Evaluating the utility of environmental DNA for detecting a large Critically Endangered lizard in tropical northern Australia
Emily P. Hoffmann
A
B
C
D
E
F
G
H
Abstract
It can be challenging to reliably detect rare or cryptic species. Environmental DNA (eDNA) is an emerging tool for detecting species and is increasingly being used to detect reptiles in terrestrial environments that are costly or difficult to survey or monitor using traditional methods. Here, we trialled eDNA metabarcoding to detect one of Australia’s most threatened and least known reptile species, the Arnhem rock skink (Bellatorias obiri). At the only site where they were known to persist, we sampled soil from 12 rock crevices, including four with high levels of activity of the target species, as well as water from three adjacent pools. We were unable to identify DNA of B. obiri in any of the soil or water samples, suggesting multiple false-negative errors, despite our successful amplification of B. obiri DNA from an incidental scat sample. We were able to identify 15 non-target vertebrate taxa from our samples. Given that samples were taken from a site where B. obiri was known to be present, the eDNA metabarcoding technique trialled here does not appear to be an effective method for detecting this species. Whilst eDNA metabarcoding is an emerging and powerful tool in ecology and conservation, our pilot study highlights that challenges remain in its application for detecting rare or cryptic terrestrial reptiles.
Keywords: Bellatorias obiri, eDNA, metabarcoding, reptile, soil, species detection, terrestrial, threatened species.
Introduction
Rare or elusive species can be challenging to detect using traditional survey methods, particularly when they are found in remote areas, where surveys are logistically complex, time-consuming, and expensive. The use of environmental DNA (eDNA) presents a promising tool for species detection, involving the collection and analysis of DNA that is shed into the environment through the excretion of bodily fluids, skin, and hair. eDNA can be extracted from water, soil, or even air samples, allowing for non-invasive detection of species without the need for visual sightings or trapping (Thomsen et al. 2012; Taberlet et al. 2018).
The application of eDNA methods in wildlife conservation and management is growing rapidly (Beng and Corlett 2020; Nordstrom et al. 2022). Two main approaches include: (1) metabarcoding, which uses general primers to amplify DNA from multiple species simultaneously; and (2) species-specific primers, which target DNA specific to a single species. Species-specific approaches can be more sensitive and are useful for targeted species surveys (Beng and Corlett 2020). However, they require reference sequences to create a reliable assay, making them unsuitable for species with little or no genomic data. While generally less sensitive, metabarcoding can detect rare or elusive species from a single sample (Holdaway et al. 2017) and offers a diverse range of applications for biodiversity monitoring, from specific species groups to wider biodiversity studies (Ruppert et al. 2019).
The recent advancements in eDNA methods may offer a more cost-effective alternative to traditional survey methods (Fediajevaite et al. 2021). This potential allows for rapid and economical surveying of expansive areas, reducing the need for extensive and labour-intensive fieldwork, particularly when dealing with rare or elusive species that require specialised expertise or significant survey effort (Biggs et al. 2015; Davy et al. 2015; Akre et al. 2019). However, the application of eDNA methodologies requires initial investment in quality control and assurance. For example, it is crucial to address the challenge of discriminating between false positives and false negatives for ensuring the accuracy and reliability of eDNA-based assessments through rigorous sampling protocols and validation techniques (Ficetola et al. 2015).
Here, we piloted the use of eDNA metabarcoding to detect the Arnhem rock skink (Bellatorias obiri), an extremely cryptic lizard found in rugged and remote rocky gorges and boulder fields. The species is listed as Critically Endangered (Gillespie et al. 2018), and is considered one of Australia’s most imminently threatened vertebrates (Garnett et al. 2022). The Arnhem rock skink is a large (snout-vent length ~200 mm), robust skink with a restricted distribution centred on Kakadu National Park, in Australia’s Northern Territory (Jolly et al. 2023). Detecting the Arnhem rock skink via active searches or live trapping poses significant challenges due to its elusive behaviour, rugged habitat, and remote distribution. Since scientists first became aware of the species in 1974, there have been fewer than 40 confirmed records of the species, primarily incidental, underscoring the difficulty and time-consuming nature of this endeavour (Cogger 1974; Sadlier 1990; Armstrong and Dudley 2004). In the 50 years since, there has been up to a decade between detections of live specimens, despite considerable survey effort. Given the difficulties of detecting this elusive species using traditional search methods, combined with the challenges and costs of accessing and surveying in a remote location, eDNA was a desirable method to trial for the detection of B. obiri.
