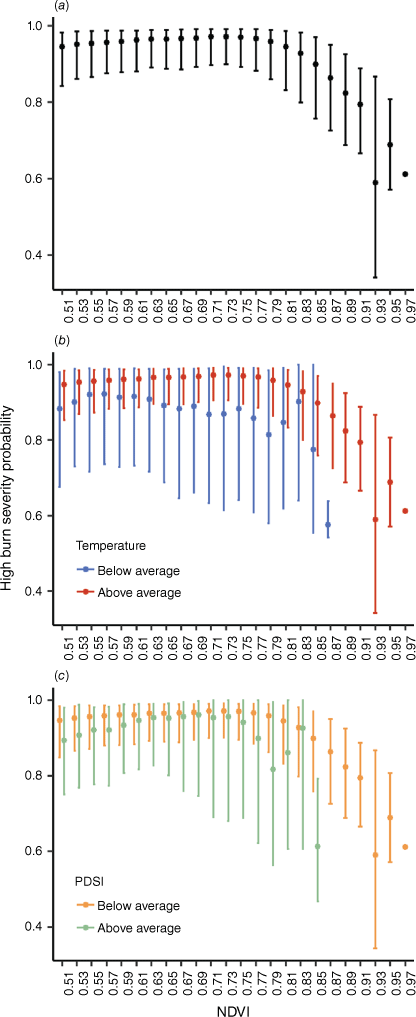
Wet forests followed by dry forests showed the largest reductions in HBSP during wet years (Fig. 5b) as well during cool years (Fig. 6). HBSP was reduced during cool or wet summers in wet and dry forests, from 0.9 to 0.53 and from 0.95 to 0.69, respectively, whereas for subalpine forests and shrublands, this probability always remained high (0.9 and ~0.95, Fig. 5a, b). However, when looking at the whole range of productivity within each forest type, subalpine forests reduced HBSP from ~0.95 to ~0.6 during cool summers only at higher productivity, but showed no reduction in HBSP at NDVI < 0.75 (Fig. 6). These lower NDVI locations coincide with north- and west-facing slopes where there is no apparent divergence in HBSP between cool and warm summers (Supplementary Figs S3 and S4). In contrast, wet forests, dry forests and shrublands show a more homogeneous reduction in HBSP during cool summers throughout their respective NDVI and topographic aspect ranges (Fig. 6).
High burn severity probability for major vegetation cover classes in different fire weather scenarios. High burn severity probability when (a) summer temperature, and (b) summer Palmer Drought Severity Index (PDSI) were above or below average. Points correspond to median values and the lower and upper whiskers extend to (IQR, inter-quartile range) of the predicted high burn severity probability (posterior mean) for all pixels of the 74 fires that occurred between 1999 and 2019. Bars represent the relative frequency of each fire weather scenario under each vegetation cover class.
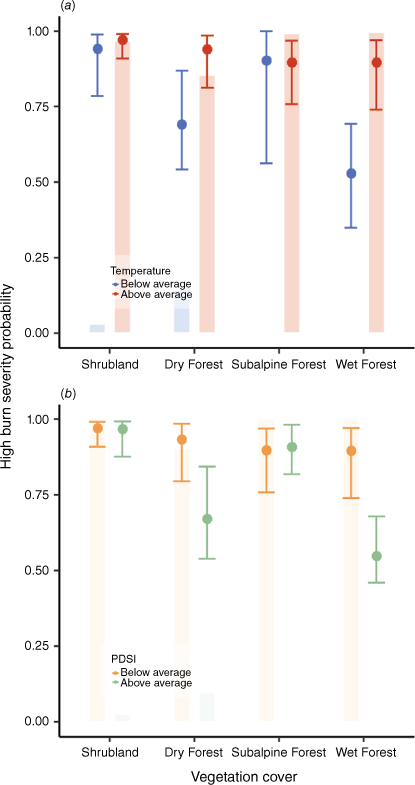
High burn severity probability as a function of NDVI for different vegetation types for fires that occurred under different summer temperature conditions. Points correspond to median values and the lower and upper whiskers extend to (IQR, inter-quartile range) of the predicted high burn severity probability (posterior mean) for all pixels of the 74 fires that occurred between 1999 and 2019.
