Impacts of changing fire regimes on hollow-bearing trees in south-eastern Australia
Philip Gibbons A * , Dejan Stojanovic A , David B. Lindenmayer A and Giselle Owens AA
Abstract
Many species use hollows or cavities that form in trees. The effect of an increasing fire frequency on hollow-bearing trees is unclear.
To predict the effects of increasing fire frequency on the abundance of hollow-bearing trees and identify how to make forests more resilient to these changes.
We simulated how increasing fire frequency will affect the abundance of hollow-bearing trees in forests of south-eastern Australia and conducted a sensitivity analysis to identify which variables affect these predictions.
Other things being equal, we found a negative relationship between the number of hollow-bearing trees and increasing fire frequency. However, we identified scenarios where the number of hollow-bearing trees remained stable, or increased, with frequent fires.
Hollow-bearing trees will decline where frequent fires co-occur with high rates at which trees collapse (or are removed) and/or where there are not a sufficient number of suitable mature trees in which new hollows can be excavated by fire.
The impact of increasing fire frequency on hollow-dependent fauna is likely to be greatest in forests where regeneration is inhibited, a large number of trees are removed before they form hollows, and/or where rates of collapse among trees is elevated.
Keywords: cavity, climate change, Eucalyptus, fire regime, forecasting, forests, simulation, tree hollows, wildfire.
Introduction
Many organisms have evolved to use hollows (or cavities) that form in trees for denning, roosting, nesting, as a growing substrate, for feeding and/or to avoid predation (von Haartman 1957; Gibbons and Lindenmayer 2002). Newton (1994) estimates that 4–11% of the world’s birds are obligate hollow-nesters. Novak (2005) recorded 456 species using tree cavities in Slovenia. Thomaes et al. (2015) recorded 161 beetle species from tree cavities in Belgium, and Gibbons and Lindenmayer (2002) estimate that 300 vertebrate species utilise tree hollows in Australia.
Trees with hollows have declined, or are forecast to decline, in landscapes managed for agriculture (Gibbons et al. 2008; Hartel et al. 2013), wood production (Linder and Östlund 1998; Cary et al. 2021) and residential development (Le Roux et al. 2014). This is because trees are removed before they can grow old enough to form hollows, and/or new hollow-bearing trees are not replaced at the same rate they are lost (Ranius et al. 2009; Cary et al. 2021). Populations of organisms that have long generation times (or rely on habitat that has long generation times) are sensitive to phenomena that increase mortality and reduce recruitment (Jennings et al. 1998; Norse et al. 2012; Turkalo et al. 2017). Thus, fauna that use hollows that form only in very old trees are also sensitive to the rates at which these trees collapse and are recruited (Gibbons et al. 2008).
Forest fires are a disturbance that can elevate the mortality and collapse of trees. Higher rates of collapse among hollow-bearing trees have been observed in Australian Eucalyptus forests after wildfire (Inions et al. 1989; Stojanovic et al. 2016; Lindenmayer et al. 2018) and prescribed burning (Conner et al. 1991; Bagne et al. 2008; Bluff 2016). Some studies have observed that the number of hollow-bearing trees that occur in stands is negatively associated with fire severity (Inions et al. 1989; Lindenmayer et al. 2018) and fire frequency (Bagne et al. 2008; Haslem et al. 2012; Salmona et al. 2018; Woolley et al. 2018). However, fire is also an agent for hollow formation. Fire can damage or kill trees, predisposing them to decay and hollow formation, and cause limbs to break, thus exposing hollows in decayed heartwood and/or excavate hollows by burning decayed heartwood (Inions et al. 1989; Gibbons and Lindenmayer 2002). Indeed, fire is a natural disturbance in most forests in south-eastern Australia, and most hollow-bearing trees have evidence of damage by fire (Gibbons 1999; Stojanovic et al. 2016).
Climate change is, or is predicted to be, associated with a larger area burnt, increasing fire frequency and/or increasing fire severity in several regions of the world (Westerling et al. 2006; Moritz et al. 2012). In Australia, Clarke et al. (2013) and Dowdy (2018) reported that the severity of fire weather has generally increased over the last 40 years, and Lucas et al. (2007) predicted there will be 10–300% more days of extreme fire danger in south-eastern Australia by 2050. Although changes to the climate may diminish fire activity over parts of Australia due to the effect on fuel accumulation (Bradstock 2010), Cary (2002), Bradstock (2010), King et al. (2013) and Abram et al. (2021) predicted that the effects of a changing climate on fire regimes in Australia’s temperate forests are likely to be realised as increasing fire extent. Abram et al. (2021) added that climate change would increase the intensity of fires in this region. In the most comprehensive study on changing fire regimes in south-eastern Australia, Canadell et al. (2021) observed that the main changes to fire regimes have been an increase in the area of forest burned and decreasing fire interval. For example, Canadell et al. (2021) reported that the mean number of years since the last fire has declined from 56–112 to 18–47 years over the last 30 years in forests across the study area.
Fire regimes outside the range of historic variation are difficult to evaluate based on retrospective studies. This is exacerbated for a slow response variable such as tree hollows, which typically take >120–220 years to develop in trees of the genus Eucalyptus (Gibbons and Lindenmayer 2002). Ecological forecasting can be used to anticipate change (Clark et al. 2001) and inform adaptive monitoring and management (Dietze et al. 2018). In this study, we therefore developed a simulation model to: (1) predict the effects of increasing fire frequency on numbers of hollow-bearing trees that occur in forests; and (2) identify management options that can make forests more resilient to these changes.
We hypothesise that a shorter interval between fires will reduce the number of hollow-bearing trees in the forests of south-eastern Australia. This is because higher rates of collapse of hollow-bearing trees have been observed after fires (Inions et al. 1989; Parnaby et al. 2010; Bluff 2016; Stojanovic et al. 2016; Lindenmayer et al. 2018) and thus the more fires to which a stand is exposed, the greater rate that trees will collapse before they reach an age that they typically form hollows (>120–220 years).
