Effect of postweaning growth and bulls selected for extremes in retail beef yield and intramuscular fat on progeny liveweight and carcass traits
J. F. Graham A B D , J. Byron A B , A. J. Clark A B , G. Kearney A B and B. Orchard A CA Cooperative Research Centre for Beef Genetic Technologies, University of New England, Armidale, NSW 2351, Australia.
B Department of Primary Industries, Primary Industries Research Victoria – Hamilton, Mt Napier Road, Hamilton, Vic. 3300, Australia.
C NSW Department of Primary Industries, Agricultural Institute, Private Bag Wagga Wagga, NSW 2650, Australia.
D Corresponding author. Email: john.graham@dpi.vic.gov.au
Animal Production Science 49(6) 493-503 https://doi.org/10.1071/EA08181
Submitted: 6 June 2008 Accepted: 14 November 2008 Published: 13 May 2009
Abstract
The present study is a component of a multi-site experiment, using Bos taurus cattle generated at four locations across southern Australia, designed to examine postweaning growth pathways for progeny whose sires were extreme in retail beef yield and intramuscular fat. Treatment and interaction effects on beef production and meat quality were examined within and across sites. The present paper describes the effect of postweaning growth and sire carcass type on liveweight and carcass traits at the Hamilton site. Angus sires selected on estimated breeding values for extremes in retail beef yield (RBY%), intramuscular fat (IMF%) (estimated breeding values for IMF% are derived by using live-animal ultrasound scanning) or both and sire breed types considered to be more extreme in those traits (Limousin, and Belgian Blue for yield, and Wagyu for intramuscular fat) were joined to crossbred and straight-bred cows. After weaning, the resultant 645 steer and heifer progeny were grown on a fast and slow growth path to ~550 kg and slaughtered, averaging 0.68 kg/day and 22.2 months, and 0.49 kg/day and 27.8 months for growth rate and age at slaughter, respectively. Growth path, sire carcass type and sex affected carcass traits; however, there were no sire carcass type by growth treatment interactions. The fast growth-path cattle were fatter, had more intramuscular fat (measured chemically), a higher Meat Standards of Australia (MSA) USA and AUS marble score, and a higher predicted MSA eating-quality score. Progeny of Wagyu sires were lighter at weaning and slaughter and had a lower hot standard carcass weight than the other sire carcass types. The Belgian Blue and Limousin progeny had a higher dressing percentage, a higher RBY% and a lower P8 and rib-fat depth and lower IMF% than the other sire breed types. Progeny of the high RBY% Angus had a lower rib-fat depth, a lower IMF% and higher RBY% than those selected for high IMF%. There was no difference in IMF% between the Wagyu or the high IMF% Angus. Progeny from the Belgian Blue, Limousin and Wagyu had a larger eye muscle area than the other sire breeds. The results indicate that simultaneous selection for supposedly antagonistic traits of IMF% and RBY% would result in carcass having high values of both measurements. Females were lighter than steers at slaughter, had a lower hot standard carcass weight, were fatter at the P8 and rib, and had a higher marble score and IMF%, a lower yield and a lower MSA-predicted eating-quality score than did steers. There was no interaction between postweaning growth and sire carcass type. These results indicate that with the use of appropriate sire carcass types and BREEDPLAN, and post-weaning nutrition, beef producers can confidently change carcass parameters to suit market specifications.
Additional keywords: sire breed type.
Introduction
To remain competitive both in the local and international arena, beef producers must continue to produce high-quality beef. Many reviews have shown that traits associated with desirable carcass composition (meat yield and marbling) can be controlled through genetics (Koots et al. 1994; Wood et al. 1999). Marshall (1994; p. 2753) stated: ‘Genetic selection on individual carcass characteristics should generally be effective. However, effectiveness of simultaneous selection of some multiple-trait combinations could be slowed by antagonistic genetic correlations.’ BREEDPLAN (Graser et al. 2005), with accurate estimated breeding values for numerous carcass traits, now enables producers to have the opportunity to produce animals with specific desirable carcass traits.
Robinson et al. (2001) and Upton et al. (2001) reported on the effects of post-weaning growth until finishing on subsequent growth and body composition of Angus, Hereford, Murray Grey and Shorthorn steers. Their studies together with those of Dundon et al. (2000) indicated that steers with a higher growth before finishing tended to have more intramuscular fat. The project described in the present paper is an element of the Regional Combinations project (McKiernan et al. 2005) where studies at sites across a range of southern Australian environments aimed to quantify the effects of varying both nutritional treatments and the sire carcass type on production and carcass quality.
Recent studies within the first Beef CRC suggested that growth depression before finishing reduced IMF% (Robinson et al. 2001). However, Pethick et al. (2004) cited Trenkle et al. (1978) and Zembayashi (1994) as examples where this did not occur and, in fact, slower growth resulted in more intramuscular fat. Pethick et al. (2004) concluded that there was limited published data available on the effect of prefinishing growth, and that the pattern of response may differ among breed types.
Results reported by Gregory et al. (1994a) showed that unfavourable correlations among traits affect carcass composition and palatability, indicating that genotypes with high RBY% potential may have lower eating quality. It is important to examine this (potential) antagonism in the context of domestic trade requirements for Australian beef, as both yield and eating quality can have large effects on carcass value.
The present study was designed to determine the most appropriate growth pathways for the progeny of sires that differ in genetic potential for carcass traits, to increase product value and herd productivity across southern Australia (McKiernan et al. 2005).
Materials and methods
The full experimental design of the project is described by McKiernan et al. (2005). In 2000, 2001 and 2002 at Hamilton, Victoria (37.45°S, 142.02°E, annual average rainfall 700 mm), mature autumn- and spring-calving cows were joined to sires of seven different breed types, representing a range in carcass traits of retail beef yield and intramuscular fat. The number of bulls used was as follows: nine high RBY% Angus (Angus RBY), nine high IMF% Angus (Angus IMF), five Angus with both traits (Angus RBY and IMF), five Limousin, four Belgian Blue (both high-yielding breeds), and five Wagyu and one Red Wagyu (high-marbling breeds) bulls. The Angus RBY (EBV of +1.7 for RBY and –0.4 for IMF; accuracies of 79.5 and 76.8%, respectively) and Angus IMF sires (EBV of –1.3 for RBY and +1.1 for IMF; accuracies of 80.4 and 79.7%, respectively) were in the top one percentile of the Angus Group BREEDPLAN-estimated breeding values (EBV) for those traits, whereas the Angus RBY and IMF sires (EBV of +1.1 for RBY and +0.9 for IMF; accuracies of 65.2 and 66.6%, respectively) were in the top 10-percentile range for each trait at the time of sire selection. Limousin, Belgian Blue and Wagyu bulls were chosen to be representative of their breed. Both the Angus and Limousin bulls had high accuracy EBV (65–75%), there being no BREEDPLAN values for Belgian Blue or Wagyu sires.
