Use of electrolyte and betaine water supplementation to support improved liveweight gain of commercial Pekin ducks exposed to adverse high temperature in the week prior to processing
J. A. Downing
A
Abstract
Commercial Pekin Ducks housed in conventional open-sided sheds often experience heat stress in summer. Electrolyte or osmolyte supplements can help birds cope under heat stress.
To assess the effects of water electrolyte/betaine supplementation or betaine in feed on the growth performance of Pekin ducks exposed to high ambient temperature.
Commercial Cherry Valley Pekin ducks were fed diets with a dietary electrolyte balance (DEB) of 160 (L-DEB) or 209 (H-DEB) over Days 15–41 (D15–41) of age. Over D36–41, ducks were provided with water alone or supplemented with betaine in the feed (BF), or betaine and an ‘in-house’ electrolyte (E) formulation at 50%, 100% or 150% (D36–41) or at 100% for only 36 h on D40–41. There were 12 treatments with four replicate pens for each treatment. On D36–41 of age, temperature was increased to 28–32°C for 9 h (from 08:30 hours to 17:30 hours) and then returned to 22–24°C for the remainder of the day. Individual liveweights were taken on D28, D35, D41. During these times, feed intake and water consumption were determined. On D41, one male and one female from each pen were weighed, euthanised and breast muscle was removed and weighed. Birds were collected for commercial processing at 04:00 hours on D42.
Over D15–35, the DEB had no effect on bird performance. Over D36–41, for liveweight gain (LWG) there were significant interactions between treatment × week (P < 0.001) and treatment × diet (P = 0.017). Supplements E150 and E100 + 36 h supported LWG more than did other treatments, while treatments BF, E50 and E100 supported higher LWG than in controls. On the L-DEB diet, the control birds had a LWG lower than in other treatments (P < 0.05). On the H-DEB diet, the E50 supplement had highest LWG, but comparable to that in E100 + 36 h. The supplements had no effects on breast muscle yield, weight losses during transport and lairage or processed carcass weights.
The electrolyte supplements supported higher LWG during exposure to moderately adverse high temperature. Supply for 36 h supported LWG equivalent or better than did other treatments given over 6 days. The benefits could be related to increased water intake and not just electrolyte supply.
Water electrolyte plus betaine supplementation supports improved Pekin duck performance during a moderately high temperature challenge.
Keywords: breast muscle weight, heat stress, liveweight gain, Pekin ducks, water intake.
Introduction
During periods of high temperature, commercial meat birds dissipate excess heat by panting and this results in excretion of CO2 at a rate greater than cellular production. This can result in respiratory alkalosis as the HCO3− concentration in blood increases. Coupled with Na+ and K+ ions, the kidney excretes HCO3− in an effort to restore normal blood pH (Johnson 2008; Wasti et al. 2020). The loss of these ions can result in an acid–base imbalance (Borges et al. 2007; Popoola et al. 2020a), which is detrimental to bird performance (Ahmad et al. 2009; Mushtaq et al. 2013). The acid–base imbalance affects enzyme activities and can result in metabolic disorders (Mushtaq et al. 2013).
Supplying additional electrolytes in feed or water are strategies used to replace electrolyte losses and help meat birds cope with the electrolyte imbalance caused by heat stress (Lin et al. 2006; Mushtaq et al. 2013; Roushdy et al. 2018; Shao et al. 2019; Popoola et al. 2020b). The diet electrolyte balance (DEB) participates in acid–base regulation (Murakami et al. 2001; Sayed and Downing 2015; Popoola et al. 2020a; Araujo et al. 2022) and the concentrations of Na+, K+ and Cl− are used to estimate DEB as milliequivalents per kilogram (mEq/kg; Mongin 1981).
Effects of DEB depend on the basal diet, genotype, temperature, and the electrolytes used and the relative concentrations of these (Borges et al. 2003; Ahmad et al. 2005; Ahmad and Sarwar 2006; Mushtaq et al. 2013; Popoola et al. 2020a; Yu et al. 2022). This means that identifying an optimal DEB would be difficult and could account for the variation in published results. Under thermoneutral conditions, a DEB ranging from 200 to 330 mEq/kg has been shown to support best performance in different studies (Johnson and Karunajeewa 1985; Borges et al. 2004; Szabó et al. 2011; Popoola et al. 2020b).
Under summer conditions, a DEB of 240 mEq/kg is optimal (Borges et al. 2003), whereas under tropical conditions (temperature range of 26.1–37.5°C) bird performance has been shown to be poorer when the DEB is 0 and 350 mEq/kg than when it is 50 or 250 mEq/kg (Ahmad et al. 2009). At high temperature, a range of 150–250 mEq/kg was found suitable by Ahmad et al. (2009). A DEB of 240 and 270 mEq/kg enhances protein efficiency, liveweight gain (LWG) and feed efficiency under high temperature (Popoola et al. 2020b). At 29–34°C, diets with a DEB of 120, 220 and 320 mEq/kg, when fed to finisher meat birds, have been shown to have no effect on performance (Saki et al. 2016).
Consequences of high ambient temperature causing heat stress (HS) in meat chickens are reduced LWG and feed intake (FI), with resultant poorer feed conversion efficiency (FCR) (Mushtaq et al. 2013; Zhong et al. 2018; Liu et al. 2019: Shin et al. 2019; Downing 2022). At high temperature (27–38°C), FI was 15% lower (Yalcin et al. 1997) and even at a moderately high temperature of 27°C FI was 7–14% lower than at 21°C (Suk and Washburn 1995). Supplying electrolytes in drinking water rather than in feed could compensate for their reduced uptake associated with the lower FI (Dai et al. 2009) during high temperature. Salts of sodium and potassium (NaCl, Na2CO3, KCl and K2CO3) are common water supplements used to support poultry during HS (Smith and Teeter 1993; Smith 1994; Mushtaq et al. 2013; Wasti et al. 2020). Under high ambient temperatures, providing NaHCO3-supplemented drinking water has been reported to improve broiler performance and survivability (Ahmad et al. 2006). Using a combination of NaHCO3, NaCl and KCl helps improve the performance of meat chickens exposed to high temperature (Downing et al. 2017; Elshafaei et al. 2020). The effect of electrolytes, at different inclusion rates, have proved beneficial under different, challenging high temperatures regimes.
The effects that DEB and water electrolyte supplementation have on Pekin duck performance have received limited investigation. Of the limited research, most has examined the effects of DEB on egg-laying duck strains (Wang et al. 2011; Nawab et al. 2018). So, under high temperature stress Pekin ducks can achieve greater electrolyte intake by increasing DEB or supplementing the water. However, it is often difficult to predict periods of adverse high temperature, so providing supplemented water provides management with the potential to supply extra electrolytes with limited warning of upcoming, challenging high-temperature conditions. In a previous publication, providing water supplemented with electrolytes and betaine improved LWG in Pekin ducks exposed to a high-temperature thermal challenge in the final week of the 6-week production cycle (Downing 2022).
Betaine is a trimethyl derivative of glycine and can function as a methyl-group donor, an organic osmolyte and antioxidant (reviewed by Saeed et al. 2019, Mishra et al. 2021 and Alagawany et al. 2022). As an osmolyte, betaine can help regulate osmotic pressure in cells and help limit dehydration during HS (Sayed and Downing 2015; Al-Abdullatif et al. 2021). When included as a feed additive, betaine can improve commercial chicken and duck performance and meat quality, especially when they are exposed to high-temperature HS (Liu et al. 2019; Park and Zammit 2019; Shin et al. 2019; Al-Garadi et al. 2023).
In earlier studies, Cherry Valley Pekin ducks were fed a basal diet, or the same diet supplemented with either 400, 800 or 1200 mg/L betaine in the drinking water and exposed to cyclic high temperature (33–43°C for 6 h daily 22°C) on Days 22–42 of age (Shin et al. 2019). The betaine supplemented from the lowest to highest rates supported higher LWG by 9.8%, 10.4% and 15.7% respectively. The improved growth was supported by increases of 9.2%, 7.6% and 10.1% in FI. The supplements also increased the plasma Na+, K+ and Cl− electrolyte concentrations. Meat ducks during 22–42 days of age were exposed to thermo-neutral (TN) temperature of 24°C, high temperature (HT) of 34°C for 5 h/day, and feed withdrawal (5 h both morning and evening) and supplied with 800 g/kg betaine in the feed. The cyclic HT depressed LWG by 28.6%, while the betaine supplementation limited this to 10.1%. Interestingly, the researchers included a fourth treatment where betaine was included in the feed along with 300 mg/L of ascorbic acid in the drinking water, and this supported LWG equivalent to that of the TN control birds (Park and Zammit 2019).
The objective of the current experiment was to assess the effects that DEB and different concentrations of a previously used water electrolyte supplement had on the growth performance of Pekin ducks exposed to cyclic high ambient temperature during the last week of a 41-day production cycle. The working hypothesis was that increased electrolyte intake would benefit performance during the period of high temperature and that this could be met by increasing DEB or electrolyte supplementation of the water.
