Differential impacts of fire and inundation on a wetland plant community after wildfire
K. D. Mackay
A
B
C
D
Abstract
Understanding fire and inundation impacts on wetland vegetation communities is crucial for effective post-fire wetland management.
We aimed to determine the impact of post-fire inundation on plant community structure and seedling germination and establishment after a large wildfire. We asked two questions, namely (1) did fire, drought or inundation affect plant communities the most; and (2) did fire or inundation affect seedling germination and establishment?
Using a before–after–control–impact (BACI) design, we monitored vegetation changes in water-couch wetland communities before and after a wildfire. Also, soil samples were collected from burnt and unburnt sites and assessed for impacts of fire and post-fire inundation regimes on seed germination and seedling establishment.
Inundation variables had more pronounced and more consistent impacts on vegetation measures than did fire or drought variables. Fire impacts were mainly short-term, with impact thresholds at 72 and 143 days after fire. Low germination levels and zero seedling survival were observed without inundation.
Fire was a major but short-term contributor to wetland vegetation change, whereas drought had longer-term impacts, and inundation regimes had the greatest impacts.
Providing a range of inundation conditions post-fire may enhance wetland vegetation recovery and have modifying effects on invasive species.
Keywords: conditional inference tree analysis, managed wetland inundation, post-fire community change, seedling germination, vegetation recovery, wetland fire, wetland flooding following wildfire, wetland seedbank.
Introduction
Inland floodplain wetlands are dynamic, productive and biodiverse ecosystems (Tockner and Stanford 2002) in which the hydrology or inundation regimes play a central role in shaping structure and function (Hughes 1997; McCosker 1999). Other disturbances such as fire, grazing and land-clearing can modify these ecosystems, in particular the composition and structure of wetland vegetation communities (Wilson et al. 2009; Bixby et al. 2015). Following disturbance events, recovery of wetland vegetation communities is heavily influenced by the prevailing climatic conditions and the resultant inundation regime (Whited et al. 2007; Colloff and Baldwin 2010).
Wetland ecosystems, with their high to very high vegetative cover, can be highly susceptible to fire during dry periods (Taufik et al. 2019; Garcia et al. 2021). Despite this, the effect of fire on wetland vegetation is not well understood (Kotze 2013; Bixby et al. 2015). Recovery of wetland vegetation following fire can be influenced by many factors, including the amount of vegetative cover present, the type (wildfire or management burn) and severity of the fire, the antecedent moisture conditions of the wetland, and the post-fire inundation regime (Kirkman 1995; NSW Department of Primary Industries 2009; Kotze 2013). Although wildfire is a natural feature of wetlands, in regulated catchments where the wetlands have environmental water allocations, that water can be managed to support wetland vegetation recovery after the impact of a fire. However, currently there is very little information to guide water managers about the best way to manage water before or after a fire to support recovery. In systems where managed river flows can be used to provide inundation to assist in post-fire wetland recovery, knowledge of the influence of different watering regimes may be critically important for their effective and successful management. Being able to quantify the environmental benefits of utilising limited water resources is becoming increasingly important, because further demands are placed on freshwater systems by agriculture (Boretti and Rosa 2019; Engelbert and Scheuring 2022), making water allocation a contentious issue (Graham 2009; Kosovac et al. 2023).
The Gwydir Wetlands are a biodiverse, highly fertile inland floodplain wetland complex located west of Moree in northern central New South Wales (NSW) (Wilson et al. 2009; Fig. 1). The wetlands, in addition to being internationally recognised under the Ramsar Convention, also support substantial areas of floodplain and wetland vegetation communities that are poorly conserved elsewhere in NSW, including water-couch marsh wetland (also known as water-couch meadows, water-couch marsh grasslands) (Wilson et al. 2009; Bowen and Simpson 2010). Water-couch marsh wetland communities are in decline in the Gwydir Wetlands (K. D. Mackay, unpubl. data). Wetlands have been in decline in Australia and globally for centuries, largely owing to clearing for agriculture but also owing to river regulation and as a consequence of fire (Fluet-Chouinard et al. 2023). In September 2019, a wildfire burnt ~16 km2 of the wetlands (NSW Department of Climate Change, Energy, the Environment and Water 2024), primarily within the Gwydir Wetlands State Conservation Area (SCA) (Fig. 2). This included areas of ‘core wetland’ dominated by water-couch (Paspalum distichum) meadows and vast stands of typha (Typha domingensis). Some fringing coolibah (Eucalyptus coolabah) woodland was also burnt. Fire severity ranged from high to low throughout the fire ground (Department of Planning, Infrastructure and Environment 2019), with available post-fire mapping too coarse to determine the exact severity at the 20 × 20-m plot scale. It was recognised after this fire that there was a knowledge gap in understanding the impacts of fire and post-fire inundation on the various wetland vegetation communities in the Gwydir Wetlands (Vincent et al. 2020) and that filling this gap could help inform effective post-fire management activities.
Location of field sites (‘water couch sites’) within and adjacent to the 2019 fire ground in the Gwydir Wetlands State Conservation Area (SCA) in NSW (inset), Australia. Study plots were spread between 29.2625°S, 149.3548°E and 29.2655°S, 149.3850°E, ~2 km across both sides of the main Gingham water channel.
