Risk v. reward for responsive movements by a highly mobile fish species in a flow-constrained and barrier-laden river
Gavin L. Butler



A
B
C
D
Abstract
Understanding the movement behaviour of flow-dependent fish species is a foundational principle underlying the effective management of highly modified riverscapes.
To determine how variations in river discharge and instream barriers affect the residency, survival and movement of golden perch (Macquaria ambigua) in the degraded Gwydir River system within the northern Murray–Darling Basin.
We monitored the movement of 25 acoustic-tagged golden perch for up to 3 years by using a linear array spanning ~180 km of the main river channels across the lower Gwydir system.
Golden perch were largely sedentary for extended periods, with movements constrained by the barrier maze that now defines the system. High flows facilitated passage over instream barriers, with the highest periods of activity occurring in spring and early summer, and to a lesser extent in autumn.
Our findings are indicative of a highly constrained and isolated population of golden perch that is now likely to be neither a source nor a sink, but is in effect a false sink perpetuated by re-stocking practices.
The rehabilitation of the fish community in the Gwydir and other systems in similarly poor condition throughout the Murray–Darling Basin will require major institutional and societal change.
Keywords: environmental flows, false sink, golden perch, human-induced rapid environmental change, Macquaria, rheophilic, river regulation, source–sink dynamics.
Introduction
Understanding when and why freshwater fish move through their environment is fundamental for the effective management of rivers and streams (Ogburn et al. 2017). As the human population continues to increase exponentially, the demand for water is placing ever-increasing pressure on freshwater ecosystems across the globe (Gleick 1994; Wake 2021). Climate change adds yet another dimension to the stressors already affecting freshwater environments, with new and unprecedented extremes in the natural drying and flooding cycles of rivers now common place on all inhabited continents (Gudmundsson et al. 2021). The battle for what is effectively a finite resource in fresh water, not only looms as a risk to the ongoing existence of the anthropocentric world, but also threatens the persistence of the biota that inhabit these ecosystems (Cosgrove and Loucks 2015).
River discharge naturally varies in magnitude, timing and duration, and fish have evolved to exploit these challenging environments (e.g. Puckridge et al. 2000). All freshwater fish need to move at various scales to complete different aspects of their life cycles, whether it is to undertake daily foraging activities over distances of as little as 1–2 m or vast migrations of hundreds to thousands of kilometres to reach breeding grounds (Brönmark et al. 2014). Many of these movements are facilitated or cued by increases in river discharge, with even the smallest rise triggering individuals to move upstream, downstream or laterally into new habitats (Taylor and Cooke 2012). However, because river systems have become increasingly modified by humans, once reliable hydrological cues in many cases now have a lower likelihood of positive outcomes (Schlaepfer et al. 2002).
Source–sink population dynamics and the risk v. reward concept relate to movement and migration of fauna and are commonly used narratives in the management of a wide range of biota (Furrer and Pasinelli 2016; Hays et al. 2016). Source populations are self-sustaining and produce excess young or propagules that supply other areas known as sinks. Sinks receive individuals but do not supply other localities with individuals (Diffendorfer 1998). One of the fundamental notions that intrinsically link the two concepts is that there is an unconditional need for consistent connectivity between source and sink populations, and the risk is that populations that cannot reconnect become isolated and potentially cease to be self-sustaining (Furrer and Pasinelli 2016). For many taxa, migratory behaviour including moving between source and sink populations, is innately embedded within their evolutionary history. However, because migration paths are increasingly disrupted by anthropogenic influences, once reliable cues for migration now lead to risk without reward and potentially perilous outcomes.
Human-induced rapid environmental change (HIREC) relates to the state of the environment shifting at such a rate that it effectively outpaces the ability of biota to evolve (Sih 2013). In many cases, HIREC can result in animals misreading environmental cues and moving to suboptimal habitats or separating themselves from the main population for extended periods of time, therefore compromising their fitness, and increasing the likelihood of localised extirpations (Schlaepfer et al. 2002). Where HIREC has disrupted migration paths and movement has largely become unidirectional such as when dams and weirs are constructed across rivers and upstream passage is restricted, emigration most often outstrips immigration and upstream populations decline in abundance (Kemp 2015). One of the primary responses to offset HIREC in riverine fisheries management has been to introduce hatchery-reared fish above barriers. In some cases, a self-recruiting population is established, whereas in other instances, what can best be termed a false-sink population, is established, whereby stocking masks the absence of migrants or localised recruitment (see Claussen and Philipp 2023; Terui et al. 2023).
Golden perch (Macquaria ambigua) is a large-bodied (maximum ~600-mm total length) and long-lived species (26+ years; Wright et al. 2020) that inhabits most of the permanent and semipermanent freshwater rivers of the Murray–Darling Basin (MDB), Australia. The species is potamodromous and can be highly mobile, with some adults moving distances of >1000 km during periods of high flow (Reynolds 1983). Although these large-scale movements have received prominence in the scientific literature, populations are also capable of reproducing, recruiting or at least subsisting across relatively small scales for extended periods of time (Balcombe et al. 2006; Marshall et al. 2016; Zampatti et al. 2018). Like most fish species in the MDB, there has been a marked reduction in the abundance of golden perch relative to numbers pre-European settlement (Koehn et al. 2020). The highly responsive and plastic life-history strategies of golden perch, the widespread stocking of hatchery-produced fingerlings for recreational fishing, and the effects of river regulation across the entire MDB, continue to confound efforts to understand the natural population dynamics of the species.
The aim of the current study was to determine how variations in river discharge and instream barriers affect the residency, survival and movement of golden perch in a heavily modified river system in the northern MDB. Using the Gwydir River system as a case study, our objective was to better understand the subtle nuances in the behaviour of a flow-dependent specialist (Baumgartner et al. 2014) to inform water management, and to inform generalisations whereby the species is used as a flow indicator more widely in the MDB. The information gleaned will not only be of benefit to the management of golden perch more generally, but will ultimately contribute to the sustainability and conservation of the river ecosystems they inhabit as well.
Methods
Study area
The Gwydir catchment located in north-western New South Wales (NSW), Australia, is one of nine major catchments within the NSW portion of the MDB (Fig. 1). The study area was across the lowland sections of the Gwydir catchment and encompassed the upper Mehi and lower Gwydir rivers (Fig. 1). In the Mehi River, the study reach extended from Tareelaroi Weir, where the Mehi diverges from the Gwydir, downstream to the township of Moree (Fig. 1). In the Gwydir River, the study reach extended from 6 km upstream of Tareelaroi Weir, downstream into the Gwydir and Gingham watercourses below their confluence (Fig. 1). Typically, the river channel within both rivers is less than 25 m wide and 3 m deep. The Mehi is generally narrower (<15 m) and has the steeper gradient of the two systems. The Gwydir catchment is considered highly regulated, and the natural variability and the volume of water that now passes through its various rivers and streams is much reduced and highly controlled (Copeland et al. 2003). Through its two main tributaries, namely, the Gingham Watercourse and Gwydir River lower arm (Fig. 1), the Gwydir River terminates in a series of ephemeral wetlands that only connect to the mainstem of the much larger Barwon River during extreme flood events. Conversely, the Mehi River, although largely reliant on the diversion of water from the Gwydir River to flow, connects more often to the Barwon River, but fish passage is made difficult by the changed hydraulics the system now experiences and the presence of several large man-made instream barriers present along its length. Land use across the Gwydir catchment is primarily intensive agriculture, with the land adjacent to both rivers used mainly for irrigated crops. The system receives irrigation, stock and domestic, and environmental water releases from Copeton Dam (29.900742°S, 150.927682°E; capacity, 1346 GL), ~200 km upstream of the study area.