Materials and methods
All experimental protocols were approved by the University of Sydney Animal Care and Ethics Committee (Protocol: 2013/5903) and complied with the Australian Code of Practice for the use of Animals for Scientific Purposes National Health and Medical Research Council (2013).
Birds and husbandry
The experimental housing was a tunnel-ventilated shed with 48 individual pens allocated to four blocks of 12 pens in each block. There were 12 treatments, with these randomly allocated to one pen in each of the four blocks, giving four replicates per treatment. Pens floors were covered with wood shavings which were turned and replaced when needed, to maintain a good friable condition.
Cherry Valley Farms Ltd Pekin ducks were reared as mixed sex groups. Ducks were bred by PEPE’s Pty Ltd (Windsor, NSW, Australia), with the eggs being incubated at their commercial hatchery. At hatch, day-old ducklings (N = 1152) were vent sexed and transported to the experimental facility at The University of Sydney, Camden, New South Wales (NSW), Australia. On arrival, 24 ducklings (12 males and 12 females) were randomly allocated to each of the 48 floor pens. On Day 14 (D14) of age, all birds were fitted with coloured plastic numbered leg bands. At the end of Week 4, the number of birds in each pen was reduced to 20 (10 males and 10 females). Birds had access to feed and water ad libitum. The shed was lit with fluorescent lighting that was maintained for the full 24 h of each day.
Supplementary heat was provided with overhead lamps with a temperature of 35°C, as measured directly beneath the brooder lamp, at D1. The temperature was gradually reduced to 28°C by D7, to 26°C by D14 and then 24°C by D21 of age.
Diets
The diets were formulated and supplied by Inghams Pty Ltd, Berrima, NSW, Australia. For reasons of ‘commercial-in-confidence’, details of the ingredient amounts used in the formulations of these diets were not available from the manufacturer. The starter diet was fed from D1 to D14 in crumble form and was formulated to provide 12.52 MJ metabolisable energy (ME)/kg, 22.0% protein, 0.17% sodium, 0.70% potassium and 0.19% chloride. Two grower diets were fed from D15 to D41 and formulated with the same energy content of 12.62 MJ ME/kg, 18.3% protein, 4.2% fat and 0.8% calcium. A low DEB (L-DEB) grower diet was formulated to contain 0.15% sodium, 0.62% potassium and 0.18% chloride. The high DEB (H-DEB) grower diet was formulated to contain 0.35% sodium, 0.65% potassium and 0.30% chloride.
To determine the actual DEB of the grower diets, they were analysed for mineral concentrations by the UNSW Analytical Centre, University of New South Wales, Kensington, NSW, Australia. Ground feed samples were extracted with water and analysed by ion chromatography for chloride concentration. Samples were digested using nitric acid and hydrogen peroxide and analysed for sodium and potassium by inductively coupled plasma–atomic emission spectrometry (ICP–OES). The calculated and analytically determined DEB are given in Table 1. The actual DEB was 187 mEq/kg for the starter diet, and 160 and 209 mEq/kg for the L-DEB and H-DEB diets respectively.
(a) | ||||
---|---|---|---|---|
Component | Starter | Grower L-DEB | Grower H-DEB | |
ME (MJ/kg) | 12.59 | 12.62 | 12.62 | |
Protein (%) | 22.0 | 18.3 | 18.30 | |
Fat (%) | – | 4.2 | 4.20 | |
Calcium (%) | – | 0.80 | 0.80 |
(b) | ||||
---|---|---|---|---|
Component | Actual analytical DEB | |||
Na (%) | 0.154 | 0.140 | 0.355 | |
K (%) | 0.669 | 0.575 | 0.595 | |
Cl (%) | 0.184 | 0.169 | 0.317 | |
DEB (mEq/kg) | 187 | 160 | 217 |
(c) | ||||||
---|---|---|---|---|---|---|
Betaine concentration in feed (g/kg) and water supplements (g/L) | ||||||
Control (g/kg) | BF (g/kg) | E50 | E100 | E150 | E100 + 36 h | |
0 | 1.088 | 0.4 | 0.4 | 0.4 | 0.4 |
Electrolyte supplements
The 100% electrolyte supplement (E100) contained sodium chloride (157 g), sodium bicarbonate (171 g) and potassium chloride (88.4 g) dissolved in 100 L of tap water. The 50% (E50) electrolyte supplement was formulated by adding only half the quantity of salts used in E100 to 100 L of water, while the 150% supplement was formulated by adding 1.5 times the quantity of salts used in the E100 to 100 L of water. Betaine was added to all electrolyte supplements at 40 g/100 L, while the control treatments received tap water only.
Betaine in feed
Betaine (Treatments 5 and 11, see below) was added to the grower pelleted diet by dissolving 284 g of betaine (Betafin®) in 1.9 L of warm tap water and then spraying onto 259 kg of the control mash grower feeds (both L-DEB and H-DEB) while gently being turned in a paddle mixer. The mixture was then steam pelleted. This gave a betaine concentration of 1.088 g/kg. The water content of these grower diets was increased by 0.73%.
Treatments
During D15–41 of age, all allocated groups were fed a grower diet with a L-DEB and or a H-DEB. During D36–41, the control treatments received tap water only. Three electrolytes were added to the tap water at concentrations of E50, E100 and E150, as identified above. These electrolyte treatments were provided over D36–41 of age. A further electrolyte treatment (E100 + 36 h) with the 100% (E100) electrolyte concentration was supplied during only the 36 h (D40–41) prior to bird pickup and transport for processing. It is regular practice to add betaine to duck grower feeds and so the two betaine feed treatments included over D15–35 were continued in Week 6 (D36–41) and birds on these treatments received tap water only.
The 12 treatments were as follows:
L-DEB + water only (Control-L)
L-DEB + E50 + betaine on D36–41 (E50-L)
L-DEB + E100 + betaine on D36–41 (E100-L)
L-DEB + E150 + betaine on D36–41 (E150-L)
L-DEB + betaine in feed + water only on D29–41 (BF-L)
L-DEB + E100-36 h + betaine on D40–41 (E100 + 36 h-L)
H-DEB + water only (Control-H)
H-DEB + E50 + betaine on D36–41 (E50-H)
H-DEB + E100 + betaine on D36–41 (E100-H)
H-DEB + 150 + betaine on D36–41 (E150-H)
H-DEB + betaine in grower feed + water only on D29–41 (BF-H)
H-DEB + E100-36 h + betaine on D40–41 (E100 + 36 h-H)
High-temperature thermal challenge
Over D1–35, birds in all allocated groups were exposed to the same conditions. On D36–41, all birds were exposed to an ambient temperature of 28–32°C from 08:30 hours to 17:30 hours and then to 22–24°C from 17:30 hours to 08:30 hours each day. A thermal humidity index (THI) was determined on the basis of the formula reported by Moraes et al. (2008), where THI (%) = 0.8Tdb + RH (Tdb – 14.3)/100 + 46.3 where Tdb is the dry bulb temperature and RH the relative humidity. Birds were categorised as being ‘in absolute comfort’ (THI ≤ 72%), or as experiencing ‘light discomfort’ (THI = 72–76%), ‘discomfort’ (THI = 77–80%), or ‘severe discomfort’ (THI = 81–84%), and when ≥85%, as experiencing ‘life-threatening conditions’.
Performance measures
Individual liveweights (LW) were recorded on D14, D28, D35 and D41 of age by using Wedderburn WS209B digital platform scales. At these intervening times, FI was determined as the difference between weights of feed provided and the weights of feed refused. From D15 to 41, water consumption was recorded daily.
Carcass measurements
On D41 of age, one male and one female duck were removed from each pen. The total of eight birds (four males and four females) per supplementary treatment were individually weighed, euthanised and the total breast muscle was removed and weighed. The remaining birds were taken off feed at 22:00 hours (D41) and then at 04:00 hours on D42 loaded and transported to a commercial processing plant. While in lairage and prior to processing, birds were individually weighed and then processed at 10:00 hours. After the birds were removed from the water chiller and allowed to drip dry, the eviscerated carcass weight was recorded.
Statistical analyses
Statistical analysis of performance was conducted using the REML linear mixed-model function of Genstat® 17.1 edition (url: vsni.co.uk/software/genstat). Data were first assessed for equality of variance by using residual plots. When the equality of variance could be improved using a loge transformation, data were transformed. The fixed model included the effects of treatment, DEB, sex and day and the random model included the effects of block, pen number and bird tag number. Initially, all two-way interactions between fixed effects were included in the model. Any non-significant interactions were removed from the model. The least significant difference (l.s.d.) was used to make pairwise comparisons of means.
There were different time periods at which the performance of the ducks was analysed. In the first 14 days, all ducks were under the same management and the analysis was undertaken to check that there were no differences among the allocated groups. Over D15–35 of age, the birds were either on the grower diet with a low DEB or the diet with a high DEB, while groups allocated to betaine in feed received this during the full grower period (D15–41 of age). On D36–41 of age, the treatments were supplied while the cyclic temperature was applied. The effects of treatment and DEB on the breast muscle yields were analysed by ANOVA (Statview Ed., 3 (SAS publishing; url: sas.com/en/books/publish.html) and when effects were significant, individual comparisons were performed using the Tukey–Kramer test.