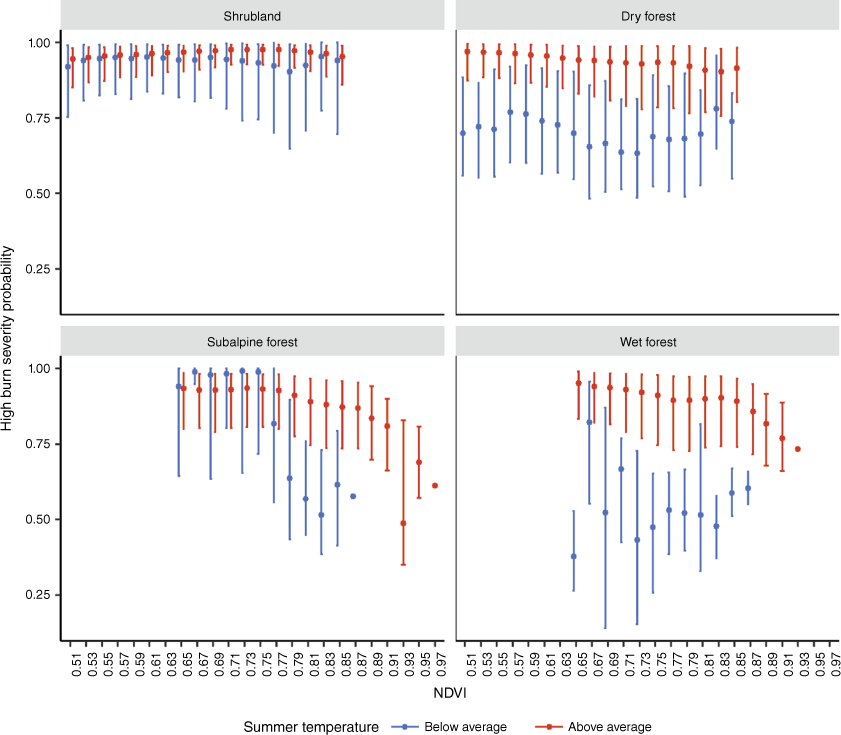
Discussion
Biomass-rich and fire-sensitive communities of north-western Patagonia typically burn at high severity. Of the 113 521 ha burned during 20 fire seasons, only 8% was classified as low severity, suggesting that certain very specific fuel, weather and topographic conditions must be met to burn at low severity. Spatially, these conditions are related to low-productivity environments with low fuel loads/continuity (e.g. sandy/rocky outcrops, tree lines), wet environments that generate highly productive wet or subalpine forests, and moist topographic aspects (south and east-facing slopes). In addition, burn severity is also attenuated along fire edges where fires were less intense and eventually stopped burning. Temporally, fires burning in cool/wet summer conditions in productive forest ecosystems tend to reduce the probability of high burn severity. The existence of these microsites where burn severity is attenuated is crucial for the persistence of fire-sensitive taxa in the landscape (in our case, obligate-seeder trees) as they allow for forests’ natural regeneration and persistence in the landscape.
Our results suggest that spatial attenuation of burn severity in systems subject to high burn severity can derive from the interplay between different bottom–up and top–down controls: (1) fuel amount and condition: fuels become sparser when resources for plants are reduced (limiting water, rocky/sandy substrates) and thus fires may burn at lower intensity, leaving unburned patches (Landesmann et al. 2015; Meddens et al. 2018). Fires also burn with lower intensity where fuels are subject to moister environments or where vegetation structure allows for less severe fires (Bradstock et al. 2010; Cawson et al. 2017; Zylstra et al. 2023). (2) Fire breaks: fire intensity decreases near perimeters owing to topographic restrictions like steep downslopes, fire flank areas were fires slowly creep in on rolling debris, dominant winds restrictions (e.g. windward, fire tail areas), or where and when fires encounter man-made or natural suppression conditions (fire lines, heavy rain). (3) Weather: areas that burn under cool/wet summer conditions have a higher probability of burning with low burn severity than the ones burning under hot/dry summer conditions, thus increasing the chances of canopy tree survival, particularly in productive fuel-rich forests (less and sparser surface fuels, wet and dark microenvironments, low connectivity) (Parks et al. 2018).