Cows used in the project were originally generated for a multi-breed project (Graham et al. 2000), by mating Angus and Hereford cows to Angus, Hereford, Limousin or Simmental sires, resulting in a wide range of dam genotypes. Spring and autumn cows (average calving dates of 8 September and 15 March, respectively) were joined by using a synchronised CIDR (DEC International, Hamilton, NZ), an artificial insemination (AI) mating program, as follows: CIDR insertion on Day 0, injection of 2 mg oestradiol benzoate (ODB) and removal of the CIDR and injection of 2 mL prostaglandin (PG) on Day 8, injection of 1 mg ODB on Day 9, and insemination of every cow on Day 10. A resynchrony program was used with reinsertion of CIDR and injection of 1 mg of ODB on Day 23, removal of CIDR on Day 31, injection of ODB on Day 32, and AI of cows to heat detection with KAMAR Heatmount Detectors on Days 33 and 34.
At weaning in January (autumn calving), and May (spring calving), progeny were randomly allocated within each sire-breed type to fast- or slow-growth treatments until slaughter at a target group liveweight of 550 kg to suit the heavy domestic or European Union trade. Mean age at slaughter was 22.2 and 27.8 months for the fast- and slow-growth groups, respectively (s.e.d. ± 0.09 months). Growth path was manipulated through stocking rate and the use of strategic supplementary feeding (silage based) where necessary to endeavour to have each seasonal growth-treatment group slaughtered at the average liveweight of 550 kg. Progeny (steers and females) were slaughtered at the same commercial abattoir (Cargill’s, Wagga Wagga NSW) with the spring-fast and autumn-slow, and spring-slow and autumn-fast groups being slaughtered together (Fig. 1). In most instances these cattle were slaughtered at the same time as the cattle from the NSW component of the project (McKiernan et al. 2005).
![]() |
Liveweight preslaughter was measured before transport to the meatworks. Carcasses were dressed according to AUS-MEAT standard specifications (AUS-MEAT 1998). Hot carcass weight (HSCW) and P8-fat depth [located at the intersection of a line parallel to the spine from the tuber ischium (pin bone) and a line perpendicular to it from the spinous process of the third sacral vertebrae (Moon 1980)] were measured before chilling. Qualified Meat Standard Australia (MSA) graders assessed USDA ossification score (Romans et al. 1994), rib-fat depth over the longissimus dorsi muscle, eye muscle area (EMA), MSA marble score (AUS-MEAT marbling score of 0–6, with decimal divisions within each score) and USDA marbling score (Romans et al. 1994).
At 18–24 h after slaughter, chiller and VIA-scan (Ferguson et al. 1995; Tong et al. 1999) details were recorded. After quartering, a transverse slice of 100–200 g, cranial to the 12–13th rib quartering site was removed from the longissimus dorsi muscle, frozen at −20°C and stored for future analysis of the intramuscular fat (IMF%). The left side of each carcass was described according to MSA (see Perry et al. 2001; Thompson 2002) by qualified MSA graders who determined their grade and the predicted eating quality of meat cuts. MSA feedback (chiller) data were used to predict the eating quality of the meat (PEQ) as generated by the MSA model.
Statistical analyses
Data were analysed by using REML variance components analysis procedure on Genstat 9 Release 91 for Windows XP (Lawes Agricultural Trust, Rothamstead, UK). The initial model included the following: the fixed effects of season; postweaning growth path (fast or slow); breed of dam (sire and dam of dam), sire breed type, sire class, sex and all interactions; and random effects of sire (within breed), dam, year of birth, owner (property of origin) and all interactions (chiller grader was also used for appropriate parameters) were used as absorbing factors. Sire class was a term where the sire-breed carcass types were classified as high yielding (Belgian Blue, Limousin and Ang RBY), high marbling (Ang IMF and both Wagyu breeds) or high yielding and high marbling (Ang IMF and RBY). The model was refined over progressive runs by eliminating random terms that showed negative (or aliased) variance components and by removing non-significant fixed effects. The final model was then used to evaluate treatment effects and produce predicted means. ‘Dimensional’ traits (P8- and rib-fat depths and EMA) can be affected by the size of the carcass so we needed to account for differences in HSCW to detect effects independent of response in HSCW (growth-rate response). HSCW was included as a covariate for all other carcass traits and was significant in most cases. Age was used as a covariate for the liveweight and HSCW traits. The fixed model used was as follows:
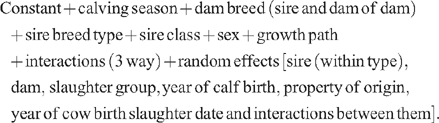
Results
Effect of growth treatment
Growth rate
There were significant differences between the high and low growth-rate treatments, with the predicted mean postweaning growth rate of the autumn-born fast and slow growth paths being 0.62 and 0.49 kg/day, respectively, and for the spring-born groups, 0.74 and 0.49 kg/animal.day, respectively (s.e.d. ± 0.04), with an overall mean of 0.69 and 0.49 kg/head.day for the fast and slow groups, respectively (s.e.d. ± 0.006). The resultant difference in mean slaughter age between the high and low growth-path groups was 172.5 days. Liveweight change owing to growth treatment is shown in Fig. 1.
Carcass traits
Postweaning growth treatment affected dressing percentage, P8-fat depth, marble score, IMF%, and ossification (Table 1). Even though the project aimed to have cattle from both growth treatments slaughtered at the same liveweight, the slow growth-path cattle were 11.9 kg heavier (P = 0.016) at slaughter than the fast growth-path cattle. There was no effect of growth path (P > 0.05) on HSCW, owing to the higher dressing percentage (P = 0.030) of the high growth treatment (53.2% compared with 52.3%). The effects of growth treatment on weight and carcass traits are shown in Table 1.
All fatness traits of the cattle on the fast-growth treatment increased compared with the slow-growth treatment. The cattle on the fast-growth treatment were fatter at the P8 site (P < 0.001, 12.8 v. 11.4 mm) and at the rib site (P = 0.011, 7.2 v. 6.6 mm), had a higher MSA AUS (P < 0.001, 1.49 v. 1.25) and MSA US (P < 0.001, 377.9 v. 349.8) marble score, a higher IMF% (P < 0.001, 3.1 v. 2.6%) and a higher number of carcasses meeting the MSA PEQ (for the anterior striploin cut) (P < 0.001, 53.5 v. 52.1%). Because the fast growth-treatment cattle were younger, they had a lower ossification score than did the slow-treatment group (P < 0.001, 158.9 v. 192.3). There was no difference in VIA-scan carcass yield between the growth treatments.