Results
Temperature and humidity
The average daily temperature and humidity are shown in Fig. 1. The average daily temperature during the 9 h of high-temperature challenge was 28.8 ± 0.1°C (range 25.1–31.0°C) and humidity 81.7 ± 1.2%. For the remainder of the day, the temperature averaged 23.7 ± 0.1°C and humidity 87.7 ± 0.8%. On the basis of the average temperatures and RH, the average THI during the 9 h of the high-temperature period was 81.6% and during the remainder of the day it was 74.7%, but this included the transition period (from 17:30 hours to 19:30 hours) when the shed was returning to thermo-neutral conditions and the THI averaged 78.1%. During the high-temperature challenge, on the basis of the criteria of Moraes et al. (2008), birds would have experienced ‘severe discomfort’.
Betaine intake
Over D15–35, the betaine intake from feed was 0.184 ± 0.002 g/day for birds on both the L-DEB and H-DEB diets. Over D36–41, the betaine intake from feed was 0.188 ± 0.005 g/day for birds on the L-DEB diet and 0.193 ± 0.005 g/day for birds on the H-DEB diet.
Over D36–41, the electrolyte supplement concentration (P < 0.001) and diet DEB (P = 0.043) affected betaine intake, with no interaction between these (P = 0.813). Birds supplemented with E150 had the highest betaine intake (4.04 ± 0.07 g/day), followed by birds on E100 (3.78 ± 0.07 g/day), E50 (3.57 ± 0.07 g/day) and E100 + 36 h (3.30 ± 0.07 g/day), with all comparisons being significantly (P < 0.05) different. Birds on the L-DEB diet consumed 3.60 g/day of betaine and those on H-DEB consumed 3.75 g/day.
Liveweight (LW)
The LW and LWG performance measures are given in Table 2. At D14, all groups allocated to the proposed treatment groups had a similar LW (P = 0.466), while males (714 ± 7 g) were heavier than females (693 ± 7 g) (P < 0.001). Over D15–35, LW was not different among the allocated treatments groups (P = 0.852). Over D15–35, the DEB had no effect on LW, with birds on the L-DEB having LW of 2402 ± 29 g and those on the H-DEB diet had LW of 2409 ± 29 g. Over D15–35, compared with the control diet (2419 ± 33 g), adding betaine to the grower diet (2411 ± 26 g) had no effect on LW. Sex had a significant effect on LW at D35 (P < 0.05), with LW being higher in males (2463 ± 29 g) than in females (2350 ± 68 g). The sex effects were independent of the DEB and whether betaine was added to the feed or not.
Week | DEB | Treatment | s.e.m. | P-value | ||||||
---|---|---|---|---|---|---|---|---|---|---|
Water | BF | E50 | E100 | E150 | E100 + 36 h | |||||
Liveweight (g) | ||||||||||
1 + 2 A | ̶ | 711 | 709 | 704 | 700 | 708 | 705 | 10 | T: 0.466 | |
3–5 B | Low | 2429 | 2421 | 2418 | 2398 | 2418 | 2383 | 35.0 | T: 0.852 D: 0.705 T × D: 0.081 | |
High | 2415 | 2398 | 2400 | 2407 | 2417 | 2459 | ||||
6 | Low | 2703c | 2739abc | 2711Bbc | 2767ab | 2788a | 2747abc | 41.0 | T: 0.148 D: 0.133 T × D: 0.047 | |
High | 2760ab | 2740b | 2774Aab | 2723b | 2764ab | 2802a | ||||
Liveweight gain (g/day) | ||||||||||
1 + 2 A | ̶ | 47.2 | 47.1 | 46.3 | 46.2 | 46.4 | 46.3 | 0.7 | T: 0.531 | |
3–5 B | Low | 81.9 | 80.8 | 82.1 | 80.3 | 81.3 | 79.6 | 1.63 | T: 0.991 D: 0.417 T × D: 0.421 | |
High | 80.9 | 80.8 | 80.5 | 82.1 | 82.1 | 83.4 | ||||
6 | Low | 45.7Bc | 53.7Bb | 51.3Bb | 61.0Aa | 64.1Aa | 62.3Aa | 1.66 | T: <0.001 D: 0.003 T × D: <0.001 | |
High | 58.4Ab | 59.1Ab | 64.2Aa | 56.0Bb | 57.9Bb | 60.2Aab |
Values with different lower-case letters in the same row are different (at P = 0.05).
Values with different upper-case letters in the same column for different weeks are different (at P = 0.05).
P-values: T, treatment; D, diet (low or high); T × D, treatment × diet.
Over D36–41, there was an interaction between treatment and diet DEB (P = 0.047). Birds supplemented with E150 while on the L-DEB diet had a significantly (P < 0.05) higher LW than did those supplemented with E50 or water alone, but it was equivalent to the LW in other treatments. Birds on E100 had a higher LW than did those on water alone (P < 0.05). Birds on the H-DEB diet and supplied with E100 + 36 h had a higher LW than did those on E100 and BF (P < 0.05), but the LW was equivalent to that of birds in other supplement treatments. Birds on the H-DEB diet (2760 ± 8 g) and those fed the L-DEB (2738 ± 8 g) had similar LWs. Over D36–41, males (2864 ± 24 g) were heavier (P < 0.05) than females (2651 ± 24 g).
Liveweight gain (LWG)
Over D1–14, the birds allocated to the treatment groups had similar LWGs (P = 0.531). Over D15–35, average LWG for birds on the L-DEB was 81.0 ± 1.0 g/day and that of the birds on the H-DEB diet was 81.6 ± 1.0 g/day (P = 0.417).
During D36–41, when the supplements were provided, and birds were exposed to the elevated temperature, there were both supplement (P < 0.001) and diet DEB (P = 0.003) effects on LWG and an interaction between these (P < 0.001). On the L-DEB diet, birds supplied with E100, E150 and E100 + 36 h had higher LWGs than did birds on all other supplements (all, P < 0.05). Also, birds on this same diet and supplemented with water alone had a lower LWG than did those on E50 or BF (P < 0.05). Birds fed the H-DEB diet and supplied with E50 had a higher LWG than those in the other treatments, except those supplied with E100 + 36 h (all, P < 0.05).
Birds on the L-DEB and supplied with water, BF and E50 had lower LWGs than did those on the same supplements and fed the H-DEB diets (all, P < 0.05). Birds on the H-DEB and supplied with E100 or E150 had a lower LWG than did those on the same supplements and fed the L-DEB diets (all, P < 0.05). Birds supplemented with E100 + 36 h had similar LWGs on both DEB diets.
Feed intake
During all weeks, there were no differences in average daily FI (Table 3).
Week | DEB | Treatment | s.e.m. | P-value | ||||||
---|---|---|---|---|---|---|---|---|---|---|
Water | BF | E50 | E100 | E150 | E100 + 36 h | |||||
Feed intake (g/day) | ||||||||||
1 + 2 A | ‒ | 39.5 | 39.4 | 39.1 | 38.9 | 38.8 | 39.0 | 0.6 | T: 0.875 | |
3–5 B | Low | 166 | 169 | 168 | 164 | 166 | 163 | 3 | T: 0.678 D: 0.126 T × D: 0.376 | |
High | 167 | 168 | 167 | 169 | 168 | 172 | ||||
6 | Low | 168 | 172 | 171 | 173 | 175 | 167 | 5 | T: 0.933 D: 0.095 T × D: 0.439 | |
High | 174 | 177 | 174 | 175 | 171 | 178 | ||||
Water intake (mL/day) | ||||||||||
1 + 2 A | ‒ | 142 | 143 | 141 | 140 | 143 | 140 | 3 | T: 0.888 | |
3–5 B | Low | 542Bab | 552ab | 569a | 539Bab | 523Bb | 546Bab | 11 | T: 0.832 D: <0.001 T × D: 0.049 | |
High | 600A | 581 | 583 | 606A | 606A | 602A | ||||
6 | Low | 832 | 889 | 872 | 916 | 989 | 872 | 36 | T: <0.001 D: 0.042 T × D: 0.723 | |
High | 879 | 855 | 911 | 975 | 1033 | 838 | ||||
Feed to gain (FCR) | ||||||||||
1 + 2 A | ‒ | 1.26 | 1.25 | 1.27 | 1.26 | 1.26 | 1.27 | 0.11 | T: 0.706 | |
3–5 B | Low | 2.03 | 2.08 | 2.05 | 2.03 | 2.05 | 2.05 | 0.21 | T: 0.098 D: 0.239 T x D: 0.840 | |
High | 2.07 | 2.12 | 2.08 | 2.04 | 2.05 | 2.05 | ||||
6 | Low | 4.09 | 3.57 | 3.70 | 3.08 | 3.08 | 3.06 | 0.23 | T: 0.194 D: 0.409 T × D: 0.074 | |
High | 3.35 | 3.15 | 3.09 | 3.57 | 3.26 | 3.26 | ||||
Water to feed | ||||||||||
1 + 2 A | ‒ | 3.60 | 3.64 | 3.61 | 3.60 | 3.70 | 3.59 | 0.07 | T: 0.810 | |
3–5 B | Low | 3.26 | 3.27 | 3.38 | 3.30 | 3.16 | 3.34 | 0.08 | T: 0.549 D: <0.001 T × D: 0.072 | |
High | 3.59 | 3.44 | 3.49 | 3.64 | 3.59 | 3.40 | ||||
6 | Low | 4.95 | 5.11 | 5.29 | 5.29 | 5.64 | 4.95 | 0.17 | T: <0.001 D: 0.355 T × D: 0.269 | |
High | 5.05 | 4.91 | 5.29 | 5.59 | 6.07 | 4.73 |
Values with different lower-case letters in the same row are different (at P = 0.05).
