Prescribed fire increases forage mineral content in grazed rangeland
Megan R. Wanchuk A , Devan Allen McGranahan
A
B
C
D
Abstract
Sustainable rangeland management balances production and conservation. While a broad literature describesthe conservation benefits of prescribed fire, benefits for livestock production have emerged more slowly. Mineral nutrition is important for livestock health and performance, but the impact of prescribed fire on mineral concentration of forages, especially in the northern US Great Plains, remains unknown.
We investigated how burning affects the mineral concentration of forage early and late in the growing season.
Data were collected on mixed-grass prairie in south-central North Dakota, USA. Vegetation was clipped from recently burned, 1 year post-fire, 2 years post-fire, and not-yet-burned patches at the same sampling points in spring and late summer. Samples were analysed for calcium, phosphorus, magnesium, potassium, copper, iron, manganese, and zinc concentration.
Burning increased forage mineral concentration across most minerals. Phosphorous, potassium, copper and zinc were higher in burned areas in late spring and summer; calcium, magnesium and manganese were only higher during the late summer; Late-season iron levels increased with time since fire.
Prescribed fire has a positive effect on forage mineral content.
Prescribed fire has the potential to reduce mineral supplementation costs and improve cow performance.
Keywords: beef cattle, fire-grazing interaction, forage nutritive value, grassland fire management, livestock nutrition, mixed-grass prairie, pyric herbivory, rangeland management.
Introduction
Rangelands are globally-ubiquitous examples of open ecosystems that have evolved under the top-down control of disturbance regimes, which commonly include fire and grazing (Bond 2022). Interactions between fire and grazing often create pyrodiversity, which is define as a heterogeneous landscape where heterogeneity initiated by spatially-patchy fire is maintained by focal grazing in burned patches (Archibald et al. 2005; Fuhlendorf et al. 2017). At the broad landscape scale, this diversity in landscape pattern and vegetation structure maintains biodiversity through the provision of habitat niches and other necessary resources within fine-scale patches (McGranahan et al. 2013; McGranahan and Wonkka 2021).
Although fire–grazer interactions characterised pre-colonial landscapes with native herbivores and natural and/or indigenous burning, the patterns can be re-created in modern landscapes with spatially-patchy prescribed fire in grazed rangeland (Fuhlendorf and Engle 2004; McGranahan and Kirkman 2013). Patch-burning concentrates grazer activity in recently-burned patches within larger pastures, creating spatial heterogeneity that enhances ecosystem functioning and biodiversity (Fuhlendorf et al. 2017). Meanwhile, low-quality forage in unburned areas generally deters grazers, allowing aboveground vegetation to accumulate and provide dense litter and other resources that benefit biodiversity (McGranahan et al. 2012, 2013).
Accruing evidence indicates fire can also enhance livestock production, which has important implications for fire management in grazed ecosystems. Following fire, forage is high in crude protein and low in acid detergent fibre and neutral detergent fibre (Dufek et al. 2014; Spiess et al. 2024; Wanchuk et al. 2024). Although burning aboveground plant biomass reduces overall forage availability, the high quality of the regrowth maintains or even increases livestock weight gains in both mesic and semi-arid grasslands (Spiess et al. 2020, 2024; Wanchuk et al. 2024). Although most reports on burning benefits come from prescribed fire studies, additional research confirms that forage resources recover quickly after wildfire, and can support grazing shortly after being burned (Gates et al. 2017; Kral-O’Brien et al. 2020; Williams et al. 2022). Understanding fire impacts on forage is especially important in grassland-dominated regions such as the north-western Great Plains where commercial livestock grazing is the primary land use and wildfire frequency on public grazing lands is increasing (McGranahan and Wonkka 2024).
Often overlooked in fire × grazing research is forage mineral nutrition, which is an important consideration to enhance livestock reproduction, health, and growth, and maintain ranch sustainability (Greene 2000; Suttle 2010). To our knowledge, there are no published data on whether prescribed burning has a positive or negative effect on forage mineral concentration. Several minerals are essential for cattle, including the macro-minerals calcium, magnesium, phosphorus, potassium, sodium, chlorine, and sulfur; and the trace or micro-minerals chromium, cobalt, copper, iodine, iron, manganese, molybdenum, nickel, selenium, and zinc (NASEM 2016). However, the extensively-managed rangelands that comprise most ranching operations are not always able to satisfy the mineral requirements of grazing cattle throughout the grazing season, decreasing forage utilisation and performance (McDowell 1996; Arthington and Ranches 2021). In particular, phosphorous, magnesium, copper, zinc, manganese, cobalt, selenium, and iodine concentrations are often deficient for cattle (Greene 2000; Suttle 2010; Arthington and Ranches 2021). Free-choice mineral supplementation can be used to meet grazing livestock requirements, but this adds cost to production and individual animal intake can be variable (Greene 2000).