Acoustic telemetry array layout in the Gwydir (upper and lower), Mehi and Gingham rivers used to monitor the movement of golden perch (Macquaria ambigua). The locations of the larger weirs and regulators are also illustrated.
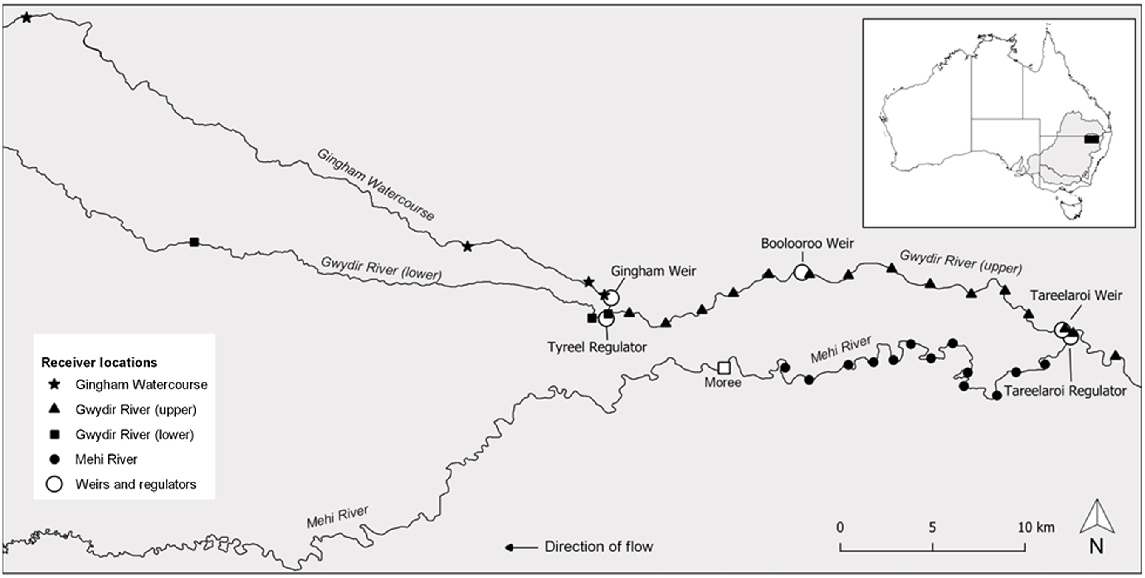
The instream habitat of both systems comprises a diversity of meso-habitats, including woody debris, undercut banks, overhanging riparian vegetation and aquatic macrophytes. The lower Gwydir traditionally supported populations of a range of native fish species, including notable large-bodied species (growing to >100 mm) such as freshwater catfish (Tandanus tandanus), Murray cod (Maccullochella peelii) and golden perch. Populations of all three species in the lower Gwydir are now in a poor state (Crook et al. 2023) and golden perch populations specifically are thought to be largely dependent on the stocking of hatchery-reared fingerlings to maintain local populations (Commonwealth Environmental Water Office 2019).
Fish collection and tagging
The intention at the outset of the project was to collect and tag a total of 25 ‘resident’ golden perch from within the confines of the study area, ideally with numbers similar from each of the Gwydir and Mehi rivers. However, after extensive sampling on 9–11 February 2021 using boat-mounted electrofishing (2.5-kW Smith-Root Model GPP 2.5 H/L), only 11 resident golden perch of suitable size were captured and tagged in the two rivers combined (Mehi, n = 2; Gwydir, n = 9). To meet quota, on 19–20 April 2021, gill nets (mesh size 75–100 mm) were used to source an additional 14 golden perch from upstream in Copeton Dam for translocation and release into the study area. Previous studies involving the translocation of golden perch in the Broken River (Crook 2004) and freshwater catfish from Copeton Dam to the lower Gwydir (Carpenter-Bundhoo et al. 2020) reported little or no difference between the behaviour of resident and translocated fish after an initial settling period. Copeton Dam fish were held overnight in a 4-m3 floating cage, before being transported in aerated drums (220 L) to riverine sites the following day.
All riverine fish were tagged and released on the day of capture, whereas those from Copeton Dam were tagged and released on the day of transportation. Prior to tagging, fish were anaesthetised in ambient water containing 50 mg L−1 benzocaine (ethyl-p-aminobenzoate) (Sigma–Aldrich, Shanghai) and weighed (g) and measured (mm). Fish were then transferred to an operating cradle, with water containing an equivalent level of anaesthetic (50 mg L−1) continually pumped over the gills to maintain anaesthesia. To access the peritoneal cavity, an incision was made through the body wall of the fish, adjacent to the linea alba and anterior of the anal vent. The sex of the fish was determined by attempting catheterisation of the fish’s vents as described by Ogburn et al. (1994) for silver perch (Bidyanus bidyanus), where the catheter can be easily inserted into the oviduct of the female with minimal pressure but is not able to be inserted into the male. Acoustic tags used were Innovsea V9 69 KHz acoustic transmitter (delay 80–160 s, approximate battery life of 859 days), which conformed to the recommended maximum tag weight of 2.25% of bodyweight for all fish (Jepsen et al. 2002; Butler et al. 2009; Wagner et al. 2011). Incisions were closed with two or three sutures using 0.3-mm pseudo-monofilament, absorbable thread (Vetafil Bengen; WdT, Garbsen, Germany). After suturing, the fish were returned to an aerated tub to recover before being released within 10–20 min.
This project was undertaken following the guidelines of and in accordance with the Australian Code of Practice for the Care and Use of Animals for Scientific Purposes (NSW Department of Primary Industries permit number 14/10).
Acoustic array
The movement of tagged fish was monitored by a linear array comprising 40 Innovasea VR2W 69-kHz receivers that covered ~180 km of the main river channels across the lower Gwydir catchment (Fig. 1). For visualisation and analysis purposes, the array was broken into four subarray sections: the Mehi River array, the Gingham Watercourse downstream of the Gingham Weir array, the Gwydir River lower arm downstream of the Tyreel Weir array, and the Gwydir River array, which extended from above Tareelaroi Weir to the junction of the lower arm and Gingham Watercourse (Fig. 1). The Mehi array spanned 31 km using 13 receivers, the Gingham array spanned 72 km using 8 receivers, the lower Gwydir array spanned 43 km using 4 receivers, and the upper Gwydir array spanned 37 km using 15 receivers. The receivers recorded binary presence or absence data, with the presence of a tagged fish logged when it entered the reception range of a given receiver (>50 m).
Statistical analysis
River path spatial objects were created using current satellite imagery of the study reaches in QGIS (ver. 3.20, see www.qgis.org). A linear distance matrix for acoustic receiver locations was then generated using Euclidean distances along the river paths in the V-Track package (ver. 2.2, see https://github.com/RossDwyer/VTrack; Campbell et al. 2012) in R (ver. 4.2.2, R Foundation for Statistical Computing, Vienna, Austria, see www.r-project.org) and the distance between individual fish detections was calculated. A daily sum of total distance moved, including both upstream and downstream movements, was then calculated for each fish. The first 24 h of detection for each fish was removed from analysis to discount any abnormal behaviour resulting from the translocation and tagging procedures (Carpenter-Bundhoo et al. 2020). A binary movement response was calculated as a detection of an individual on a minimum of two receivers within a given 24-h period for movement (1) or, otherwise, no-movement (0). In this daily movement database, individual fish were also assigned a no-movement (0) on days where they were not detected if the day was not after the final detection for that given fish, and the last and next detections were not from terminal receivers for that given fish. Otherwise, non-detected days were omitted from the database. Total linear range was calculated as the river distance between the most upstream and downstream detections for each fish over the study period, and cumulative distance moved was calculated as the sum of all upstream and downstream movements for each fish for the study period.