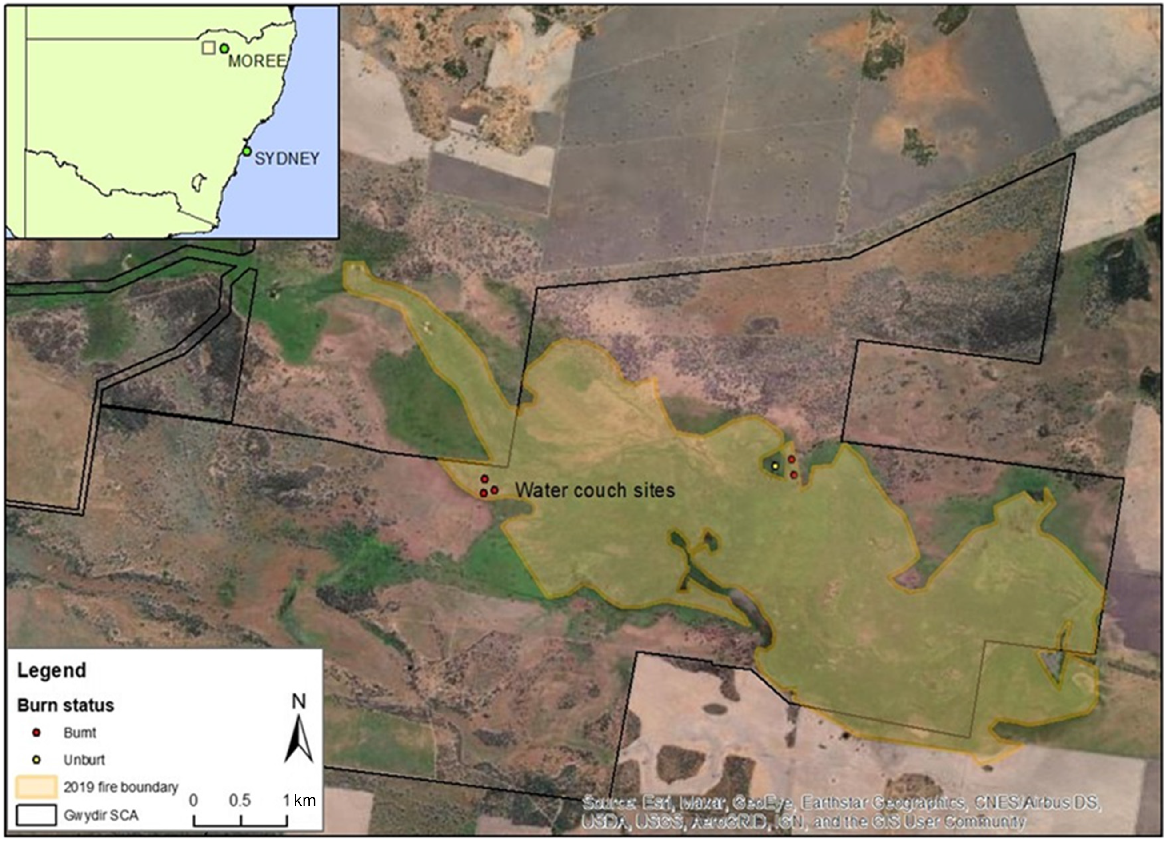
The Gingham fire burning through wetland vegetation (photograph: D. Preston, Department of Planning, Infrastructure and Environment).
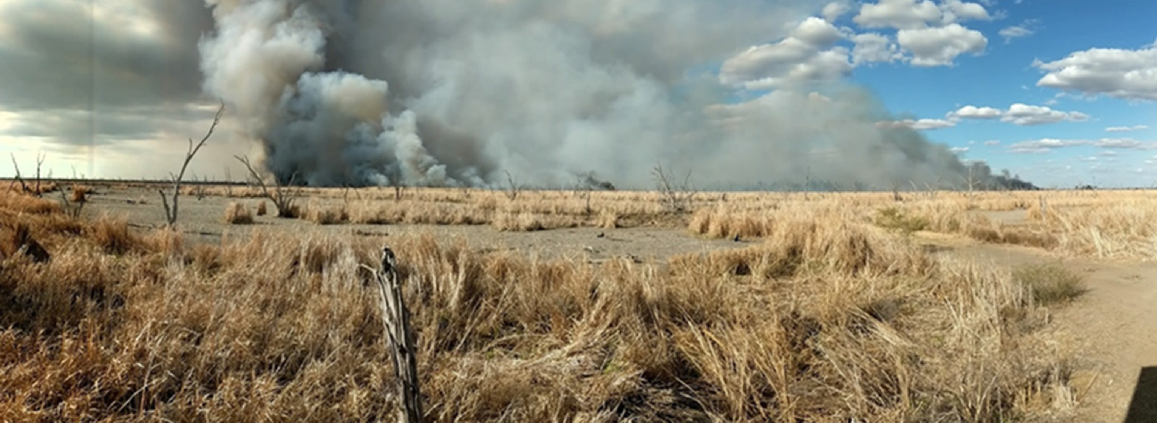
This paper presents the findings from pre- and post-fire vegetation surveys and a seedbank experiment investigating the influence of a single fire event and three inundation regimes on vegetation recovery post-fire. Our primary aims were to measure impacts of fire, drought and inundation on vegetation change within a water-couch marsh wetland community and to determine whether post-fire inundation affected seedling germination and establishment after fire. We asked the following two questions: (1) did fire, drought or inundation have the stronger impact on plant community change; and (2) was there an impact of fire or inundation on seedling germination and establishment? We hypothesised that fire had an impact on vegetation, but that this effect would be relatively short term compared with the impacts of drought and inundation. We further hypothesised that the vegetation would recover from the fire, but that it may change, in terms of floristics or community structure, depending on inundation regimes after the fire.
Study area and methods
This study was conducted within the Gwydir Wetlands State Conservation Area in northern NSW, Australia (Fig. 1). The study plots were located within water-couch (Paspalum distichum, Poaceae) marsh wetland vegetation community. The wildfire around which this study is focused burnt through the Gwydir Wetlands State Conservation Area from 18 September to 21 September 2019 (Fig. 2).
Vegetation survey
Site selection for the vegetation survey and seedbank experiment was dependent on the sites having been previously established and surveyed before the fire, for pre-fire data to be included in analyses. Vegetation surveys were conducted in six 20- × 20-m plots, three plots at each site (Fig. 1). All sites were surveyed once prior to the fire, in March 2018 (Survey time 1), and soon after the fire, in November 2019 (Survey time 2), plus a further six more times approximately every 6 months between November 2019 and October 2023 (Survey times 3–8). Surveys could not be conducted in two of the 6-month periods, owing to the wetland being completely inundated by flooding. Surveys included the identification of all plant species in the plots and the number of individuals of each species (to calculate species diversity), the total number of species per plot (species richness), and the percentage of the 20 × 20 m covered by each species (percentage cover). All species were classified as either native or exotic. To further explain changes in vegetation patterns, individual species were grouped into one of the following four plant functional groups (Casanova 2011): (1) amphibious responders (AmR) – plants that change their growth form in response to flooding and drying cycle, including morphologically plastic (ARp) and floating or stranded (ARf) groups; (2) amphibious tolerators (AmT) – plants that tolerate flooding patterns without changing their growth form, including low growing (ATI) and woody growth form (ATw); (3) terrestrial damp plants (Tda) – plants that are terrestrial species but tend to grow close to the water margin on damp soils; and (4) terrestrial dry plants (Tdr) – plants that are terrestrial species that do not normally grow in wetlands, but may encroach into the area because of prolonged drying. Because of very small sample sizes, the two amphibious groups (AmR and AmT) were combined for analyses and treated as one functional group (Am).