Mineral concentration of forage can vary spatially and temporally because rangeland plant communities and soils are often variable. Precipitation is also a factor, with many mineral concentrations increasing during drought and essentially being diluted in plant tissues when rainfall exceeds average (Grings et al. 1996; Ganskopp and Bohnert 2003). As plants use their roots to take up plant-available minerals via aqueous solution in the soil, forage mineral concentrations are generally a reflection of the amount of absorbable minerals in the soil (Suttle 2010). Furthermore, soil pH can influence plant mineral uptake, with pH values above six increasing molybdenum, and decreasing manganese and cobalt uptake (Suttle 2010). Over the grazing season, forage mineral concentration usually decreases with maturation for phosphorus, potassium, zinc, copper, manganese, and iron since plant maturity causes dilution and translocation of minerals to roots (McDowell 1996; Suttle 2010). Conversely, calcium and magnesium concentration usually increase as the grazing season progresses as forage matures and accumulated minerals are less diluted in plant tissue (Metson and Saunders 1978; Ganskopp and Bohnert 2003).
Limited studies have examined fire effects on forage mineral concentration, with contradicting results (Umoh et al. 1982; Van de Vijver et al. 1999; Anderson et al. 2007; Eby et al. 2014; Vermeire et al. 2020). Although the majority of work has found some minerals to increase in recently burned forage, there is a lack of consistency as to which minerals are impacted by fire (Van de Vijver et al. 1999; Anderson et al. 2007; Eby et al. 2014; Vermeire et al. 2020). Much of this variability can likely be attributed to different soil mineral concentration, prescribed fire timing, and differences in plant community composition; mineral concentration is typically greater in legumes and forbs than in grasses, with species-level differences within plant families (Grings et al. 1996; Ganskopp and Bohnert 2003; Suttle 2010). The short duration of forage mineral increase is the one aspect consistent between studies, with forage mineral concentration returning to unburned levels by a few months post-fire (Van de Vijver et al. 1999; Eby et al. 2014; Vermeire et al. 2020).
The lack of consistent outcomes in the limited studies examining forage minerals under prescribed fire complicates managers’ ability to ensure proper mineral nutrition of livestock grazing post-fire rangeland. We determined whether prescribed fire impacts several forage minerals that are important to beef cattle nutrition. Our objectives were to: (1) determine if prescribed fire increases mineral concentration of forage in rangeland pastures; and (2) ascertain if forage after prescribed fire will better meet recommendations for beef cattle. We hypothesised that post-fire regrowth in patches following spring fire would have greater mineral concentrations than forage in unburned patches, and that this increase would be short-term, lasting the first 3 months of the grazing season. We also expect that forage in recently burned patches will be closer to meeting the requirements of grazing beef cows.
Materials and methods
Study location & design
We conducted this study at the North Dakota State University Central Grasslands Research Extension Centre in south-central North Dakota, USA (46°45′N, 99°28′W). The climate is characterised as temperate, with a previous 30-year average growing season precipitation of 333 mm and air temperature 17°C. During the 2017–2020 study period, growing season precipitation averaged 339 mm (range, 212–447 mm) and air temperature averaged 17°C (range, 16–18°C) (NDAWN 2023). A drought occurred in 2017 when seasonal rainfall was 27% below average (Spiess et al. 2020).
Vegetation in the region is typical northern mixed-grass prairie. Herbaceous cover of the study pastures consists primarily of Kentucky bluegrass (Poa pratensis L.), smooth brome (Bromus inermis L.), western wheatgrass (Pascopyron smithii [Rydb] À. Löve), and little bluestem (Schizachyrium scoparium [Michx] Nash.). Study pastures had low dissimilarity in vegetation composition and each expressed increasing native species dominance over the course of the study period (Duquette et al. 2022). Soils were consisted of the Zahl-Williams-Zahill complex and Zahl-Max-Bowbells loams (NRCS 2023). Prior to this research, there are no records or knowledge of fire within the study pastures for at least several decades, if ever, since Euro-American settlement approximately a century ago.
We conducted this study on three replicate pastures (experimental units) managed with patch-burn grazing. Commercial crossbred Angus cow-calf pairs (Bos taurus) grazed each of the three 65 ha patch-burn pastures from mid-May to mid/late-October at a stocking rate of 0.5–0.6 ha Animal Unit (454 kg) per month. These pastures underwent a spring burning treatment (April–May; see Supplementary Table S1) where a quarter (~16 ha) of the pasture was burned each spring, creating a 4-year fire return interval (Fig. S1). Patches classified as ‘recently burned’ were those that received a fire treatment in the spring of the sampling year. Fire spread covered 80–100% of intended burn areas and spread at an average rate of 2.4 m min−1; 50% of soil surface temperatures fell between 50 and 250°C (McGranahan et al. 2023).
Data collection and analysis
Forage sampling occurred from 2017 to 2020 and began 3–6 weeks after spring burns, from late spring (May) to late summer (September), which corresponded to when cattle started the grazing season and within a month of the end of the grazing season. To minimise variability in mineral concentration due to inherent differences in soil, we only selected clipping points from the widespread thin-loamy ecological site (Sedivec et al. 2021), which was the dominant site (53–73%) in each of the three study pastures.
We clipped forage samples from patches along a chronosequence of four fire histories: (1) yet to be burned; recently burned, 1 year since fire; and (4) 2 years since fire (Table S2). Observational units consisted of digitally geo-referenced sampling points, which remained the same for the duration of the study. Each sample point was sampled in each year throughout the study, each year being reassigned into a longer time-since-fire category. Because the number of points per patch varied with the proportionate area of the thin-loamy site, and changed as patches proceeded through the time-since-fire chronosequence (Fig. S1), the number of observational units for each treatment in each year varied between 13 and 43.