By using eDNA sampling, we aimed to assess the effectiveness of detecting this Critically Endangered lizard species from water and soil samples collected from the only confirmed location where the species was known to persist. Our study trialled a metabarcoding approach as no reference sequences existed for the target species, preventing the design of a species-specific assay. If effective, this method could be employed more widely to survey areas with historical records of the species and to search new potential habitats. Given that eDNA is not yet widely used in vertebrate surveys in northern Australia, we suggest that our results will also provide valuable information for agencies seeking to efficiently and cost-effectively survey for B. obiri and other rare or cryptic reptile species.
Materials and methods
Sample collection
In October 2022, we collected samples from the only site where Bellatorias obiri was known to persist; a spring-fed, rocky gorge in Kakadu National Park, Northern Territory (approximately 13.6°S, 132.3°E), where it inhabits deep rock crevices (Fig. 1). We collected both soil and water samples, as these materials have previously been used to detect skink eDNA (Kyle et al. 2022; West et al. 2023).
Location of the study site (black circle) in Kakadu National Park, Northern Territory, Australia and the study species, the Arnhem rock skink (Bellatorias obiri) (inset). Photo credit: C. Jolly.
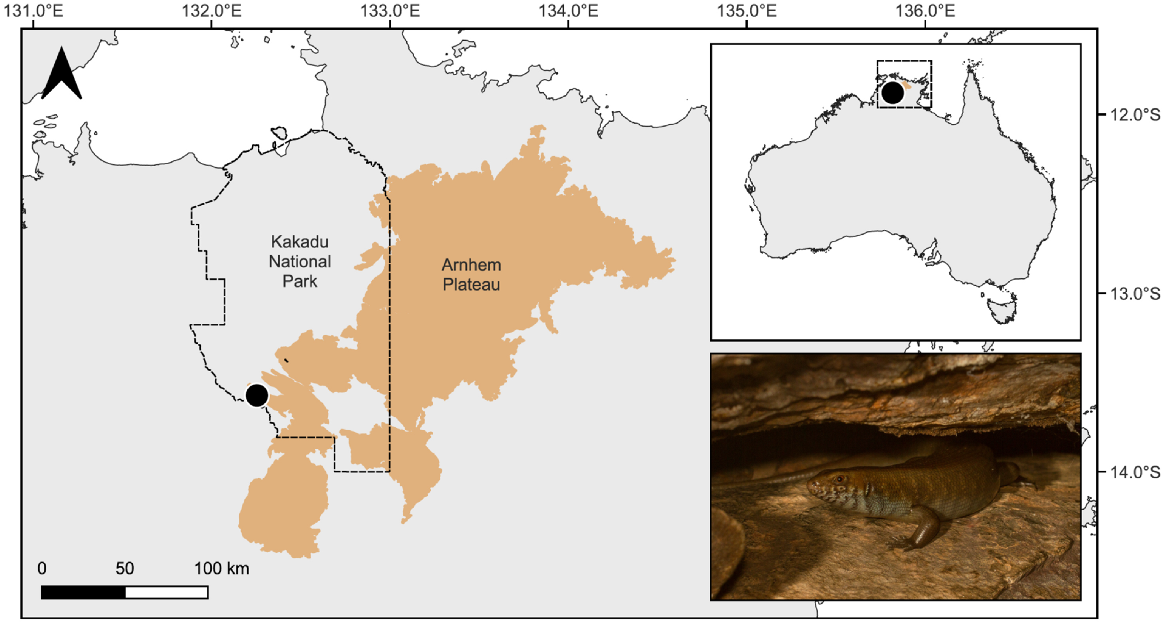
We collected two soil samples from each of 12 rock crevices, where pre-existing camera trap data allowed for quantification of B. obiri activity levels (NT Government and Kakadu National Park, unpublished data). Bellatorias obiri activity was defined as the average number of motion-trigger camera images in which the species was detected in a crevice during the preceding build-up and wet season (from August 2021 to February 2022). To investigate how effective eDNA methods may be for detecting the species at different levels of activity, we stratified the sampled crevices into three categories based on the number of detections from camera trapping: (1) high activity; (2) low activity; and (3) no activity (see Supplementary material S1, Table S1). An average was used as each crevice had one or two cameras that were operational for the entire sampling duration. We arbitrarily defined crevices with high activity as those with >80 detections, low activity as crevices with 15–29 detections, and no activity as crevices with zero B. obiri detections. Soil samples were collected from rock crevices while wearing clean gloves and using sterile containers for each sample. A new clean plastic spatula was used to scrape the upper soil layer into a 50-mL centrifuge tube. Tubes were not filled greater than halfway (25 mL). The lid of the tube was screwed on and then wrapped with parafilm. Tubes containing soil samples were immediately placed into a cooler with ice bricks and then frozen at −20°C within eight hours.