Methods
Study area
Our study focused on temperate forest ecosystems in south-eastern Australia dominated by the genus Eucalyptus. Fires in these forests have become larger and more frequent over time due to climate change (Canadell et al. 2021). We focused on 10 widespread tree species that occur in eastern Victoria, eastern New South Wales, the Australian Capital Territory and/or south-eastern Queensland for which we could source sufficient data (Supplementary Table S1).
Simulation model
We forecast impacts of increasing fire frequency on hollow-bearing trees using a modified version of a model developed to simulate mature and hollow-bearing trees in wood production forests, agricultural landscapes and urban areas (Gibbons et al. 2008, 2010; Fischer et al. 2010; Manning et al. 2013; Le Roux et al. 2014). Because many of the factors that influence the number of hollow-bearing trees are inherently variable or uncertain, we forecast impacts of increasing fire frequency on hollow-bearing trees using a Monte Carlo simulation. That is, the simulation model was run 400 times with key parameters in the model drawn randomly from a range of feasible values for each run of the model, so changes to, or uncertainty in, each of these parameters on hollow-bearing trees could be evaluated in sensitivity analyses. Data used to populate the simulation model are discussed in the next section.
Our model simulated the dynamics of hollow-bearing trees in stands as follows:
All simulations began with a stand (a small area of forest that shares similar abiotic and biotic features) containing one hollow-bearing tree with a diameter at breast height (DBH) randomly drawn from a uniform distribution between 100 and 150 cm and a random number (0–10) of additional trees without hollows with a DBH drawn randomly from a range of values between 50 and 80 cm.
The age of each tree initially retained in the stand was predicted using relationships between tree age and DBH for each tree species and the DBH for each living tree was predicted from tree age using the inverse of this relationship every 5 years.
Fires were introduced stochastically. The probability of fire in a given year was based on a mean fire interval selected randomly from a range of values. If a random value drawn from a uniform distribution between 0 and 1 was less than the probability of fire in a given year, then a fire occurred.
The proportion of trees that collapsed annually was selected randomly from a uniform distribution of values, with a different rate of collapse employed in the 5 years after fires.
Trees that did not collapse had a finite standing life, which was selected randomly from a range of ages that the tree species in the study area are known to attain plus the expected standing life of dead trees.
Sufficient new trees were recruited into a stand at each fire event to keep the number of stems initially retained at step 1 constant. For example, if five trees were initially retained in addition to one hollow-bearing tree, then sufficient new trees were recruited at each fire event to ensure six trees occurred in the stand.
The number of standing trees, their age, diameter, whether they were living or dead and the number of trees with hollows was recorded every 5 years for the duration of the simulation (300 years).
Data used to populate the simulation model
Mortality and collapse among trees occur continuously due to processes such as competition, disease and senescence. Spikes in mortality and collapse among trees in Eucalyptus-dominated forests occur with significant disturbances such as fire (Inions et al. 1989; Conner et al. 1991; Bagne et al. 2008; Fairman et al. 2015; Bluff 2016; Stojanovic et al. 2016; Lindenmayer et al. 2018). The majority of trees of the species examined here are not killed by fire, and even when trees die, they may remain standing and provide hollows suitable for fauna (Gibbons and Lindenmayer 2002). Therefore, rates at which trees collapse is more important than rates of mortality, with respect to the abundance of tree hollows in a stand.
There are relatively little published data on the rates at which trees collapse after fire in Eucalyptus forest. In the montane ash forests of Victoria, which are outside our study area and include tree species that are killed by fire, Lindenmayer and Wood (2010) observed that hollow-bearing trees collapsed at a rate of 3.6–8.5% per annum over 15 years. In forests dominated by mountain ash (E. regnans) in Victoria, 18–26% of trees collapsed after fire over 10 years (Lindenmayer et al. 2023). In drier forests inland from our study area, Parnaby et al. (2010) observed that 15% of hollow-bearing trees collapsed in the first year after a planned burn. In Tasmania, also outside our study area, Stojanovic et al. (2016) predicted that 43% of nest trees for the swift parrot (Lathamus discolor) collapsed over 10 years after wildfire, compared with 4% in unburnt forest over the same period. In the southern part of our study area (East Gippsland, Victoria), Bluff (2016) found that 19.3% of hollow-bearing trees within the boundary of planned burns collapsed within the first year after fire, rising to 26.5% for hollow-bearing trees that were directly impacted by fire.
We also examined published data on the rates at which trees retained in harvested areas collapse after the post-harvest slash-burn. In the southern part of our study area, 0.5% of all trees collapsed within 2–5 years after harvesting and a low-severity slash-burn; and in the same forest type after harvesting and a high-severity slash-burn, the rate of collapse among all trees over the same period was 1.5% (Gibbons et al. 2000a). In Tasmania, Koch et al. (2018) examined collapse among trees in clumps retained within harvested areas treated with no post-harvest burning or a low severity post-harvest slash-burn. Over 8 years, 11.7% of all trees and 1.5% of hollow-bearing trees collapsed on harvested sites, compared with 2.8% of all trees and 0.3% of hollow-bearing trees in unharvested sites (Koch et al. 2018).
In summary, we selected annual rates of collapse of trees from a uniform distribution between the lower and upper bounds of annual collapse reviewed. Using the following formula for mortality:
where S is the proportion of trees surviving after t years, annual rates of collapse among trees in unburnt forests were between 0.04 and 0.9% per annum; and in the first 5 years after fire, annual rates of collapse were between 0.1 and 5.7% per annum (excluding estimates for montane ash forests, which do not occur in the study area and are more likely to be killed by fire than most of the tree species in our study area). Rates or mortality and/or collapse of trees after fire in different mixed-species Eucalyptus forest have been variously reported to be highest in the smaller DBH classes (Bennett et al. 2016; Prior et al. 2016), highest in the largest DBH classes (Forestry Corporation of NSW 2016) or not vary with DBH (Gibbons et al. 2000a; Koch et al. 2018). Given this uncertainty, we applied the same annual rates of collapse to all stems in each run of the model, although an additional rate of mortality is applied to reflect the maximum standing life of older stems as described in the next section. Nevertheless, as we discuss later, improved data are required for the rates at which trees collapse.