We clipped standing live and dead plant material from a 25 × 25 cm frame, 3 cm above the soil surface to minimise contamination from soil and litter. The clipped standing material from each quadrat comprised the samples used for analysis. This forage clipping method simulated feeding station forage removal by bulk grazers (feeding station, the area immediately available to a grazing animal; WallisDeVries et al. 1998). After clipping, we dried samples for 48 h at 60°C in a forced air oven, then ground samples with a Willey Mill (Model 4, Thomas Scientific, Swedesboro, NJ, USA) using a 1-mm screen; ground samples were stored in plastic bags ahead of chemical analysis. We analysed samples for calcium (Ca), phosphorous (P), magnesium (Mg), potassium (K), copper (Cu), iron (Fe), manganese (Mn), and zinc (Zn) using atomic absorption spectrophotometry (AOAC 2010).
Finally, we compared our forage mineral concentration in pastures to recommended levels of forage mineral concentrations. Forage mineral concentration recommendations were based on the average animal grazing the study pastures: an April calving, 572 kg cow with 8 kg/day peak milk production and 40 kg calf birth weight (NASEM 2016). For Ca, P, K, and Mg, we compared observed early season values to recommended mineral levels for lactating beef cows, and late season values were compared to general beef cow recommendations (NASEM 2016). For Cu, Zn, Fe, and Mn, we considered general season-long beef cow mineral recommendations (NASEM 2016).
Statistical analysis
All data were analysed in the R statistical environment (R Core Team 2019) using procedures robust to unbalanced sample sizes due to variability in observational units within the three replicate pastures. We compared mineral concentration between seasons and across a time since fire gradient using linear mixed-effect models using the ‘lmer’ function from the lme4 package (Bates et al. 2015). To account for non-independence due to repeated measurements and the hierarchical nestedness of patches within the three experimental units, we modelled pasture and year as crossed random effects. We developed model sets, which included a null model, time since fire only, season only, and time since fire with season as an additive and as an interaction. We then ranked candidate models using Akaike information criterion (AIC). We considered models with ΔAICc ≤ 2 to have the same explanatory power (Burnham and Anderson 2004). To get pairwise comparisons between time since fire, season or time since fire and season we used ‘emmeans’ function from the emmeans package.
To determine dissimilarity between treatments, we used principal component analysis (PCA) in the vegan package (Oksanen et al. 2016) on scaled data. We then conducted post hoc pairwise comparisons using vegan’s ‘envfit’ (permutations = 199) to determine if there were differences between treatments, years, and sampling periods. We incorporated pasture and year as a random effect (strata) within ‘envfit’ to account for repeated sampling and pasture differences. Pairwise comparisons of multivariate analysis were done using ‘pairwise.factorfit’ from RVAideMemoire (permutations = 199), with variability from pasture and year accounted for as a random effect (Hervé 2018). We considered results to be significant for all analyses at α ≤ 0.05.
Results
With the exception of iron (Fe), almost all minerals tested showed a difference between recently burned and not-recently burned patches in at least one sampling period (Fig. 1). Regression models including interaction terms between time since fire and sampling season had the best fit for all minerals except phosphorous, indicating statistically-significant seasonal patterns for those minerals. In general, forage in recently burned patches best met recommended mineral intake levels based on NASEM (2016) (Fig. 1).
Mean and s.e. of concentration of eight minerals in rangeland forage by sampling season and time-since-fire. Concentrations are expressed as either percentage of forage dry matter (%) or parts per million (ppm). ‘Early’ and ‘late’ refer to late spring (May) and late summer (September), respectively. Horizontal lines in calcium (Ca), phosphorous (P), potassium (K), and magnesium (Mg) represent recommended mineral levels for lactating beef cows early in the grazing season, and general beef cow late season beef cow recommendations late in the season (NASEM 2016). Horizontal lines in copper (Cu), zinc (Zn), iron (Fe), and manganese (Mn) represent general season long beef cow recommended mineral levels (NASEM 2016). Data represent means from 4 years of collection, tested with linear mixed-effect regression.
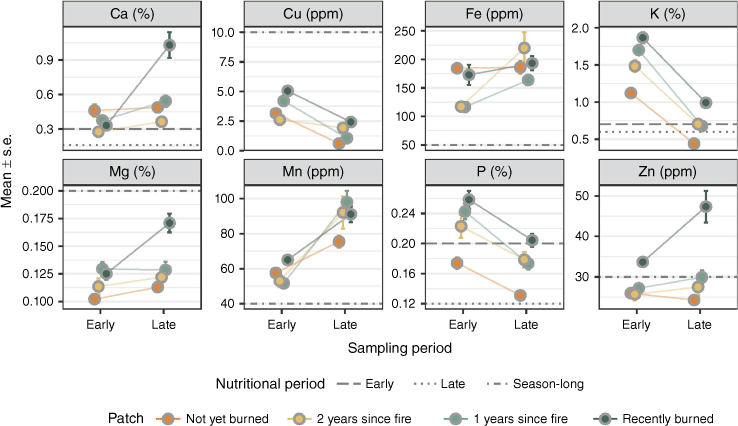
Calcium (Ca) did not differ among any patches early in the grazing season (P > 0.05), but was greater in the recently burned patches than all other patches (recently burned vs 1-year-since-fire, t = 4.08, P < 0.001; burned vs 2-years-since-fire, t = 5.03, P < 0.001; recently burned vs not yet burned, t = 6.98, P < 0.001). Ca was consistently above late season beef cattle recommendations, and either at or above recommendations for early season lactating cows.