Generalised additive mixed models (GAMMs) fitted with binomial distributions and a logit link were used to analyse probability of movement. All models were built using the mgcv package (ver. 1.9-1, S. Wood, see https://cran.r-project.org/package=mgcv/; Wood 2003). Total daily river discharge, antecedent flow, river (Gwydir or Mehi), day of year (DOY), mean daily air temperature, lunar illumination, and fish length, weight and sex were all included as candidate independent variables. Source (river or impoundment) was also considered as a candidate variable but was not found to be a significant predictor of either likelihood of movement or distance moved and was removed during model selection. Antecedent flow was calculated as a rolling sum of the previous 30-day river discharge, generated from flow gauges relevant to an individual’s current position in the river system. Lunar illumination (%) was generated using the R package lunar (ver. 0.2-1, E. Lazaridis, see http://statistics.lazaridis.eu). Discharge and antecedent flow were transformed with a natural logarithm because they were highly skewed. A random intercept was included for each transmitter (ID) in the models because the data comprised repeated observations of each tagged fish. Year of study (Year) was included as another potential random intercept to account for yearly variation in environmental parameters not accounted for by the fixed effects. Continuous predictors were fitted with cubic splines, DOY was fitted with cyclic cubic splines, and river was included as a parametric term. An autocorrelation structure (AR1) was incorporated in each GAMM to account for temporal autocorrelation. All possible models and interactions were examined, and the best model was selected using Chi-Square tests of restricted maximum likelihood (fREML) scores. Following the protocol of Zuur et al. (2010), data were checked for outliers and collinearity among predictor variables was assessed using variance inflation factors (VIF) in R.
Results
Tagged golden perch were detected from February 2021 to October 2023. Golden perch in the Gwydir River were detected for longer (183 ± 43 days; mean ± s.e.) and were recorded moving greater cumulative distances (139 ± 37 km; Table 1) than were those in the Mehi River. Five of the nine fish released in the Mehi River array were not recorded moving from their initial detection location (Supplementary Table S1). The maximum number of movements and cumulative distance moved by individual fish were of similar magnitude between the two rivers (Table 1).
Item | Number of detections | Days detected in array | Number of movements | Cumulative dist. moved (km) | Linear range (km) | |||||
---|---|---|---|---|---|---|---|---|---|---|
Gwydir | Mehi | Gwydir | Mehi | Gwydir | Mehi | Gwydir | Mehi | |||
Min. | 0 | 5 | 0 | 2 | 1 | 0 | 2 | 0 | 0 | |
Max. | 110,038 | 56,585 | 570 | 256 | 81 | 51 | 474 | 354 | 73 | |
Mean ± s.e. | 28,141 ± 8568 | 8218 ± 6519 | 183 ± 43 | 47 ± 29 | 24 ± 5 | 7 ± 6 | 139 ± 37 | 43 ± 39 | 20 ± 5 |
Number of movements, linear range and cumulative distance were generated using only the data from the 23 fish that were detected. Linear ranges were generated using fish from both rivers combined because some individuals crossed between the two systems.
Of the 25 tagged fish, 23 were recorded on one or more receivers. The reason for the non-detection of Fish 51061 (Gwydir) and Fish 51071 (Mehi) is unknown but may have been due to mortality, angler or predator capture, tag failure or inactivity. Overall, the movement behaviour of golden perch was individualistic in nature, with several fish moving little and residing within the vicinity of their release receiver for extended periods of >100 days, whereas others moved regularly, including one individual tagged and released in the Mehi River undertaking 52 separate relocations among receivers within a relatively confined ~20-km stretch of the river and amassing over 340 km of cumulative movement during the study (Supplementary Fig. S1). No fish moved from the Gwydir River to the Mehi River, but two individuals (Fish 51057 and Fish 51073; Fig. S1) crossed the Tareelaroi Regulator from the Mehi River into the upper Gwydir River (Fig. 1), with one exiting the Gwydir River array permanently at the upstream end. One fish (Fish 51054; Fig. S1b) also exited the downstream extent of the array in the Mehi River in early 2021 and did not return. Data from receivers deployed around the downstream junction of the Mehi and Barwon rivers (<100 km downstream of lowest Mehi receiver) for a concurrent but unrelated study were cross-referenced at the completion of the current study, and no tagged fish from the current study had been detected. In all, 10 of the 16 golden perch tagged in the Gwydir River moved downstream into either the Gingham Watercourse or Gwydir River lower arm arrays, with five fish last detected in these reaches (51063, 51066, 51069, 51070 and 51076; Fig. S1a). Most crossings of the Gingham Weir, between the Gwydir River and Gingham Watercourse, and all crossings of Tyreel Weir, between the Gwydir River and Gwydir River lower arm, were undertaken during high-flow events (10th percentile and above).
The behaviour of fish in the Gwydir River comprised the following three broad movement types: (i) largely resident despite there being various opportunities to move during increased discharge; rarely detected on more than one receiver (n = 5; Fig. 2a, b); (ii) substantial periods of weeks or even months moving constantly within the barrier maze (a network of human-made in-channel barriers, including an extensive incidental raft cf. Copeland et al. 2003; Erskine et al. 2012); detected on multiple receivers upstream and downstream of their release point, but did not leave the confines of the barrier maze (n = 5; Fig. 2c, d); and (iii) consistent movements within the barrier maze before moving downstream into the lower Gwydir or Gingham wetland complexes during a high-discharge event (n = 5; Fig. 2e, f). Of those from Category 3, three fish moved substantial distances once out of the barrier maze, making multiple and rapid upstream and downstream (~50 km) movements, before returning to an upstream barrier and either moving over and back into the barrier maze or residing below the barrier for some time before disappearing (Fig. 2e, f).
(a–f) Linear distance travelled from most downstream receivers for select golden perch (Macquaria ambigua) individuals (51059, 51064, 51057, 51065, 51063 and 51076) in the Gwydir River system for the recording period of February 2021–October 2023. Dashed horizontal lines denote weir or regulator position (TW, Tareelaroi Weir; BW, Boolooroo Weir; G-TW, Gingham–Tyreel Weir). The Gingham and Tyreel weirs are located approximately equidistant down their respective tributaries. River position is denoted by shaded circles: grey, Gwydir River; black, lower Gwydir River; white, Gingham Watercourse.