The influence of burning on species richness, cover, diversity, three functional plant groups and assemblage structure was tested using permutational ANOVA (PERMANOVA) (Anderson 2001; Clarke and Gorley 2015). We used a before–after–control–impact (BACI) design to analyse the data. We had one control site that was not burnt, and five impact sites that were burnt in the September 2019 wildfire. Before–after (B-A) and control–impact (C-I) factors were defined as fixed factors and the time factor was defined as a random factor nested within the before–after factor. Bray Curtis dissimilarity was calculated between samples for the assemblage structure and Euclidean distance for univariate variables and used as input into the PERMANOVA analyses. Probabilities of differences between factors were calculated following 9999 permutations of the data. Similarity percentages (SIMPER) was used to identify species that contributed differences in the similarity between burnt and unburnt sites through time. All statistical analyses were conducted in the PERMANOVA+ add on to PRIMER (ver. 7, see https://www.primer-e.com/our-software/; Anderson et al. 2008).
Impacts of time since inundation (the time elapsed since the most recent inundation event ended), inundation duration (the duration of the most recent inundation event), time since drought (using Bureau of Meteorology records, with drought defined as more than 6 months of below-average rainfall each month), drought duration, and time since fire on species richness, cover and diversity were analysed using conditional inference tree analysis using the partykit package (ver. 1.2-20, see https://cran.r-project.org/package=partykit; Hothorn et al. 2006; Winter 2013; Hothorn and Zeileis 2015) in R (ver. 4.3.1, R Foundation for Statistical Computing, Vienna, Austria, see https://www.r-project.org/). All species were analysed together, then native and exotic species were analysed separately, then plant functional groups, namely aquatic species (Am), terrestrial damp species (Tda) and terrestrial dry species (Tdr) (Casanova 2011), were analysed separately.
Three species of particular interest in this study were P. distichum (water couch), E. plana (flat-leaved spike sedge) and J. aridicola (tussock rush). All three species are dominant understorey vegetation elements in water-couch communities and are key species in determining the water-couch–spike-rush–tussock-rush grassy meadows vegetation community within the Gwydir Wetlands. Their response to fire was examined, along with that of the exotic herb, Phyla canescens (lippia, Verbenaceae). Lippia is listed as a serious invasive weed and a key threatening process in Australian wetland communities (Department of Planning, Infrastructure and Environment 2023) and elsewhere in the floodplains of the Murray–Darling Basin (Lucy et al. 1995), which is why it was a fourth species of interest in our study.
Seedbank
Soil samples were collected in October 2020 from three of the six plots in water-couch marshlands where vegetation surveys were conducted (two burnt and one unburnt) plus another burnt and two unburnt plots. Each sampling site was located within similar inundation-frequency zones to minimise the influence of inundation history on seedbank response. At each site, three replicate 20- × 20- × 10-cm soil samples were collected, giving a total of 18 soil samples. Replicate soil samples were separated by ~20–30 m at each site. Soil was disaggregated and left to air dry for at least 2 weeks, before the commencement of the glasshouse experiment.
In the glasshouse, 4-L plastic tubs containing 350 g of soil sample were randomly assigned to a bench space. In each tub, an absorbent fabric cloth was placed into the bottom to aid in water retention, then this was covered by 250 g of sterilised sand and 350 g of soil sample. Each tub was exposed to one of the following three inundation treatments: rainfall, equivalent to 1.0-mm average rainfall per day (estimated from long-term spring rainfall data from Moree (from The Bureau of Meteorology’s Climate Data Online website, see http://www.bom.gov.au/climate/data/, accessed 11 September 2023), applied every 3 days, wetted (soil substrate covered to 1-cm depth) or inundated (soil substrate covered to 10-cm depth). Water levels in wetted and inundated treatments were topped up in tubs every 2–3 days to replace moisture lost by evaporation; i.e. the soil samples in the wetted treatments were maintained as damp to wet and in the inundated treatment as flooded. Soil samples in the rainfall treatment dried out between rainfall applications. Glasshouse temperatures in the climate-controlled glasshouse room were set to emulate average September–October conditions at Moree (7.0–22.5°C; see http://www.bom.gov.au/climate/data/, accessed 11 September 2023). The experiment ran for 8 weeks during summer 2020–21 (1 December 2020–27 January 2021), after which the tubs were harvested and individual plants were counted and identified to species (see Brock et al. 2003). Each species identified in this seedbank study was assigned a pre-determined plant functional group (PFG), i.e. Am, Tda or Tdr (Casanova 2011).
Species richness and abundance (total count of individuals from each species) were calculated in each tub. A multifactor ANOVA with abundance as the dependent variable was used with fire regime (burnt or unburnt) and water regime (inundated or wetted) as main effects. Similarity on species diversity among the treatments was compared using Jaccard’s similarity index.
Results
Vegetation survey
Before the fire, species richness was the same across sites to be burnt (mean = 10 ± 4.7 s.d.; Supplementary Table S1) and the unburnt site (8), as was percentage cover (mean of 100% across the sites to be burnt and 97% in the unburnt site). Two months after the fire, species richness was still the same (8.2 and 8.0 in burnt and unburnt sites) but vegetation cover in the unburnt site was more than triple that across the burnt sites. However, at only 6.2% cover (unburnt) and 2.0% cover (burnt), owing to severe drought conditions, this difference was not significant. Six months later, in autumn 2020, following inundation of the sites in the previous month, species richness had significantly increased in the burnt sites from an average of 8.2 to an average of 21.4 species per plot (P = 0.0003), whereas species richness in the unburnt plot remained low, at 10 species. Vegetation cover in the burnt and unburnt sites had recovered following inundation and was not different between the burnt sites (84%) and unburnt site (94%). By spring 2020, 1 year after the fire and ~7 months after inundation of the sites, species richness had increased further in the burnt plots, to 31 ± 4 species, and was significantly higher than in the unburnt plot (7 species; P = 0.005), but vegetation cover was not different between burnt (97%) and unburnt (100%) plots. Vegetation cover remained the same between burnt (96%) and unburnt (100%) plots in mid-2021, but species richness declined in the burnt plots from 31 ± 4 to 20 ± 3, being still significantly higher than in the unburnt plot (6 species; P = 0.02). Lengthy inundation of ~9-month duration, leading up to the March 2022 survey, led to declines in species richness and cover across all plots, with cover being significantly lower in the unburnt plot in that survey (P = 0.02) and the next, in March 2023 (P = 0.003), whereas species richness was not significantly different between burnt and unburnt plots in the March 2022 and March 2023 surveys. By October 2023, the final survey, species richness and cover had returned to pre-fire levels and were not different between burnt and unburnt plots (10.4 species and 98% cover in burnt plots, 11 species and 97% cover in the unburnt plot).