Early in the season, copper (Cu) concentration was not different between recently burned and 1-year-since fire patches (recently burned vs 1-year-since-fire, t = 2.0, P = 0.19), but both previously-burned patches had more Cu than the longest interval and not yet burned patches (recently burned vs 2-years-since-fire, t = 5.8, P = < 0.001; 1-year-since-fire vs 2-years-since-fire, t = 3.93, P < 0.001; recently burned vs not yet burned, t = 5.68, P < 0.001; 1-year-since-fire vs not yet burned, t = 3.12, P = 0.01). But late in the season, Cu was only greater in recently burned and 2-years-since-fire patches than not yet burned patches (recently vs not yet burned: t = 5.08, P < 0.0001; 2-years-since-fire vs not yet burned: t = 3.02, P < 0.02). Cu concentration was consistently below season-long recommendations, although Cu was often twice as concentrated in the recently burned patch than not yet burned patches.
Early in the season, potassium (K) levels were significantly different among several patch types. Not only was K higher in recently burned than all other patches (recently burned vs 1-year-since-fire: t = 2.76, P = 0.03; recently burned vs 2-years-since-fire, t = 4.81, P < 0.001; recently burned vs not yet burned, t = 10.08, P < 0.001), but patches burned 1 and 2 years ago had more K than not yet burned patches (1-year-since-fire vs not yet burned, t = 6.37, P < 0.001; 2-years-since-fire vs not yet burned, t = 3.19, P < 0.02). By the late season sampling, K was only significantly higher in recently burned patches (recently burned vs 1-year-since-fire, t = 4.6, P < 0.001; recently burned vs 2-years-since-fire, t = 3.73, P = 0.001; recently burned vs not yet burned, t = 7.28, P < 0.001). Early season K concentrations across all treatments exceeded recommendations, but late in the season only recently burned patches had K concentration above recommended levels.
Magnesium (Mg) was not different between any time since fire patches early in the grazing season, but as with Ca, Mg concentration was greater in the recently burned patch than all other patches by late grazing season sampling (recently burned vs 1-year-since-fire, t = 4.78, P < 0.001; recently burned vs 2-years-since-fire, t = 4.94, P < 0.001; recently burned vs not yet burned, t = 7.2, P < 0.001). Mg was consistently below recommended levels in all patches.
Manganese (Mn) concentration did not differ among patches early in the season, but late in the season Mn was greater in recently burned and 1-year-since-fire patches than the not yet burned (recently burned vs not yet burned, t = 2.72, P = 0.04; 1-year-since-fire vs not yet burned, t = 3.67, P = 0.002). All patches exceeded Mn recommendations.
Phosphorous (P) concentration was greater in recently burned patches than all other patches during both early and late season sampling (recently burned vs 1-year-since-fire, t = 3.7, P = 0.001; recently burned vs 2-years-since-fire, t = 4.46, P < 0.001; recently burned vs not yet burned, t = 8.59, P < 0.001). All patches that had been previously burned exceeded early season lactating cow recommendations for P, while all samples exceeded recommendations late in the season.
Early in the season, zinc (Zn) was greater in recently burned patches than unburned patches (recently burned vs not yet burned, t = 3.43, P = 0.004), and by the end of the season, zinc in recently burned patches exceeded all other patches (recently burned vs 1-year-since-fire, t = 5.23, P < 0.001; recently burned vs 2-years-since-fire, t = 5.17, P < 0.001; recently burned vs not yet burned, t = 8.9, P < 0.001). Only the most recently burned patches met requirements for Zn.
The PCA showed clusters of different time since fire groups, with calcium, zinc, magnesium, manganese, copper, phosphorous, and potassium more strongly associated with recently burned patches than those that had not yet been burned (Fig. 2). The first three axes explained 70% of variation in forage mineral concentration. Values along PCA Axis 1 (32% of variation) and PCA Axis 2 (24% of variation) fairly equally associated with all minerals. However, iron was not associated with PCA Axis 1 and is likely responsible for much of the variability. Time since fire (r2 = 0.13, P = 0.005) and season (r2 = 0.25, P = 0.005) were each significantly correlated with variability within the PCA. While year was a statistically significant term, it had very low correlation with variation in the PCA (r2 = 0.05, P = 0.005). In pairwise tests, forage mineral concentration of patches between the 1- and 2-years-since-fire measurements were not significantly different from each other (P = 0.13), while all other patches were significantly different (P = 0.012). Pairwise comparisons also indicated that 2017 was significantly different than all other years of sampling (P = 0.02), which were not different from each other.