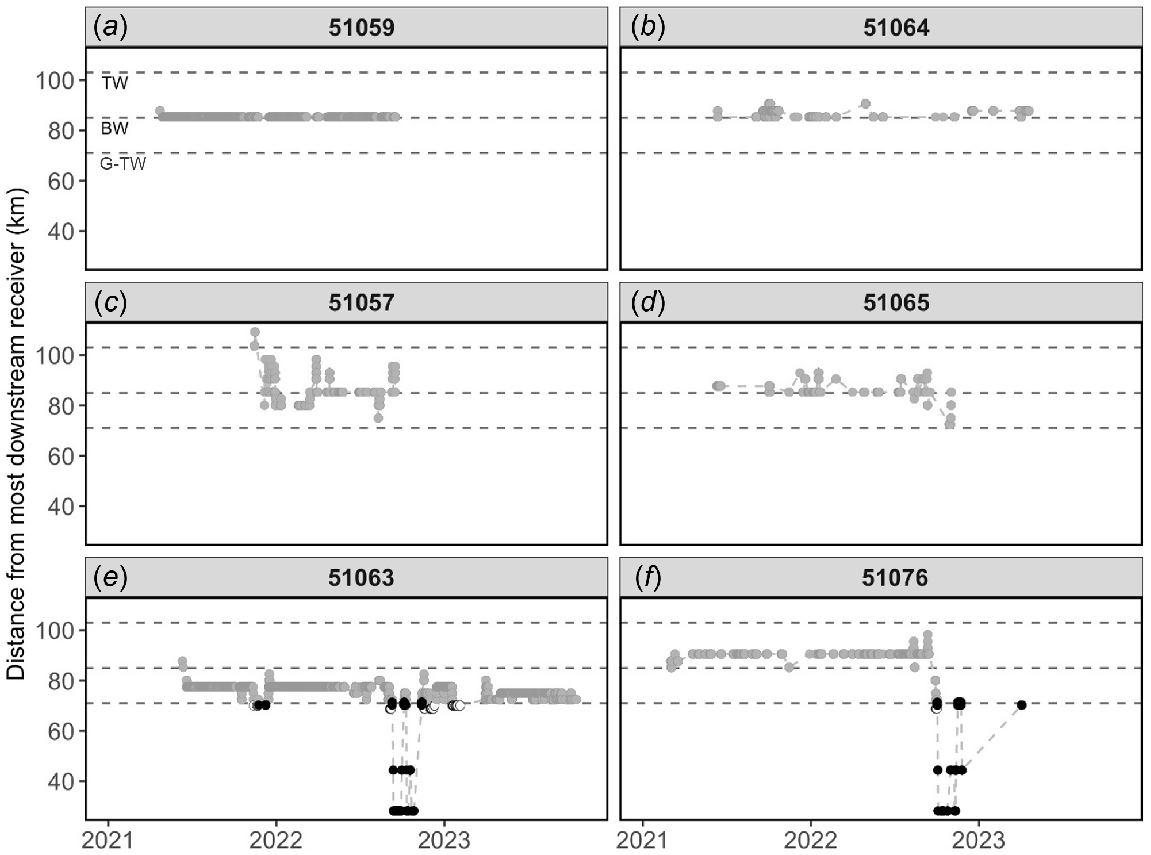
Overall, the probability of golden perch moving was not significantly different between the Gwydir and Mehi rivers (P = 0.063; Table 2). However, the response to discharge varied significantly between rivers (Fig. 3a, b). Fish in the Gwydir River were more likely to move as river discharge increased (P = 0.011), with the likelihood of movement increasing to 75% before reaching a plateau at ~2400 ML day−1. In the Mehi River, probability of movement and river discharge had a bimodal relationship (P < 0.001), with the likelihood of movement peaking at ~40 ML day−1 before falling and then peaking again at 750 ML day−1 (Fig. 3b). Golden perch were less likely to move when antecedent flows were higher (P = 0.013; Fig. 3c), with the probability of movement decreasing to 40% when total discharge of ~40,000 ML had been attained in the past 30 days. The movement behaviour of golden perch was somewhat seasonal, with movement probability being highest in early summer and lowest in winter (P < 0.001; Fig. 3d). Golden perch were also more likely to move during periods of higher lunar illumination (P = 0.018; Fig. 3e).
Response | Adj. R2 | Type | Variable | Estimate | s.e. | z-stat | P-value | ||
---|---|---|---|---|---|---|---|---|---|
Movement (1/0) | 0.13 | Fixed | River: Mehi | −0.607 | 0.326 | −1.861 | 0.063 |
Type | Basis | Variable | d.f.e | d.f.ref | χ2 | P-value | |||
---|---|---|---|---|---|---|---|---|---|
Spline | Cubic regression | log(Discharge): Gwydir | 1 | 1 | 6.496 | 0.011 | |||
Cubic regression | log(Discharge): Mehi | 7.115 | 8.093 | 110.90 | <0.001 | ||||
Cubic regression | log(Antecedent) | 2.506 | 3.173 | 11.13 | 0.013 | ||||
Cubic cyclic regression | DOY | 5.319 | 8 | 112.05 | <0.001 | ||||
Cubic regression | Lunar illumination | 1 | 1 | 5.62 | 0.018 | ||||
Random effect | Fish ID | 15.682 | 22 | 145.54 | <0.001 |
Spline statistics are presented with estimated degrees of freedom (d.f.e), reference degrees of freedom (d.f.ref) and P-values.
Predicted probabilities (±95% CI) of golden perch (Macquaria ambigua) movement as a function of daily discharge in (a) the Gwydir and (b) Mehi rivers, (c) antecedent discharge, (d) day of year and (e) lunar illumination. Discharge and antecedent flow were transformed with a natural logarithm because they were highly skewed.
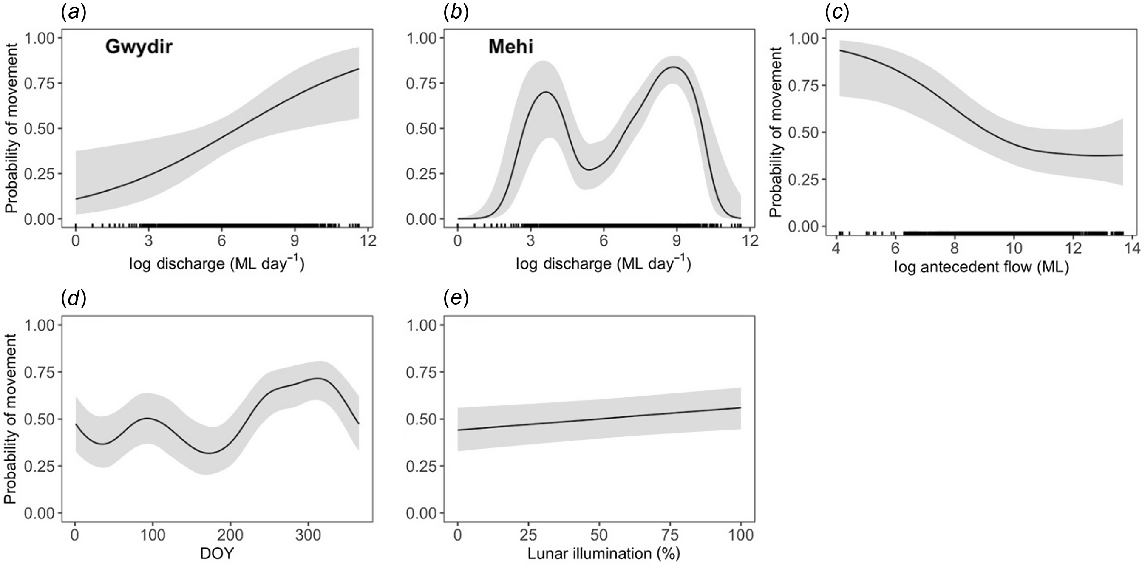
Discussion
The combination of myriad anthropogenic in-channel barriers and the artificial flow regime in the Gwydir catchment strongly influence the adult movement behaviour and persistence of golden perch across the entirety of the system. The loss of pelagophils and challenges of managing fish assemblages in highly modified riverscapes exists as an issue for river managers globally, in attempting to balance the needs of humans while protecting biodiversity (Perkin and Gido 2011; Yarnell et al. 2015; Mollenhauer et al. 2021). Here, we discuss the impact of barriers to movement of golden perch in the Gwydir and conclude that the population is likely to be no longer self-sustaining. Subsequently, we canvas the potential for using alternative taxa as local flow indicators.