The fire treatment significantly influenced the overall community structure and the assemblage composition of Am and Tda PFGs, but not Tdr species, as indicated by the significant control–impact (CI) × time (before–after, BA) interaction (Table 1). This interaction indicated that differences between impact and control locations alter when comparisons are made among times.
Source | d.f. | Floristics | Richness | Diversity | Am | Tda | Tdr | |
---|---|---|---|---|---|---|---|---|
Control–impact | 1 | |||||||
Before–after | 1 | |||||||
Time (B-A) | 8 | 3.4*** (29%) | 13.2*** (21%) | 33.7*** (50%) | ||||
C-I × BA | 1 | |||||||
C-I × time (B-A) | 6 | 2.9*** (46%) | 10.5*** (48%) | 7.5*** (26%) |
Only significant results are presented. Probabilities are significant at: ***, P < 0.001.
The burnt and unburnt sites had an 80% similarity in community structure (floristics) prior to the fire but less than 20% following the fire (Fig. 3). By contrast, the two affected PFGs (Am and Tda) at burnt and unburnt sites were not similar prior to the fire, and the similarity varied post-fire (Fig. 3).
Similarity of community structure and Am and Tda PFGs between burnt and unburnt sites through time. Times (1–8) are the eight survey times described in the methods.
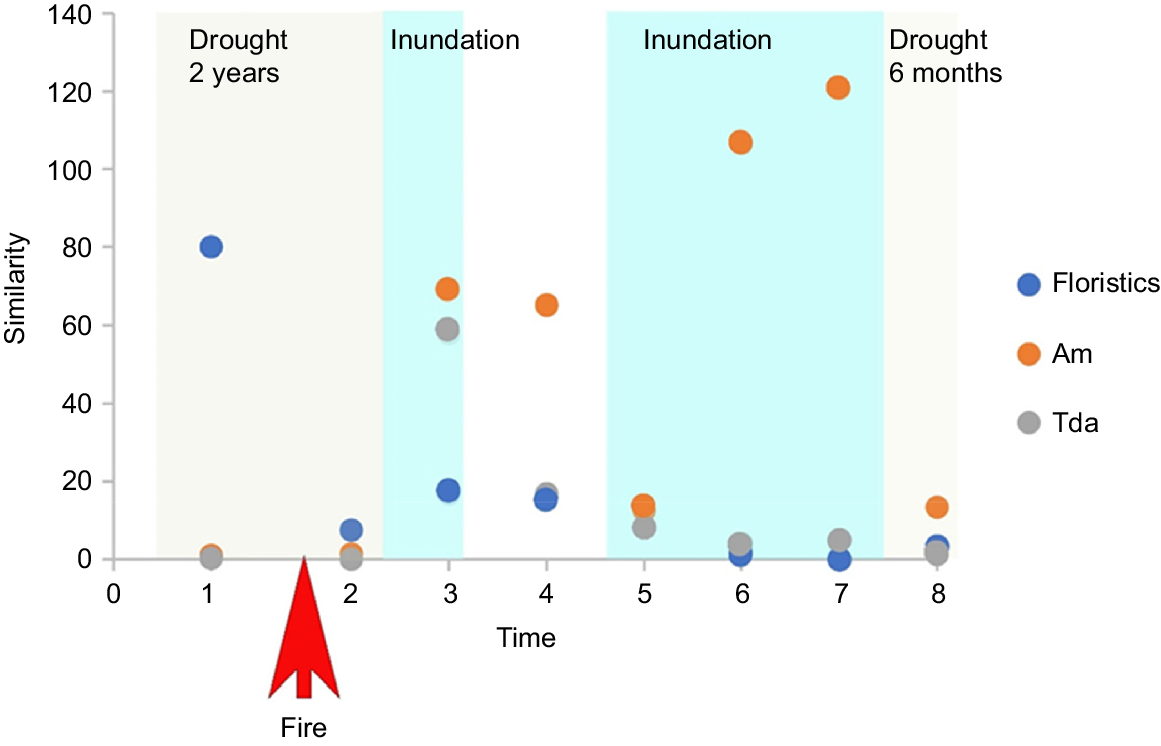
In the inference-tree analysis, we found that inundation variables, namely, time since inundation and inundation duration, had more substantial and consistent impacts on the measured vegetation variables of species richness, cover and diversity than did fire and drought variables (Table 2). Fire impacts were short term, with impact thresholds at 72 and 143 days after fire. Droughts had longer-term impacts, with thresholds identified at 91 and 487 days after drought (Table 2). Most vegetation measures were affected by only one or two factors (Table 2). Exotic richness (the number of weed species recorded within a 20- × 20-m plot) was affected by a more complex array of factors, presented in Fig. 4. Exotic species richness was the highest when the time since the last inundation was more than 466 days. Exotic species richness was the lowest when time since inundation was less than or equal to 466 days, inundation duration was more than 15 days, time since fire was more than 72 days, and time since drought was more than 487 days.