Discussion
Patch-burning generally increased forage mineral concentration in recently burned patches for the extent of the grazing season, creating a potential benefit for use of burned grazing lands. Most of the eight minerals tested exceeded forage mineral concentration recommendations for beef cattle nutrition throughout the grazing season on recently burned patches. While unlikely to eliminate the added cost of mineral supplementation for cattle grazing in extensively-managed grassland, livestock producers might find burning patches within pastures can simultaneously reduce input costs and enhance conservation outcomes. Likewise, leasees of public grazing lands affected by wildfire might also realise potential benefits to livestock within burned allotments, assuming stocking is otherwise determined to be appropriate.
The higher forage mineral concentrations observed here are likely due to the combined interaction of fire and grazing implemented in patch-burn grazing, rather than fire alone. While most studies have found some minerals to increase with fire, specific mineral responses vary among studies and fire effects are generally limited to the first 3 months after burning in east Africa (Van de Vijver et al. 1999; Eby et al. 2014). For example, some studies reported greater forage calcium concentrations following fire (Eby et al. 2014; Vermeire et al. 2020), while another study reported that not only did calcium increase, but the increase persisted longer than other minerals (Van de Vijver et al. 1999). However, in our study, all minerals except iron were highest in the most recently-burned patch 5 months after spring burns. Furthermore, in the spring following fires, copper, potassium, and manganese remained higher in patches 1 year after burning compared with patches that had not yet been burned. This indicates that patch-burning sustains some mineral increases beyond the first grazing season post-fire. Higher mineral levels have been attributed to younger plant tissue, greater leaf to stem ratio, and higher concentration due to the low biomass of post-fire vegetation (Van de Vijver et al. 1999). Therefore, differences in the duration of mineral increase between our study and previous research are likely due to continued attraction and grazing of the recent burn patch, which delays plant maturity.
In addition to the general fire effect, we observed seasonal changes in forage mineral concentrations (Fig. 1). Similar seasonal patterns were observed in a rotational stocking study, in which seasonal mineral fluctuations persisted even though forage remained in an immature state (Kappel et al. 1985). Seasonal differences in forage minerals with fire have not been well established in previous research (Van de Vijver et al. 1999; Anderson et al. 2007; Eby et al. 2014; Vermeire et al. 2020), but were apparent in all minerals we measured. The same factors that affect grassland mineral concentration such as plant species, soil characteristics, precipitation, and maturity also influence mineral concentration in grassland managed with fire (Suttle 2010; Arthington and Ranches 2021). Although forage mineral concentration in our study was highest in recently burned patches, phosphorous, potassium and copper still declined as expected in forage managed without fire (Metson and Saunders 1978; Suttle 2010). While zinc is expected to decline as the grazing season advances, we only observed this trend in areas that had not been burned, with patches that received fire increasing over the course of the grazing season.
Mineral concentration in recently burned patches was generally adequate to meet recommended levels of calcium, phosphorous, potassium, zinc, iron, and manganese for lactating cows (NASEM 2016). Calcium, phosphorous, magnesium, and potassium recommendations are dependent upon cow size, physiological state, and milk production (NASEM 2016); therefore, so requirements will vary between individual animals and throughout the grazing season. However, plant tissue containing recommended mineral levels does not indicate that animal requirements are actually being met (Suttle 2010). Factors such as the location of the mineral in the plant, chemical form, and mineral interactions all influence bioavailability (i.e. accessibility, absorbability, retainability, functionality) of minerals, thereby modulating the capacity of minerals in forage to actually satisfy requirements (Suttle 2010). Conversely, even if forage mineral concentration does not meet requirements, it does not necessarily mean animals will be deficient (Arthington and Ranches 2021). Cattle can often withstand short periods of dietary mineral inadequacy because bones, blood, and liver provide a substantial pool of minerals to draw from when needed (Spears and Weiss 2014; Arthington and Ranches 2021).
Mineral ratios are an important consideration when determining cattle mineral requirements since many minerals interact with each other. Calcium to phosphorous ratios between 1:1 and 7:1 are considered to produce similar cattle performance (NASEM 2016). Early grazing and late summer calcium to phosphorous ratios were within the recommendations at 1.2:1 and 5:1 in our study. Ratios of potassium to calcium plus magnesium are important to consider in early grazing season for the prevention of grass tetany, with incidence of disease increasing linearly above 2.2:1 ratio (Kemp and t’ Hart 1957; Suttle 2010). In our recently burned patch, the tetany ratio is 4:1, potentially increasing the likelihood of grass tetany occurrence in cows. As a result, mineral supplementation to cattle grazing patch-burn pastures may still be required to adequately meet requirements and prevent mineral-related disease.
Copper levels were consistently below recommendations (NASEM 2016), and the higher concentration of copper following fire might not be sufficient to overcome antagonistic interactions with molybdenum, sulfur, and zinc (Suttle 2010). Zinc concentrations were high in the recently burned patch, especially late in the grazing season, and since both minerals use the same absorption sites in the animal, copper absorption is likely to be impaired (Suttle 2010). Other studies have also found increases in sulfur with prescribed fire compared to control plots, which further indicates bioavailability of copper is likely decreased with patch-burning (Vermeire et al. 2020). Drinking water can also be high in sulfur, reducing copper concentration in beef cattle (Smart et al. 1986; Penner et al. 2020). While we did not measure molybdenum concentration of forage, a previous study at the same location found forage molybdenum in continuous grazing pastures to be below maximum recommended intake levels (McCarthy et al. 2021). To our knowledge, no previous work has examined fire’s impact on molybdenum, but levels reported by McCarthy et al. (2021) in the absence of burning would be high enough to impair copper absorption due to low copper levels (Paterson and Engle 2005).