We also collected two water samples from each of three waterbodies that were adjacent to the crevices where B. obiri was known to occur (Main Pool, Upper Pool, and Lower Pool). All three waterbodies had little flow at the time of collection, as sampling occurred in October, which is late in the dry season during the build-up. An incidental scat sample was also collected from a crevice where B. obiri had been detected recently (in the preceding 9 months). A reference tissue sample from a B. obiri specimen (stored in ethanol at room temperature) was obtained from the Museum and Art Gallery of the Northern Territory (Museum Sample ID: TS0052) and was included to validate that the assay used would successfully amplify B. obiri DNA from a higher quality sample (refer to Laboratory analysis below and Supplementary material S2 for molecular methods).
Water samples were also collected while wearing clean gloves and using sterile plastic containers. Containers were initially rinsed internally by half-filling with site water, and were then capped and shaken, before the water was discarded away from the sample collection site. Water samples (1 L) were collected by submerging the container below the water surface, and then containers were sealed and placed immediately into a cooler with ice bricks. Water samples were filtered within 4 h of collection in the field using 0.45 μm mixed cellulose ester with a Sentino peristaltic pump (PALL Corporation, Port Washington, USA) to capture eDNA present in the water. Each sample was filtered for up to 30 min and the volume of water filtered for each sample was recorded. The filter was then removed and folded in half, with the filtrate on the inward side, and placed in a labelled sample bag using clean forceps. Filter papers were stored at −20°C. Common equipment (e.g. forceps) was cleaned with a 10% bleach solution for 10 min and then rinsed thoroughly before re-use. A blank control sample from the cleaning solution was also processed and sent for analysis to detect any cross-contamination.
All samples were stored at −20°C before and after transportation to the eDNA Frontiers Laboratory at Curtin University, Perth, Western Australia, for preparation, DNA extraction, sequencing, and bioinformatics analysis.
Laboratory analysis
For detailed methods regarding sample preparation, DNA extraction, and library preparation, see Supplementary material S2. In summary, whole-genomic DNA was extracted from each sample, and the target DNA fragment was amplified using established metabarcoding techniques using a 16S rDNA assay targeting reptiles (West et al. 2023). Species-specific qPCR techniques could not be used for this study, as no reference sequences exist for the target species to allow an assay to be designed. Samples were tagged with individual identifiers, pooled into a library, and sequenced on an MiSeq platform (Illumina, Inc., San Diego). All PCR preparations were performed in an ultra-clean laboratory to minimise the risk of contamination.