Eucalyptus species from the study area have a maximum life-span in the range of 300–400 years (Mackowski 1984; Gibbons et al. 2000b; Wormington et al. 2003). Once trees reach the end of their life-span, they may remain standing and provide suitable hollows as dead trees. Although dead trees appear to collapse at higher rates than living trees (Parnaby et al. 2010; Koch et al. 2018), there are no published estimates of the longevity of dead trees, other than an observation that ringbarked trees in alpine ash (E. delegatensis) forest remained standing as dead trees for at least 50 years (Shepherd 1957), and many dead trees killed in the fires of 1939 remain standing in the Central Highlands of Victoria (Lindenmayer and Wood 2010). For each run of the simulation model, we therefore selected the maximum age that trees are likely to remain living at random from a uniform distribution between 300 and 400 years, enabled dead trees to remain standing for a random length of time drawn from a uniform distribution between 0 and 100 years and applied the same rate of collapse to standing dead trees and living trees. Thus, the maximum standing life of trees was simulated to be 300–500 years.
The interval between fires was selected randomly for each run of the model. As indicated previously, Canadell et al. (2021) reported that the mean number of years since the last fire has declined from 56–112 to 18–47 years over the last 30 years in forests across south-eastern Australia. Prescribed burning, where it is employed, may occur as frequently as every 5 years (NSW Rural Fire Service 2006). We therefore selected the fire interval randomly for each run of the model from a uniform distribution between 5 and 60 years.
Tree age within the genus Eucalyptus can be predicted from DBH. We compiled coefficients to predict age from DBH for 10 Eucalyptus species in the study area (Supplementary Table S1) in the form:
where Age is tree age (years), a is the regression coefficient, DBH is tree diameter (cm) and we assumed a y-intercept of zero. Coefficients for predicting tree age from DBH were taken from published linear models for E. fastigata (H. Deane & Maiden), E. obliqua (L’Her.) and E. microcorys (F. Muell) (Supplementary Table S1). However, growth rates for most tree species of the genus Eucalyptus in the study area are published as diameter increments (Wormington and Lamb 1999; Bauhus et al. 2002; Bowman et al. 2014; Ngugi et al. 2015). Age can be predicted from tree diameter increments using the equation:
where Age is tree age (years) and b is the mean diameter increment for each tree species (cm year−1).Growth rates vary among trees of the same species and different species for many reasons (e.g. competition, site productivity, stochastic events such as fire, senescence), and there is uncertainty about the effects of climate change and changing fire regimes on growth rates of eucalypts (Murphy et al. 2010; Drake et al. 2015; Jordan et al. 2017). We implemented simple linear relationships between tree age and DBH based on the mean annual diameter increment over the life of each tree species. To simulate variation in growth rates for trees, a coefficient to predict age from DBH for each run of the model was selected randomly from a uniform distribution that was up to ±30% of the values for each species in Supplementary Table S1. This resulted in highly variable simulated rates at which each tree grows (Supplementary Fig. S1).
The occurrence of hollows visible in trees of the genus Eucalyptus is, on average, strongly associated with DBH (Lindenmayer et al. 1993; Wormington and Lamb 1999; Gibbons and Lindenmayer 2002). We estimated the proportion of trees by species and age-cohort that contain visible hollows, using DBH as the explanatory variable in generalised linear models (GLM) with a logit-link and binomial distribution (Supplementary Table S2). GLMs for the data in Williams (2001) were fitted for each species using stem counts for each diameter class as weights. To simulate changes in the rate at which hollow formation can occur in trees of the genus Eucalyptus after fire (Inions et al. 1989; McLean et al. 2015), a coefficient to predict the proportion of trees with hollows from DBH for each run of the model was selected randomly from a uniform distribution that was 1–2 times the values for each species in Supplementary Table S2 for the first 5 years after each fire. That is, we simulated a rate of hollow-development up to two times the mean after each fire.
Sensitivity analyses
We examined how increasing fire frequency and the other variables in the simulation model (Table 1) affected the number of hollow-bearing trees that are perpetuated in stands using a Monte Carlo approach in which the simulation model was run 400 times. In each run of the simulation model, we selected a value for each variable randomly from a uniform distribution between the values provided in Table 1.
Tree species | Coefficient for predicting age from DBH | Coefficient for predicting hollows from DBH | Maximum standing life of trees (years) | Fire interval (years) | Additional trees recruited into the stand (for each hollow-bearing tree) | Percentage of trees that collapse annually in the first 5 years after fire | Percentage of trees that collapse annually between fires | |
---|---|---|---|---|---|---|---|---|
1–10 in Supplementary Table S1 | ± up to 30% of the values in Supplementary Table S1 | 1–2 times the values in Supplementary Table S2 | 300–500 | 5–60 | 0–10 | 0.7–5.7 | 0.04–0.9 |
We examined how each of the variables in Table 1 affected the number of hollow-bearing trees by fitting a linear regression model in which the response variable was the mean predicted number of hollow-bearing trees over 300 years (n = 400), and the explanatory variables were the seven variables in Table 1. We selected the best additive model (lowest AIC) by first fitting all of the variables in Table 1 and then used an automated model selection algorithm (Barton 2022) to select the additive model with the lowest AIC value. We then tested whether the effect of increasing fire frequency is exacerbated by increasing fire severity by adding an interaction term to this model (i.e. fire interval × the annual collapse of trees after fire) and an interaction term to reflect the higher rate at which hollows can form in trees after fire (i.e. fire interval × the rate at which trees develop hollows after fire) and then compared these models using Akaike’s Information Criterion (AIC).
Before fitting models we log-transformed the response variable (i.e. the mean number of hollow-bearing trees occurring over 300 years) so it was normally distributed. We tested for correlation between covariates in the models by calculating variance inflation factors (VIFs) with the ‘Performance’ package in R (Lüdecke et al. 2021). All models were checked for homoscedasticity, normality and outliers among the residuals using the ‘DHARMa’ package in R (Hartig and Hartig 2017). We ranked the relative importance of variables in the additive model using the method described by Kruskal (1987) implemented within the ‘Relaimpo’ package in R (Grömping 2007).