Within catchment movement
Region- or subcatchment-specific population processes (e.g. nurseries, source–sink dynamics) are important in understanding golden perch ecology (Zampatti and Leigh 2013). In the southerly extremities of its distribution, golden perch movement has been reported to be constrained by low-head weirs used to control irrigation deliveries (e.g. O’Connor et al. 2005; Koster et al. 2014, 2017), and weir pools have also been shown to lessen the effects of river-level rises, resulting in the reduced frequency of large-scale movements (Koster et al. 2020). In addition, not unlike the movement predicament the species experiences in the Gwydir system, golden perch in the Moonie River in the far northern MDB were confined to waterholes for extended periods because of a lack of connectivity along the main channel (Marshall et al. 2016). These studies have parallels with the behaviour of golden perch in the current study and reiterate the effect river regulation is having on the species across the entire MDB.
Although most river systems across the MDB share similarities in how they are regulated, the surface-water connectivity of the Gwydir system is also somewhat unique from other locations where golden perch movement has been studied (e.g. Llewellyn 2014; Koehn and Nicol 2016; Koster et al. 2017; Zampatti et al. 2018; Thiem et al. 2020). The substantial ‘raft’ of large wood debris that spans over 12 river kilometres in the lower Gwydir River acts as a substantial in-channel barrier for water and fish (Copeland et al. 2003; Erskine et al. 2012) and has no equivalence elsewhere in the MDB. The Gwydir Raft is an artefact of upstream land-clearing activities that commenced in the 1800s (Copeland et al. 2003), and has resulted in considerable stream braiding and shallow-bypass channelling in its vicinity both upstream and downstream (Erskine et al. 2012). Only three tagged fish traversed the raft in our study, and this occurred only on the highest discharges recorded during the study (Fish 51060, Fish 51063, Fish 51076; Fig. S1). The combination of water management and the raft is preventing migratory fish such as golden perch from transiting the catchment and the flow-cued response of adult fish is also leading them to ephemeral waterways, which are both clear illustrations of the impacts of HIREC and highlight how what was once a low risk now has no reward (Sih 2013).
Although our model did not predict a difference in golden perch movement between the Mehi and Gwydir rivers individually, there was a strong difference in movement responses in relation to discharge between the two rivers. Although being physically linked, the Gwydir and Mehi rivers have distinct flow regimes, with the Gwydir receiving far higher flow magnitudes because of differences in the amounts of irrigation water it receives and due to the different flood-mitigation strategies employed for the two systems. A similar disparity in movement responses between the two rivers was observed in Murray cod (Maccullochella peelii) and freshwater catfish (Tandanus tandanus) (Carpenter-Bundhoo et al. 2023), with both species being more likely to move with increased discharge in both systems but there was a higher probability of movement on smaller discharges in the Mehi River. The difference in golden perch responses at the river scale most likely reflects alternative movement strategies for differing hydrologic habitats. In the Gwydir River, where higher-magnitude and more frequent flows occur, golden perch exhibited an almost linear movement response to increased discharge. However, in the Mehi River, golden perch displayed a more bimodal response in movement strategies, opportunistically moving as connectivity increased on smaller discharges (as per Carpenter-Bundhoo et al. 2023), and then moving again only on high-magnitude discharges. This disparity in behaviour highlights the need to identify and consider the specific hydro-ecological responses of fishes and the characteristics of the rivers they inhabit when developing water-management strategies for a region.
Despite the numerous high-discharge events and flooding experienced during the current study, there was no evidence of simultaneous large-scale movement by golden perch indicative of a synchronised spawning migration. This contrasts the telemetry study of O’Connor et al. (2005) in the mid-Murray River system, where golden perch moved unidirectionally in spring, before aggregating below the confluence of rivers. Golden perch in the Goulburn River acted similarly, moving downstream in spring on elevated environmental-flow releases, with spawning being confirmed by larval drift net sampling (Koster et al. 2017). The increase in the activity by some golden perch individuals from September through to December in the current study does fit the perceived window of spawning for the species (e.g. O’Connor et al. 2005), but was largely individualistic and coincided with an increased flow, and is likely to have represented opportunistic relocations rather than spawning-related movements (i.e. no synchronised movements or aggregations of tagged fish). Unlike their southern conspecifics, golden perch in the northern MDB have been reported as spawning across an expansive window within an annual cycle (Ebner et al. 2009; Thiem et al. 2023). However, the Darling River and its tributaries now support suitable conditions for system-wide spawning and recruitment only during extended and consistent periods of high flows (e.g. La Niña conditions), which occur infrequently across the broader northern MDB and even more rarely in the Gwydir (Stuart and Sharpe 2020; Thiem et al. 2023).
The perceived poor state of the golden perch population in the Gwydir system is supported by the findings of several surveys undertaken in recent decades, with only low numbers captured in all years sampled and negligible evidence of natural recruitment (e.g. Commonwealth Environmental Water Office 2019; Crook et al. 2023). Collectively, this suggests that the Gwydir now functions as neither a source nor a sink for golden perch within the broader context of the MDB (Fig. 4). Effectively, it now appears to be more akin to terminal wetland system like the Lachlan River located in the southern MDB, which, like the Gwydir, has little or no migration of golden perch into the system and lacks any substantial natural recruitment (e.g. Shams et al. 2020; Schilling and Crook 2023).
Migration and immigration
The Gwydir system is a highly modified riverscape that is effectively unrecognisable in the context of what could be considered a naturally flowing and unimpeded system (Yarnell et al. 2015). The combination of the ‘barrier maze’ created by myriad instream anthropogenic infrastructure and the Gwydir raft, and the resulting modified flow regime, has resulted in the Gwydir system transitioning to become a largely isolated compartment of the MDB. In addition, Copeton Dam is one of the largest instream dams in the MDB and, like large headwater impoundments globally (Barbarossa et al. 2020; Belletti et al. 2020), has also profoundly affected the flow regime across the entire system below. Water quality is also affected for vast distances below the dam, including cold-water plumes that extend 100 km into the systems lower reaches (Copeland et al. 2003). The combined impact of these environmental changes on the broader riverscape means that the fish community in the Gwydir no longer functions as it once did.
The results of the current study suggest that movement of adult golden perch between the Gwydir system and the broader MDB population is limited at best. This was not the case historically, with a healthy population of non-supplemented golden perch present throughout the system prior to river regulation (Copeland et al. 2003). The construction of Copeton Dam has seen the virtual loss of natural winter flow peaks, an increase in smaller flow events in summer because of irrigation releases, and the almost total absence of large floods (the latter originally the main means by which the Gwydir connected to the Barwon River) (Thoms et al. 2001). In this study, several golden perch exited at the downstream extent of the array in the Gwydir and Gingham channels in a possible attempt to move to the Barwon River, but the majority returned to the upstream reaches sometime after or were presumably trapped in the wetland below, due to there being insufficient flow to fully connect the Gwydir and Barwon rivers.
The degree of riverscape modification that has occurred over the past century in the Gwydir catchment appears to be too great to allow a self-sustaining population of golden perch to perpetuate. River regulation and barriers have led to a loss of pelagophils in arid and semi-arid rivers globally, mainly due to the loss of flow-related life-history cues, the lack of stream connectivity constraining spawning aggregations, and that there are now insufficent stretches of uninterrupted river channel in many systems to support drifting eggs and larvae (e.g. Dudley and Platania 2007; Hoagstrom and Turner 2015; Lechner et al. 2016). Furthermore, a decline in stream invertebrate productivity, as a further consequence of regulation, has rendered many rivers incapable of sustaining drifting pelagic larvae and juvenile pelagophiles (Balcombe et al. 2005; Humphries et al. 2020). Effectively, HIREC means that the life-history strategies of pelagophils have become largely ineffective in highly regulated rivers like the Gwydir. Juvenile and subadult golden perch immigration is one possible means by which the Gwydir population could be bolstered, but requires intimate knowledge of golden perch dynamics and the reinstatement of local connectivity (e.g. Stuart and Sharpe 2020; Stuart et al. 2024).