Detailed methods regarding sequencing and bioinformatics pipeline are provided in Supplementary material S2. In brief, raw sequence data were processed using an established eDNA bioinformatics pipeline, involving read demultiplexing, trimming, filtering, and transformation into zero-radius operational taxonomic units. These taxonomic units were then assigned to a genus and/or species based on the NCBI GenBank database (https://www.ncbi.nlm.nih.gov/genbank/), further filtered based on several factors, and the apparent presence or absence of each taxonomic unit in each sample was tabulated.
Results
We retained 2,689,909 reads after the initial read filtering steps, producing 1090 taxonomic units. Of these, 56 matched to a GenBank entry at ≥95% similarity after clustering. Once the filters for non-eukaryotes, environmental contaminants, and non-vertebrates had been applied, and duplicated taxa were merged, we retained 16 genus or species level taxonomic units (including the museum-collected Arnhem rock skink sample). These included taxa from five animal classes, including 12 vertebrate genera in the water samples (six samples from three pools), and five vertebrate genera in the soil samples (24 samples from 12 crevices) (Table 1). Of the 15 taxa identified from the water or soil samples, we were able to identify 10 taxa to species, including a range of non-target vertebrate groups (mammal, bird, amphibian, and fish species) (Table 1).
Class | Family | Common name | Scientific name | Soil | Water | Control | Scat | Tissue | ||||||||||||||
---|---|---|---|---|---|---|---|---|---|---|---|---|---|---|---|---|---|---|---|---|---|---|
High activity crevice | Low activity crevice | No activity crevice | Main pool (2) | Lower pool (2) | Upper pool (2) | Blank (1) | BO5 (1) | TS0052 (1) | ||||||||||||||
BO1 (2) | BO3 (2) | BO23 (2) | BO26 (2) | BO30 (2) | BO22 (2) | BO18 (2) | BO16 (2) | BO14 (2) | BO15 (2) | BO8 (2) | BO9 (2) | |||||||||||
Squamata | Scincidae | Arnhem rock skink | Bellatorias obiri | * | * | |||||||||||||||||
Homalopsidae | Macleay’s water snake | Pseudoferania polylepis | * | |||||||||||||||||||
Actinopterygii | Melanotaeniidae | Rainbowfish | Melanotaenia sp. | * | * | |||||||||||||||||
Ambassidae | Sailfin glassfish | Ambassis agrammus | * | |||||||||||||||||||
Eleotridae | Northern purple spotted gudgeon | Mogurnda mogurndaA | * | * | * | * | ||||||||||||||||
Terapontidae | Grunter | Hephaestus sp. | * | |||||||||||||||||||
Spangled grunter | Leiopotherapon unicolor | * | * | |||||||||||||||||||
Amphibia | Bufonidae | Cane toad | Rhinella marina | * | * | |||||||||||||||||
Pelodryadidae | Spalding’s rocket frog | Litoria spaldingiA | * | * | * | |||||||||||||||||
Aves | Alcedinidae | Blue-winged kookaburra | Dacelo leachiiA | * | ||||||||||||||||||
Dicruridae | Drongo | Dicrurus sp. | * | |||||||||||||||||||
Oriolidae | Oriole | Oriolus sp. | * | |||||||||||||||||||
Mammalia | Pteropodidae | Fruit bat | Pteropus sp. | * | ||||||||||||||||||
Dasyuridae | Sandstone false antechinus | Pseudantechinus bilarni | * | |||||||||||||||||||
Macropodidae | Black wallaroo | Osphranter bernardus | * | * | ||||||||||||||||||
Muridae | Common rock-rat | Zyzomys argurus | * | * | * |
Positive identification of the species in each sample is indicated by an asterisk. Bold and shaded cells indicate the target species. Taxonomic classifications have been standardised according to GBIF (accessed December 2022). Number in parentheses indicates the number of samples collected. Bellatorias obiri tissue was provided by the Museum and Art Gallery of the Northern Territory.