As predicted, our results show that the relationship between productivity and burn severity followed a hump-shaped relationship across different vegetation types, with dry forests, shrublands and exotic plantations displaying the highest burn severity probability (Fig. 3). In shrublands and dry forests, microclimatic conditions favour fuel desiccation (Paritsis et al. 2015; Barberá et al. 2023), and fuels are more flammable than in broadleaf forests (Blackhall et al. 2015, 2017; Blackhall and Raffaele 2019). Exotic conifer plantations are highly flammable, promoting highly active canopy-consuming fires (Paritsis et al. 2018). In all these vegetation types, dry fine fuel loads are relatively high and fuels have high vertical connectivity (climbers, tall shrubs, untreated plantations) that allow efficient crowning, explaining the pattern we see in our results (Blackhall et al. 2015, 2017; Paritsis et al. 2015, 2018; Tiribelli et al. 2018). Weather does not substantially modify severity when shrublands burn (Figs 5, 6, Supplementary Fig. S2), possibly owing to high levels of fine fuels that do not require long rain-free periods to dry out (Barberá et al. 2023). Despite high burn severity, two of these systems show high levels of resilience. Shrublands have a high proportion of resprouting species that rapidly dominate the post-fire stand (Veblen and Lorenz 1987, 1988) and field measurements show the ability of these species to readily resprout after severe fires and even replace post-fire forest communities (Kitzberger et al. 2016a; Landesmann et al. 2021; Franco et al. 2022). Exotic conifers are highly adapted to fire and rapidly recolonise burned plantations (Raffaele et al. 2016; Franzese et al. 2022). Finally, dry forests (e.g. Austrocedrus-dominated forests) are more fire sensitive, but the ability to grow and survive on dry unproductive rock outcrops (Landesmann et al. 2015) and relative high shade tolerance that enable the species to regenerate under a resprouting shrub canopy (Landesmann et al. 2016) allow long term persistence of the species in the landscape despite overall high levels of burn severity (Gowda et al. 2012).
The lower end of the productivity gradient coincides with rocky areas typical of alpine vegetation as well as some rocky, stony and sandy outcrops at mid elevations (Fig. 3). Here, low burn severity is possibly driven by lower fuel loads and continuity, creating islands of unburned patches promoting some fire resilience. The most productive forest types, such as wet forest and subalpine forests, showed the lowest levels of burn severity (Fig. 3). With high precipitation (>1500 Mean annual precipitation) or south- and east-facing slopes of moister higher elevations (1000–1600 m above sea level), the vegetation structure of dense, highly productive forest allows for darker and moister microclimatic conditions. Here, the discontinuity between understorey and tall canopy fuels (Blackhall et al. 2017; Tiribelli et al. 2018) reduces the probability of fire crowning, particularly during wetter/cooler fire seasons (Figs 5, 6, Supplementary Fig. S2). Under these conditions, and if forests have not burned during the last 100–150 years, larger thick-barked trees can survive and regenerate as a dense new forest cohort (Veblen and Lorenz 1987). If in contrast, fires burn under dry/hot summer conditions (Figs 5, 6, Supplementary Fig. S2), and forests are younger owing to the more frequent occurrence of past fires, trees cannot survive high fire intensity and may transition towards shrublands (Kitzberger et al. 2016b; Tiribelli et al. 2018; Landesmann et al. 2021). This pattern holds along the entire productivity gradient for wet forest but not for subalpine forests. Burn severity in subalpine forests of low productivity was not affected by weather (Fig. 6, Supplementary Fig. S2). These areas are located on north- and west-facing slopes, suggesting a strong topographic control on burn severity in subalpine forests (Supplementary Figs S3, S4).