Results
The best additive linear regression model (AIC = 69.97) used to predict which variables were most sensitive to the number of hollow-bearing trees predicted to occur over 300 years from 400 runs of the simulation model contained all of the variables in Table 1. This model had an adjusted R2 of 0.84. None of the variables in this model were highly correlated (VIFs ≤ 1.63). Predictions for each term in this model (while keeping the other terms constant) are illustrated in Fig. 1. The mean number of hollow-bearing trees predicted to occur over the long term increased with: the interval between fires, the number of additional trees recruited after each fire per hollow-bearing tree, the rate at which trees grow and form hollows and the standing life of trees; and decreased with the proportion of trees that collapse annually (in the first 5 years after fires and between fires). Tree species with faster growth rates (i.e. smaller coefficients used to predict age from DBH) were predicted to support more hollow-bearing trees than tree species with relatively slow growth rates. The relative importance of the variables used to predict how many hollow-bearing trees occurred are illustrated in Fig. 2. The number of trees recruited into stands after each fire was the most important variable affecting the numbers of trees with hollows over the long term.
Predicted mean (±95% confidence interval) numbers of hollow-bearing trees (expressed as a multiple of the number of hollow-bearing trees that initially occurred in the stand) over 300 years, with changes to each of the variables in the simulation model. The dashed horizontal lines intersect scenarios where the mean numbers of hollow-bearing trees remain constant over 300 years. Predictions for each variable are illustrated with the species fixed at C. maculata, the number of trees recruited after each fire = 4 and all other variables held at their median.
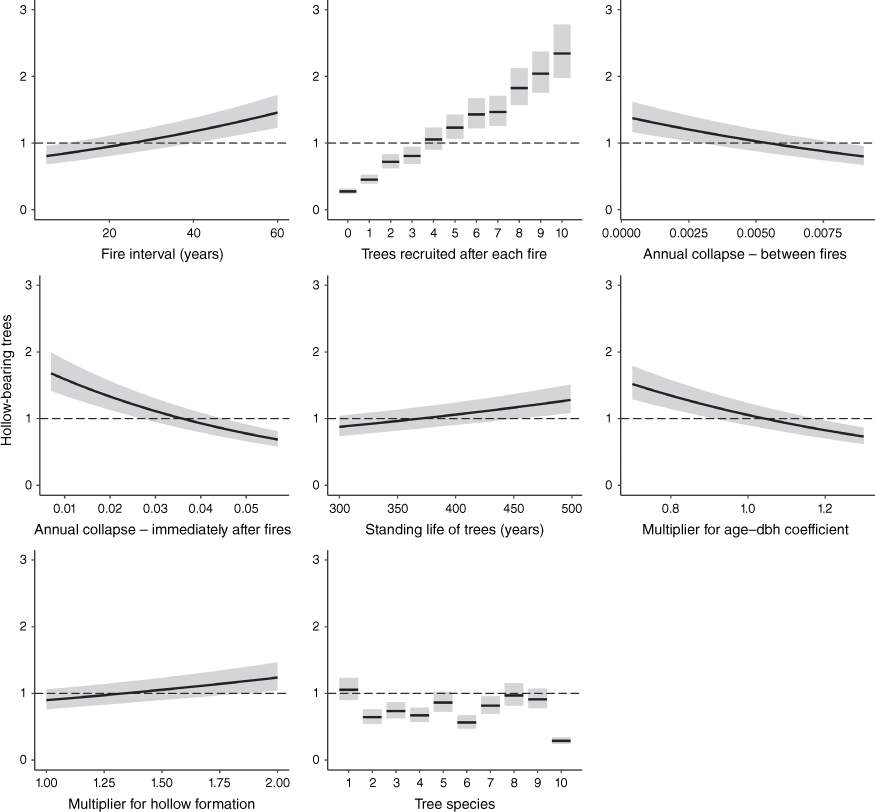
The relative importance of each variable in the linear regression model that contained all of the variables used in the Monte Carlo simulation.
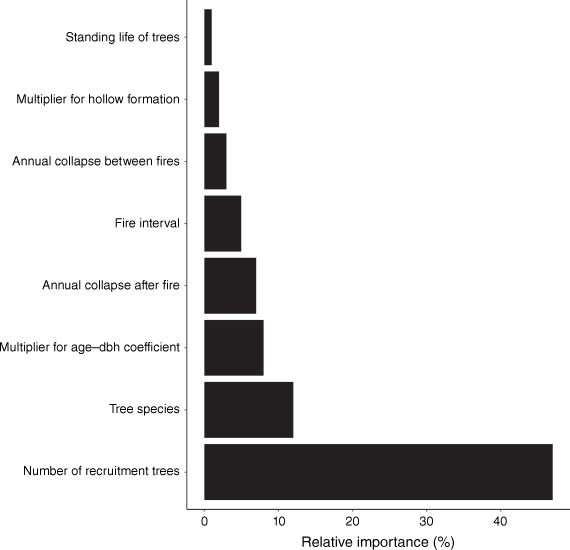
Adding the interaction terms (fire interval × the annual collapse of trees after fire and fire interval × the rate at which trees develop hollows after fire) improved the additive model. The regression model with the interaction terms added had an AIC of −4.38 (74.35 below the additive model) and an adjusted R2 of 0.87. Predictions from this model indicated that the number of hollow-bearing trees is likely to decline where higher fire frequencies co-occur with lower rates at which trees are recruited into the stand after each fire and there are higher rates of collapse (or removal) among all trees (Fig. 3). The number of hollow-bearing trees is predicted to remain stable, or increase, over the long term only where higher fire frequencies co-occur with the recruitment of multiple trees after each fire and there are low rates of collapse (or removal) among all trees (Fig. 3).