Despite successfully amplifying B. obiri DNA from the tissue and incidental scat samples, we were unable to detect the DNA of our target species, B. obiri, in any of the in situ water or soil samples. Laboratory extraction controls were all negative and no contamination was found in the field blank sample. The only reptile detected in the water or soil samples was Macleay’s water snake (Pseudoferania polylepis), which was identified in a single water sample. The introduced cane toad (Rhinella marina), which is widespread and common in the region, was detected in two of the three sampled waterbodies.
Discussion
We trialled eDNA metabarcoding for detecting the Critically Endangered B. obiri in soil and water samples from a site known to be occupied by the species. Our aim was to trial the approach before applying it more widely as a detection method across the species’ known historical range in Kakadu National Park. While the species’ DNA was successfully identified from a preserved B. obiri tissue sample and a suspected scat sample, it could not be detected in any of the soil or water samples collected in the field. It is acknowledged that a species-specific qPCR assay is a more sensitive technique than metabarcoding for detecting a single target species and therefore may have displayed improved performance in this study; however, this technique requires a comprehensive reference sequence database (ideally spanning the genetic diversity of the target species) in order to design a robust assay, and reference sequences are not currently available for B. obiri. Additionally, the eDNA method trialled here did not detect nine additional reptile species that were known to be present in the crevices in the preceding 2 months (i.e. detected by camera trapping; see Supplementary material S1, Table S2). The lack of the target species’ DNA in the soil and water samples, as well as the lack of DNA from any other terrestrial reptile species, suggests that the eDNA techniques trialled here may not be a reliable tool for detecting terrestrial reptiles in the study region using these types of substrates and the 16S rRNA fragment that we used.
Although possible, it is unlikely that B. obiri was inactive at the crevices in our study area between the time of camera trapping and substrate sampling (~7 weeks). Subsequent camera surveys documented use at all high activity crevices in the weeks immediately following the eDNA sampling, including activity at one crevice on the same day as the camera trap deployment (3 days after the eDNA sampling was conducted). This supports that our results are primarily due to false negative errors. One likely explanation for this is that B. obiri, like many other terrestrial reptiles, sheds little DNA into the environment (Nordstrom et al. 2022). Previous studies have suggested that eDNA detection success rates may be lower for animals with hard keratinised outer layers, such as reptiles, due to differences in DNA shedding rates (Adams et al. 2019; Allan et al. 2021). However, Matthias et al. (2021) successfully detected DNA of a small snake in soil to which an individual was exposed for only 10 minutes, and terrestrial skinks have been detected in surface water samples from north-eastern Australia (West et al. 2023), suggesting eDNA methods can be effective in some circumstances.
Other explanations for our results may be that our choice of target DNA fragment was inappropriate, or that high levels of microbial activity and UV solar radiation in tropical environments results in rapid degradation of eDNA, reducing the chances of successful detection (Zulkefli et al. 2019). However, as our chosen DNA fragment has been widely used for eDNA metabarcoding in a variety of taxa and we were able to detect numerous non-target species, we suggest that these explanations are less relevant.
We were unable to conduct an ex situ experiment using a captive population as no individuals exist in captivity and the species was only known from a single site. However, further research using captive individuals of one or more surrogate skink species and different substrates and target DNA fragments may help to improve the use of eDNA methods for detecting reptiles in northern Australia. For example, swabbing for surface DNA on artificial cover objects has successfully detected terrestrial reptiles (Matthias et al. 2021; Kyle et al. 2022). Kyle et al. (2022) found surface swabbing using a paint roller performed better than collecting soil for detection of a terrestrial skink. This ‘roller’ method could be used to sample larger surface areas of rock crevices or from artificial surfaces (e.g. metal) that may have higher detection probability (Kyle et al. 2022). However, the cost of alternative eDNA trials should be considered versus other potentially viable detection methods, such as camera trapping.