Although forests may burn with low severity under certain climatic, productivity and topographic conditions, they are vulnerable communities even under these low burn severity scenarios if the first few years post-fire are not masting years for tree species, if cattle are introduced (Blackhall et al. 2008, 2017; Arroyo-Vargas et al. 2019), or if the climatic conditions post-fire do not allow for seed establishment or seedling survival (Kitzberger et al. 2000, 2005). This would lead to increases in burn severity due to vegetation shifts. As in other parts of the world (Karna et al. 2021; Lindenmayer et al. 2021), this positive feedback where severe fires beget severe fires ultimately increases shrubland areas to the detriment of forest area. Thus, the present empirical results underscore the importance of interannual climatic variability in modulating fire severity in northern Patagonia (Holz and Veblen 2011; Veblen et al. 2011) and elsewhere (Abatzoglou et al. 2017; Keyser and Leroy Westerling 2017), and alert us to possible increases in overall fire severity under the ongoing trends and expected scenarios of increasing aridity and temperature in the region (CONAMA 2006; IPCC 2013).
Here, we have shown several mechanisms by which fire regimes that are dominated by high burn severity can, under certain conditions, burn at lower severity, thus increasing forest resilience. These processes have acted in the past, producing the present north-Patagonian forest landscape (Veblen and Lorenz 1987, 1988; Gowda et al. 2012). Ongoing and projected future climate changes for this region suggest that these windows of opportunity for fire-sensitive forest taxa will lessen, thus promoting transitions towards alternative states dominated by more flammable species (Mermoz et al. 2005), and thereby positively reinforcing a regime dominated by frequent and severe fires (Kitzberger et al. 2016b; Tiribelli et al. 2019). Management and mitigation of these projected changes should focus on finding ways to preserve sites in the landscape that promote forest resilience attenuating burn severity. Spatially, we have identified certain site conditions where fires tend to burn at lower severity. Fire crews could take advantage of this information and use arrays of these a priori known locations to concentrate suppression actions as a way to more effectively fight fires and to secure the integrity of key forest patches (e.g. fire refugia on rocky outcrops (Landesmann and Morales 2018)) that will likely function as seed sources for the regeneration of obligate-seeder trees across vast areas burned at higher severity.
Although using temporal windows of wetter/cooler fire seasons seems tempting as opportunities for prescribed burning practices, our data suggest that it is still a risky recommendation as fires spreading under these conditions still have ~50% chance of becoming severe canopy-destroying fires. Nevertheless, identifying temporal windows of severity attenuation can be helpful to guide fire suppression actions (e.g. applying let-burn policies under certain fire weather condition assuming that considerable portions of the landscape will burn at lower severity). Under future climatic scenarios where the probability of fire occurrence will increase (Kitzberger et al. 2022) and opportunities for severity attenuation will become scarcer or more spatially restricted, it is imperative to develop efficient science-based fire management strategies that will help decrease burn severity and favour natural regeneration of closed-canopy broadleaf forests.
Declaration of funding
Funding was provided by Ministerio de Ciencia, Tecnología e Innovación Productiva (Argentina), Agencia Nacional de Promoción Científica y Tecnológica, grants PICT-2017-2142 and PICT-2019-406.
Author contributions
F. Tiribelli, T. Kitzberger and J. Paritsis conceived the ideas and designed the methodology; I. Barberá developed the GEE code to calculate severity indexes and calculate weather anomalies; T. Kitzberger, J. Paritsis and F. Tiribelli did the Google Earth pro vegetation classification. F. Tiribelli analysed the data; F. Tiribelli led the writing of the manuscript. All authors interpreted results, contributed critically to the drafts and gave final approval for publication.
Acknowledgements
We thank Paula Presti and Martin Müller form the Dirección de Lucha contra los Incendios Forestales y Emergencias (DLIFE), Parques Nacionales, for providing field fire severity measures and reports.