Although EMA was not significantly affected by growth path alone, there was a growth path by sire breed interaction, owing to the influence of the Belgian Blue. The Belgian Blue progeny on the slow-growth treatment had a larger EMA, whereas the progeny from the other breeds had a smaller EMA on the same slow-growth treatment (Fig. 2). When the Belgian Blue sires were omitted from this analysis (numbers in parentheses Table 1) the growth treatment by breed interaction disappeared, and the cattle grown on the fast-growth treatment had a larger EMA (P < 0.006) than those on the slow-growth treatment.
![]() |
Effect of sire breed type
It should be noted that this project was not designed specifically as a breed comparison, it was designed to examine effects of growth pathways on cattle that were bred using sires extreme in carcass traits. Thus, the sires used were chosen to represent extremes in IMF% and RBY%, both within breed (Angus) and between breed. The breeds other than the Angus were chosen because it was considered that the sires used would be more extreme in those particular traits. Sire breed type had a significant effect on all liveweight and carcass traits (Table 2). It should be noted that there were considerably fewer progeny from the Belgian Blue and Red Wagyu sires, which emphasises that the results for these breeds should be viewed with some caution.
![]() |
Liveweights
The Belgian Blue-sired progeny were significantly (P < 0.008) heavier at weaning (266.9 kg) than those sired by either the Red Wagyu or Wagyu, (249.4 and 238 kg, respectively). The progeny of the Red Wagyu (514.6 kg) and Wagyu (502 kg) sires were significantly (P < 0.001) lighter than the other sire breeds at slaughter; there were no significant differences among the other sire types.
Carcass traits
The Red Wagyu (274.8 kg) and Wagyu (262.9 kg) sires produced progeny with the lowest HSCW. There was no difference among the Limousin (298.8 kg), Angus RBY (295.7 kg), Angus RBY and IMF (294.6 kg) or Angus IMF (293.9 kg). The HSCW of the Belgian Blue progeny at 306.8 kg was higher than that of the Wagyu and Angus IMF, whereas it was no higher than that of the Limousin, Angus RBY or Angus RBY and IMF. Progeny from both the Belgian Blue (3.9 mm) and Limousin (5.2 mm) sires had lower rib-fat depth than the progeny of other sire breeds, and the progeny of Angus sires selected for high RBY% had a significantly lower rib-fat depth (6.8 mm) than those from Angus sires selected for high IMF% (8.5 mm) and those from the Red Wagyu and Wagyu sires (8.0 and 8.1 mm, respectively) (P < 0.001). Sire breed type had a similar effect on P8 fat, with the Belgian Blue and Limousin (7.5 and 9.1 mm, respectively) having significantly (P < 0.001) less fat at that site than the other sire types. There was no significant difference between the Angus sire types and the Wagyus progeny.
The progeny from the Angus IMF sires had higher MSA AUS and US marble scores (1.6 and 393, respectively) than the progeny from the Angus sires selected for high RBY%. (1.3 and 362, respectively, P < 0.001). There was no significant difference between the progeny of the Angus IMF sires and those from Wagyu sires. The progeny of the Belgian Blue and Limousin sires had lower MSA AUS (0.75 and 0.91, respectively) and US marble scores (277 and 297, respectively) than all the other sire types (P < 0.001). Sire breed type also significantly affected intramuscular fat (IMF%). Progeny of all sires selected for high IMF%, (including the Wagyu sires but excluding the Angus sires selected for both traits of high IMF% and high RBY%) had a higher IMF% than those selected for high RBY% (including the Belgian Blue, Limousin and Angus RBY) (P < 0.001).
There was no significant difference in the EMA of progeny from the different Angus sire types. The Limousin-sired progeny had a significantly (P < 0.001) higher EMA (76.5 cm2) than those from all sires apart from the Belgian Blue (75.7 cm2). The progeny of sires selected for high RBY% and grown on the fast growth path tended to have larger EMA, as did the progeny of other sire types, apart from the Belgian Blue (Fig. 3).
![]() |
The VIA-scan carcass yields (%) of the progeny of sires elected for high IMF%, namely Angus IMF (67.5%), Wagyu (67.8%) and Red Wagyu (67.7%), were significantly (P < 0.001) lower than those of the progeny of sires selected for high RBY%, namely Limousin (70%), Belgian Blue (70.8%), Angus RBY (68.6%) as well as the Angus RBY and IMF (68.3%) (Table 2).
The progeny of both the Limousin (53.9%) and Belgian Blue (54.4%) had significantly (P < 0.001) higher dressing percentages than did the progeny of the other five sire types; there was no difference between the Angus sire types. The sire types selected for high RBY%, i.e. Belgian Blue (50.3) and Wagyu (50.7), had progeny with a significantly (P < 0.001) lower predicted eating-quality score (PEQ) for the strip loin cut predicted from the MSA model than did the other breed types. The PEQ of 54.5 for the progeny of the Red Wagyu was significantly higher than that for both the Angus RBY (52.8) and Wagyu (53.3). There was a trend for the Angus IMF progeny to have a higher PEQ than that for the Angus RBY. There was no effect of sire breed type on ossification.
Effect of sex
Liveweight traits
Steers were heavier than females at weaning (284.8 v. 262.2 kg, P < 0.001) and slaughter (568.5 v. 520.6 kg, P < 0.001) and had a higher HSCW than did females (301.1 v. 278.1 kg, P < 0.001). There was a calving season by sex interaction, with the autumn-born male calves (284.8 and 262.2 kg) being heavier than their female counterparts at weaning (243.7 and 232.9 kg) (P < 0.001) (Table 3).
![]() |
Carcass traits
Female progeny were fatter than the steer progeny at both the P8 (12.8 v. 11.4 mm, P < 0.001) (Fig. 3) and rib site (7.3 v. 6.6 mm, P < 0.001), and had higher marble scores (AUS – 1.5 v. 1.2, P < 0.001; US – 383.4 v. 344.2, P < 0.001) and a higher IMF% (3.1 v. 2.6%; P < 0.001) than did the steer progeny. They also had a larger eye muscle area when HSCW was used as a covariate (75.0 v. 72 cm2, P < 0.001), whereas the steer progeny had a higher MSA PEqn (53.6 v. 52.1, P < 0.001). There was no difference in dressing percentage between the sexes (P = 0.08). Females had a higher ossification score (192.3) than did the males (158.9) (P < 0.001). There was an interaction (P < 0.001) between the sex and sire breed type on fat depth, with the females from the Angus RBY and IMF and Angus IMF sires having more P8 fat than their steer counterparts (86.8% and 71.4%, respectively), compared with the females from the Angus RBY sires (52.8% more fat than their steer siblings). The females from the Wagyu sires also had less fat than their steer siblings, in comparison to the females from the Angus sires selected for IMF% (Fig. 3).