Empirical information is critical to understanding source–sink dynamics (Diffendorfer 1998). Whether or not the Gwydir catchment historically contained a source or sink population of golden perch is unknown. Results from the current study suggest that adult golden perch are now unlikely to move between the Gwydir and Barwon river systems even in wet years. Under the current scenario, a better management strategy to promote golden perch movement (including adult and subadult movement) may be to work toward reconnecting the Mehi River through targeted flow delivery, rather than the assumed traditional pathway via the Gwydir wetland complex (Fig. 4).
Flow indicators
Functional groups of fishes have been identified and used in monitoring, prescribing, and managing environmental flows across the MDB, and golden perch has been a prominent species used as a key indicator species in this regard (King et al. 2009; Zampatti and Leigh 2013; Baumgartner et al. 2014; Stuart and Sharpe 2020; Stuart et al. 2024). However, our study indicated that golden perch is not currently a useful flow indicator species within the Gwydir system. Species that respond to localised changes in flow delivery in-channel or on floodplains are likely to be better indicators and potentially should be given precedence when designing managed flow releases.
Baumgartner et al. (2014) recognised flow-dependent specialists and floodplain specialists as two important functional groups in a review of the southern MDB. Of the two flow-dependent specialists recognised in that study (golden perch, silver perch), neither exists as self-sustaining populations in the Gwydir system. Small-body in-channel species such as the Darling River hardyhead (Craterocephalus amniculus) (Moy et al. 2018), or floodplain specialists such as olive perchlet (Ambassis agassizii), both of which can complete their lifecycle at a smaller extent (e.g. reach scale), may be better environmental indicators in the Gwydir than are larger-bodied ranging species such as golden perch. Although the concept of using small-bodied species as flow indicators is sound, society rarely rallies behind small non-iconic fishes (Ebner et al. 2016). As such, at least one large-bodied, non-rheophilic species, with some level of flow dependency (e.g. freshwater catfish), is likely to be a better focal species for targeted environmental flow releases in the Gwydir system (Carpenter-Bundhoo et al. 2020, 2023). Alternatively, the fall-back position is to monitor species that have shown some level of response to environmental flows in recent decades in the Gwydir and elsewhere in the MDB (e.g. spangled perch (Leiopotherapon unicolor) and bony herring (Nematalosa erebi) Wilson et al. 2010). For the foreseeable future, the ongoing put-and-take fishery for golden perch (Fig. 4) will remain important to local anglers and, by extension, for maintaining relevance for fish and river management for local people. To rehabilitate the fish community in the Gwydir system, and especially to recover flow-dependent specialists, major institutional and societal change at all levels of government and the wider community alike is required (Fig. 4) (Johnson et al. 2017; Claussen and Philipp 2023; Terui et al. 2023).
Conclusions
Adult golden perch, which are generally considered highly mobile pelagophiles, were largely sedentary in the Mehi River and were constrained within the barrier maze of the Gwydir River, with the individuals that moved downstream in the latter system not exiting to the greater Barwon–Darling River system. Flow-stimulated downstream movements into the wetlands located in the lower reaches of the system resulted in the isolation of golden perch from the remainder of the upstream population for extended periods. Systematic and regular connectivity of the river channels that feed these wetlands is required to ensure that fish trapped in these ephemeral habitats can move back into the main sections of the river. Further research is required to quantify the timing and magnitude of the flows needed to allow golden perch to utilise all available habitats throughout the Gwydir system. Our results suggest that the golden perch population in the Gwydir is now neither a source nor a sink, but is in effect a false sink perpetuated by re-stocking practices. The findings we have presented and the alternate strategies we have suggested for reinstating and monitoring the fish community in the Gwydir system, serve to promote clear policy and management options for highly modified riverscapes and for those wide-ranging fish species such as golden perch that once resided and thrived naturally within them.
Data availability
The datasets generated or analysed during the current study are available from the corresponding author on a reasonable request.
Declaration of funding
The research was supported by funding from the Commonwealth Environmental Water Holder, the Department of Climate Change, Energy, the Environment and Water, and from the New South Wales Recreational Fishing Trust (Freshwater).
Acknowledgements
We thank the Traditional Owners, the Kamilaroi people, as well as the current Landholders for allowing us access onto their land. Thanks also go to the devoted staff who helped with the collection and tagging of fish, and the management of the acoustic array and associated data, including Sean Blake, John St Vincent Welch, Chris Bowen, Jarrod Chapman and Lauren Stoot. Finally, thanks go to the various reviewers for comments that improved this paper.
References
Balcombe SR, Bunn SE, McKenzie-Smith FJ, Davies PM (2005) Variability of fish diets between dry and flood periods in an arid zone floodplain river. Journal of Fish Biology 67, 1552-1567.
| Crossref | Google Scholar |
Balcombe SR, Arthington AH, Foster ND, Thoms MC, Wilson GG, Bunn SE (2006) Fish assemblages of an Australian dryland river: abundance, assemblage structure and recruitment patterns in the Warrego River, Murray–Darling Basin. Marine and Freshwater Research 57, 619-633.
| Crossref | Google Scholar |
Barbarossa V, Schmitt RJP, Huijbregts MAJ, Zarfl C, King H, Schipper AM (2020) Impacts of current and future large dams on the geographic range connectivity of freshwater fish worldwide. Proceedings of the National Academy of Sciences 117, 3648-3655.
| Crossref | Google Scholar |
Baumgartner LJ, Conallin J, Wooden I, Campbell B, Gee R, Robinson WA, Mallen-Cooper M (2014) Using flow guilds of freshwater fish in an adaptive management framework to simplify environmental flow delivery for semi-arid riverine systems. Fish and Fisheries 15(3), 410-427.
| Crossref | Google Scholar |
Belletti B, Garcia de Leaniz C, Jones J, Bizzi S, Borger L, Segura G, Castelletti A, van de bund W, Aarestrup K, Barry J, Belka K, Berkhuysen A, Birnie-Gauvin K, Bussettini M, Carolli M, Consuegra S, Dopico E, Feierfeil T, Fernández S, Fernandez Garrido P, Garcia-Vazquez E, Garrido S, Giannico G, Gough P, Jepsen N, Jones PE, Kemp P, Kerr J, King J, Łapińska M, Lázaro G, Lucas MC, Marcello L, Martin P, McGinnity P, O’Hanley J, Olivo Del Amo R, Parasiewicz P, Pusch M, Rincon G, Rodriguez C, Royte J, Schneider CT, Tummers JS, Vallesi S, Vowles A, Verspoor E, Wanningen H, Wantzen KM, Wildman L, Zalewski M (2020) More than one million barriers fragment Europe’s rivers. Nature 588, 436-441.
| Crossref | Google Scholar |
Brönmark C, Hulthén K, Nilsson PA, Skov C, Hansson L-A, Brodersen J, Chapman BB (2014) There and back again: migration in freshwater fishes. Canadian Journal of Zoology 92(6), 467-479.
| Crossref | Google Scholar |
Butler GL, Mackay B, Rowland SJ, Pease BC (2009) Retention of intra-peritoneal transmitters and post-operative recovery of four Australian native fish species. Marine and Freshwater Research 60, 361-370.
| Crossref | Google Scholar |
Campbell HA, Watts ME, Dwyer RG, Franklin CE (2012) V-Track: software for analysing and visualising animal movement from acoustic telemetry detections. Marine and Freshwater Research 63, 815-820.
| Crossref | Google Scholar |
Carpenter-Bundhoo L, Butler GL, Espinoza T, Bond NR, Bunn SE, Kennard MJ (2020) Reservoir to river: quantifying fine-scale fish movements after translocation. Ecology of Freshwater Fish 29, 89-102.