Variable | Factor(s) associated with the highest level of variable | Factor(s) associated with the lowest level of variable | |
---|---|---|---|
All species richness | Greatest if TSI > 205 days | Least if TSI ≤ 205 days | |
All species cover | Greatest if DD ≤ 608 days | Least if DD > 608 days | |
All species diversity | Greatest if TSF ≤ 72 days | Least if TSF > 72 days | |
Native richness | Greatest if TSI > 205 days and DD < 213 days | Least if TSI ≤ 205 days | |
Native cover | Greatest if DD ≤ 608 days | Least if DD > 608 days | |
Native diversity | Nil | ||
Exotic richness A | Greatest if TSI > 466 days | Least if TSI ≤ 466 days, ID > 15 days, TSF > 72 days, and TSD > 487 days | |
Exotic cover | Nil | ||
Exotic diversity | Greatest if TSI > 466 days | Least if ID > 75 days and TSI ≤ 45 days | |
Am richness | Greatest if TSF ≤ 143 days | Least if TSF > 143 days | |
Am cover | Greatest if DD ≤ 608 days | Least if DD > 608 days | |
Am diversity | Greatest if TSF ≤ 143 days | Least if TSF > 143 days | |
Tda richness | Greatest if TSI > 466 days | Least if TSI ≤ 466 days, ID > 15 days, and TSF > 72 days | |
Tda cover | Nil | ||
Tda diversity | Greatest if TSI > 466 days | Least if TSI ≤ 466 days, and ID > 75 days | |
Tdr richness | Greatest if TSI > 205 days | Least if TSI ≤ 205 days, and TSD > 91 days | |
Tdr cover | Greatest if TSI > 208 days | Least if TSI ≤ 208 days, TSD > 91 days and TSI ≤ 10 days | |
Tdr diversity | Greatest if TSI > 466 days | Least if TSI ≤ 466 days |
Factors are time since inundation (TSI), inundation duration (ID), time since drought (TSD), drought duration (DD) and time since fire (TSF).
Results of conditional inference-tree analysis of the significant factors (circled) affecting on exotic richness, with thresholds (days) written below the relevant factors. Box and whisker plots show median values, upper and lower quartiles and maximum and minimum values for exotic richness resulting from the outlined factors above and below the specified thresholds.
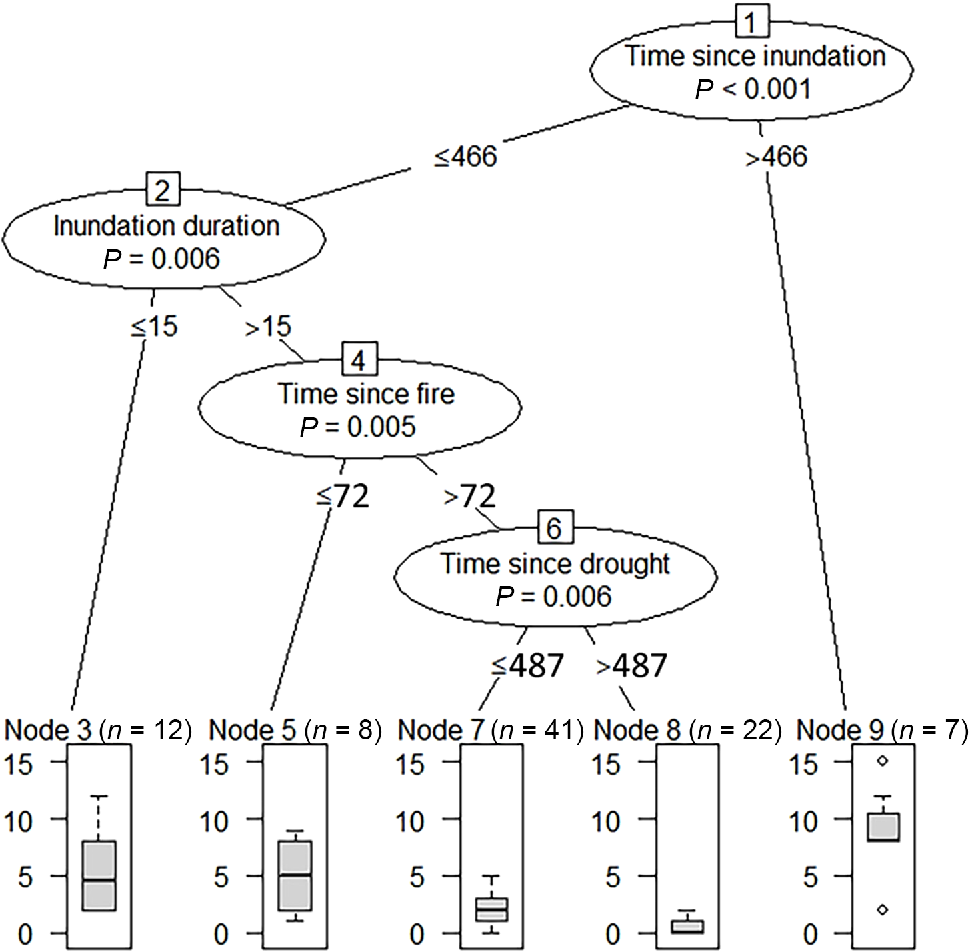
The species indicated by SIMPER to contribute the most to the differences between burnt and unburnt sites were the marshland plants P. distichum (water couch), E. plana (flat-leaved spike sedge) and J. aridicola (tussock rush) and Ludwigia peploides (water primrose) (Table 3). Water-couch cover rebounded after the drought in the burnt sites but not in the unburnt sites (Kruskal–Wallis F = 8.1, P = 0.004), and no effect of fire on water-couch abundance was observed in the seedbank experiment. Flat-leaved spike sedge increased in cover after fire, but not significantly (average cover in burnt sites = 7.5%, 1.5% in unburnt sites), and did not respond to fire treatment in the seedbank experiment, only to watering regime with more spike sedge in the inundated than wetted treatments (F = 5.27, P = 0.03). Tussock rush occurred in too few sites post-fire to determine any differences in the fire response, and nor did it respond to the fire or watering treatments in the seedbank experiment.