Discussion
Growth treatments
Although the growth treatments chosen were not extreme in terms of weight gain per day achieved, they resulted in a mean difference of 172.5 days in the age of slaughter and were different enough to affect several carcass traits. They had the greatest influence on the fatness traits, with the animals finishing at a younger age being fatter with greater P8 and rib-fat depths, and having higher IMF% at the same weight.
These findings agree with those of Cafe et al. (2006). These authors found that when Wagyu × Hereford steers were grown slowly or rapidly to weaning and then backgrounded to a similar feedlot entry liveweight of 543 and 548 kg, respectively, the steers from the group that grew fastest (P < 0.001) during backgrounding (0.63 kg/day) were fatter (11.4 v. 9.5 mm) (P = 0.049) at the P8 site than those from the group that grew more slowly at 0.55 kg/day. Even though there was a 79-kg difference between these two groups at weaning and only a 43-day difference at feedlot entry, it seemed to have been the growth period from weaning to feedlot entry that influenced the fat deposition, rather than the preweaning growth period. The majority of previous studies published on effect of growth rate on carcass composition report on the effects of growth restriction before feedlot entry and subsequent compensatory growth during the feedlot phase, (Drouillard and Kuhl 1999; Choat et al. 2003; Sainz and Vernazza Paganini 2004), with the animals grown faster during the feedlot period tending to be fatter at slaughter. Accumulation of body fat occurs in proportion to the excess energy provided beyond that required for maintenance, skeletal growth and protein accretion. During periods of suboptimal nutrition, deposition of muscle and skeletal tissue proceeds at the expense of body fat, potentially leading to a lower percentage of body fat (Drouillard and Kuhl 1999). McCurdy et al. (2005) found that steers that grew at 0.95 kg/day, compared with those that grew at 1.03 kg/day, and finished to a similar liveweight, had a lower marble score; however, there was no difference in rib fatness or yield. Steers that grew faster also had a higher dressing percentage. Sainz and Vernazza Paganini (2004) concluded that prolonged grazing may decrease quality grade, either by impairing the ability of the animal to deposit intramuscular fat or by decreasing the time during which dietary energy supply is adequate for intramuscular fat deposition to occur. Owens et al. (1995), in a review of aspects of growth and development of feedlot cattle, stated that when net energy intake is restricted, the rate of fat accretion is often reduced although protein growth may continue at nearly normal rates if protein intake is adequate. These authors suggested that the rate of fat accretion during the finishing period was more closely related to time rather than bodyweight. Even though our results showed that the differences in treatment growth rates were relatively small, they were divergent enough for differences in fat accretion rate to have been affected.
The results of the present study add to the debate regarding body composition at a given liveweight, with the widely held concept being that composition is fixed for a particular liveweight (Jesse et al. 1976; Owens et al. 1995). Owens et al. (1995), in their review, reported that some researchers (Slabbert et al. 1992) found that by restricting feed intake, fat content of gain was reduced compared with animals fed ad libitum. Likewise, Murphy and Loerch (1994) found that steers fed to the same liveweight, but on a lower feed intake, had less fat at the 12th rib, and a lower carcass quality grade, even though the low-growth group were fed for an additional 20 days. Tudor et al. (1980) also concluded that composition is not wholly dependent on bodyweight. Their comparison between intensive (fast growth) and pasture-finished (slow growth) animals (slaughtered between 370 and 400 kg of liveweight at 462 and 771 days of age, respectively) showed large differences in body composition that was not explained by diet alone. Ridenour et al. (1982), after examining the effect of different nutritional and management programs on carcass characteristics, found that steers slaughtered at a similar liveweight had different dressing percentages, EMA, and kidney-, heart- and pelvic-fat percentages (KHP%), depending on days on feed. Those fed to the same liveweight over a shorter period had a higher dressing %, higher KPH% and a larger EMA than did those fed over a longer period. Those authors found no effect on fat thickness, and as with the present study, they found no effect on yield. Hersom et al. (2001) also found that animals reaching a similar liveweight at a younger age were fatter at the 12th rib site, and had a higher KPH% than those reaching a similar weight at an older age. Those authors and Hersom et al. (2004) also found no difference in either yield or dressing percentage.
The reported effect of growth patterns between weaning and slaughter on carcass measurements seem to be variable. Ross et al. (2005) concluded that it may be possible to influence fat deposition of fat depots independently of each other because gene expression in different adipose tissue depots might be regulated differently throughout their accretion during grazing or finishing. However, the results of the present study generally agree with the earlier findings of Berg and Butterfield (1968), Murray et al. (1974), Coleman et al. (1993) and Byers (1982), who stated that a higher plane of nutrition has often been shown to increase the proportion of fat in the carcass, and that it is clear that the fattening process can be enhanced or retarded relative to muscle and bone development by altering the plane of nutrition.
The faster-grown cattle exhibited a higher MSA-predicted eating-quality score, which is not surprising as they had a higher marble score, greater fat depth and lower ossification, which are the drivers of the MSA eating-quality model (Perry et al. 2001; Thompson 2002). Perry and Thompson (2005) showed a curvilinear relationship between finishing growth rate and palatability, which appeared to plateau at a growth rate of ~1.2 kg/day. They concluded that management of contemporary groups to achieve high growth rates resulted in small improvements in eating quality, closely related to differences in group age at slaughter. McKiernan and Wilkins (2007) found no differences in predicted MSA eating quality of animals of similar breeding as used in the present project and subjected to fast- or slow-growth treatment before being finished in a feedlot; however, they did find that cattle on fast-growth treatment had a higher IMF%, and meat with a lower shear force. There was also a tendency (P = 0.06) for the cattle on fast-growth treatment to have a higher eating-quality score as determined by an MSA Sensory Eating Quality Panel (Thompson 2002).
Sire breed type
This project was set up specifically to examine differences in sire breed types and, the different breeds (Belgian Blue, Limousin and Wagyu) were used as vehicles to examine the effects of extremes in the carcass traits; therefore, it is not surprising that sire breed type influenced all traits measured, apart from ossification. Casas et al. (2007) reported a non-significant difference of 17 kg in favour of Angus progeny compared with Wagyu at weaning (215 days of age); however, by yearling age Angus progeny were significantly heavier than Wagyu. The Limousin, Belgian Blue and Angus sires in the present study produced calves that were weaned at liveweights similar to those in other research (Williams et al. 1995; Lunstra and Cundiff 2003). Afolayan et al. (2007) found that the Wagyu were lighter at weaning than Angus, Limousin and Belgian blue; however, there was no difference among the latter three breeds.