Our findings highlight the challenges of applying eDNA metabarcoding techniques for detecting rare or cryptic terrestrial reptiles – in this case, the Critically Endangered lizard, B. obiri – emphasising the need for further refinement and exploration of this or alternative methods to improve species detection in terrestrial reptiles and tropical environments.
Data availability
Due to the sensitive nature of the species location the datasets from the current study are not publicly available but are available from the authors on reasonable request.
Declaration of funding
This project was supported through funding from the Australian Government. CJJ is supported by a Macquarie University Research Fellowship and the Australian Research Council. BvT is supported by the Western Australian Government through the Premier’s Science Fellowship Program.
Author contributions
Emily P. Hoffmann: Conceptualisation (supporting); Methodology (supporting); Data curation (lead); Investigation (lead); Project administration (lead); Writing original draft (equal lead); Writing-review and editing (equal lead). Chris J. Jolly: Conceptualisation (supporting); Methodology (supporting); Writing original draft (supporting); Writing-review and editing (equal lead). Kathryn L. Dawkins: Data curation (supporting); Formal analysis (lead); Writing original draft (supporting); Writing-review and editing (supporting). Kelly M. Dixon: Funding acquisition; Project administration (supporting); and Supervision (lead); Writing-review and editing (supporting). Luke D. Einoder: Resources; Supervision (supporting); Writing-review and editing (supporting). Graeme Gillespie: Investigation (supporting); Writing-review and editing (supporting). Brett P. Murphy: Conceptualisation (supporting); Funding acquisition; Writing-review and editing (supporting). Brenton von Takach: Conceptualisation (lead); Methodology (lead); Writing original draft (equal lead), Writing-review and editing (equal lead).
Acknowledgements
We thank the Traditional Owners of Kakadu National Park and their families for their consent and assistance with site access for the collection of soil and water samples. We also thank Kakadu National Park Rangers from the Mary River District and Graduate Ranger, Georgia Kielbaska. We acknowledge the Northern Territory Government staff for sharing data on activity use of crevices at the sample site, in particular Peter McDonald and Tony Griffiths. All research was conducted in accordance with Charles Darwin University Animal Ethics Approval number A22024 and Permit to carry out Scientific Research in a Commonwealth Reserve number RK962.
References
Adams CIM, Hoekstra LA, Muell MR, Janzen FJ (2019) A brief review of non-avian reptile environmental DNA (eDNA), with a case study of painted turtle (Chrysemys picta) eDNA under field conditions. Diversity 11(4), 50.
| Crossref | Google Scholar |
Akre TS, Parker LD, Ruther E, Maldonado JE, Lemmon L, McInerney NR (2019) Concurrent visual encounter sampling validates eDNA selectivity and sensitivity for the endangered wood turtle (Glyptemys insculpta). PLoS ONE 14(4), e0215586.
| Crossref | Google Scholar | PubMed |
Allan EA, Zhang WG, Lavery AC, Govindarajan AF (2021) Environmental DNA shedding and decay rates from diverse animal forms and thermal regimes. Environmental DNA 3(2), 492-514.
| Crossref | Google Scholar |
Beng KC, Corlett RT (2020) Applications of environmental DNA (eDNA) in ecology and conservation: opportunities, challenges and prospects. Biodiversity and Conservation 29(7), 2089-2121.
| Crossref | Google Scholar |
Biggs J, Ewald N, Valentini A, Gaboriaud C, Dejean T, Griffiths RA, Foster J, Wilkinson JW, Arnell A, Brotherton P, Williams P, Dunn F (2015) Using eDNA to develop a national citizen science-based monitoring programme for the great crested newt (Triturus cristatus). Biological Conservation 183, 19-28.
| Crossref | Google Scholar |
Davy CM, Kidd AG, Wilson CC (2015) Development and validation of environmental DNA (eDNA) markers for detection of freshwater turtles. PLoS ONE 10(7), e0130965.
| Crossref | Google Scholar |
Fediajevaite J, Priestley V, Arnold R, Savolainen V (2021) Meta-analysis shows that environmental DNA outperforms traditional surveys, but warrants better reporting standards. Ecology and Evolution 11(9), 4803-4815.