References
Abatzoglou JT, Kolden CA, Williams AP, et al. (2017) Climatic influences on interannual variability in regional burn severity across western US forests. International Journal of Wildland Fire 26, 269-275.
| Crossref | Google Scholar |
Abatzoglou JT, Dobrowski SZ, Parks SA, Hegewisch KC (2018) TerraClimate, a high-resolution global dataset of monthly climate and climatic water balance from 1958-2015. Scientific Data 5, 170191.
| Crossref | Google Scholar |
Arroyo-Vargas P, Fuentes-Ramírez A, Muys B, Pauchard A (2019) Impacts of fire severity and cattle grazing on early plant dynamics in old-growth Araucaria–Nothofagus forests. Forest Ecosystems 6, 44.
| Crossref | Google Scholar |
Assal TJ, González ME, Sibold JS (2018) Burn severity controls on postfire Araucaria–Nothofagus regeneration in the Andean Cordillera. Journal of Biogeography 45, 2483-2494.
| Crossref | Google Scholar |
Barberá I, Paritsis J, Ammassari L, et al. (2023) Microclimate and species composition shape the contribution of fuel moisture to positive fire-vegetation feedbacks. Agricultural and Forest Meteorology 330, 109289.
| Crossref | Google Scholar |
Blackhall M, Raffaele E (2019) Flammability of Patagonian invaders and natives: when exotic plant species affect live fine fuel ignitability in wildland–urban interfaces. Landscape and Urban Planning 189, 1-10.
| Crossref | Google Scholar |
Blackhall M, Raffaele E, Veblen TT (2008) Cattle affect early post-fire regeneration in a Nothofagus dombeyi–Austrocedrus chilensis mixed forest in northern Patagonia, Argentina. Biological Conservation 141, 2251-2261.
| Crossref | Google Scholar |
Blackhall M, Veblen TT, Raffaele E (2015) Recent fire and cattle herbivory enhance plant‐level fuel flammability in shrublands. Journal of Vegetation Science 26, 123-133.
| Crossref | Google Scholar |
Blackhall M, Raffaele E, Paritsis J, et al. (2017) Effects of biological legacies and herbivory on fuels and flammability traits: a long-term experimental study of alternative stable states. Journal of Ecology 105, 1309-1322.
| Crossref | Google Scholar |
Bowman DMJS, Williamson GJ, Gibson RK, et al. (2021) The severity and extent of the Australia 2019–20 Eucalyptus forest fires are not the legacy of forest management. Nature Ecology & Evolution 5, 1003-1010.
| Crossref | Google Scholar | PubMed |
Bradstock RA, Hammill KA, Collins L, Price O (2010) Effects of weather, fuel and terrain on fire severity in topographically diverse landscapes of south-eastern Australia. Landscape Ecology 25, 607-619.
| Crossref | Google Scholar |
Cansler CA, Mckenzie D (2014) Climate, fire size, and biophysical setting control fire severity and spatial pattern in the northern Cascade Range, USA. Ecological Applications 24, 1037-1056.
| Crossref | Google Scholar | PubMed |
Cawson JG, Duff TJ, Tolhurst KG, et al. (2017) Fuel moisture in Mountain Ash forests with contrasting fire histories. Forest Ecology and Management 400, 568-577.
| Crossref | Google Scholar |
Collins L, Clarke H, Clarke MF, et al. (2022) Warmer and drier conditions have increased the potential for large and severe fire seasons across south-eastern Australia. Global Ecology and Biogeography 31, 1933-1948.
| Crossref | Google Scholar |
Franco MG, Mundo IA, Veblen TT (2022) Burn severity in Araucaria araucana forests of northern Patagonia: tree mortality scales up to burn severity at plot scale, mediated by topography and climatic context. Plant Ecology 223, 811-828.
| Crossref | Google Scholar |
Fick SE, Hijmans RJ (2017) WorldClim 2: new 1‐km spatial resolution climate surfaces for global land areas. International Journal of Climatology 37, 4302-4315.
| Crossref | Google Scholar |
Franzese J, Raffaele E, Chiuffo MC, Blackhall M (2022) The legacy of pine introduction threatens the fuel traits of Patagonian native forests. Biological Conservation 267, 109472.
| Crossref | Google Scholar |
Gill AM (1975) Fire and the Australian flora: a review. Australian Forestry 38, 4-25.