Values with different upper-case letters in the same column for different weeks are different (at P = 0.05).
P-Values: T, treatment; D, diet (Low or high); T × D, treatment × diet.
Feed to gain ratio
Over D15–35, there was no DEB effect on feed to gain ratio (P = 0.239). Over D36–41, there was no effect of supplement (P = 0.194) on feed to gain ratio, while there was a trend for there to be a supplement × diet DEB effect (P = 0.074). Birds on the control water supplement and the L-DEB diet tended to have a poorer feed to gain ratio than did those on the control water supplement and the H-DEB diet.
Water intake
Over D15–35, diet DEB influenced water intake significantly (P < 0.001), with birds on the L-DEB diet consuming 545 ± 5 mL/day and those on the H-DEB diet consuming 593 ± 7 mL/day. All allocated treatment groups on the L-DEB diet had a lower water intake than did those of the H-DEB diet, except birds allocated to the E50 treatment applied on D36–41. Betaine in feed had no effect on water intake.
On D36–41, both diet (P = 0.042) and supplement (P < 0.001) had effects on water intake. Birds on the H-DEB diet (918 ± 11 mL/day) consumed more water than did those and on the L-DEB (887 ± 11 g/day). Water intake was higher for birds supplied with E150 (1011 ± 18 g/day) than for those in other treatments (P < 0.05), except those with E100 (945 ± 18 g/day), while intake for birds on this treatment was higher than those on other treatments except E50 (P < 0.05). Birds on E50 supplement (891 ± 18 g/day) had a higher water intake (P < 0.05) than did those on E100 + 36 h (833 ± 18 g/day), but the intake was equivalent to those on water alone and on BF.
Water to feed ratio
Over D15–35, diet DEB had effects on water to feed ratio (P < 0.001). The ratio was higher for birds on the H-DEB diet (3.53 ± 0.05) than it was for those fed the L-DEB diet (3.29 ± 0.05) (P < 0.05). Betaine in feed had no effect on water to feed ratio.
Over D36–41, the water to feed ratio was higher for birds supplemented with E150 (5.85 ± 0.13) than with other supplements (P < 0.05), and then higher for those supplemented with E100 (5.44 ± 0.13) than for birds on E100 + 36 h (4.84 ± 0.13 mL/day) (P < 0.05), but equivalent to those of birds on E50 (5.19 ± 0.13 mL/day), BF (5.02 ± 0.13 mL/day) and on water alone (5.0 ± 0.13 mL/day), with these three latter supplements having similar water to feed ratios.
Total electrolyte intake during the high temperature (D36–41)
The sodium, potassium, and chloride electrolyte intakes over D36–41 from the diet and supplements are given in Table 3. The supplement provided (P < 0.001) and diet DEB (P < 0.001) affected electrolyte intakes. The monovalent cation–anion difference (CAD) as milliequivalents (mEq) was calculated as follows (Moore et al. 2000):
The total electrolyte intake for birds on the L-DEB and H-DEB diets are given in Table 4. The birds on the L-DEB diet had a CAD of 38.3 ± 0.6 mEq/day and those on the H-DEB diet a CAD of 47.8 ± 0.6 mEq/day. Birds on the E150 supplement (58.8 ± 0.8 mEq/day) had the highest CAD, followed in order by E100 (48.7 ± 0.8 mEq/day), E100 + 36 h (48.7 ± 0.8 mEq/day) and E50 (39.7 ± 0.8 mEq/day), all being different from CAD of birds on BF (32.3 ± 0.8 mEq/day) and water alone (31.7 ± 0.8 mEq/day), which had equivalent values of CAD (all, P < 0.05).
Treatment | Monovalent ion | CAD | ||||||||||
---|---|---|---|---|---|---|---|---|---|---|---|---|
Diet | Na+ | K+ | Cl− | Na+ | K+ | Cl− | Na+ | K+ | Cl− | |||
mEq | ||||||||||||
Diet contribution | Water contribution | Sum (diet + water) | ||||||||||
Control | L-DEB | 10.2 | 24.7 | 8.0 | 0 | 0 | 0 | 10.2 | 24.7 | 8.0 | 27.0 | |
BF | L-DEB | 10.5 | 25.3 | 8.2 | 0 | 0 | 0 | 10.5 | 25.3 | 8.2 | 27.6 | |
E50 | L-DEB | 10.4 | 25.1 | 8.1 | 20.6 | 5.2 | 18.0 | 31.0 | 30.3 | 26.1 | 35.1 | |
E100 | L-DEB | 10.5 | 25.5 | 8.2 | 43.2 | 10.9 | 37.8 | 53.8 | 36.3 | 46.1 | 44.1 | |
E150 | L-DEB | 10.7 | 25.8 | 8.3 | 70.1 | 17.6 | 61.3 | 80.7 | 43.4 | 69.6 | 54.5 | |
E100 + 36 h (HT) | L-DEB | 10.2 | 24.6 | 7.9 | 39.1 | 9.8 | 34.2 | 49.2 | 34.4 | 42.1 | 41.5 | |
Control | H-DEB | 25.3 | 26.5 | 15.5 | 0 | 0 | 0 | 10.2 | 24.7 | 8.0 | 36.3 | |
BF | H-DEB | 25.8 | 27.0 | 15.8 | 0 | 0 | 0 | 10.5 | 25.3 | 8.2 | 37.0 | |
E50 | H-DEB | 25.3 | 26.4 | 15.5 | 21.5 | 5.2 | 18.0 | 31.0 | 30.3 | 26.1 | 44.3 | |
E100 | H-DEB | 25.4 | 26.6 | 15.6 | 46.0 | 10.9 | 37.8 | 53.8 | 36.3 | 46.1 | 53.8 | |
E150 | H-DEB | 24.8 | 26.0 | 15.2 | 73.1 | 17.6 | 61.3 | 80.7 | 43.4 | 69.6 | 63.1 | |
E100 + 36 h (HT) | H-DEB | 25.9 | 27.1 | 15.9 | 39.6 | 9.8 | 34.2 | 49.2 | 34.4 | 42.1 | 52.1 |
Pekin ducks were fed a grower diet with a low DEB or with a high DEB (Days 15–35) and the same diets (Days 36–41) and exposed to high ambient temperature for 9 h/day and provided with water alone (water), betaine in the feed (BF); electrolytes at 50% (E50), 100% (E100) and 150% (E150) and betaine in water, or electrolytes at 100% for 36 h (E100 + 36 h) before processing at Day 42 of age.
L-DEB, low-DEB diet; H-DEB, high-DEB diet; Control, water alone; BF, betaine in feed; E50, E100 and E150, electrolyte solutions + betaine in water; E100 + 36 h, electrolyte solution + betaine in water for 36 h before processing.
Treatments repeated for birds in both L-DEB and H-DEB diets.
The significant (P = 0.019) relationship between CAD and LWG is shown in Fig. 2. The highest predicted LWG (61.7 g/day) was achieved at an CAD of 48, with a range of 44–54 supporting LWG of between 61.0 and 61.7 g/day.
The relationship between CAD (cation–anion difference, mEq) and LWG (g/day) for Pekin Ducks exposed to high ambient temperature for 9 h/day at 36–41 days of age and provided with either water, betaine in the feed (BF), one of three water electrolyte supplements (E50, E100, E150) and betaine for all days, or a water electrolyte (E100 + 36 h) and betaine supplement for 36 h before processing on Day 42 of age.