Predicted mean (±95% confidence interval) numbers of hollow-bearing trees over 300 years with: different rates of annual collapse among trees in the first 5 years after fire (x-axis); 1, 3 or 6 recruitment trees for each hollow-bearing tree; and for fire intervals of 5, 30 and 60 years. The dashed horizontal lines intersect scenarios where the mean numbers of hollow-bearing trees do not significantly change over 300 years. Predictions are based on a linear regression model containing all terms in Fig. 2 and interaction terms between fire interval and the rate at which trees collapse after fire. Predictions for each variable are illustrated with the species fixed at C. maculata, the rate of hollow formation in the first 5 years after fire fixed at two times higher than the mean rate and all other variables held at their median.
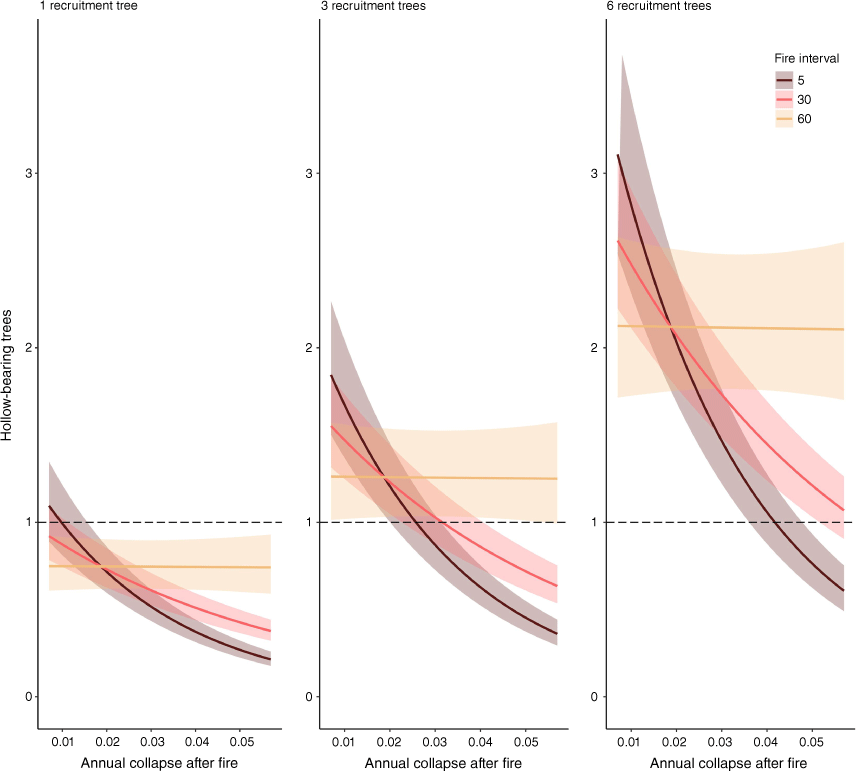
Discussion
Climate change is resulting in forest fires that occur more frequently in south-eastern Australia (Canadell et al. 2021). In this study we sought to predict the impacts of these changes on numbers of hollow-bearing trees that occur in forests and identify management options that will make forests more resilient to these changes.
Effects of changing fire regimes on the abundance of hollow-bearing trees
Although we found a negative relationship between the number of hollow-bearing trees and fire frequency (Fig. 2), there were scenarios where the number of hollow-bearing trees could remain constant, or even increase, with more frequent fires (Fig. 3). Therefore, our results only partially supported our hypothesis that a shorter interval between fires would reduce the number of trees with hollows in these forests.
Several other studies and simulations within other Eucalyptus-dominated forests and woodlands have observed or predicted fewer hollow-bearing trees where the fire interval is shorter (Bagne et al. 2008; Haslem et al. 2012; Salmona et al. 2018; Woolley et al. 2018; Cary et al. 2021). Where fire kills the majority of standing trees (e.g. the montane ash forests of southern Australia), these trees decay and collapse at a faster rate than living trees, and therefore fires must occur at sufficiently long interval to allow regenerating trees to develop hollows (Haslem et al. 2012; Cary et al. 2021). However, in mixed-species Eucalyptus forest, McLean et al. (2015) observed that the number of trees with hollows was positively associated with the number of fires that had occurred in the stand, but only where the stand was not harvested intensively for timber. The observations by McLean et al. (2015) are consistent with our simulations, which indicated that hollow-bearing trees decline where frequent fires co-occur with high rates at which trees collapse (or are removed) (Fig. 3).
Making forests more resilient to changing fire regimes
Our results indicated that hollow-bearing trees can be maintained at current numbers across the widest range of fire intervals in stands where there is a sufficient number of replacement or recruitment trees for every hollow-bearing tree and there is a low rate of collapse (or removal) among all trees. With all other variables in Table 1 fixed at their median or mode, we predicted that the number of hollow-bearing trees will not decline where ≥4 trees per extant hollow-bearing tree are recruited after each fire and the rate at which trees collapse (or are removed) between, and immediately after, fires remains at or below the approximate median simulated (Fig. 1). Fires are more likely to result in the creation of new hollows in larger or older trees (Lindenmayer et al. 1993; Wormington and Lamb 1999; Gibbons and Lindenmayer 2002). Thus, to be resilient to frequent fires, stands must always contain sufficient large trees in addition to each hollow-bearing tree. Sufficient recruitment of new trees and their protection over the long-term is therefore important and particularly applicable in forests in which natural regeneration is affected (e.g. due to over-grazing) or trees are removed before they reach an age where they begin to form hollows (e.g. due to timber harvesting). Indeed, McLean et al.(2015) observed that hollow-bearing trees declined in stands where multiple fires co-occurred with a history of intensive timber harvesting.
Our results implied that hollow-bearing trees can theoretically be maintained at current numbers, or even increased, in stands managed with frequent planned fires, provided these fires result in low rates at which trees collapse (Fig. 3). However, Bluff (2016) and Parnaby et al. (2010) observed rates of collapse among hollow-bearing trees in planned fires that occur at the upper range of the values published. This may be because the loss of hollow-bearing trees in mixed-species Eucalyptus forest is often associated with excavation of a cavity at the base of the tree, which can occur from a fire burning at low severity. Further, planned fires do not exclude subsequent wildfires, particularly in severe fire weather (Price 2012; Campbell et al. 2022), and thus frequent planned fires could exacerbate the effects of an increasing fire frequency. We therefore suggest that maintaining hollow-bearing trees using planned fires should only be implemented experimentally and monitored as part of a program of active adaptive management before it is adopted widely.