| Crossref | Google Scholar |
González ME, Veblen TT, Sibold JS (2010) Influence of fire severity on stand development of Araucaria araucana–Nothofagus pumilio stands in the Andean cordillera of south-central Chile. Austral Ecology 35, 597-615.
| Crossref | Google Scholar |
Gorelick N, Hancher M, Dixon M, et al. (2017) Google Earth Engine : Planetary-scale geospatial analysis for everyone. Remote Sensing of Environment 202, 18-27.
| Crossref | Google Scholar |
Gowda JH, Kitzberger T, Premoli AC (2012) Landscape responses to a century of land use along the northern Patagonian forest–steppe transition. Plant Ecology 213, 259-272.
| Crossref | Google Scholar |
He T, Lamont BB, Pausas JG (2019) Fire as a key driver of Earth’s biodiversity. Biological Reviews 94, 1983-2010.
| Crossref | Google Scholar | PubMed |
Hessl AE (2011) Pathways for climate change effects on fire: models, data, and uncertainties. Progress in Physical Geography: Earth and Environment 35, 393-407.
| Crossref | Google Scholar |
Holz A, Veblen TT (2011) Variability in the Southern Annular Mode determines wildfire activity in Patagonia. Geophysical Research Letters 38, L14710.
| Crossref | Google Scholar |
Holz A, Wood SW, Veblen TT, Bowman DMJS (2015) Effects of high-severity fire drove the population collapse of the subalpine Tasmanian endemic conifer Athrotaxis cupressoides. Global Change Biology 21, 445-458.
| Crossref | Google Scholar | PubMed |
IPCC (2013) Annex I: Atlas of Global and Regional Climate Projections. van Oldenborgh GJ, Collins M, Arblaster J, Christensen JH, Marotzke J, Power SB, Rummukainen M, Zhou T, Eds. In ‘Climate Change 2013: The Physical Science Basis. Contribution of Working Group I to the Fifth Assessment Report of the Intergovernmental Panel on Climate Change’. (Eds TF Stocker, D Qin, G-K Plattner, M Tignor, SK Allen, J Boschung, A Nauels, Y Xia, V Bex, PM Midgley) pp. 1311–1394. (Cambridge University Press: Cambridge, United Kingdom and New York) 10.1017/CBO9781107415324.029
Jayen K, Leduc A, Bergeron Y (2006) Effect of fire severity on regeneration success in the boreal forest of northwest Québec, Canada. Écoscience 13, 143-151.
| Crossref | Google Scholar |
Karna YK, Penman TD, Aponte C, et al. (2021) Indications of positive feedbacks to flammability through fuel structure after high-severity fire in temperate eucalypt forests. International Journal of Wildland Fire 30, 664-679.
| Crossref | Google Scholar |
Keeley JE (2009) Fire intensity, fire severity and burn severity: a brief review and suggested usage. International Journal of Wildland Fire 18, 116-126.
| Crossref | Google Scholar |
Keyser A, Leroy Westerling A (2017) Climate drives inter-annual variability in probability of high severity fire occurrence in the western United States. Environmental Research Letters 12, 065003.
| Crossref | Google Scholar |
Kitzberger T, Steinaker DF, Veblen TT (2000) Effects of climatic variability on facilitation of tree establishment in northern Patagonia. Ecology 81, 1914-1924.
| Crossref | Google Scholar |
Kitzberger T, Raffaele E, Heinemann K, Mazzarino MJ (2005) Effects of fire severity in a north Patagonian subalpine forest. Journal of Vegetation Science 16, 5-12.
| Crossref | Google Scholar |
Kitzberger T, Grosfeld J, Gowda JH, et al. (2016a) Diagnóstico de la severidad de fuego y propuestas de restauración y manejo a nivel predial para áreas afectadas por el incendio de Cholila de 2015. [In Spanish] Available at https://inibioma.conicet.gov.ar/incendio‐de‐cholila‐2015‐diagnostico‐de‐la‐severidad‐del‐fuego‐y‐propuestas‐de‐restauracion/
Kitzberger T, Perry GLW, Paritsis J, et al. (2016b) Fire–vegetation feedbacks and alternative states: common mechanisms of temperate forest vulnerability to fire in southern South America and New Zealand. New Zealand Journal of Botany 8643, 1-26.