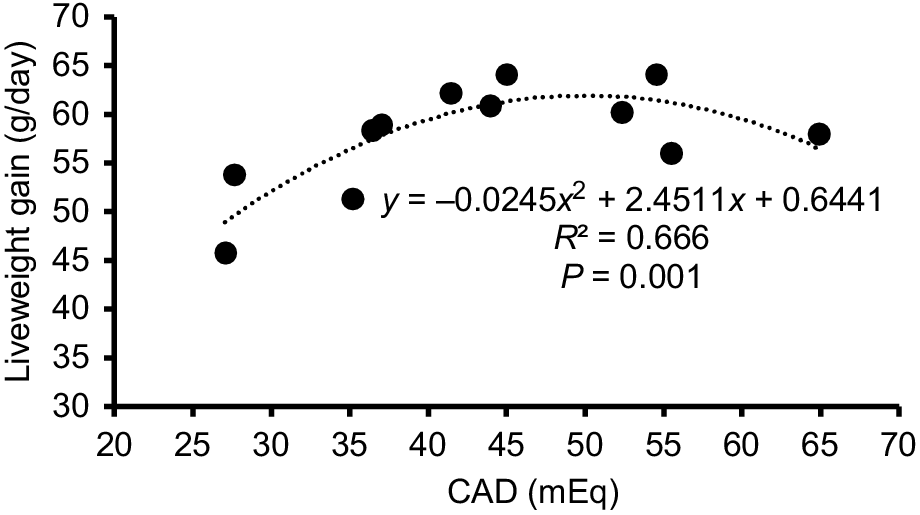
Treatment effects on transport, processing, breast muscle weights and yields
The effects of treatments on processing and breast weights are given in Table 4. The treatments had no effect on the pre-processing weight (P = 0.644) or the %weight loss (P = 0.969) from the final on-farm weight until processing. The DEB had no effect (P = 0.526) on the %weight loss, with it being 5.11 ± 0.5% for birds on the L-DEB diet and 5.33 ± 0.5% for birds on the H-DEB diet. Treatment had no effect on the eviscerated dry processed carcass weight (P = 0.610). The eviscerated processed carcass weight was not affected by DEB (P = 0.610), with it being 1959 ± 12 g for birds on the L-DEB diet and 1964 ± 12 g for birds on the H-DEB diet.
The processing weights and breast muscle yeilds are given in Table 5. Treatment (P = 0.874), DEB (P = 0.762) or sex (P = 0.470) had no effect on breast weight. The breast weight of birds on the L-DEB diet was 228 ± 9 g and that of birds on H-DEB was 226 ± 9 g. The breast weight of females was 225 ± 9 g and of males 229 ± 9 g. Treatment (P = 0.413) and diet (P = 0.485) had no effect on the %breast weight of final on-farm weight. The breast percentage of birds on the L-DEB was 8.18 ± 0.19% and of those on the H-DEB 8.06 ± 0.19%. The breast percentage of females at 8.39 ± 0.18% was higher than that of males at 7.84 ± 0.18% (P = 0.003). Treatment (P = 0.891), diet (P = 0.369) or sex (P = 0.672) did not have any effect on feed weight to breast weight ratio. The feed weight to breast weight ratio for birds on the L-DEB diet was 24.3 ± 0.9 and for those on the H-DEB diet it was 24.9 ± 0.9. The feed weight to breast weight ratio of females was 24.7 ± 0.8 and for males it was 24.4 ± 0.8.
Item | Supplementary treatment | s.e.m. | P-value | ||||||
---|---|---|---|---|---|---|---|---|---|
Control | BF | E50 | E100 | E150 | E100 + 36 h | ||||
On-farm weight (g) | 2729 | 2741 | 2744 | 2749 | 2795 | 2771 | 19 | T: 0.637 | |
Pre-processing weight A (g) | 2588 | 2592 | 2594 | 2609 | 2640 | 2631 | 27 | T: 0.644 | |
Processed weight (g) | 1957 | 1953 | 1953 | 1953 | 1973 | 1981 | 20.2 | T: 0.610 | |
Actual weight loss (g) B | 141 | 149 | 150 | 140 | 155 | 140 | 17 | T: 0.983 | |
Total weight loss (%) C | 5.16 | 5.43 | 5.46 | 5.08 | 5.16 | 5.04 | 0.06 | T: 0.969 | |
Breast muscle weight (g) | 225 | 227 | 231 | 228 | 220 | 231 | 11 | T: 0.874 | |
Breast weight to final on-farm weight (%) | 8.17 | 8.23 | 8.17 | 8.28 | 7.64 | 8.25 | 0.27 | T: 0.413 | |
Feed weight to breast weight | 24.7 | 24.9 | 24.1 | 24.5 | 25.3 | 24.1 | 1.11 | T: 0.891 |
Discussion
High temperature reduces performance of meat chickens and, depending on severity, affects LWG, FI and feed efficiency (Lara and Rostagno 2013; Liu et al. 2020). Similar temperature challenges affect duck performance (Sun et al. 2019; Downing 2022), although there is much less research evidence. On the basis of the THI categories of Moraes et al. (2008), birds in the current study were likely to be suffering ‘severe discomfort’ and the reduced LWG during the high-temperature challenge over D36–41 would support this degree of stress. Previous performance of the same duck strain under thermoneutral conditions, raised as mixed sexes and in the same housing as used in the current study, was 73.7 g/day (84.9 g/day for males, 62.9 g/day for females; Downing 2010). This suggests that the high-temperature challenge depressed LWG in the control L-DEB-fed birds by ~38% and in control H-DEB-fed birds by 21%. At 20°C, LWG of Pekin ducks was 85.6 ± 2.5 g/day and at 30°C it was 59.4 ± 7.5 g/day, and this was a 31% reduction (Sun et al. 2019). Depending on the DEB diet and the shed temperature, it was comparable to LWG losses observed in the current study.
The optimal DEB varies according to a range of factors, but high temperature and the duration have a major influence (Ahmad and Sarwar 2006; Mushtaq et al. 2007, 2013). For meat chickens, under thermo-neutral conditions, a DEB of 200–270 mEq/kg has often been quoted as suitable (Borges et al. 2004; Szabó et al. 2011; Arantes et al. 2013; Popoola et al. 2020b). In the present study, over D15–35, under thermo-neutral conditions, ducks fed the diets with DEB of 160 and 217 mEq/kg had similar performance. For ducks, grower diets with a lower DEB could be more ideal because wet litter is a major management concern for duck producers (Karcher et al. 2013; Mushtaq et al. 2013). Borges et al. (2003) found that litter moisture increased linearly with DEB, as did also Ahmad et al. (2009). At DEB of 375 mEq/kg, excreta moisture content has been shown to be 81.2%, compared with 73.1% when the DEB is 150 mEq/kg (Ravindran et al. 2008). It is possible that the salts used, sodium especially, may have more influence than the absolute DEB value on litter moisture content (Mushtaq et al. 2013).
The control birds on the L-DEB diet had a 28% lower LWG over D36–41 than did control birds on the H-DEB diet, suggesting that the additional need for electrolytes under high temperature could, at least in part, be met by a diet with a higher DEB. During persistent periods of high temperature, meat birds pant and the physiological changes increase the loss of electrolyte ions, which causes acid–base imbalance (Borges et al. 2007). An obvious strategy to replace this loss would be to increase DEB. However, often the research supporting this concept has been less than convincing. Under tropical conditions (temperature ranged 26.1–37.5°C), bird performance was poorer when the DEB was 0 and 350 mEq/kg than when it was 50, and 250 mEq/kg (Ahmad et al. 2009). At a high temperature, a range of 150–250 mEq/kg was found suitable by Ahmad et al. (2009). At 29–34°C, feeding diets with DEB of 120, 220 and 320 mEq/kg to ‘finisher’ meat birds has been shown to support equivalent growth performance (Saki et al. 2016). Under thermo-neutral conditions, a DEB of 240 mEq/kg has been shown to support better performance than when the DEB is 40 and 340 mEq/kg, but the DEB had no effect when birds experienced high ambient temperature (Borges et al. 2003).
During the high-temperature challenge, for birds on the L-DEB diet, all electrolyte supplements increased LWG compared with the control birds. The highest LWG was achieved by suppling E100, E150 and E100 + 36 h supplements. For birds on the H-DEB diet, except for the E50 supplement, all other supplements supported LWG being equivalent to that of the control birds. On the E50 supplement, LWG was highest but equivalent to that of birds on the E100 + 36 h supplement. Betaine increased the LWG for birds on the L-DEB diet but had no effect on the LWG of birds fed the H-DEB diet. Together, these observations suggested that the effect of the supplementary treatments on LWG might be associated with the total electrolyte intake. This prompted an investigation of the relationship between LWG and the CAD, with CAD being the difference between the intake of cations (Na+ + K+) and the Cl− ion concentrations. This relationship was found to be quadratic with the predicated maximum LWG being achieved when the CAD ranged from 44 to 55 mEq/day. The maximum LWG for birds on the L-DEB was observed when supplemented with E150 and a CAD of 54.5, while on the H-DEB diet, the maximum LWG was observed when birds were supplemented with E50 and a CAD of 44.3.
On the L-DEB diet, the betaine feed supplement supported a higher LWG than for the control birds, while the CAD was not different between these treatments (27.6 vs 27.0 mEq/day). However, on the H-DEB diet, the betaine feed supplement had no effect on LWG compared with the control treatment, with these treatments again having a similar CAD intake (37.0 vs 36.3 mEq/day), but the CAD values were ~10 mEq/day higher than for the same treatments on the L-DEB diet. The betaine effect may depend on the CAD intake, with a benefit when it is low and minimal benefit when high.