| Crossref | Google Scholar | PubMed |
Ficetola GF, Pansu J, Bonin A, Coissac E, Giguet-Covex C, De Barba M, Gielly L, Lopes CM, Boyer F, Pompanon F, Rayé G, Taberlet P (2015) Replication levels, false presences and the estimation of the presence/absence from eDNA metabarcoding data. Molecular Ecology Resources 15(3), 543-556.
| Crossref | Google Scholar | PubMed |
Garnett ST, Hayward-Brown BK, Kopf RK, Woinarski JCZ, Cameron KA, Chapple DG, Copley P, Fisher A, Gillespie G, Latch P, Legge S, Lintermans M, Moorrees A, Page M, Renwick J, Birrell J, Kelly D, Geyle HM (2022) Australia’s most imperilled vertebrates. Biological Conservation 270, 109561.
| Crossref | Google Scholar |
Gillespie G, Woinarski J, McDonald P, Cogger H, Fenner A (2018) Bellatorias obiri. IUCN Red List of Threatened Species 2018, e.T47155317A47155335.
| Crossref | Google Scholar |
Holdaway RJ, Wood JR, Dickie IA, Orwin KH, Bellingham PJ, Richardson SJ, Lyver POB, Timoti P, Buckley TR (2017) Using DNA metabarcoding to assess New Zealand’s terrestrial biodiversity. New Zealand Journal of Ecology 41(2), 251-262.
| Crossref | Google Scholar |
Kyle KE, Allen MC, Dragon J, Bunnell JF, Reinert HK, Zappalorti R, Jaffe BD, Angle JC, Lockwood JL (2022) Combining surface and soil environmental DNA with artificial cover objects to improve terrestrial reptile survey detection. Conservation Biology 36(6), e13939.
| Crossref | Google Scholar | PubMed |
Matthias L, Allison MJ, Maslovat CY, Hobbs J, Helbing CC (2021) Improving ecological surveys for the detection of cryptic, fossorial snakes using eDNA on and under artificial cover objects. Ecological Indicators 131, 108187.
| Crossref | Google Scholar |
Nordstrom B, Mitchell N, Byrne M, Jarman S (2022) A review of applications of environmental DNA for reptile conservation and management. Ecology and Evolution 12(6), e8995.
| Crossref | Google Scholar |
Ruppert KM, Kline RJ, Rahman MS (2019) Past, present, and future perspectives of environmental DNA (eDNA) metabarcoding: a systematic review in methods, monitoring, and applications of global eDNA. Global Ecology and Conservation 17, e00547.
| Crossref | Google Scholar |
Sadlier RA (1990) A new species of scincid lizard from western Arnhemland, Northern Territory. The Beagle 7(2), 29-33.
| Crossref | Google Scholar |
Taberlet P, Bonin A, Zinger L, Coissac E (2018) ‘Environmental DNA: for biodiversity research and monitoring, Vol. 1.’ (Oxford University Press) 10.1093/oso/9780198767220.001.0001
Thomsen PF, Kielgast J, Iversen LL, Wiuf C, Rasmussen M, Gilbert MTP, Orlando L, Willerslev E (2012) Monitoring endangered freshwater biodiversity using environmental DNA. Molecular Ecology 21(11), 2565-2573.
| Crossref | Google Scholar | PubMed |
West KM, Heydenrych M, Lines R, Tucker T, Fossette S, Whiting S, Bunce M (2023) Development of a 16S metabarcoding assay for the environmental DNA (eDNA) detection of aquatic reptiles across northern Australia. Marine and Freshwater Research 74, 432-440.
| Crossref | Google Scholar |
Zulkefli NS, Kim K-H, Hwang S-J (2019) Effects of microbial activity and environmental parameters on the degradation of extracellular environmental DNA from a eutrophic lake. International Journal of Environmental Research and Public Health 16(8), 3339.
| Crossref | Google Scholar |