In choosing sires to represent the three Angus sire types for extremes in carcass traits, sires with moderate growth EBV were selected; consequently, as could be expected, there was no difference in weaning liveweight among the progeny of these Angus sire types. Irwin et al. (2006) also found no differences in weaning weight of Angus selected for the same diverse traits as in the present study, or in that of Charolais, Limousin, Wagyu- or Red Wagyu-sired progeny.
Although the 600-day liveweight breed-comparison values reported by Afolayan et al. (2007) were generally smaller than those in the present study, the differences in the sire breed type were similar, with the Wagyu progeny being 65.6, 64.5 and 75.9 kg lighter, respectively, than Angus, Limousin and Belgian Blue progeny. Wertz et al. (2002) and Wheeler et al. (2004) reported similar differences between Wagyu- and Angus-sired progeny, and Mir et al. (2002) found that Wagyu progeny comprising greater than 87% Wagyu were 94 kg lighter at slaughter compared with Limousin.
Wertz et al. (2002) and Wheeler et al. (2004) also reported differences among Angus, Wagyu and Limousin in HSCW similar to those in the present study, and Pitchford et al. (2002) reported that Wagyu-cross carcasses were lighter than those from Limousin, Angus and Belgian Blue, with no differences among the latter three breeds. On the basis of the foregoing, it is obvious that if Wagyu sires are used with the aim to increase marbling and IMF%, their progeny will be smaller and would be disadvantaged if traded on a liveweight or carcass weight basis only.
The higher dressing percentages (DP) of the Euro sire types than the Angus and Wagyu types were expected, and have also been reported by Ríos-Utrera et al. (2006) who found that increases owing to Limousin ranged from 1.6 to 2.2%, depending on carcass weight. Gregory et al. (1994b) Koch et al. (1976) and Wheeler et al. (2005) found a 2.1, 1.0 and 1.2% advantage in favour of Limousin compared with Angus. As in the present study, Wheeler et al. (2001) reported that Belgian Blue progeny had a higher dressing percentage than did Angus progeny, and as with the present study, Casas and Cundiff (2006) found little difference in DP between Wagyu and Angus. As in the present study, McIntyre (2007) also reported no differences among the Angus sire types. Although differences in DP are apparent in breeds selected for extreme RBY%, such as the Belgian Blue and Limousin, differences owing to the selection for RBY% within the Angus sire carcass types are not apparent.
Fatness traits
Sire carcass type strongly influenced all fatness traits (P8 and rib fatness, marbling and IMF%) which is not surprising as the sire types were chose to represent extremes in both IMF% and yield. Whereas Pitchford et al. (2006) reported significant differences between Angus and Wagyu, the present study and Wheeler et al. (2004) found no difference in P8 measurements between progeny of those two sire-breed carcass types. However, differences between Limousin and Belgian Blue, and Angus were consistent with those in the present study and those of Afolayan et al. (2007), Pitchford et al. (2002), Ríos-Utrera et al. (2006) and Wheeler et al. (2001). Although there was a trend for the progeny of the Angus IMF sires to be fatter at the P8 site than those of the Angus RBY, because of the variation encountered, the difference was not significant. However, there were significant differences in rib-fat measurements, with the progeny of the Angus IMF being fatter than those of Angus RBY. McIntyre (2007), who used similar Angus sire types, also found no difference in P8-fat depth, although the progeny of Angus IMF sires had more rib fat than those of Angus RBY sires. This is not surprising, as others (Reverter et al. 2003; Wheeler et al. 2005) have found a significant relationship between marbling and subcutaneous fatness and Wheeler et al. (2005) stated that it would be difficult, although not impossible, to decrease subcutaneous-fat thickness without lowering the marbling level. Wheeler et al. (2001) also found a moderate correlation among marbling, IMF% and fat thickness. Even though the mean marble scores and IMF percentages were lower than those in other published reports (Burrow et al. 2001; Pitchford et al. 2002), the ranking of differences owing to sire breed type were comparable across all breed types. Although there may have been an expectation that the Wagyu-sired progeny would have had a higher intramuscular fat content than the progeny sired by Angus (Wertz et al. 2002), that was generally not the case, apart from the progeny from Angus selected for high RBY%. Pitchford et al. (2006) and Lunt et al. (1993) also found no difference in IMF% between Angus and Wagyu, and Wheeler et al. (2004) found no marble-score differences between Angus and Wagyu.
Direct selection for IMF% and RBY% in the Angus sires used in the present study was certainly reflected in significant differences in IMF% and marbling of the progeny. This finding was supported by McIntyre (2007), McKiernan and Wilkins (2007) and Vieselmeyer et al. (1996) who compared six Angus bulls with high (> +0.4) and six bulls with low (< –0.16) expected progeny differences (EPD) for marbling to evaluate the impact of marbling on progeny production and carcass traits. These authors found that marbling EPD class had no effect on rib-fat thickness, yield grade, carcass weight or EMA; however, more carcasses of calves from sires with high EPD for marbling graded USDA Choice (contained a higher degree of marbling) than did from low EPD-marbling sires. They concluded that animals could be selected for marbling to increase grading in choice without effecting yield or increasing subcutaneous fat. The present study indicated that the appropriate use of sires selected within a breed for IMF% can result in positive changes in marbling, without compromising yield. Heritability estimates for marbling support this, and have been reported to range from 0.23 to 0.73 (Vieselmeyer et al. 1996; Wheeler et al. 1996; Ríos-Utrera et al. 2006), with more recent studies (Burrow et al. 2001) reporting that carcass-composition traits are at least moderately heritable and should respond well to genetic selection.
Eye muscle area
Selection for either yield or IMF% in the Angus sires (supported by Vieselmeyer et al. 1996) had no effect on EMA. As supported by Pitchford et al. (2006), Young et al. (1978), Wheeler et al. (2005) and Ríos-Utrera et al. (2006), the present study found that Limousin-sired progeny had larger EMA than did the three Angus types. Unlike Pitchford et al. (2006) and Wheeler et al. (2004), who found no difference between the Wagyu and Angus, the present study and Lunt et al. (1993) found that the Wagyu progeny, when adjusted to HSCW, had larger EMA than those of the Angus, although the Wagyu were significantly lighter at slaughter than the Angus. As with Lunt et al. (1993), the HSCW adjustment produced the EMA differences between these two breeds, and it is not clear in the study of Pitchford et al. (2006) whether age, finish or weight was used as a covariate. Wertz et al. (2002) found no difference in Longissimus thoracis muscle area between Angus and Wagyu, although numerically the Wagyu had a higher Longissimus thoracis area, measured as cm2/100 kg of carcass weight, than did the Angus. However, the difference was not significant.