Adaptive monitoring
Models of the type described here can be used iteratively to inform forest management but also can be used iteratively to direct future monitoring which, in turn, can be used to improve management. Given we found that the number of hollow-bearing trees was sensitive to the rate at which trees grow, form hollows and collapse, there should be a focus on improving these data. For example, rates of collapse for trees were derived from three published studies (Gibbons et al. 2000a; Bluff 2016; Koch et al. 2018), and rates of collapse were applied uniformly across all DBH classes and to living and dead trees, which is simplistic given we know from studies in other Eucalyptus-dominated forests and woodlands that rates of mortality and/or collapse of trees after fire can vary with DBH (Bennett et al. 2016) and whether trees are living or dead (Lindenmayer and Wood 2010). We also used DBH to predict the proportion of trees with hollows that were visible from the ground. Most hollows visible from the ground are not suitable for occupancy by vertebrate fauna (Gibbons et al. 2002; Koch et al. 2008; Stojanovic et al. 2021), so our forecasts would be improved with better models to predict which trees are suitable for occupancy by hollow-dependent fauna.
Conclusion
Climate change is affecting the extent and thus the frequency of fires in south-eastern Australia. We predicted that the number of hollow-bearing trees is likely to decline where increasing fire frequencies co-occur with low rates of recruitment of new trees after fire and elevated rates at which trees collapse (or are removed) after fire. Forests are likely to be most resilient to changes in fire regimes where they contain several trees that co-occur with each hollow-bearing tree and rates of collapse (or removal) among trees is relatively low. Thus, impacts of changing fire regimes on fauna that use hollows will be greatest in forests where regeneration is inhibited (e.g. from over-grazing), the majority of trees are removed before they form hollows (e.g. due to timber harvesting) and/or where rates of collapse among trees is elevated (e.g. where frequent planned fires result in high rates of collapse among mature trees).
Data availability
The data that support this study are available in the ANU Data Commons at https://datacommons.anu.edu.au/DataCommons/.
References
Abram NJ, Henley BJ, Sen Gupta A, Lippmann TJ, Clarke H, Dowdy AJ, Sharples JJ, Nolan RH, Zhang T, Wooster MJ (2021) Connections of climate change and variability to large and extreme forest fires in southeast Australia. Communications Earth & Environment 2, 8.
| Crossref | Google Scholar |
Bagne KE, Purcell KL, Rotenberry JT (2008) Prescribed fire, snag population dynamics, and avian nest site selection. Forest Ecology and Management 255, 99-105.
| Crossref | Google Scholar |
Barton K (2022). MuMIn: Multi‐Model Inference. R package version 1.46.0, https://CRAN.R‐project.org/package=MuMIn.
Bauhus J, McElhinny C, Alcorn P (2002) Stand structure and tree growth in uneven‐aged spotted gum (Corymbia maculata) forests: some implications for management. Forestry 75, 451-456.
| Crossref | Google Scholar |
Bennett LT, Bruce MJ, MacHunter J, Kohout M, Tanase MA, Aponte C (2016) Mortality and recruitment of fire-tolerant eucalypts as influenced by wildfire severity and recent prescribed fire. Forest Ecology and Management 380, 107-117.
| Crossref | Google Scholar |
Bowman DMJS, Williamson GJ, Keenan RJ, Prior LD (2014) A warmer world will reduce tree growth in evergreen broadleaf forests: evidence from Australian temperate and subtropical eucalypt forests. Global Ecology and Biogeography 23, 925-934.
| Crossref | Google Scholar |
Bradstock RA (2010) A biogeographic model of fire regimes in Australia: current and future implications. Global Ecology and Biogeography 19, 145-158.
| Crossref | Google Scholar |
Campbell T, Bradshaw SD, Dixon KW, Zylstra P (2022) Wildfire risk management across diverse bioregions in a changing climate. Geomatics, Natural Hazards and Risk 13, 2405-2424.
| Crossref | Google Scholar |
Canadell JG, Meyer C, Cook GD, Dowdy A, Briggs PR, Knauer J, Pepler A, Haverd V (2021) Multi-decadal increase of forest burned area in Australia is linked to climate change. Nature Communications 12, 6921.
| Crossref | Google Scholar | PubMed |
Cary GJ, Blanchard W, Foster CN, Lindenmayer DB (2021) Effects of altered fire intervals on critical timber production and conservation values. International Journal of Wildland Fire 30, 322-328.
| Crossref | Google Scholar |
Clark JS, Carpenter SR, Barber M, Collins S, Dobson A, Foley JA, Lodge DM, Pascual M, Pielke Jr R, Pizer W, Pringle C, Reid WV, Rose KA, Sala O, Schlesinger WH, Wall DH, Wear D (2001) Ecological forecasts: an emerging imperative. Science 293, 657-660.
| Crossref | Google Scholar | PubMed |
Clarke H, Lucas C, Smith P (2013) Changes in Australian fire weather between 1973 and 2010. International Journal of Climatology 33, 931-944.
| Crossref | Google Scholar |
Conner RN, Rudolph DC, Kulhavy DL, Snow AE (1991) Causes of mortality of red-cockaded woodpecker cavity trees. The Journal of Wildlife Management 55, 531-537.
| Crossref | Google Scholar |
Dietze MC, Fox A, Beck-Johnson LM, Betancourt JL, Hooten MB, Jarnevich CS, Keitt TH, Kenney MA, Laney CM, Larsen LG (2018) Iterative near-term ecological forecasting: needs, opportunities, and challenges. Proceedings of the National Academy of Sciences 115, 1424-1432.
| Crossref | Google Scholar | PubMed |
Dowdy AJ (2018) Climatological variability of fire weather in Australia. Journal of Applied Meteorology and Climatology 57, 221-234.