Lower amounts of fibrous forage components in recently burned patches might provide additional benefits for mineral absorption in livestock. Recently burned patches are lower in acid detergent fibre, which includes lignin and cellulose, than other patches (Dufek et al. 2014; Gullap et al. 2018; Wanchuk et al. 2024). Lignin and cellulose often form clusters, bound by minerals, which reduces the absorbability of these minerals (Moreira et al. 2013). Therefore, fire could both increase mineral concentration and absorption of those minerals via decreasing acid detergent fibre in forage from recently burned patches.
Precipitation plays a strong role in mineral concentration patterns and differences between mineral concentrations in 2017 and subsequent years (data not shown) is likely attributable to a drought during the 2017 grazing season (Lalman and McMurphy 2009; Suttle 2010; Spiess et al. 2020). Drought can increase forage mineral concentration for most minerals, while above average precipitation years tend to decrease forage mineral concentrations (Grings et al. 1996; Ganskopp and Bohnert 2003). In 2019, the study area had above average growing season precipitation that did not appear to affect forage mineral concentrations relative to 2018, which had average precipitation. All previous studies cited here evaluated mineral concentration for just one or two growing seasons. Some of the mineral concentration variability seen with previous studies is likely due to variable precipitation patterns, and timing of fire in relation to wet or dry seasons over short study durations (Van de Vijver et al. 1999; Eby et al. 2014; Vermeire et al. 2020).
Soil pH can directly influence mineral uptake by plants (Suttle 2010) and might interact with plant uptake in our grasslands. Immediate increases of soil pH are a common fire effect, as heating-induced mineralisation releases cations into the soil (McGranahan and Wonkka 2021). While we did not measure soil pH in our sampling, a concurrent study conducted on the same burned areas we report on here found post-fire soil pH ranged from 6.2 to 7.2 (Gerhard et al. 2022). At similar soil pH, Suttle (2010) reported lower manganese and zinc concentrations than under lower pH. But we found higher forage manganese and zinc concentrations in samples from the recently-burned patches in our study. Given that Gerhard et al. (2022) reported no difference between pH across burned and unburned areas, pH was likely not affected by fire, and as such the higher mineral concentrations observed here are likely more attributable to altered plant physiology, morphology, and/or phenology than to soil pH (Van de Vijver et al. 1999).
This study provides a basis for understanding forage mineral concentrations when grazing patch-burned pastures; however, gaps are apparent in current literature. While prescribed fire increased forage mineral concentration, further research is needed to determine if these differences are also apparent within the cattle themselves. Differences between patch-burning and other grazing strategies should also be explored to further determine how grazing management impacts forage mineral concentrations. Because of potential trace mineral antagonistic effects between copper, sulfur, and molybdenum, future research should include these minerals in analysis to provide a better understanding of how patch-burning alters mineral concentration requirements. Vitamin and mineral supplementation of cattle often coincide, but to our knowledge, there are no published studies examining the effects of forage vitamin concentration with prescribed fire, which could be another potential avenue for future research.
Conclusions
Our study suggests that fire increases the mineral concentration of grassland forage. Furthermore, when prescribed patch burning is applied at least once a year, an area is created with forage regrowth in each grazing season following the fire. Patch burning could thus potentially sustain mineral concentrations for longer periods than fire alone, due to delaying forage maturity. While mineral concentrations varied between the beginning and end of grazing sampling, the recently burned patch was above recommendations except for copper and early season magnesium. Supplementation is still likely necessary to ensure adequate copper and prevent grass tetany in the early grazing season due to low magnesium concentrations. Greater forage mineral concentration in recently burned areas has the potential to reduce producer mineral supplementation costs and increase cow performance, which is a benefit to livestock production following both prescribed fire and wildfire in fire-prone, extensively-managed grazing lands.
Data availability
The data that support the findings of this study are openly available in USDA National Agricultural Library Ag Data Commons at https://doi.org/10.15482/USDA.ADC/25464097.
Declaration of funding
This research was supported by the NDSU Central Grasslands Research Extension Centre.