Carcass yield
Selection for RBY in the Angus sires in the present study and in that of McIntyre (2007) resulted in a higher-yielding progeny for both sire types selected for that trait than did selection for high IMF% in the Angus sires; however, as stated previously, this selection for RBY did not result in a higher EMA. Published heritability estimates for yield ranged from moderate (0.39) to high (0.73); Wheeler et al. (1996), Burrow et al. (2001) and Reverter et al. (2003). Thus, the differences in the yield of the progeny from the Angus sire types were to be expected, and indicate the changes that can be made through appropriate sire selection. As anticipated, both of the Euro sire types produced a higher-yielding progeny, as supported by Wheeler et al. (2001, 2005) and Ríos-Utrera et al. (2006), with the Belgian Blue having the highest yield compared with the other sire types. The yield of Wagyu-sired progeny was not different from that of the Angus IMF-sired progeny, although it was lower than that of the Angus RBY-sired progeny. Wertz et al. (2002) found no difference in a calculated yield estimate between Wagyu and Angus, whereas Wheeler et al. (2005) found that Wagyu yielded more than Angus corrected for age; however, the yields were not different when adjusted for carcass weight. The present study demonstrated that appropriate selection of sires within a breed for both IMF% and RBY% can produce carcasses with both increased marbling and yield, without compromising other carcass traits.
Predicted eating quality
As stated earlier, predicted eating quality produced from the MSA model is derived from many parameters, including IMF%, fatness and ossification. This is reflected in the fat-content differences owing to sire breed type, with the higher-yielding Belgian Blue and Limousin sire types having the lowest predicted eating-quality score of the sire-breed carcass types examined. Although there were no significant differences in ossification score among the sire types, the Belgian Blue and Limousin had the highest predicted means compared with the other breeds, and the Red Wagyu had the lowest. This, together with the differences in fatness traits, would have combined to result in the predicted MSA eating-quality differences. McKiernan and Wilkins (2007) found that sires selected and grouped for high IMF% (including Wagyu and high IMF% Angus) produced progeny with higher MSA-predicted eating-quality scores and higher sensory eating-quality scores as derived from taste panels than did those selected for high RBY% (including Charolais, Limousin and Angus RBY sires).
Although the present study and that of McKiernan and Wilkins (2007) found no differences in the predicted MSA eating quality among the three Angus-sire carcass types, McKiernan and Wilkins (2007) found that Angus selected for high IMF% produced progeny with higher taste panel eating-quality scores than the progeny of Angus sires selected for high RBY%. This indicates that the MSA prediction model may not be sensitive enough to determine the actual differences in the eating quality. McKiernan and Wilkins (2007) stated that there was a clear association of IMF% and marbling scores with palatability, and any strategies that increase IMF% in the carcass should improve eating quality. Gregory et al. (1994a) found sensory taste-panel differences between Angus and Limousin, with the Angus scoring significantly higher in two of the three traits examined. These authors also found a positive correlation between fatness and palatability attributes.
Effects of sex
Sex affected most traits in our study, except dressing percentage. Differences between steers and heifers at Hamilton are, in some aspects, similar to those obtained by Choat et al. (2006), where heifers in one experiment had a lower HSCW, a higher marbling score, a higher EMA and a lower yield, with no difference in rib fat. However, in the same paper, these authors reported that in another experiment they found that heifers had more rib fat, whereas there was no difference in HSCW or marble score. Owens and Gardner (1999) detected significant differences in means for most carcass measurements between steers and heifers. They found that heifers had a lower HSCW, a lower dressing percentage, a higher EMA when adjusted to cm2/100 kg HSCW, were fatter, and had a higher marble score. However, unlike in the present study, Owens and Gardner (1999) found no difference in yield grade. Saul (1983) also found that heifers were fatter, had a higher IMF% and a higher yield than steers.
Jones et al. (1990) reported an interaction with sex and fatness as found in the present study, although with seam fat. As yield decreased, heifers had higher levels of seam fat than did steers at the same yield grade. It is obvious that the relationships between sex and carcass characteristics are not always consistent with fat deposition, generally being more variable (Murphey et al. 1985) in heifers. The present study indicated that if high IMF% sires are used, there is a possibility that, although higher marbling may be achieved, it may result in heifers that may have too much subcutaneous fat and thus be penalised at market. This effect can be seen in Fig. 3. This should not apply to heifers from sires chosen for the combined traits of high RBY% and IMF% as they were not significantly fatter than those chosen for high RBY% alone, and had a similar marble score.
Conclusions
The present Hamilton study showed that postweaning growth, sire carcass type and sex significantly influence carcass traits, and that it is possible to change carcass parameters by using postweaning nutrition and genetic selection of appropriate sire carcass types using EBV, without the implications of sire type by environment interaction. The extreme carcass sire types used (Belgian Blue, Limousin and Wagyu) and sires chosen were obviously reasonably representative of those breeds because carcass differences produced in their progeny were similar to those previously reported and as they produced progeny whose carcasses reflected the two specific traits of interest, namely RBY% and IMF%. The lack of an interaction between postweaning nutrition and the extreme carcass sire types, including the Angus sire carcass types, was pleasing because it simplifies the selection of sires to change particular carcass parameters.
The use of specific carcass-type breeds rather than a selection within a breed to achieve the desired carcass trait in progeny will certainly have the desired effect on that trait; however, consideration should be given to the consequences of doing so, as it may also negatively affect another desirable trait. For instance, choosing a Belgian Blue or Limousin to increase yield would achieve a higher yielding carcass, as shown by the present study; however, it may have a detrimental effect on desirable fatness or eating-quality traits. However, the present study has shown that a selection of traits often considered antagonistic, e.g. IMF% and RBY%, within a breed is possible through the use of BREEDPLAN, with no loss of desirable characteristics. Careful selection of a sire of a different breed that has high EBV of both of these traits should also be possible. These results also indicate that beef industry can have confidence in the use of BREEDPLAN carcass-trait EBV for sire selection, to enable more accurate targeting of carcass specifications; the Angus sires chosen for their traits on EBV produced differences in their progeny commensurate to those traits. The results also showed that weaners grown faster, even though they were slaughtered at liveweights similar to slower-grown weaners, produced the most desirable carcasses regardless of breed.