| Crossref | Google Scholar |
Drake JE, Aspinwall MJ, Pfautsch S, Rymer PD, Reich PB, Smith RA, Crous KY, Tissue DT, Ghannoum O, Tjoelker MG (2015) The capacity to cope with climate warming declines from temperate to tropical latitudes in two widely distributed Eucalyptus species. Global Change Biology 21, 459-472.
| Crossref | Google Scholar | PubMed |
Fairman TA, Nitschke CR, Bennett LT (2015) Too much, too soon? A review of the effects of increasing wildfire frequency on tree mortality and regeneration in temperate eucalypt forests. International Journal of Wildland Fire 25, 831-848.
| Crossref | Google Scholar |
Fischer J, Zerger A, Gibbons P, Stott J, Law BS (2010) Tree decline and the future of Australian farmland biodiversity. Proceedings of the National Academy of Sciences 107, 19597-19602.
| Crossref | Google Scholar | PubMed |
Gibbons P, Lindenmayer D, Barry S, Tanton M (2000a) The effects of slash burning on the mortality and collapse of trees retained on logged sites in south-eastern Australia. Forest Ecology and Management 139, 51-61.
| Crossref | Google Scholar |
Gibbons P, Lindenmayer D, Barry S, Tanton M (2000b) Hollow formation in eucalypts from temperate forests in southeastern Australia. Pacific Conservation Biology 6, 218-228.
| Crossref | Google Scholar |
Gibbons P, Lindenmayer DB, Barry SC, Tanton MT (2002) Hollow selection by vertebrate fauna in forests of southeastern Australia and implications for forest management. Biological Conservation 103, 1-12.
| Crossref | Google Scholar |
Gibbons P, Lindenmayer DB, Fischer J, Manning AD, Weinberg A, Seddon J, Ryan P, Barrett G (2008) The future of scattered trees in agricultural landscapes. Conservation Biology 22, 1309-1319.
| Crossref | Google Scholar | PubMed |
Gibbons P, McElhinny C, Lindenmayer DB (2010) What strategies are effective for perpetuating structures provided by old trees in harvested forests? A case study on trees with hollows in south-eastern Australia. Forest Ecology and Management 260, 975-982.
| Crossref | Google Scholar |
Grömping U (2007) Relative importance for linear regression in R: the package relaimpo. Journal of Statistical Software 17, 1-27.
| Google Scholar |
Hartel T, Dorresteijn I, Klein C, Máthé O, Moga CI, Öllerer K, Roellig M, von Wehrden H, Fischer J (2013) Wood-pastures in a traditional rural region of Eastern Europe: characteristics, management and status. Biological Conservation 166, 267-275.
| Crossref | Google Scholar |
Hartig F (2022). DHARMa: Residual Diagnostics for Hierarchical (Multi‐Level / Mixed) Regression Models. R package version 0.4.6, https://CRAN.R‐project.org/package=DHARMa.
Haslem A, Avitabile SC, Taylor RS, Kelly LT, Watson SJ, Nimmo DG, Kenny SA, Callister KE, Spence-Bailey LM, Bennett AF, Clarke MF (2012) Time-since-fire and inter-fire interval influence hollow availability for fauna in a fire-prone system. Biological Conservation 152, 212-221.
| Crossref | Google Scholar |
Inions G, Tanton M, Davey S (1989) Effect of fire on the availability of hollows in trees used by the common Brushtail Possum, Trichosurus vulpecula Kerr, 1792, and the Ringtail Possum, Pseudocheirus peregrinus Boddaerts, 1785. Australian Wildlife Research 16, 449-458.
| Crossref | Google Scholar |
Jennings S, Reynolds JD, Mills SC (1998) Life history correlates of responses to fisheries exploitation. Proceedings of the Royal Society B: Biological Sciences 265, 333-339.
| Crossref | Google Scholar |
Jordan R, Hoffmann AA, Dillon SK, Prober SM (2017) Evidence of genomic adaptation to climate in Eucalyptus microcarpa: implications for adaptive potential to projected climate change. Molecular Ecology 26, 6002-6020.
| Crossref | Google Scholar | PubMed |
King KJ, Cary GJ, Bradstock RA, Marsden-Smedley JB (2013) Contrasting fire responses to climate and management: insights from two Australian ecosystems. Global Change Biology 19, 1223-1235.
| Crossref | Google Scholar | PubMed |
Koch A, Munks S, Driscoll D (2008) The use of hollow-bearing trees by vertebrate fauna in wet and dry Eucalyptus obliqua forest, Tasmania. Wildlife Research 35, 727-746.
| Crossref | Google Scholar |
Koch AJ, Chuter A, Barmuta LA, Turner P, Munks SA (2018) Long-term survival of trees retained for hollow-using fauna in partially harvested forest in Tasmania, Australia. Forest Ecology and Management 422, 263-272.
| Crossref | Google Scholar |
Kruskal W (1987) Relative importance by averaging over orderings. The American Statistician 41, 6-10.
| Crossref | Google Scholar |
Le Roux DS, Ikin K, Lindenmayer DB, Manning AD, Gibbons P (2014) The future of large old trees in urban landscapes. PLoS One 9, e99403.
| Crossref | Google Scholar | PubMed |
Lindenmayer DB, Wood JT (2010) Long-term patterns in the decay, collapse, and abundance of trees with hollows in the mountain ash (Eucalyptus regnans) forests of Victoria, southeastern Australia. Canadian Journal of Forest Research 40, 48-54.
| Crossref | Google Scholar |
Lindenmayer DB, Cunningham RB, Donnelly CF, Tanton MT, Nix HA (1993) The abundance and development of cavities in Eucalyptus trees: a case study in the montane forests of Victoria, southeastern Australia. Forest Ecology & Management 60, 77-104.
| Crossref | Google Scholar |
Lindenmayer DB, Blanchard W, Blair D, McBurney L (2018) The road to oblivion–Quantifying pathways in the decline of large old trees. Forest Ecology and Management 430, 259-264.