While exposure to high temperature increases water intake (Donkoh 1989; Lott 1991; Sayed and Downing 2011; Mushtaq et al. 2013), there is also an effect of electrolyte supplement. Birds supplemented with E100 and E150 consumed ~10% and 18% more water respectively, than did the control birds. The effect of electrolyte supplement is well supported by the differences recorded for birds supplemented with E100 for 36 h (E100 + 36) prior to farm pick-up for processing. In the first 4.5 days of high temperature, the average water intake was 816 mL/day and in the 36 h of supplementation prior to farm pick-up for processing it was 1106 mL/day.
The average LWG was determined over 6 days of high temperature. This equated to a total of 370 g and 368 g for the E100 + 36 h and E100 treatments respectively. The limited supplementary period of treatment E100 + 36 h could mean that total LWG of birds on the E100 + 36 h was related to increased hydration rather than greater tissue mass. During high temperature, the water intake and its retention were higher in meat chickens given water electrolyte supplements than those on tap water alone (Sayed and Downing 2011). The effect of electrolytes on LWG during high temperature might be limited and not continuous. During exposure to high temperature, electrolyte supplementation over D32–42 of age has been found to support higher LWG over the first 5 days (D32–36), but no further improvement in LWG was observed over the final 6 days (D37–42) of supplementation (Sayed and Downing 2011).
The higher water intake in birds provided electrolytes could, in some manner, reduce the stress birds experience during high temperature. The heterophil to lymphocyte ratio (H:L) has been identified as a measure of stress in poultry (Zulkifli and Siegel 1995; Puvadolpirod and Thaxton 2000). HS in meat chickens increases the H:L ratio (Yalçin et al. 2003; Borges et al. 2004; Gu et al. 2012; Barreto Sánchez et al. 2022). During high temperature, meat chickens supplied with electrolyte supplements have been shown to have a lower H:L ratio, suggesting that they experience less stress (Sayed and Downing 2011). The higher water intake in birds provided electrolytes could, in some manner, reduce stress. High temperature exposure decreases whole-blood viscosity (Zhou et al. 1998), which is likely to alter the peripheral resistance and reduce blood circulation to heat-exchange skin surfaces (Zhou et al. 1999a). Higher water intake increases blood and plasma volume and reduces whole-blood viscosity (Zhou et al. 1999a, 1999b). Under high temperature, an increased water intake acts as a heat sink and is associated with higher evaporative heat load and reduced respiration rate, all supporting higher respiratory efficiency (Belay and Teeter 1993). These same researchers also reported that supplementing water with 0.75% KCl increased water intake and the evaporative heat load and apparent respiratory efficiency. In the current study, the increased water intake could increase blood viscosity, but there is likely a limit to the benefit, depending on the temperature challenge. Whole-blood viscosity has been shown to increase as the temperature increases from 20°C to 25°C, with no further effect as the temperature increases to 30°C (Zhou et al. 1999b). Electrolyte supplementation will assist meat birds cope with HS at moderately high temperature effects (<30°C), but be less beneficial at a severely high temperature (>30°C); however, realistically, the severity depends not only on the absolute temperature but also the duration.
Betaine improves growth performance by regulating the osmotic pressure in animals under HS to prevent dehydration, maintain water content in cells, save energy and enhance nutrient bioavailability (Ratriyanto and Mosenthin 2018). Depending on the temperature conditions, betaine added to feed or water supports better performance in meat chickens and ducks (Wang et al. 2004; Zulkifli et al. 2004). In the current study, betaine had no effect on LWG over D15–35 of age under thermo-neutral conditions. During the high-temperature treatment period (D36–41), betaine in the feed increased LWG by 17.5% for birds on the L-DEB, with no effect when birds were fed the H-DEB diet, with no effects on FI or feed to gain. Under suitable temperature conditions, meat ducks supplemented with 0.5% betaine in feed had better performance (Wang et al. 2004). When meat ducks, 21–42 days of age, while exposed to cyclic high temperature (33–42°C for 6 h/day; 70% RH), were provided feed containing betaine at 0.7, 1.0 or 1.3 g/kg feed, betaine supported a 46–49% improvement in LWG, a 6.7–7.3% higher feed intake and a 9% better feed to gain ratio, with no differences among the different inclusion rates (Park and Kim 2017). Under these temperature conditions, betaine at 1.2 g/kg feed improved LWG by 4.3% and FCR by 15% (Park and Park 2017). Under the same high-temperature conditions, water supplementation with betaine at 0.4, 0.8 or 1.2 g/L on Days 22–42 of age increased duck FI by 4.8–5.5% and improved LWG by 9.8–15.7%, with the highest betaine dosage being superior to the lower doses (Shin et al. 2019). The betaine supplements increased blood electrolyte concentrations of sodium (Na+), potassium (K+), and chloride (Cl−) and this might be, in part, the way betaine supports duck performance under severe high-temperature stress (Shin et al. 2019). This might account for the differences seen in the current study where feed betaine improved LWG when birds were fed the L-DEB diet but not the H-DEB. The H-DEB diet would provide higher electrolyte intake because there were no differences in FI; however, there is a need to determine whether this increased blood electrolyte concentrations. The much higher response to betaine in the studies discussed above than in the current study is likely to be related to the severity of the stress imposed. The current results suggest that even under a moderate high-temperature stress, betaine in feed can be of benefit.
Neither the electrolyte supplement nor DEB had any effect on LW loss during transport and lairage, or on processed carcass weight. The supplements had no effect on the breast meat yield. High-temperature exposure can decrease breast yield in meat chickens (Smith 1993; Rosa et al. 2007; Zhang et al. 2012). The severity of the thermal challenge, the absolute temperature and duration, are factors affecting the performance and breast yield in meat chickens. Baziz et al. (1996) subjected meat chickens to constant temperatures of 22°C or 32°C over Weeks 4–7 of age and reported a depressed %breast weight to LW (11.9% vs 13.4%) at the higher temperature. Meat chickens exposed to a constant 34°C on Days 29–42 of age had a reduction in breast weight of 31.5%, while a cyclic temperature regime (23–36°C) reduced breast weight by only 9.9% (Zhang et al. 2012). In the current study, the lower maximum temperature, for a duration of 6 days, and the overnight recovery period limited the severity of thermal challenge compared with these earlier studies where a higher temperature range was imposed. The negative effects of high temperature on breast yields has been associated with reduced FI (Smith 1993; Lu et al. 2007; Rosa et al. 2007; Zhang et al. 2012). In the current study, there was no effect on feed intake.
Conclusions
Under the high-temperature challenge that can be considered to cause moderately severe HS conditions, providing water supplemented with electrolytes and betaine improved LWG. The requirement for increased electrolytes can be partly met by increased DEB. There is evidence that, at least in part, some of the benefit can be attributed to increased water intake. It is proposed that the intake of electrolytes is likely to be even more relevant under more extreme high temperatures, but this needs further evaluation.
Data availability
The data that support this study cannot be publicly shared due to funding privacy reasons and may be shared upon reasonable request to the corresponding author and if agreed to by the funding body.
Declaration of funding
Funding for this project was provided by the Rural Industries Research and Development Corporation, Canberra, ACT, Australia (now known as Agri-Futures Australia), Project No. PRJ-003776.
Acknowledgements
The author thanks the technical staff at The University of Sydney’s Poultry Research Unit for their assistance with maintenance and care of the animals. The assistance of Don Nicolson with laboratory analysis is much appreciated.
References
Ahmad T, Sarwar M (2006) Dietary electrolyte balance: implications in heat stressed broilers. World’s Poultry Science Journal 62, 638-653.
| Crossref | Google Scholar |
Ahmad T, Sarwar M, Mahr-un-Nisa , Ahsan-ul-haq , Zia-ul-hasan (2005) Influence of varying sources of dietary electrolytes on the performance of broilers reared in a high temperature environment. Animal Feed Science and Technology 120, 277-298.
| Crossref | Google Scholar |
Ahmad T, Mushtaq T, Mahr-Un-Nisa , Sarwar M, Hooge DM, Mirza MA (2006) Effect of different non-chloride sodium sources on the performance of heat-stressed broiler chickens. British Poultry Science 47, 249-256.
| Crossref | Google Scholar | PubMed |
Ahmad T, Mushtaq T, Khan MA, Babar ME, Yousaf M, Hasan ZU, Kamran Z (2009) Influence of varying dietary electrolyte balance on broiler performance under tropical summer conditions. Journal of Animal Physiology and Animal Nutrition 93, 613-621.
| Crossref | Google Scholar | PubMed |
Al-Abdullatif AA, Al-Sagan AA, Hussein EOS, Saadeldin IM, Suliman GM, Azzam MM, Al-Mufarrej SI, Alhotan RA (2021) Betaine could help ameliorate transport associated water deprivation stress in broilers by reducing the expression of stress-related transcripts and modulating water channel activity. Italian Journal of Animal Science 20, 14-25.
| Crossref | Google Scholar |
Alagawany M, Elnesr SS, Farag MR, El-Naggar K, Taha AE, Khafaga AF, Madkour M, Salem HM, El-Tahan AM, El-Saadony MT, Abd El-Hack ME (2022) Betaine and related compounds: chemistry, metabolism and role in mitigating heat stress in poultry. Journal of Thermal Biology 104, 103168.