References
Anderson TM, Ritchie ME, Mayemba E, Eby S, Grace JB, McNaughton SJ (2007) Forage nutritive quality in the serengeti ecosystem: the roles of fire and herbivory. American Naturalist 170, 343-357.
| Crossref | Google Scholar | PubMed |
Archibald S, Bond WJ, Stock WD, Fairbanks DHK (2005) Shaping the landscape: Fire-grazer interactions on an African savanna. Ecological Applications 15, 96-109.
| Crossref | Google Scholar |
Arthington JD, Ranches J (2021) Trace mineral nutrition of grazing beef cattle. Animals 11, 2767.
| Crossref | Google Scholar | PubMed |
Bates D, Mächler M, Bolker B, Walker S (2015) Fitting linear mixed-effects models using lme4. Journal of Statistical Software 67, 1-48.
| Crossref | Google Scholar |
Bond WJ (2022) Out of the shadows: ecology of open ecosystems. Plant Ecology & Diversity 14, 205-222.
| Crossref | Google Scholar |
Burnham KP, Anderson DR (2004) Multimodel inference: understanding AIC and BIC in model selection. Sociological Methods & Research 33, 261-304.
| Crossref | Google Scholar |
Dufek NA, Vermeire LT, Waterman RC, Ganguli AC (2014) Fire and nitrogen addition increase forage quality of Aristida purpurea. Rangeland Ecology & Management 67, 298-306.
| Crossref | Google Scholar |
Duquette C, McGranahan DA, Wanchuk M, Hovick T, Limb R, Sedivec K (2022) Heterogeneity-based management restores diversity and alters vegetation structure without decreasing invasive grasses in working mixed-grass prairie. Land 11, 1135.
| Crossref | Google Scholar |
Eby SL, Anderson TM, Mayemba EP, Ritchie ME (2014) The effect of fire on habitat selection of mammalian herbivores: the role of body size and vegetation characteristics. Journal of Animal Ecology 83, 1196-1205.
| Crossref | Google Scholar | PubMed |
Fuhlendorf SD, Engle DM (2004) Application of the fire–grazing interaction to restore a shifting mosaic on tallgrass prairie. Journal of Applied Ecology 41, 604-614.
| Crossref | Google Scholar |
Fuhlendorf SD, Fynn RWS, McGranahan DA, Twidwell D (2017) Heterogeneity as the Basis for Rangeland Management. In ‘Rangeland Systems’. Springer Series on Environmental Management. (Ed. DD Briske) pp. 169–196. (Springer International Publishing: Cham, Switzerland) 10.1007/978-3-319-46709-2_5
Ganskopp D, Bohnert D (2003) Mineral concentration dynamics among 7 northern Great Basin grasses. Journal of Range Management 56, 174-184.
| Crossref | Google Scholar |
Gates EA, Vermeire LT, Marlow CB, Waterman RC (2017) Reconsidering rest following fire: Northern mixed-grass prairie is resilient to grazing following spring wildfire. Agriculture, Ecosystems & Environment 237, 258-264.
| Crossref | Google Scholar |
Gerhard L, Gasch CK, Sedivec K (2022) Soil properties are resilient despite grass invasion, fire, and grazing. Agrosystems, Geosciences & Environment 5, e20257.
| Crossref | Google Scholar |
Greene LW (2000) Designing mineral supplementation of forage programs for beef cattle. Journal of Animal Science 77, 1-9.
| Google Scholar |
Grings EE, Haferkamp MR, Heitschmidt RK, Karl MG (1996) Mineral dynamics in forages of the Northern Great Plains. Journal of Range Management 49, 234-240.
| Crossref | Google Scholar |
Gullap MK, Erkovan S, Erkovan HI, Koc A (2018) Effects of fire on litter, forage dry matter production, and forage quality in steppe vegetation of Eastern Anatolia, Turkey. Journal of Agriculture and Technology 20, 61-70.
| Google Scholar |
Hervé M (2018) RVAideMemoire: testing and plotting procedures for biostatistics. R Package Version 09-69 3. Available at https://cran.r-project.org/package=RVAideMemoire
Kappel LC, Morgan EB, Kilgore L, Ingraham RH, Babcock DK (1985) Seasonal changes of mineral content of Southern forages. Journal of Dairy Science 68, 1822-1827.
| Crossref | Google Scholar |
Kemp A, t’ Hart ML (1957) Grass tetany in grazing milking cows. Netherlands Journal of Agricultural Science 5, 4-17.
| Crossref | Google Scholar |
Kral-O’Brien KC, Sedivec KK, Geaumont BA, Gearhart AL (2020) Resiliency of native mixed-grass rangelands and crested wheatgrass pasture lands to spring wildfire. Rangeland Ecology & Management 73, 119-127.
| Crossref | Google Scholar |
McCarthy KL, Undi M, Becker S, Dahlen CR (2021) Utilizing an electronic feeder to measure individual mineral intake, feeding behavior, and growth performance of cow–calf pairs grazing native range. Translational Animal Science 5, txab007.
| Crossref | Google Scholar | PubMed |
McDowell LR (1996) Feeding minerals to cattle on pasture. Animal Feed Science and Technology 60, 247-271.
| Crossref | Google Scholar |
McGranahan D, Kirkman K (2013) Multifunctional rangeland in Southern Africa: managing for production, conservation, and resilience with fire and grazing. Land 2, 176-193.
| Crossref | Google Scholar |
McGranahan DA, Wonkka CL (2024) Pyrogeography of the Western Great Plains: a 40-year history of fire in semi-arid rangelands. Fire 7, 32.
| Crossref | Google Scholar |
McGranahan DA, Engle DM, Fuhlendorf SD, Winter SJ, Miller JR, Debinski DM (2012) Spatial heterogeneity across five rangelands managed with pyric-herbivory. Journal of Applied Ecology 49, 903-910.