Survey time and species | Percentage cover at impact site | Percentage cover at control site | |
---|---|---|---|
T1 | |||
Paspalum distichum (AmR) | 79.66 | 98.3 | |
Eleocharis plana (AmT) | 8.28 | 0 | |
Juncus aridicola (AmT) | 7.06 | 0 | |
T2 | |||
Glinus lotoides (Tdr) | 0 | 3 | |
Ludwigia peploides (AmR) | 0.24 | 2 | |
Eleocharis sphacelata (AmT) | 0 | 1 | |
T3 | |||
Ludwigia peploides (AmR) | 10.01 | 87 | |
Echinochloa colona (Tda) | 55 | 3 | |
T4 | |||
Ludwigia peploides (AmR) | 13.33 | 80 | |
Eleocharis plana (AmT) | 23.25 | 3 | |
Paspalum distichum (AmR) | 18.75 | 1 | |
Azolla rubra (AmR) | 0.13 | 15 | |
T5 | |||
Ludwigia peploides (AmR) | 6.06 | 55 | |
Azolla rubra (AmR) | 2.04 | 45 | |
Paspalum distichum (AmR) | 30.42 | 0.01 | |
Juncus aridicola (AmT) | 20.2 | 0 | |
T6 | |||
Paspalum distichum (AmR) | 62.4 | 1 | |
Juncus aridicola (AmT) | 19.22 | 0 | |
T7 | |||
Paspalum distichum (AmR) | 73 | 0 | |
Typha domingensis (AmT) | 29 | 0 | |
T8 | |||
Persicaria orientalis (AmT) | 0.1 | 70 | |
Paspalum distichum (AmR) | 40 | 0 | |
Typha domingensis (AmT) | 31 | 0 |
Values are average percentage cover.
Lippia abundance and cover increased greatly in response to fire, from 20 plants (0.1% cover) in only one of the five sites later burnt, to occurring in all burnt sites in numbers ranging from 2 plants to 10,000 plants (0.05–10% cover) 1 year after fire. Lippia numbers declined again in the second and subsequent years after fire, following inundation of the sites in the second and third years after the fire, with inundation known to greatly reduce abundance and cover of this weed (Price et al. 2010, 2011).
Seedbank experiment
Seeds successfully germinated in all treatments. However, in the rainfall-treated tubs, seedling establishment was poor and all seedlings in these tubs died before the end of the 8-week germination trial. Therefore, all further results and analyses pertain to only the wetted and inundated treatments across the burnt and unburnt soil seedbank samples.
In total, 5319 seedlings belonging to 28 species (22 native, 5 exotic, 1 unknown) from 17 families were recorded. Species richness did not vary significantly among the treatments (range 14–18 species). Cyperus difformis was the most common species found, occurring in 100% of seedbank tubs, followed by Eleocharis plana (68%), Myriophyllum crispatum (63%), Juncus usitatus (58%) and Echinochloa colona (53%). Significantly more seedlings were recorded in the inundated water treatment than in the wetted treatment. There were no other significant results involving fire regime or interaction (Fig. 5, Table 4).
Mean abundance ± s.e.m. of seedlings from the glasshouse experiment with the treatments inundated and wetted applied to soil seedbank samples from burnt and unburnt sites.
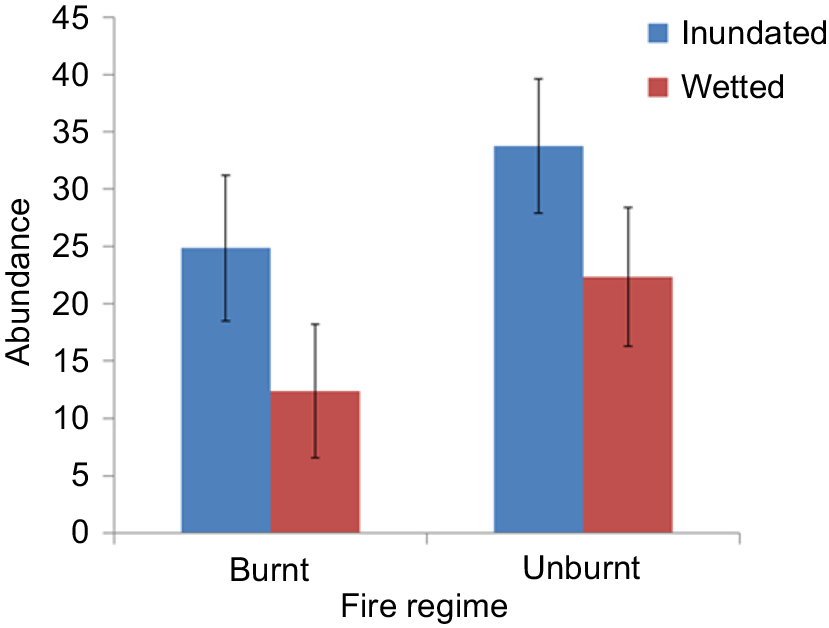
Source | Sum of squares | d.f. | Mean square | F-ratio | P-value | |
---|---|---|---|---|---|---|
Main effects | ||||||
Fire regime | 5039.31 | 1 | 5039.31 | 2.45 | 0.1193 | |
Water regime | 8069.73 | 1 | 8069.73 | 3.92 | 0.0491 | |
Interactions | ||||||
Fire regime × water regime | 13.9213 | 1 | 13.9213 | 0.01 | 0.9346 | |
Residual | 461,645 | 224 | 2060.92 | |||
Total (corrected) | 475,612 | 227 |
All F-ratios are based on the residual mean square error.
Germination trials resulted in different community structures (floristics) among fire regimes and water treatments (39–61% similarities). Species composition was most similar (61% similarity) between sites that were burnt and inundated, unburnt and inundated and burnt and wetted. Species composition was most dissimilar (39% similarity) between sites that were burnt and inundated and sites that were unburnt and wetted.
Discussion
Fire and drought both affected vegetation change, but the impacts of fire appeared to be short term (<1 year) compared with those of drought (>1 year). Inundation factors (timing between and duration of inundation events) had longer-term impacts on vegetation change and these impacts had higher significance (lower P-values) in the results for almost all aspects of species richness, cover and diversity in our vegetation plots than did the impacts of fire or drought. Fire and post-fire inundation had significant impacts on seedling germination and growth, as shown in other studies (e.g. Kirkman 1995; Kotze 2013). Our results indicated that average rainfall alone is not sufficient to support seedling germination and establishment in these wetland communities after fire and that inundation after fire is required to recover this plant community in the short term and potentially to sustain it in the longer term. Above-average rainfall was not tested in this study but could potentially support seedling establishment and could be tested in future studies.