| Crossref | Google Scholar | PubMed |
Al-Garadi MA, Suliman GM, Hussein EO, Al-Owaimer AN, Swelum AA, Almalamh NA, Alhotan RA, Qaid MM (2023) The effects of betaine and nano-emulsified plant-oil supplementation on growth performance and serum biochemistry indices of heat-stressed broiler chickens. Italian Journal of Animal Science 22, 398-406.
| Crossref | Google Scholar |
Arantes UM, Stringhini JH, Oliveira MC, Martins PC, Rezende PM, Andrade MA, Leandro NSM, Café MB (2013) Effect of different electrolyte balances in broiler diets. Brazilian Journal of Poultry Science 15, 233-237.
| Crossref | Google Scholar |
Araujo AC, Araújo RdS, Dourado LRB, Machado JS, Farias LA, de Sousa DM, de Sousa FCB, Biagiotti D, Bayão GFV, Sousa KRS (2022) Effects of dietary electrolyte balance on performance, energy balance, and expression of genes related to acid–basic balance, absorption, and transport of nutrients in broilers. Tropical Animal Health and Production 54, 165.
| Crossref | Google Scholar | PubMed |
Barreto Sánchez AL, Wang Q, Thiam M, Wang Z, Zhang J, Zhang Q, Zhang N, Li Q, Wen J, Zhao G (2022) Liver transcriptome response to heat stress in Beijing You chickens and Guang Ming broilers. Genes 13, 416.
| Crossref | Google Scholar |
Baziz HA, Geraert PA, Padilha JCF, Guillaumin S (1996) Chronic heat exposure enhances fat deposition and modifies muscle and fat partition in broiler carcasses. Poultry Science 75, 505-513.
| Crossref | Google Scholar | PubMed |
Belay T, Teeter RG (1993) Broiler water balance and thermobalance during thermoneutral and high ambient temperature exposure. Poultry Science 72, 116-124.
| Crossref | Google Scholar |
Borges SA, Fischer da Silva AV, Ariki J, Hooge DM, Cummings KR (2003) Dietary electrolyte balance for broiler chickens under moderately high ambient temperatures and relative humidities. Poultry Science 82, 301-308.
| Crossref | Google Scholar | PubMed |
Borges SA, da Silva AVF, Moura ASAMT, Maiorka A, Ostrensky A (2004) Electrolyte balance in broiler growing diets. International Journal of Poultry Science 3, 623-628.
| Crossref | Google Scholar |
Borges SA, Da Silva AVF, Maiorka A (2007) Acid‒base balance in broilers. World’s Poultry Science Journal 63, 73-81.
| Crossref | Google Scholar |
Dai NV, Bessel W, Quang NH (2009) The effects of sodium chloride and potassium chloride supplementation in drinking water on performance of broilers under tropical summer conditions. Archiv für Geflügelkunde 73, 41-48.
| Google Scholar |
Donkoh A (1989) Ambient temperature: a factor affecting performance and physiological response of broiler chickens. International Journal of Biometeorology 33, 259-265.
| Crossref | Google Scholar | PubMed |
Downing JA (2022) Nutritional strategies to support performance of commercial Pekin ducks exposed to a high-temperature thermal challenge over 29–41 days of age. Animal Production Science 62, 572-580.
| Crossref | Google Scholar |
Downing JA, Kerr MJ, Hopkins DL (2017) The effects of pre-transport supplementation with electrolytes and betaine on performance, carcass yield and meat quality of broilers in summer and winter. Livestock Science 205, 16-23.
| Crossref | Google Scholar |
Elshafaei HE, Rashed RR, Goma AA, El-Kazaz SE, Kerr MJ, Smith M, Hopkins DL, Downing JA (2020) Use of water electrolyte supplementation for three days prior to processing helps alleviate the consequences of a severe thermal challenge on performance in meat chickens. Livestock Science 242, 104260.
| Crossref | Google Scholar |
Gu XH, Hao Y, Wang XL (2012) Overexpression of heat shock protein 70 and its relationship to intestine under acute heat stress in broilers: 2. Intestinal oxidative stress. Poultry Science 91, 790-799.
| Crossref | Google Scholar | PubMed |
Johnson RA (2008) Respiratory alkalosis: a quick reference. Veterinary Clinics of North America: Small Animal Practice 38, 427-430.
| Crossref | Google Scholar | PubMed |
Johnson RJ, Karunajeewa H (1985) The effects of dietary minerals and electrolytes on the growth and physiology of the young chick. The Journal of Nutrition 115, 1680-1690.
| Crossref | Google Scholar | PubMed |
Karcher DM, Makagon MM, Fraley GS, Fraley SM, Lilburn MS (2013) Influence of raised plastic floors compared with pine shaving litter on environment and Pekin duck condition. Poultry Science 92, 583-590.
| Crossref | Google Scholar | PubMed |
Lara LJ, Rostagno MH (2013) Impact of heat stress on poultry production. Animals ‒ Basel 3, 356-369.
| Crossref | Google Scholar | PubMed |
Lin H, Jiao HC, Buyse J, Decuypere E (2006) Strategies for preventing heat stress in poultry. World’s Poultry Science Journal 62, 71-86.
| Crossref | Google Scholar |
Liu W, Yuan Y, Sun C, Balasubramanian B, Zhao Z, An L (2019) Effects of dietary betaine on growth performance, digestive function, carcass traits, and meat quality in indigenous Yellow-feathered broilers under long-term heat stress. Animals 9, 506.
| Crossref | Google Scholar | PubMed |
Liu L, Ren M, Ren K, Jin Y, Yan M (2020) Heat stress impacts on broiler performance: a systematic review and meta-analysis. Poultry Science 99, 6205-6211.
| Crossref | Google Scholar | PubMed |
Lott BD (1991) The effect of feed intake on body temperature and water consumption of male broilers during heat exposure. Poultry Science 70, 756-759.
| Crossref | Google Scholar | PubMed |
Lu Q, Wen J, Zhang H (2007) Effect of Chronic heat exposure on fat deposition and meat quality in two genetic types of chicken. Poultry Science 86, 1059-1064.
| Crossref | Google Scholar | PubMed |
Mishra A, Verma AK, Das A, Singh P, Bisht P, Wankar AK (2021) Exploring the potentials of betaine supplementation in poultry and pig: a review. Indian Journal of Animal Nutrition 38, 1-14.
| Crossref | Google Scholar |
Mongin P (1981) Recent advances in dietary anion-cation balance: applications in poultry. Proceedings of the Nutrition Society 40, 285-294.
| Crossref | Google Scholar | PubMed |
Moore SJ, VandeHaar MJ, Sharma BK, Pilbeam TE, Beede DK, Bucholtz HF, Liesman JS, Horst RL, Goff JP (2000) Effects of altering dietary cation-anion difference on calcium and energy metabolism in peripartum cows. Journal of Dairy Science 83, 2095-2104.
| Crossref | Google Scholar | PubMed |
Moraes SRPd, Yanagi Júnior T, Oliveira ALRd, Yanagi dNM, Café MB (2008) Classification of the temperature and humidity index (THI), aptitude of the region, and conditions of comfort for broilers and layer hens in Brazil. In ‘Proceedings of the International Conference of Agricultural Engineering, XXXVII Brazilian Congress of Agricultural Engineering, International Livestock Environment Symposium ‒ ILES VIII’, 31 August, Iguassu Falls City, Brazil. Ref.14. Pub. International Commission of Agricultural Engineering (CIGR), Institut furLandtechnik, Germany.
Murakami AE, Oviedo-Rondón EO, Martins EN, Pereira MS, Scapinello C (2001) Sodium and chloride requirements of growing broiler chickens (twenty-one to forty-two days of age) fed corn soybean diets. Poultry Science 80, 289-294.
| Crossref | Google Scholar | PubMed |
Mushtaq T, Mirza MA, Athar M, Hooge DM, Ahmad T, Ahmad G, Mushtaq MMH, Noreen U (2007) Dietary sodium and chloride for twenty-nine-to-forty-two-day-old broiler chickens at constant electrolyte balance under subtropical summer conditions. Journal of Applied Poultry Research 16, 161-170.
| Crossref | Google Scholar |
Mushtaq MMH, Pasha TN, Mushtaq T, Parvin R (2013) Electrolytes, dietary electrolyte balance and salts in broilers: an updated review on growth performance, water intake and litter quality. World’s Poultry Science Journal 69, 789-802.
| Crossref | Google Scholar |
National Health and Medical Research Council (2013) Australian code for the care and use of animals for scientific purposes. Available at https://www.nhmrc.gov.au/about-us/publications/australian-code-care-and-use-animals-scientific-purposes/australian-code-care-and-use-animals-scientific-purposes-code
Nawab A, Ibtisham F, Li G, Kieser B, Wu J, Liu W, Zhao Y, Nawab Y, Li K, Xiao M, An L (2018) Heat stress in poultry production: mitigation strategies to overcome the future challenges facing the global poultry industry. Journal of Thermal Biology 78, 131-139.