| Crossref | Google Scholar |
Lindenmayer D, McBurney L, Blanchard W (2023) Drivers of collapse of fire‐killed trees. Austral Ecology 48, 134-142.
| Crossref | Google Scholar |
Linder P, Östlund L (1998) Structural changes in three mid-boreal Swedish forest landscapes, 1885-1996. Biological Conservation 85, 9-19.
| Crossref | Google Scholar |
Lüdecke D, Ben-Shachar M, Patil I, Waggoner P, Makowski D (2021) performance: an R package for assessment, comparison and testing of statistical models. Journal of Open Source Software 6, 3139.
| Crossref | Google Scholar |
Manning AD, Gibbons P, Fischer J, Oliver DL, Lindenmayer DB (2013) Hollow futures? Tree decline, lag effects and hollow‐dependent species. Animal Conservation 16, 395-403.
| Crossref | Google Scholar |
McLean CM, Bradstock R, Price O, Kavanagh RP (2015) Tree hollows and forest stand structure in Australian warm temperate Eucalyptus forests are adversely affected by logging more than wildfire. Forest Ecology and Management 341, 37-44.
| Crossref | Google Scholar |
Moritz MA, Parisien M-A, Batllori E, Krawchuk MA, Van Dorn J, Ganz DJ, Hayhoe K (2012) Climate change and disruptions to global fire activity. Ecosphere 3, 1-22.
| Crossref | Google Scholar |
Murphy BP, RUSSELL‐SMITH J, Prior LD (2010) Frequent fires reduce tree growth in northern Australian savannas: implications for tree demography and carbon sequestration. Global Change Biology 16, 331-343.
| Crossref | Google Scholar |
Newton I (1994) The role of nest sites in limiting the numbers of hole-nesting birds - a review. Biological Conservation 70, 265-276.
| Crossref | Google Scholar |
Ngugi MR, Doley D, Cant M, Botkin DB (2015) Growth rates of Eucalyptus and other Australian native tree species derived from seven decades of growth monitoring. Journal of Forestry Research 26, 811-826.
| Crossref | Google Scholar |
Norse EA, Brooke S, Cheung WWL, Clark MR, Ekeland I, Froese R, Gjerde KM, Haedrich RL, Heppell SS, Morato T, Morgan LE, Pauly D, Sumaila R, Watson R (2012) Sustainability of deep-sea fisheries. Marine policy 36, 307-320.
| Crossref | Google Scholar |
Novak T (2005) Terrestrial fauna from cavities in Northern and Central Slovenia, and a review of systematically ecologically investigated cavities. Acta Carsologica 34, 170-210.
| Crossref | Google Scholar |
Parnaby H, Lunney D, Shannon I, Fleming M (2010) Collapse rates of hollow-bearing trees following low intensity prescription burns in the Pilliga forests, New South Wales. Pacific Conservation Biology 16, 209-220.
| Crossref | Google Scholar |
Price OF (2012) The drivers of effectiveness of prescribed fire treatment. Forest Science 58, 606-617.
| Crossref | Google Scholar |
Prior LD, Williamson GJ, Bowman DMJS (2016) Impact of high-severity fire in a Tasmanian dry eucalypt forest. Australian Journal of Botany 64, 193-205.
| Crossref | Google Scholar |
Ranius T, Niklasson M, Berg N (2009) Development of tree hollows in pedunculate oak (Quercus robur). Forest Ecology and Management 257, 303-310.
| Crossref | Google Scholar |
Salmona J, Dixon KM, Banks SC (2018) The effects of fire history on hollow-bearing tree abundance in montane and subalpine eucalypt forests in southeastern Australia. Forest Ecology and Management 428, 93-103.
| Crossref | Google Scholar |
Shepherd KR (1957) Some aspects of ringbarking in Alpine Ash stands. Australian Forestry 21, 70-75.
| Crossref | Google Scholar |
Stojanovic D, Webb nee Voogdt J, Webb M, Cook H, Heinsohn R (2016) Loss of habitat for a secondary cavity nesting bird after wildfire. Forest Ecology and Management 360, 235-241.
| Crossref | Google Scholar |
Stojanovic D, Rayner L, Cobden M, Davey C, Harris S, Heinsohn R, Owens G, Manning AD (2021) Suitable nesting sites for specialized cavity dependent wildlife are rare in woodlands. Forest Ecology and Management 483, 118718.
| Crossref | Google Scholar |
Thomaes A, Crévecoeur L, Wijnants M (2015) Tree cavity beetles in Haspengouw and Pays De Herve: Crepidophorus mutilatus (Elateridae) new for the Belgian fauna and rediscovery of Gnorimus variabilis (Cetoniidae). Bulletin de la Societe Royale Belge d’Entomologie 151, 40-51.
| Google Scholar |
Turkalo AK, Wrege PH, Wittemyer G (2017) Slow intrinsic growth rate in forest elephants indicates recovery from poaching will require decades. Journal of Applied Ecology 54, 153-159.
| Crossref | Google Scholar |
von Haartman L (1957) Adaptation in hole-nesting birds. Evolution 11, 339-347.
| Crossref | Google Scholar |
Westerling AL, Hidalgo HG, Cayan DR, Swetnam TW (2006) Warming and earlier spring increase western U.S. forest wildfire activity. Science 313, 940-943.
| Crossref | Google Scholar | PubMed |
Woolley L-A, Murphy BP, Radford IJ, Westaway J, Woinarski JCZ (2018) Cyclones, fire, and termites: the drivers of tree hollow abundance in northern Australia’s mesic tropical savanna. Forest Ecology and Management 419–420, 146-159.
| Crossref | Google Scholar |
Wormington K, Lamb D (1999) Tree hollow development in wet and dry sclerophyll eucalypt forest in south-east Queensland, Australia. Australian Forestry 62, 336-345.
| Crossref | Google Scholar |
Wormington KR, Lamb D, McCallum HI, Moloney DJ (2003) The characteristics of six species of living hollow-bearing tssrees and their importance for arboreal marsupials in the dry sclerophyll forests of southeast Queensland, Australia. Forest Ecology and Management 182, 75-92.
| Crossref | Google Scholar |