Different species responded differently to the fire treatments, including the three species noted in the methods section to be of particular importance in the water-couch–spike-sedge–tussock-rush grassy meadows vegetation community. Our findings that these dominant plant species were less abundant in burnt versus unburnt vegetation plots are consistent with the results of other studies that have shown that when soil moisture levels are low in wetland soils, fire can consume seeds or reduce seed viability, which can result in changes in community composition and structure (Austin et al. 2007; NSW Department of Primary Industries 2009). Inconsistent findings for some species and the non-significant results for water-couch seedling abundances in burnt versus unburnt plots in the seedbank study suggest that either fire severity varied across the fireground, or differed among vegetation types, or that there may be additional factors aside from fire, driving species composition, including inundation regimes, durations and timing (e.g. see Capon 2007).
Water couch and tussock rush showed a higher abundance in the wetted treatment, whereas flat-leaved spike sedge showed a greater abundance in the inundated treatment. These differing responses are possibly related to each species’ functional preferences, with the spike sedge and tussock rush being recognised as amphibious fluctuation tolerators and water couch an amphibious fluctuation responder (Casanova 2011). In a practical sense, these results suggest that no one inundation treatment favoured all key wetland vegetation species during this study, so a ‘one size fits all’ approach to watering after fire is not likely to promote all species equally. Providing a range of inundation conditions post-fire may enhance wetland vegetation seedbank response. For example, the efficacy of instituting a range of conditions may be improved by accommodating differential responses by different plant functional groups in inundation treatments (Keith et al. 2007).
Earl (2003) reported that lippia is depressed under semi-regular flooding regimes of >20-cm water depth. Price et al. (2010) showed that dry conditions favour the growth of the invader and wetter conditions favour the native water couch and that the dominance of lippia is governed by altered disturbance regimes, which give the invader a competitive advantage over the native species. We found that it increased in numbers and percentage cover in the vegetation study after the fire, but only in burnt sites, and only for the first year post-fire, before declining again to pre-fire (and lower) levels of abundance and cover.
Similar patterns in mean species richness and abundance among vegetation communities, to those observed in our results, have been noted in long-term field data collected in the Gwydir Wetlands (Commonwealth of Australia 2020). However, species richness recorded in the seedbank trial was approximately half that seen in our vegetation surveys of the same plots, or in the long-term data. Many floodplain species have specific germination requirements (Capon 2003) and the reduced mean species richness values observed in our glasshouse seedbank trial suggest that specific germination requirements for many species may not have been met in the trial. Inundation duration, for example, has been shown to affect germination success in many species (Casanova and Brock 2000), and the retreat of water after inundation is necessary for germination in many species (Nicol et al. 2003). The timing of the seedbank sampling, in October 2020, was some time after the fire, and this may have influenced differences observed in germinations between the burnt and unburnt sites. Further trials including in situ germination trials in multiple localities should be conducted to test the findings and conclusions from our experiment and in situ observations.
Conclusions
Inundation influenced species richness, species abundances and plant community composition in water-couch marsh grasslands. Our study indicated that both fire and the inundation regime employed following fire can influence wetland vegetation-community structure. Providing a range of inundation conditions post-fire may enhance the wetland vegetation seedbank response. This is also likely to have modifying effects on invasive species. It is recommended that further research on the effects of fire on wetland vegetation consider frequency, duration and timing of inundation events after fires, and that inundation events be managed to counter negative effects of droughts as well as fires that often burn in response to drought conditions.
Data availability
The data that support this study belong to the University of New England (UNE). Data will be shared upon reasonable request to the corresponding author with permission from UNE.
Conflicts of interest
S. Mika is a guest editor of this collection for Marine and Freshwater Research, but did not at any stage have editor-level access to this manuscript while in peer review, as is the standard practice when handling manuscripts submitted by an editor to this journal. Marine and Freshwater Research encourages its editors to publish in the journal and they are kept totally separate from the decision-making processes for their manuscripts. The authors have no further conflicts of interest to declare.
Declaration of funding
Funding for this study was provided by the Commonwealth Environmental Water Holder under the Long Term Intervention Monitoring (LTIM) project (July 2014–June 2019; funding provided to Ecological Australia consulting company and the NSW Office of Environment and Heritage, now the Department of Climate Change, Energy, the Environment and Water) and the Flow-Monitoring, Evaluation and Research (Flow-MER) project (July 2019–June 2024; funding provided to the University of New England and 2Rog Consulting).
Acknowledgements
We acknowledge the Kamilaroi-Gamilaraay traditional owners of the Country on which we worked. We acknowledge the Commonwealth Environmental Water Office (now the Commonwealth Environmental Water Holder) for their funding of the Gwydir Monitoring, Evaluation and Research (MER) project, through which this work was undertaken. We also thank the NSW National Parks and Wildlife Service for allowing access to the Gwydir Wetlands SCA. Zachary Lewis and staff from the NSW Department of Planning and Environment are thanked for help with the fieldwork. C. L. Gross (UNE) is thanked for comments on an earlier draft and for statistical advice.
References
Anderson MJ (2001) A new method for non-parametric analysis of variance. Austral Ecology 26(1), 32-46.
| Crossref | Google Scholar |
Austin JE, Keough JR, Pyle WH (2007) Effects of habitat management treatments on plant community composition and biomass in a Montane Wetland. Wetlands 27(3), 570-587.
| Crossref | Google Scholar |
Bixby RJ, Cooper SD, Gresswell RE, Brown LE, Dahm CN, Dwire KA (2015) Fire effects on aquatic ecosystems: an assessment of the current state of the science. Freshwater Science 34, 1340-1350.
| Crossref | Google Scholar |
Boretti A, Rosa L (2019) Reassessing the projections of the world water development report. npj Clean Water 2, 15.
| Crossref | Google Scholar |
Brock MA, Nielsen DL, Shiel RJ, Green JD, Langley JD (2003) Drought and aquatic community resilience: the role of eggs and seeds in sediments of temporary wetlands. Freshwater Biology 48, 1207-1218.
| Crossref | Google Scholar |
Capon SJ (2003) Plant community responses to wetting and drying in a large arid floodplain. River Research and Applications 19, 509-520.
| Crossref | Google Scholar |
Capon SJ (2007) Effects of flooding on seedling emergence from the soil seed bank of a large desert floodplain. Wetlands 27, 904-914.