| Crossref | Google Scholar |
Kitzberger T, Tiribelli F, Barberá I, et al. (2022) Projections of fire probability and ecosystem vulnerability under 21st century climate across a trans-Andean productivity gradient in Patagonia. Science of the Total Environment 839, 156303.
| Crossref | Google Scholar | PubMed |
Landesmann JB, Morales JM (2018) The importance of fire refugia in the recolonization of a fire-sensitive conifer in northern Patagonia. Plant Ecology 219, 455-466.
| Crossref | Google Scholar |
Landesmann JB, Gowda JH, Garibaldi LA, Kitzberger T (2015) Survival, growth and vulnerability to drought in fire refuges: implications for the persistence of a fire-sensitive conifer in northern Patagonia. Oecologia 179, 1111-1122.
| Crossref | Google Scholar | PubMed |
Landesmann J, Gowda JH, Kitzberger T, Michalet R (2016) Temporal shifts in the interaction between woody resprouters and an obligate seeder tree during a post-fire succession in Patagonia. Journal of Vegetation Science 27, 1198-1208.
| Crossref | Google Scholar |
Landesmann JB, Tiribelli F, Paritsis J, et al. (2021) Increased fire severity triggers positive feedbacks of greater vegetation flammability and favors plant community‐type conversions. Journal of Vegetation Science 32, 1-13.
| Crossref | Google Scholar |
Lindenmayer D, Taylor C, Blanchard W (2021) Empirical analyses of the factors influencing fire severity in southeastern Australia. Ecosphere 12, e03721.
| Crossref | Google Scholar |
Lindenmayer DB, Kooyman RM, Taylor C, et al. (2020) Recent Australian wildfires made worse by logging and associated forest management. Nature Ecology & Evolution 4, 898-900.
| Crossref | Google Scholar | PubMed |
Lindenmayer DB, Zylstra P, Kooyman R, et al. (2022) Logging elevated the probability of high-severity fire in the 2019–20 Australian forest fires. Nature Ecology & Evolution 6, 533-535.
| Crossref | Google Scholar | PubMed |
Maier MJ (2015) Companion package to the book ‘R: Einführung durch angewandte Statistik’. R package version 0.9. 3. https://CRAN.R-project.org/package=REdaS
Meddens AJH, Kolden CA, Lutz JA, et al. (2018) Fire refugia: what are they, and why do they matter for global change? Bioscience 68, 944-954.
| Crossref | Google Scholar |
Mermoz M, Kitzberger T, Veblen TT (2005) Landscape influences on occurrence and spread of wildfires in Patagonian forests and shrublands. Ecology 86, 2705-2715.
| Crossref | Google Scholar |
Murphy BP, Bradstock RA, Boer MM, et al. (2013) Fire regimes of Australia: a pyrogeographic model system. Journal of Biogeography 40, 1048-1058.
| Crossref | Google Scholar |
Owen SM, Sieg CH, Fulé PZ, et al. (2020) Persistent effects of fire severity on ponderosa pine regeneration niches and seedling growth. Forest Ecology and Management 477, 118502.
| Crossref | Google Scholar |
Palmer HD, Denham AJ, Ooi MKJ (2018) Fire severity drives variation in post-fire recruitment and residual seed bank size of Acacia species. Plant Ecology 219, 527-537.
| Crossref | Google Scholar |
Paritsis J, Holz A, Veblen TT, Kitzberger T (2013) Habitat distribution modeling reveals vegetation flammability and land use as drivers of wildfire in SW Patagonia. Ecosphere 4, 1-20.
| Crossref | Google Scholar |
Paritsis J, Veblen TT, Holz A (2015) Positive fire feedbacks contribute to shifts from Nothofagus pumilio forests to fire-prone shrublands in Patagonia. Journal of Vegetation Science 26, 89-101.