Acknowledgements
We are grateful for the cooperation and assistance of Cargill Beef for the processing stages of the experiments – thanks go to the many staff and management at the abattoir works at Wagga Wagga (Harry Waddington and Grant Garey, in particular) who provided enthusiastic assistance and access to data. Thanks also go to Don Robertson, ‘Skene’, Hamilton, for the use of his breeding herd and his keen assistance. This project was heavily supported by funding from Meat and Livestock Australia within the Beef CRC 2 (Cooperative Research Centre for Cattle and Beef Quality Australia). We are most grateful for the assistance of the staff of the Meat Science Laboratory at Armidale and the staff of MSA. Staff of the NSW State Department of Primary Industry assisted in abattoir data collection. Thanks go to Brian Clark for management support. Finally, we gratefully acknowledge the inputs of Mr Jim Walkley for his helpful advice in the drafting and revision of this paper.
Afolayan RA,
Pitchford WS,
Deland MPB, McKiernan WA
(2007) Breed variation and genetic parameters for growth and body development in diverse beef cattle genotypes. Animal 1, 13–20.
| Crossref | GoogleScholarGoogle Scholar |
[Verified 18 March 2009]
Graham JF,
Clark AJ,
Hayes GJ,
Kearney GA, Deland MPB
(2000) The effect of genotype on growth and fatness when Angus, Hereford, Limousin and Simmental sires are mated to Angus and Hereford cows. Asian–Australasian Journal of Animal Sciences 13, 325–328.
Graser HU,
Tier B,
Johnston DJ, Barwick SA
(2005) Genetic evaluation for the beef industry in Australia. Australian Journal of Experimental Agriculture 45, 913–921.
| Crossref | GoogleScholarGoogle Scholar |
Gregory KE,
Cundiff LV,
Koch RM,
Dikeman ME, Koohmaraie M
(1994a) Breed effects, retained heterosis, and estimates of genetic and phenotypic parameters for carcase and meat traits of beef cattle. Journal of Animal Science 72, 1174–1183.
Gregory KE,
Cundiff LV,
Koch RM,
Dikeman ME, Koohmaraie M
(1994b) Breed effects and retained heterosis for growth, carcase and meat traits in advanced generations of composite populations of beef cattle. Journal of Animal Science 72, 833–850.
Hersom MJ,
Horn GW,
Krehbiel CR, Phillips WA
(2004) Effect of live weight gain of steers during winter grazing. I. Feedlot performance, carcase characteristics, and body composition of beef steers. Journal of Animal Science 82, 262–272.
Irwin J,
Wilkins JF, McKiernan WA
(2006) Effects of sire genotype and growth rate on the variation in weaning and feedlot entry weights of beef feeder steers. Animal Production in Australia 25, 267.
Jesse GW,
Thompson GB,
Clark JL,
Hedrick HB, Weimer KG
(1976) Effects of ration energy and slaughter weight on composition of empty body and carcase gain of beef cattle. Journal of Animal Science 43, 418–425.
Jones DK,
Savel JW, Cross HR
(1990) The influence of sex-class, USDA yield grade and USDA quality grade on seam fat trim from the primals of beef carcases. Journal of Animal Science 68, 1987–1991.
Koch RM,
Dikeman ME,
Allen DM,
May M,
Crouse JD, Campion DR
(1976) Characterization of biological types of cattle III carcase composition, quality and palatability. Journal of Animal Science 43, 48–62.
Koots KR,
Gibson JP,
Smith C, Wilton JW
(1994) Analyses of published genetic parameter estimates for beef production traits. 1. Heritability. Animal Breeding Abstracts 62, 309–338.
Lunstra DD, Cundiff LV
(2003) Growth and pubertal development in Brahman, Boran, Tuli, Belgian Blue, Hereford and Angus sired F1 bulls. Journal of Animal Science 81, 1414–1426.
Lunt DK,
Riley RR, Smith SB
(1993) Growth and carcase characteristics of Angus and American Wagyu steers. Meat Science 34, 327–334.
| Crossref | GoogleScholarGoogle Scholar |
Marshall DM
(1994) Breed differences and genetic parameters for body composition traits in beef cattle. Journal of Animal Science 72, 2745–2755.
McKiernan WA,
Wilkin JF,
Barwick SA,
Tudor GD,
McIntyre BL,
Graham JF,
Deland MPD, Davies L
(2005) CRC ‘Regional Combinations’ Project – Effects of genetics and growth paths on beef production and meat quality: experimental design, methods and measurements. Australian Journal of Experimental Agriculture 45, 959–969.
| Crossref | GoogleScholarGoogle Scholar |
Mir PS,
Mir Z,
Kuber PS,
Gaskins CT,
Martin EL,
Dodson MV,
Elias Calles JA,
Johnson KA,
Busboom JR,
Wood AJ,
Pittenger GJ, Reeves JJ
(2002) Growth, carcase characteristics, muscle conjugated linoleic acid (CLA) content, and response to intravenous glucose challenge in high percentage Wagyu, Wagyu x Limousin, and Limousin steers fed sunflower oil-containing diets. Journal of Animal Science 80, 2996–3004.
Murphey CE,
Johnson D,
Smith GC,
Abraham HC, Cross HR
(1985) Effects of sex related differences in external fat deposition on subjective carcase fatness evaluations-steer versus heifer. Journal of Animal Science 68, 1987–1991.
Murphy TA, Loerch SC
(1994) Effects of restricted feeding of growing steers on performance, carcase characteristics and composition. Journal of Animal Science 72, 2497–2507.
Murray DM,
Tulloh NM, Winter WH
(1974) Effects of three different growth rates on empty body weight, carcase weight and dissected carcase composition of cattle. Journal of Agricultural Science 82, 535–547.
Owens FN, Gardner BA
(1999) A review of the impact of feedlot management and nutrition on carcase measurements of feedlot cattle. Journal of Animal Science 77, 1–18.
Owens FN,
Gill DR,
Secrist DS, Coleman SW
(1995) Review of some aspects of growth and development of feedlot cattle. Journal of Animal Science 73, 3152–3172.
Perry D, Thompson JM
(2005) The effect of growth rate during backgrounding and finishing on meat quality traits in beef cattle. Meat Science 69, 691–702.
| Crossref | GoogleScholarGoogle Scholar |
Perry D,
Shorthose WR,
Ferguson DM, Thompson JM
(2001) Methods used in the CRC program for the determination of carcase yield and beef quality. Australian Journal of Experimental Agriculture 41, 953–957.
| Crossref | GoogleScholarGoogle Scholar |
Pethick DW,
Harper GS, Oddy VH
(2004) Growth, development and nutritional manipulation of marbling in cattle, a review. Australian Journal of Experimental Agriculture 44(7), 705–715.
| Crossref | GoogleScholarGoogle Scholar |
Pitchford WS,
Deland MPB,
Siebert BD,
Malau-Aduli AEO, Bottema CDK
(2002) Genetic variation in fatness and fatty acid composition of crossbred cattle. Journal of Animal Science 80, 2825–2832.