| Crossref | Google Scholar |
Carpenter-Bundhoo L, Butler GL, Bond NR, Thiem JD, Bunn SE, Kennard MJ (2023) Fish movements in response to environmental flow releases in intermittent rivers. Freshwater Biology 68(2), 260-273.
| Crossref | Google Scholar |
Claussen JE, Philipp DP (2023) Assessing the role of supplementation stocking: a perspective. Fisheries Management and Ecology 30(6), 583-591.
| Crossref | Google Scholar |
Cosgrove WJ, Loucks DP (2015) Water management: current and future challenges and research directions. Water Resources Research 51, 4823-4839.
| Crossref | Google Scholar |
Crook DA (2004) Movements associated with home-range establishment by two species of lowland river fish. Canadian Journal of Fisheries and Aquatic Sciences 61(11), 2183-2193.
| Crossref | Google Scholar |
Crook DA, Schilling HT, Gilligan DM, Asmus M, Boys CA, Butler GL, Cameron LM, Hohnberg D, Michie LE, Miles NG, Rayner TS, Robinson WA, Rourke ML, Stocks JR, Thiem JD, Townsend A, van der Meulen DE, Wooden I, Cheshire KJM (2023) Multi-decadal trends in large-bodied fish populations in the New South Wales Murray–Darling Basin, Australia. Marine and Freshwater Research 74, 899-916.
| Crossref | Google Scholar |
Diffendorfer JE (1998) Testing models of source–sink dynamics and balanced dispersal. Oikos 417-433.
| Crossref | Google Scholar |
Dudley RK, Platania SP (2007) Flow regulation and fragmentation imperil pelagic-spawning riverine fishes. Ecological Applications 17(7), 2074-2086.
| Crossref | Google Scholar | PubMed |
Ebner BC, Scholz O, Gawne B (2009) Golden perch Macquaria ambigua are flexible spawners in the Darling River, Australia. New Zealand Journal of Marine and Freshwater Research 43, 571-578.
| Crossref | Google Scholar |
Ebner BC, Morgan DL, Kerezsy A, Hardie S, Beatty SJ, Seymour JE, Donaldson JA, Linke S, Peverell S, Roberts D, Espinoza T, Marshall N, Kroon FJ, Burrows DW, McAllister RRJ (2016) Enhancing conservation of Australian freshwater ecosystems: identification of freshwater flagship fishes and relevant target audiences. Fish and Fisheries 17, 1134-1151.
| Crossref | Google Scholar |
Erskine WD, Saynor MJ, Chalmers A, Riley SJ (2012) Water, wind, wood, and trees: interactions, spatial variations, temporal dynamics, and their potential role in river rehabilitation. Geographical Research 50(1), 60-74.
| Crossref | Google Scholar |
Furrer RD, Pasinelli G (2016) Empirical evidence for source–sink populations: a review on occurrence, assessments and implications. Biological Reviews 91, 782-795.
| Crossref | Google Scholar | PubMed |
Gleick PH (1994) Water, war & peace in the Middle East. Environment: Science and Policy for Sustainable Development 36(3), 6-42.
| Crossref | Google Scholar |
Gudmundsson L, Boulange J, Do HX, Gosling SN, Grillakis MG, Koutroulis AG, Leonard M, Liu J, Müller Schmied H, Papadimitriou L, Pokhrel Y, Seneviratne SI, Satoh Y, Thiery W, Westra S, Zhang X, Zhao F (2021) Globally observed trends in mean and extreme river flow attributed to climate change. Science 371, 1159-1162.
| Crossref | Google Scholar | PubMed |
Hays GC, Ferreira LC, Sequeira AMM, Meekan MG, Duarte CM, Bailey H, Bailleul F, Bowen WD, Caley MJ, Costa DP, Eguíluz VM, Fossette S, Friedlaender AS, Gales N, Gleiss AC, Gunn J, Harcourt R, Hazen EL, Heithaus MR, Heupel M, Holland K, Horning M, Jonsen I, Kooyman GL, Lowe CG, Madsen PT, Marsh H, Phillips RA, Righton D, Ropert-Coudert Y, Sato K, Shaffer SA, Simpfendorfer CA, Sims DW, Skomal G, Takahashi A, Trathan PN, Wikelski M, Womble JN, Thums M (2016) Key questions in marine megafauna movement ecology. Trends in Ecology & Evolution 31(6), 463-475.
| Crossref | Google Scholar | PubMed |
Hoagstrom CW, Turner TF (2015) Recruitment ecology of pelagic-broadcast spawning minnows: paradigms from the ocean advance science and conservation of an imperilled freshwater fauna. Fish and Fisheries 16(2), 282-299.
| Crossref | Google Scholar |
Humphries P, King A, McCasker N, Kopf RK, Stoffels R, Zampatti B, Price A (2020) Riverscape recruitment: a conceptual synthesis of drivers of fish recruitment in rivers. Canadian Journal of Fisheries and Aquatic Sciences 77(2), 213-225.
| Crossref | Google Scholar |
Jepsen N, Koed A, Thorstad EB, Baras E (2002) Surgical implantation of telemetry transmitters in fish: how much have we learned? Hydrobiologia 483, 239-248.
| Crossref | Google Scholar |
Johnson ES, Bell KP, Leahy JE (2017) Changing course: comparing emerging watershed institutions in river restoration contexts. Society & Natural Resources 30(6), 765-781.
| Crossref | Google Scholar |
Kemp PS (2015) Impoundments, barriers and abstractions. In ‘Freshwater fisheries ecology’. (Ed. JF Craig) pp. 717–769. (Wiley) doi:10.1002/9781118394380.ch52
King AJ, Tonkin Z, Mahoney J (2009) Environmental flow enhances native fish spawning and recruitment in the Murray River, Australia. River Research and Applications 25(10), 1205-1218.
| Crossref | Google Scholar |
Koehn JD, Nicol SJ (2016) Comparative movements of four large fish species in a lowland river. Journal of Fish Biology 88, 1350-1368.
| Crossref | Google Scholar | PubMed |
Koehn JD, Raymond SM, Stuart I, Todd CR, Balcombe SR, Zampatti BP, Bamford H, Ingram BA, Bice CM, Burndred K, Butler G, Baumgartner L, Clunie P, Ellis I, Forbes JP, Hutchison M, Koster WM, Lintermans M, Lyon JP, Mallen-Cooper M, McLellan M, Pearce L, Ryall J, Sharpe C, Stoessel DJ, Thiem JD, Tonkin Z, Townsend A, Ye Q (2020) A compendium of ecological knowledge for restoration of freshwater fishes in Australia’s Murray–Darling Basin. Marine and Freshwater Research 71, 1391-1463.
| Crossref | Google Scholar |
Koster WM, Dawson DR, O’Mahony DJ, Moloney PD, Crook DA (2014) Timing, frequency and environmental conditions associated with mainstem–tributary movement by a lowland River Fish, Golden Perch (Macquaria ambigua). PLoS ONE 9(5), e96044.
| Crossref | Google Scholar | PubMed |
Koster WM, Dawson DR, Liu C, Moloney PD, Crook DA, Thomson JR (2017) Influence of streamflow on spawning-related movements of golden perch Macquaria ambigua in south-eastern Australia. Journal of Fish Biology 90, 93-108.
| Crossref | Google Scholar | PubMed |
Koster WM, Dawson DR, Kitchingman A, Moloney PD, Hale R (2020) Habitat use, movement and activity of two large-bodied native riverine fishes in a regulated lowland weir pool. Journal of Fish Biology 96, 782-794.