| Crossref | Google Scholar |
Casanova M (2011) Using water plant functional groups to investigate environmental water requirements. Freshwater Biology 56, 2637-2652.
| Crossref | Google Scholar |
Casanova MT, Brock MA (2000) How do depth, duration and frequency of flooding influence the establishment of wetland plant communities? Plant Ecology 147, 237-250.
| Crossref | Google Scholar |
Colloff MJ, Baldwin DS (2010) Resilience of floodplain ecosystems in a semi-arid environment. The Rangeland Journal 32, 305-314.
| Crossref | Google Scholar |
Department of Planning, Infrastructure and Environment (2023) Key threatening process nomination form – for adding a threatening process under the Environment Protection and Biodiversity Conservation Act 1999 (EPBC Act). (DPIE) Available at https://www.dcceew.gov.au/sites/default/files/env/pages/87ef6ac7-da62-4a45-90ec-0d473863f3e6/files/nomination-expansion-of-the-weed-lippia.pdf [Verified 12 September 2023]
Fluet-Chouinard E, Stocker BD, Zhang Z, Malhotra A, Melton JR, Poulter B, Kaplan JO, Goldewijk KK, Siebert S, Minayeva T, Hugelius G, Joosten H, Barthelmes A, Prigent C, Aires F, Hoyt AM, Davidson N, Finlayson CM, Lehner B, Jackson RB, McIntyre PB (2023) Extensive global wetland loss over the past three centuries. Nature 614(7947), 281-286.
| Crossref | Google Scholar |
Garcia LC, Szabo JK, de Oliveira Roque F, de Matos Martins Pereira A, Nunes da Cunha C, Damasceno-Júnior GA, Morato RG, Tomas WM, Libonati R, Ribeiro DB (2021) Record-breaking wildfires in the world’s largest continuous tropical wetland: integrative fire management is urgently needed for both biodiversity and humans. Journal of Environmental Management 293, 112870.
| Crossref | Google Scholar | PubMed |
Graham S (2009) Irrigators’ attitudes towards environmental flows for wetlands in the Murrumbidgee, Australia. Wetlands Ecology and Management 17, 303-316.
| Crossref | Google Scholar |
Hothorn T, Zeileis A (2015) partykit: a modular toolkit for recursive partytioning in R. Journal of Machine Learning Research 16, 3905-3909.
| Google Scholar |
Hothorn T, Hornik K, Zeileis A (2006) Unbiased recursive partitioning: a conditional inference framework. Journal of Computational and Graphical Statistics 15(3), 651-674.
| Crossref | Google Scholar |
Hughes FMR (1997) Floodplain biogeomorphology. Progress in Physical Geography: Earth and Environment 21, 501-529.
| Crossref | Google Scholar |
Keith DA, Holman L, Rodoreda S, Lemmon J, Bedward M (2007) Plant functional types can predict decade-scale changes in fire-prone vegetation. Journal of Ecology 95(6), 1324-1337.
| Crossref | Google Scholar |
Kirkman LK (1995) Impacts of fire and hydrological regimes on vegetation in depression wetlands of southeastern USA. In ‘Fire in wetlands: a management perspective. Proceedings of the 19th Tall Timbers Fire Ecology Conference’, 3–6 November 1993 Tallahassee, FL, USA. (Eds SI Cerulean, RT Engstrom) pp. 10–20. (Tall Timbers Research Station)
Kosovac A, Horne AC, O’Donnell E (2023) Community perceptions of environmental water: a review. Environmental Conservation 50, 73-82.
| Crossref | Google Scholar |
Kotze DC (2013) The effects of fire on wetland structure and functioning. African Journal of Aquatic Science 38, 237-247.
| Crossref | Google Scholar |
Nicol JM, Ganf GG, Pelton GA (2003) Seed banks of a southern Australian wetland: the influence of water regime on the final floristic composition. Plant Ecology 168, 191-205.
| Crossref | Google Scholar |
NSW Department of Climate Change, Energy, the Environment and Water (2024) NPWS fire history – wildfires and prescribed burns. Available at https://datasets.seed.nsw.gov.au/dataset/fire-history-wildfires-and-prescribed-burns-1e8b6 [Verified 4 February 2024]
Price J, Gross CL, Whalley W (2010) Prolonged summer flooding switched dominance from the invasive weed lippia (Phyla canescens) to native species in one small, ephemeral wetland. Ecological Management & Restoration 11, 61-63.
| Crossref | Google Scholar |
Price JN, Berney PJ, Ryder D, Whalley RDB, Gross CL (2011) Disturbance governs dominance of an invasive forb in a temporary wetland. Oecologia 167, 759-769.
| Crossref | Google Scholar | PubMed |
Taufik M, Setiawan BI, Van Lanen HAJ (2019) Increased fire hazard in human-modified wetlands in Southeast Asia. Ambio 48, 363-373.
| Crossref | Google Scholar | PubMed |
Tockner K, Stanford JA (2002) Riverine flood plains: present state and future trends. Environmental Conservation 29, 308-330.
| Crossref | Google Scholar |
Vincent B, Southwell M, Growns I, Lewis Z (2020) Wetland wildfire: seedbank response to different watering regimes. In ‘Proceedings of the 10th Australian Stream Management Conference 2020’, Kingscliff, NSW, Australia. (River Basin Management Society) Available at https://rbms.tempurl.host/wp-content/uploads/2021/08/45-ben-e-vincent.pdf
Whited DC, Lorang MS, Harner MJ, Hauer FR, Kimball JS, Stanford JA (2007) Climate, hydrologic disturbance, and succession: drivers of floodplain pattern. Ecology 88, 940-953.
| Crossref | Google Scholar | PubMed |
Wilson GG, TO Bickel, PJ Berney, Sisson JL (2009) Managing environmental flows in an agricultural landscape: the Lower Gwydir floodplain. Final Report from University of New England and Cotton Catchment Communities Cooperative Research Centre, Armidale, NSW, Australia. p. 173. Australian Government Department of the Environment, Water, Heritage and the Arts.
Winter B (2013) A very basic tutorial for performing linear mixed effects analyses. (University of California—Merced, Cognitive and Information Sciences: Merced, CA, USA) Available at https://bodowinter.com/tutorial/bw_LME_tutorial2.pdf