| Crossref | Google Scholar | PubMed |
Park SO, Kim WK (2017) Effects of betaine on biological functions in meat-type ducks exposed to heat stress. Poultry Science 96, 1212-1218.
| Crossref | Google Scholar | PubMed |
Park BS, Park SO (2017) Effects of feeding time with betaine diet on growth performance, blood markers, and short chain fatty acids in meat ducks exposed to heat stress. Livestock Science 199, 31-36.
| Crossref | Google Scholar |
Park SO, Zammit VA (2019) Effect of feed restriction with betaine and ascorbic acid supplementation on caecal bacteria, short chain fatty acid, blood biomarker, duodenal morphology and growth performance of meat ducks under heat stress. European Poultry Science 83, 1612-1919.
| Crossref | Google Scholar |
Popoola IO, Popoola OR, Ojeniyi MO, Olajide OO, Iyayi EA (2020a) The roles of key electrolytes in balancing blood acid-base and nutrient in broiler chickens reared under tropical conditions. Natural Sciences 12, 4-11.
| Crossref | Google Scholar |
Popoola IO, Popoola OR, Adeyemi AA, Ojeniyi OM, Olaleru IF, Oluwadele FJ, Akinwumi EO (2020b) Overall performance, carcass yield, meat safety potentials and economic value of heat-stressed broilers fed diets with balanced electrolytes. Food and Nutrition Sciences 11, 615-628.
| Crossref | Google Scholar |
Puvadolpirod S, Thaxton JP (2000) Model of physiological stress in chickens 1. Response parameters. Poultry Science 79, 363-369.
| Crossref | Google Scholar | PubMed |
Ratriyanto A, Mosenthin R (2018) Osmoregulatory function of betaine in alleviating heat stress in poultry. Journal of Animal Physiology and Animal Nutrition 102, 1634-1650.
| Crossref | Google Scholar | PubMed |
Ravindran V, Cowieson AJ, Selle PH (2008) Influence of dietary electrolyte balance and microbial phytase on growth performance, nutrient utilization, and excreta quality of broiler chickens. Poultry Science 87, 677-688.
| Crossref | Google Scholar | PubMed |
Rosa PS, Faria Filho DE, Dahlke F, Vieira BS, Macari M, Furlan RL (2007) Performance and carcass characteristics of broiler chickens with different growth potential and submitted to heat stress. Revista Brasileira de Ciência Avícola 9, 181-186.
| Crossref | Google Scholar |
Roushdy EM, Zaglool AW, El-Tarabany MS (2018) Effects of chronic thermal stress on growth performance, carcass traits, antioxidant indices and the expression of HSP70, growth hormone and superoxide dismutase genes in two broiler strains. Journal of Thermal Biology 74, 337-343.
| Crossref | Google Scholar | PubMed |
Saeed M, Abbas G, Alagawany M, Kamboh AA, Abd El-Hack ME, Khafaga AF, Chao S (2019) Heat stress management in poultry farms: a comprehensive overview. Journal of Thermal Biology 84, 414-425.
| Crossref | Google Scholar | PubMed |
Saki AA, Maleckey M, Johari R, Mirzaie Goudarzi S, Abdolmaleki M (2016) The effects of protein, amino acid, and dietary electrolyte balance on broiler chicken performance and blood parameters under heat stress. Acta Scientiarum. Animal Sciences 38, 285-292.
| Crossref | Google Scholar |
Sayed MAM, Downing J (2011) The effects of water replacement by oral rehydration fluids with or without betaine supplementation on performance, acid‒base balance, and water retention of heat-stressed broiler chickens. Poultry Science 90, 157-167.
| Crossref | Google Scholar | PubMed |
Sayed MAM, Downing J (2015) Effects of dietary electrolyte balance and addition of electrolyte–betaine supplements in feed or water on performance, acid–base balance and water retention in heat-stressed broilers. British Poultry Science 56, 195-209.
| Crossref | Google Scholar | PubMed |
Shao D, Wang Q, Hu Y, Shi S, Tong H (2019) Effects of cyclic heat stress on the phenotypic response, meat quality and muscle glycolysis of breasts and thighs of yellow-feather broilers. Italian Journal of Animal Science 18, 301-308.
| Crossref | Google Scholar |
Shin J-S, Um K-H, Park H-J, Choi Y-S, Lee H-S, Park B-S (2019) Effect of betaine and ascorbic acid in drinking water on growth performance and blood biomarkers in meat ducks exposed to heat stress. South African Journal of Animal Science 49, 417-423.
| Crossref | Google Scholar |
Smith MO (1993) Parts yield of broilers reared under cycling high temperatures. Poultry Science 72, 1146-1150.
| Crossref | Google Scholar |
Smith MO (1994) Effects of electrolyte and lighting regimen on growth of heat-distressed broilers. Poultry Science 73, 350-353.
| Crossref | Google Scholar | PubMed |
Smith MO, Teeter RG (1993) Carbon dioxide, ammonium chloride, potassium chloride, and performance of heat distressed broilers. Journal of Applied Poultry Research 2, 61-66.
| Crossref | Google Scholar |
Suk YO, Washburn KW (1995) Effects of environment on growth, efficiency of feed utilization, carcass fatness, and their association. Poultry Science 74, 285-296.
| Crossref | Google Scholar | PubMed |
Sun PX, Shen ZJ, Tang J, Huang W, Hou SS, Xie M (2019) Effects of ambient temperature on growth performance and carcass traits of male growing White Pekin ducks. British Poultry Science 60, 513-516.
| Crossref | Google Scholar | PubMed |
Szabó J, Vucskits A, Andrásofszky E, Berta E, Bersényi A, Börzsönyi L, Pálfi V, Hullár I (2011) Effect of dietary electrolyte balance on production, immune response and mineral concentrations of the femur in broilers. Acta Veterinaria Hungarica 59, 295-310.
| Crossref | Google Scholar | PubMed |
Wang YZ, Xu ZR, Feng J (2004) The effect of betaine and DL-methionine on growth performance and carcass characteristics in meat ducks. Animal Feed Science and Technology 116, 151-159.
| Crossref | Google Scholar |
Wang A, Fang CH, Wang HQ (2011) Regulation of dietary electrolyte balance in summer on blood acid‒base balance and growth performance of duck layer in caged system. Feed Industry 32, 50-53.
| Google Scholar |
Wasti S, Sah N, Mishra B (2020) Impact of heat stress on poultry health and performances, and potential mitigation strategies. Animals 10, 1266.
| Crossref | Google Scholar | PubMed |
Yalcin S, Settar P, Ozkan S, Cahaner A (1997) Comparative evaluation of three commercial broiler stocks in hot versus temperate climates. Poultry Science 76, 921-929.
| Crossref | Google Scholar | PubMed |
Yalçin S, Özkan S, Çabuk M, Siegel PB (2003) Criteria for evaluating husbandry practices to alleviate heat stress in broilers. Journal of Applied Poultry Research 12, 382-388.
| Crossref | Google Scholar |
Yu H, Azzam MM, Wang YB, Lin XJ, Alqhtani AH, Al-Abdullatif AA, Alhidary IA, Jiang SQ (2022) Dietary requirements of sodium and chloride for slow-growing broiler breeds during finisher phase of production. Journal of Applied Poultry Research 31, 100243.
| Crossref | Google Scholar |
Zhang ZY, Jia GQ, Zuo JJ, Zhang Y, Lei J, Ren L, Feng DY (2012) Effects of constant and cyclic heat stress on muscle metabolism and meat quality of broiler breast fillet and thigh meat. Poultry Science 91, 2931-2937.
| Crossref | Google Scholar | PubMed |
Zhong G, Shi SR, Shao D, Song ZG, Tong HB (2018) Effects of persistent heat stress on growth performance, meat quality and blood indexes on yellow-feathered broilers. Chinese Journal of Animal Nutrition 30, 3923-3929.
| Google Scholar |
Zhou WT, Fujita M, Yamamoto S (1998) Effects of food and water withdrawal and high temperature exposure on diurnal variation in blood viscosity of broiler chickens. British Poultry Science 39, 156-160.
| Crossref | Google Scholar | PubMed |
Zhou WT, Chaiyabutr N, Fujita M, Yamamoto S (1999a) Distribution of body fluid and change of blood viscosity in broilers (Gallus domesticus) under high temperature exposure. Journal of Thermal Biology 24, 193-197.
| Crossref | Google Scholar |
Zhou WT, Fujita M, Yamamoto S (1999b) Effects of ambient temperatures on blood viscosity and plasma protein concentration of broiler chickens (Gallus domesticus). Journal of Thermal Biology 24, 105-112.
| Crossref | Google Scholar |
Zulkifli I, Siegel PB (1995) Is there a positive side to stress? World’s Poultry Science Journal 51, 63-76.
| Crossref | Google Scholar |
Zulkifli I, Mysahra SA, Jin LZ (2004) Dietary supplementation of betaine (Betafin®) and response to high temperature stress in male broiler chickens. Asian‒Australasian Journal of Animal Sciences 17, 244-249.
| Crossref | Google Scholar |