| Crossref | Google Scholar |
McGranahan DA, Engle DM, Fuhlendorf SD, Winter SL, Miller JR, Debinski DM (2013) Inconsistent outcomes of heterogeneity-based management underscore importance of matching evaluation to conservation objectives. Environmental Science & Policy 31, 53-60.
| Crossref | Google Scholar |
McGranahan DA, Zopfi ME, Yurkonis KA (2023) Weather and fuel as modulators of grassland fire behavior in the northern Great Plains. Environmental Management 71, 940-949.
| Crossref | Google Scholar | PubMed |
Metson AJ, Saunders WMH (1978) Seasonal variations in chemical composition of pasture: I. Calcium, magnesium, potassium, sodium, and phosphorus. New Zealand Journal of Agricultural Research 21, 341-353.
| Crossref | Google Scholar |
Moreira LM, Leonel F de P, Vieira RAM, Pereira JC (2013) A new approach about the digestion of fibers by ruminants. Revista Brasileira de Saúde e Produção Animal 14, 382-395.
| Crossref | Google Scholar |
NASEM (National Academies of Sciences, Engineering, and Medicine) (2016) ‘Nutrient Requirements of Beef Cattle.’ 8th Revised edn. (National Academies Press: Washington, DC, USA) 10.17226/19014
NDAWN (2023) ‘30-year daily weather for Streeter, ND 1990-2020.’ (North Dakota Ag Weather Network) Available at https://ndawn.ndsu.nodak.edu/get-table.html?station=48&variable=ddavt&variable=ddr&year=2023&ttype=daily&quick_pick=&begin_date=1990-05-01&end_date=2020-09-30
NRCS (2023) ‘Soil Survey of Kidder County ND. Web Soil Survey.’ (Soil Survey Staff, USDA-Natural Resources Conservation Service) Available at https://websoilsurvey.nrcs.usda.gov/app/WebSoilSurvey.aspx
Oksanen J, Blanchet FG, Friendly M, Kindt R, Legendre P, McGlinn D, Minchin PR, O’Hara RB, Simpson GL, Solymos P, Stevens MHH, Szoecs E, Wagner H (2016) vegan: Community Ecology Package. Available at https://cran.r-project.org/web/packages/vegan/index.html
Penner GB, Johnson JA, Sutherland BD, Clark LP, Elford CJ (2020) Effects of drinking water sulfate concentrations on feed and water intake, growth, and serum mineral concentrations in growing beef heifers. Applied Animal Science 36, 201-207.
| Crossref | Google Scholar |
R Core Team (2019) R: A Language and Environment for Statistical Computing (v. 3.5.3). Available at https://www.R-project.org/
Smart ME, Cohen R, Christensen DA, Williams CM (1986) The effects of sulphate removal from the drinking water on the plasma and liver copper and zinc concentrations of beef cows and thier calves. Canadian Journal of Animal Science 66, 669-680.
| Crossref | Google Scholar |
Spears JW, Weiss WP (2014) Invited Review: Mineral and vitamin nutrition in ruminants. The Professional Animal Scientist 30, 180-191.
| Crossref | Google Scholar |
Spiess JW, McGranahan DA, Geaumont B, Sedivec K, Lakey M, Berti M, Hovick TJ, Limb RF (2020) Patch-burning buffers forage resources and livestock performance to mitigate drought in the northern Great Plains. Rangeland Ecology & Management 73, 473-481.
| Crossref | Google Scholar |
Spiess JW, McGranahan DA, Berti MT, Gasch CK, Hovick T, Geaumont B (2024) Spatio-temporal patterns of rangeland forage nutritive value and grazer selection with patch-burning in the US northern Great Plains. Journal of Environmental Management 357, 120731.
| Crossref | Google Scholar | PubMed |
Umoh JE, Harbers LH, Smith EF (1982) The effects of burning on mineral contents of Flint Hill Range forages. Journal of Range Management 35, 231-234.
| Crossref | Google Scholar |
Van de Vijver CADM, Poot P, Prins HHT (1999) Causes of increased nutrient concentrations in post-fire regrowth in an East African savanna. Plant and Soil 214, 173-185.
| Crossref | Google Scholar |
Vermeire LT, Strong DJ, Gates EA, Marlow CB, Waterman RC (2020) Can mowing substitute for fire in semiarid grassland? Rangeland Ecology & Management 73, 97-103.
| Crossref | Google Scholar |
WallisDeVries MF, Laca EA, Demment MW (1998) From feeding station to patch: scaling up food intake measurements in grazing cattle. Applied Animal Behaviour Science 60, 301-315.
| Crossref | Google Scholar |
Wanchuk MR, McGranahan DA, Sedivec KK, Berti M, Swanson KC, Hovick TJ (2024) Improving forage nutritive value and livestock performance with spatially-patchy prescribed fire in grazed rangeland. Agriculture, Ecosystems, and Environment 368, 109004.
| Crossref | Google Scholar |
Williams AR, Vermeire LT, Waterman RC, Marlow CB (2022) Grazing and defoliation timing effects in Great Plains ponderosa pine woodland following a large summer wildfire. Forest Ecology and Management 520, 120398.
| Crossref | Google Scholar |