| Crossref | Google Scholar | PubMed |
Lechner A, Keckeis H, Humphries P (2016) Patterns and processes in the drift of early developmental stages of fish in rivers: a review. Reviews in Fish Biology and Fisheries 26(3), 471-489.
| Crossref | Google Scholar |
Llewellyn LC (2014) Movements of Golden Perch Macquaria ambigua (Richardson) in the mid Murray and lower Murrumbidgee Rivers (New South Wales) with notes on other species. Australian Zoologist 37(2), 139-156.
| Crossref | Google Scholar |
Marshall JC, Menke N, Crook DA, Lobegeiger JS, Balcombe SR, Huey JA, Fawcett JH, Bond NR, Starkey AH, Sternberg D, Linke S, Arthington AH (2016) Go with the flow: the movement behaviour of fish from isolated waterhole refugia during connecting flow events in an intermittent dryland river. Freshwater Biology 61, 1242-1258.
| Crossref | Google Scholar |
Mollenhauer R, Brewer SK, Perkin JS, Swedberg D, Wedgeworth M, Steffensmeier ZD (2021) Connectivity and flow regime direct conservation priorities for pelagophil fishes. Aquatic Conservation: Marine and Freshwater Ecosystems 31(11), 3215-3227.
| Crossref | Google Scholar |
Moy KG, Wilson GG, Ellison TL (2018) Life history and habitat preference in the Darling hardyhead, Craterocephalus amniculus (Teleostei, Atherinidae) in the northern Murray–Darling Basin, Australia. Austral Ecology 43(4), 476-487.
| Crossref | Google Scholar |
Ogburn DM, Rowland SJ, Mifsud C, Creighton G (1994) Site selection, design and operation of pond-based aquaculture systems. In ‘Silver Perch Culture; Proceedings of Silver Perch aquaculture Workshops’, 11–13 April 1994, Grafton, NSW, Australia; 18–20 April 1994. Narrandera, NSW, Australia. (Eds SJ Rowland, C Bryant) (Austasia Aquaculture: Sandy Bay, Tas., Australia)
Ogburn MB, Harrison A-L, Whoriskey FG, Cooke SJ, Mills Flemming JE, Torres LG (2017) Addressing challenges in the application of animal movement ecology to aquatic conservation and management. Frontiers in Marine Science 4, 70.
| Crossref | Google Scholar |
O’Connor JP, O’Mahony DJ, O’Mahony JM (2005) Movements of Macquaria ambigua, in the Murray River, south-eastern Australia. Journal of Fish Biology 66, 392-403.
| Crossref | Google Scholar |
Perkin JS, Gido KB (2011) Stream fragmentation thresholds for a reproductive guild of Great Plains fishes. Fisheries 36(8), 371-383.
| Crossref | Google Scholar |
Puckridge JT, Walker KF, Costelloe JF (2000) Hydrological persistence and the ecology of dryland rivers. Regulated Rivers: Research & Management 16(5), 385-402.
| Crossref | Google Scholar |
Reynolds LF (1983) Migration patterns of five fish species in the Murray–Darling River system. Marine and Freshwater Research 34, 857-871.
| Crossref | Google Scholar |
Schlaepfer MA, Runge MC, Sherman PW (2002) Ecological and evolutionary traps. Trends in Ecology & Evolution 17(10), 474-480.
| Crossref | Google Scholar |
Shams F, Dyer F, Thompson R, Duncan RP, Thiem JD, Enge TG, Ezaz T (2020) Multiple lines of evidence indicate limited natural recruitment of golden perch (Macquaria ambigua) in the Highly Regulated Lachlan River. Water 12(6), 1636.
| Crossref | Google Scholar |
Sih A (2013) Understanding variation in behavioural responses to human-induced rapid environmental change: a conceptual overview. Animal Behaviour 85, 1077-1088.
| Crossref | Google Scholar |
Stuart IG, Sharpe CP (2020) Riverine spawning, long distance larval drift, and floodplain recruitment of a pelagophilic fish: a case study of golden perch (Macquaria ambigua) in the arid Darling River, Australia. Aquatic Conservation: Marine and Freshwater Ecosystems 30, 675-690.
| Crossref | Google Scholar |
Stuart IG, Sharpe CP, O’Connor J, Lieschke J, Hale R, Fanson B (2024) Escaping the dry: native and non-native fish outmigration from a receding floodplain following managed inundation. Freshwater Biology 69(2), 240-253.
| Crossref | Google Scholar |
Taylor MK, Cooke SJ (2012) Meta-analyses of the effects of river flow on fish movement and activity. Environmental Reviews 20(4), 211-219.
| Crossref | Google Scholar |
Terui A, Urabe H, Senzaki M, Nishizawa B (2023) Intentional release of native species undermines ecological stability. Proceedings of the National Academy of Sciences 120(7), e2218044120.
| Crossref | Google Scholar |
Thiem JD, Wooden IJ, Baumgartner LJ, Butler GL, Taylor MD, Watts RJ (2020) Hypoxic conditions interrupt flood-response movements of three lowland river fish species: implications for flow restoration in modified landscapes. Ecohydrology 13(3), e2197.
| Crossref | Google Scholar |
Thiem JD, Michie LE, Butler GL, Ebner BC, Sharpe CP, Stuart I, Townsend A (2023) A protected flow breaks the drought for golden perch (Macquaria ambigua) spawning along an extensive semi-arid river system. Ecohydrology 16(7), e2576.
| Crossref | Google Scholar |
Wagner GN, Cooke SJ, Brown RS, Deters KA (2011) Surgical implantation techniques for electronic tags in fish. Reviews in Fish Biology and Fisheries 21, 71-81.
| Crossref | Google Scholar |
Wake B (2021) Water wars. Nature Climate Change 11, 84.
| Crossref | Google Scholar |
Wilson G, Spencer JA, Heagney E (2010) Chapter: 7: Responses of fish and waterbirds to flow variability in the Gwydir wetlands. In ‘Ecosystem Response Modelling in the Murray–Darling Basin’. (Eds N Saintilan, I Overton) pp. 103–118. (CSIRO) doi:10.1071/978064310021311.103.118.2010.11
Wood SN (2003) Thin plate regression splines. Journal of the Royal Statistical Society – B. Statistical Methodology 65, 95-114.
| Crossref | Google Scholar |
Wright DW, Zampatti BP, Baumgartner LJ, Brooks S, Butler GL, Crook DA, Fanson BG, Koster W, Lyon J, Strawbridge A, Tonkin Z, Thiem JD (2020) Size, growth and mortality of riverine golden perch (Macquaria ambigua) across a latitudinal gradient. Marine and Freshwater Research 71, 1651-1661.
| Crossref | Google Scholar |
Yarnell SM, Petts GE, Schmidt JC, Whipple AA, Beller EE, Dahm CN, Goodwin P, Viers JH (2015) Functional flows in modified riverscapes: hydrographs, habitats and opportunities. BioScience 65(10), 963-972.
| Crossref | Google Scholar |
Zampatti B, Leigh S (2013) Effects of flooding on recruitment and abundance of Golden Perch (Macquaria ambigua ambigua) in the lower River Murray. Ecological Management & Restoration 14, 135-143.
| Crossref | Google Scholar |
Zampatti BP, Leigh SJ, Bice CM, Rogers PJ (2018) Multiscale movements of golden perch (Percichthyidae: Macquaria ambigua) in the River Murray, Australia. Austral Ecology 43, 763-774.
| Crossref | Google Scholar |
Zuur AF, Ieno EN, Elphick CS (2010) A protocol for data exploration to avoid common statistical problems. Methods in Ecology and Evolution 1, 3-14.
| Crossref | Google Scholar |