| Crossref | Google Scholar |
Paritsis J, Landesmann J, Kitzberger T, et al. (2018) Pine plantations and invasion alter fuel structure and potential fire behavior in a Patagonian forest–steppe ecotone. Forests 9, 117.
| Crossref | Google Scholar |
Parks SA, Miller C, Nelson CR, Holden ZA (2014) Previous fires moderate burn severity of subsequent wildland fires in two large Western US wilderness areas. Ecosystems 17, 29-42.
| Crossref | Google Scholar |
Parks SA, Holsinger LM, Panunto MH, et al. (2018) High-severity fire: evaluating its key drivers and mapping its probability across western US forests. Environmental Research Letters 13, 044037.
| Crossref | Google Scholar |
Pausas JG, Paula S (2012) Fuel shapes the fire–climate relationship: evidence from Mediterranean ecosystems. Global Ecology and Biogeography 21, 1074-1082.
| Crossref | Google Scholar |
Plummer M (2003) JAGS: A program for analysis of Bayesian graphical models using Gibbs sampling. In ‘Proceedings 3rd International Workshop on Distributed Statistical Computing (DSC 2003)’. Vol. 124, No. 125.10, pp. 20–22. https://www.r‐project.org/conferences/DSC‐2003/Proceedings/Plummer.pdf
QGIS Development Team (2021) QGIS Geographic Information System. Open Source Geospatial Foundation Project. http://qgis.org
Raffaele E, Nuñez MA, Eneström J, Blackhall M (2016) Fire as mediator of pine invasion: evidence from Patagonia, Argentina. Biological Invasions 18, 597-601.
| Crossref | Google Scholar |
Tiribelli F, Kitzberger T, Morales JM (2018) Changes in vegetation structure and fuel characteristics along post-fire succession promote alternative stable states and positive fire–vegetation feedbacks. Journal of Vegetation Science 29, 147-156.
| Crossref | Google Scholar |
Tiribelli F, Morales JM, Gowda JH, et al. (2019) Non-additive effects of alternative stable states on landscape flammability in NW Patagonia: fire history and simulation modelling evidence. International Journal of Wildland Fire 28, 149-159.
| Crossref | Google Scholar |
Tran BN, Tanase MA, Bennett LT, Aponte C (2020) High-severity wildfires in temperate Australian forests have increased in extent and aggregation in recent decades. PLoS One 15, e0242484.
| Crossref | Google Scholar |
Veblen TT, Lorenz DC (1987) Post-fire stand development of Austrocedrus–Nothofagus forests in northern Patagonia. Vegetatio 71, 113-126.
| Crossref | Google Scholar |
Veblen TT, Lorenz DC (1988) Recent vegetation changes along the forest/steppe ecotone of Northern Patagonia. Annals of the Association of American Geographers 78, 93-111.
| Crossref | Google Scholar |
Veblen TT, Kitzberger T, Lara A (1992) Disturbance and forest dynamics along a transect from Andean rain forest to Patagonian shrubland. Journal of Vegetation Science 3, 507-520.
| Crossref | Google Scholar |
Veblen TT, Kitzberger T, Raffaele E, Lorenz DC (2003) Fire history and vegetation changes in northern Patagonia, Argentina. In ‘Fire and Climatic Change in the Temperate Ecosystems of the Western Americas’. (Eds TT Veblen, WL Baker, G Montenegro, TW Swetnam) pp. 265–295. (Springer-Verlag: New York, NY, USA)
Veblen TT, Holz A, Paritsis J, et al. (2011) Adapting to global environmental change in Patagonia: what role for disturbance ecology? Austral Ecology 36, 891-903.
| Crossref | Google Scholar |
Walker XJ, Rogers BM, Veraverbeke S, et al. (2020) Fuel availability not fire weather controls boreal wildfire severity and carbon emissions. Nature Climate Change 10, 1130-1136.
| Crossref | Google Scholar |
Zylstra P, Wardell‐Johnson G, Falster D, et al. (2023) Mechanisms by which growth and succession limit the impact of fire in a south‐western Australian forested ecosystem. Functional Ecology 37, 1350-1365.
| Crossref | Google Scholar |