Pitchford WS,
Mirzaei HM,
Deland MPB,
Afolayan RA,
Rutley DL, Verbyla AR
(2006) Variance components for birth and carcase traits of crossbred cattle. Australian Journal of Experimental Agriculture 46, 225–231.
| Crossref | GoogleScholarGoogle Scholar |
Reverter A,
Johnston DJ,
Perry D,
Goddard ME, Burrow HM
(2003) Genetic and phenotypic characterization of animal, carcase, and meat quality traits from temperate and tropically adapted beef breeds. 2. Abattoir carcase traits. Australian Journal of Agricultural Research 54, 119–134.
| Crossref | GoogleScholarGoogle Scholar |
Ridenour KW,
Kiesling HE,
Lofgreen GP, Stiffier DM
(1982) Feedlot performance and carcase characteristics of beef steers grown and finished under different nutrition and management programs. Journal of Animal Science 54, 1115–1119.
Ríos-Utrera A,
Cundiff LV,
Gregory KE,
Koch RM,
Dikeman ME,
Koohmaraie M, Van Vleck LD
(2006) Effects of age, weight and fat slaughter end points on estimates of breed and retained heterosis effects for carcase traits. Journal of Animal Science 84, 63–87.
Robinson DL,
Oddy VH,
Dicker RW, McPhee M
(2001) Post-weaning growth in northern New South Wales. 3. Carry-over effects on finishing, carcase characteristics and intramuscular fat. Australian Journal of Experimental Agriculture 41, 1041–1049.
| Crossref | GoogleScholarGoogle Scholar |
Ross JW,
Smith TK,
Krehbiel CR,
Malayer JR,
DeSilva U,
Morgan JB,
White FJ,
Hersom MJ,
Horn GW, Geisert RD
(2005) Effects of grazing program and subsequent finishing on gene expression in different adipose tissue depots in beef steers. Journal of Animal Science 83, 1914–1923.
Sainz RD, Vernazza Paganini RF
(2004) Effects of different grazing and feeding periods on performance and carcase traits of beef steers. Journal of Animal Science 82, 292–297.
Saul GR
(1983) The composition, fat distribution and yield of carcase beef from steers and heifers when entired, spayed, pregnant, or fitted with an intravaginal device. Australian Journal of Experimental Agriculture and Animal Husbandry 23, 354–357.
| Crossref | GoogleScholarGoogle Scholar |
Slabbert N,
Campher JP,
Shelby T,
Leeuw KJ,
Kuhn GP, Meissner HH
(1992) The influence of dietary energy concentration and feed intake level on feedlot steers. 3. Carcase composition and tissue growth as influenced by rate of gain. South African Journal of Animal Science 22, 115–121.
Thompson JM
(2002) Managing meat tenderness. Meat Science 62, 295–308.
| Crossref | GoogleScholarGoogle Scholar |
Tong AKW,
Robinson DJ,
Robertson WR,
Zawadski SM, Liu T
(1999) Evaluation of the Canadian vision system for beef carcase grading. Proceedings of the 45th International Congress of Meat Science and Technology 45, 374–375.
Trenkle A,
DeWitt DL, Topel DG
(1978) Influence of age, nutrition and genotype on carcase traits and cellular development of the M. longissimus of cattle. Journal of Animal Science 46, 1597–1603.
Tudor GD,
Utting DW, O’Rourke PK
(1980) The effect of pre- and post-natal nutrition on the growth of beef cattle. III. The effect of severe restriction in early post-natal life on the development of the body components and chemical composition. Australian Journal of Agricultural Research 31, 191–204.
| Crossref | GoogleScholarGoogle Scholar |
Upton W,
Burrow HM,
Dundon A,
Robinson DL, Farrell EB
(2001) CRC breeding program design, measurements and database, methods that underpin CRC research results. Australian Journal of Experimental Agriculture 41, 943–952.
| Crossref | GoogleScholarGoogle Scholar |
Vieselmeyer BA,
Rasby RJ,
Gwartney BL,
Calkins CR,
Stock RA, Gosey JA
(1996) Use of expected progeny differences for marbling in beef. I. Production traits. Journal of Animal Science 74, 1009–1013.
Wertz AE,
Berger LL,
Walker PM,
Faulkner DB,
McKeith FK, Rodriguez-Zas SL
(2002) Early-weaning and post weaning nutritional management effect feedlot performance, carcase merit, and the relationship of 12th-rib fat, marbling score, and feed efficiency among Angus and Wagyu heifers. Journal of Animal Science 80, 28–37.
Wheeler TL,
Cundiff LV,
Koch RM, Crouse D
(1996) Characterization of biological types of cattle (Cycle IV), carcass traits and longissimus palatability. Journal of Animal Science 74, 1023–1035.
Wheeler TL,
Cundiff LV,
Shackelford SD, Koohmaraie M
(2001) Characterization of biological types of cattle (Cycle V), carcase traits and longissimus palatability. Journal of Animal Science 79, 1209–1222.
Wheeler TL,
Cundiff LV,
Shackelford SD, Koohmaraie M
(2004) Characterization of biological types of cattle (Cycle VI), carcase, yield, and longissimus palatability traits. Journal of Animal Science 82, 1177–1189.
Wheeler L,
Cundiff LV,
Shackelford SD, Koohmaraie M
(2005) Characterization of biological types of cattle (Cycle VII), carcase, yield, and longissimus palatability traits. Journal of Animal Science 83, 196–207.
Williams CB,
Bennett GL, Keele JW
(1995) Simulated influence of post weaning production system on performance of different biological types of cattle. I. Estimation of model parameters. Journal of Animal Science 73, 665–673.
Wood JD,
Enser M,
Fisher AV,
Nute GR,
Richardson RI, Sheard PR
(1999) Manipulating meat quality and composition. Proceedings of the Nutrition Society 58, 363–370.
Young LD,
Cundiff LV,
Crouse JD,
Smith GM, Gregory KE
(1978) Characterization of biological types of cattle. VIII. Post weaning growth and carcase traits of three-way cross steers. Journal of Animal Science 46, 1178–1191.
Zembayashi M
(1994) Effects of nutritional planes and breeds on intramuscular-lipid deposition in M. longissimus dorsi of steers. Meat Science 38, 367–374.
| Crossref | GoogleScholarGoogle Scholar |