An improved method of capture and immobilisation for medium to large-size macropods
Miguel A. Bedoya-Pérez
A
B
C
D
E
F
G
H
Abstract
Macropods are very susceptible to stress during capture. Capture methods for macropods fall into two categories: trapping and darting. Trapping by nets or a triggered trap mechanism is commonly used for small macropods. Darting is most often used for large macropods that are more prone to stress and capture myopathy when caught in traps.
To describe a modified ‘nylon drop-net’ technique for safely capturing medium to large macropods; and post-capture treatments that reduce stress and the potential for myopathy.
We used a drop-net to capture 40 agile wallabies (Notamacropus agilis) (24 females and 16 males), ranging in weight from 6 to 24 kg. For immobilisation, a single dose of intramuscular Diazepam (1 mg/kg) and Richtasol, a multivitamin, was administered to reduce the risk of capture myopathy. The longer-term effects of capture on animal condition were monitored in 34 radio-collared individuals for 2 months.
No deaths occurred during or as a result of capture or in the 8 weeks following capture.
Our modified drop-net and handling/treatment regime provides a cost-effective method for capturing medium and small-sized macropod species with very low risk of mortality or morbidity.
Our methods improve the welfare and safety of captured medium-sized macropods.
Keywords: agile wallaby, capture stress, drop-net capture method, live-capture, macropods, medium-sized macropod, muscle relaxant, myopathy.
Introduction
Capture and restraint are often a necessary prequel to studying wild animals to obtain information on their physiological parameters, movement, and realised niche (Tarszisz et al. 2014; Blumstein et al. 2015; Breed et al. 2019). How an animal is captured and restrained affects its welfare during and post-capture. Minimising capture-related injuries and conditions such as capture-induced myopathy is integral to any successful capture method.
Capture-induced myopathy is a response to excessive stress and exertion (Wallace et al. 1987; Breed et al. 2019), where damage to the skeletal and cardiac muscles occurs after pursuit, capture, holding, handling and manipulation of an animal (Blumstein et al. 2015). Fatalities can occur during capture and restraint or after release (Breed et al. 2019). Capture myopathy has been described for many taxa, including birds (Rogers et al. 2004), reptiles (Phillips et al. 2015), and mammals (Braud et al. 2019), but in particular in ungulate species (Meyer et al. 2008a; Blumstein et al. 2015; Ali et al. 2023). Macropods appear similarly susceptible to stress-related capture myopathy (Shepherd et al. 1988; Vogelnest and Woods 2008; McMahon et al. 2013; Green-Barber et al. 2018). Clinical signs are severe and range from lethargy and weakness to ataxia, paresis and death (West et al. 2014; Breed et al. 2019), with laboratory findings of myoglobinuria (leading to an acute kidney injury), metabolic acidosis and increased blood cortisol concentration (Meyer et al. 2008b; Breed et al. 2019), the combination of which leads to fatalities.
In general, non-lethal wildlife studies aim to use the most effective capture methods with minimal or no capture-related mortalities. While the occurrence and severity of capture myopathy have been investigated in several macropod species (Latham et al. 2020), little information is available for medium-sized wallabies such as the agile wallaby (Notamacropus agilis) (see Latham et al. (2020)). The agile wallaby is a species in decline, which inhabits the seasonally wet-dry tropical savannas of northern Australia and the Indonesian province of Papua and Papua New Guinea (Stirrat 2008; Aplin et al. 2013; Hunt et al. 2021). Agile wallabies occupy various habitats, including savanna woodland, mangrove, open, riparian, and dense monsoon rain forest (Stirrat 2000; Hunt et al. 2021). High densities occur on regenerating grasslands and the woody margins of paddocks and riparian vegetation in northern Australia (Stirrat 2000; Hunt et al. 2021). While not declining at a rate considered threatening (Aplin et al. 2013), the agile wallaby is a potential an indicator species, as it prefers ecotone areas within a mix of habitats (Hunt et al. 2021), and population change is indicative of severe habitat disturbance across broad areas of ecological importance.
We captured 40 agile wallabies using a modified ‘nylon drop-net’ technique previously developed to capture ungulates (Kock et al. 1987; Mdetele et al. 2021). Here, we present our experience with this modified trapping method and the steps we took when handling the wallabies to reduce the risk of capture myopathy.
Materials and methods
Study species
The agile wallaby is sexually dimorphic, with males weighing 16–27 kg and females 9–15 kg. It is generally solitary but forms groups when feeding on pastures or open ground. Breeding occurs at any time of the year, and in agricultural environments, agile wallaby populations can double in size every 2–3 years (Stirrat 2008).
Study area
The study was conducted on the Douglas Daly Research Farm (DDRF; 13°50′S, 131°11′E) in the Northern Territory, Australia. The DDRF is a 3100-ha agricultural research facility managed and owned by the Northern Territory Government since the 1960s (Shotton 2011). Currently, the property has approximately 2500 ha of cleared land, mainly for improved pastures, wet season cattle fodder, and some grain crops, focusing primarily on research related to cattle production (Shotton 2011). Agile wallaby densities on the farm are high, i.e. 205 individual per km2 (95% CI 170–247), especially on the improved pastures (Bedoya-Pérez et al. 2017). The region is characterised by rainfall seasonality: a wet season from October to April (Mean annual rainfall = 1207 mm) and a dry season from May to September, receiving little or no rain (Shotton 2011). Temperatures range from 13 to 37°C, and mean monthly maximum temperatures range from 31 to 37°C (Shotton 2011).
Capture and collaring
From 25 March 2016 to 30 May 2016, 40 agile wallabies were caught, and 34 were fitted with a very high frequency (VHF) collar (V5C 163E 130–260 mm VHF Std Collar Sirtrack Ltd). Across two areas of the DDRF, we established 17 capture locations, each of which was separated by at least 2.5 km. This gave us eight capture locations around the farm homestead and nine in grazing paddocks (Fig. 1). The longer-term effects of capture on animal condition were monitored daily at midday, dusk, midnight and dawn in radio-collared individuals during four 7-day tracking periods from 13 June 2016 to 21 July 2016.
A map of seventeen drop-net locations at the Douglas Daly Research Farm in two different areas: around the homestead on the west part of the farm and on grazing paddocks on the east side of it. Both areas are within 2 km of the Douglas River that fringes the north of the farm.
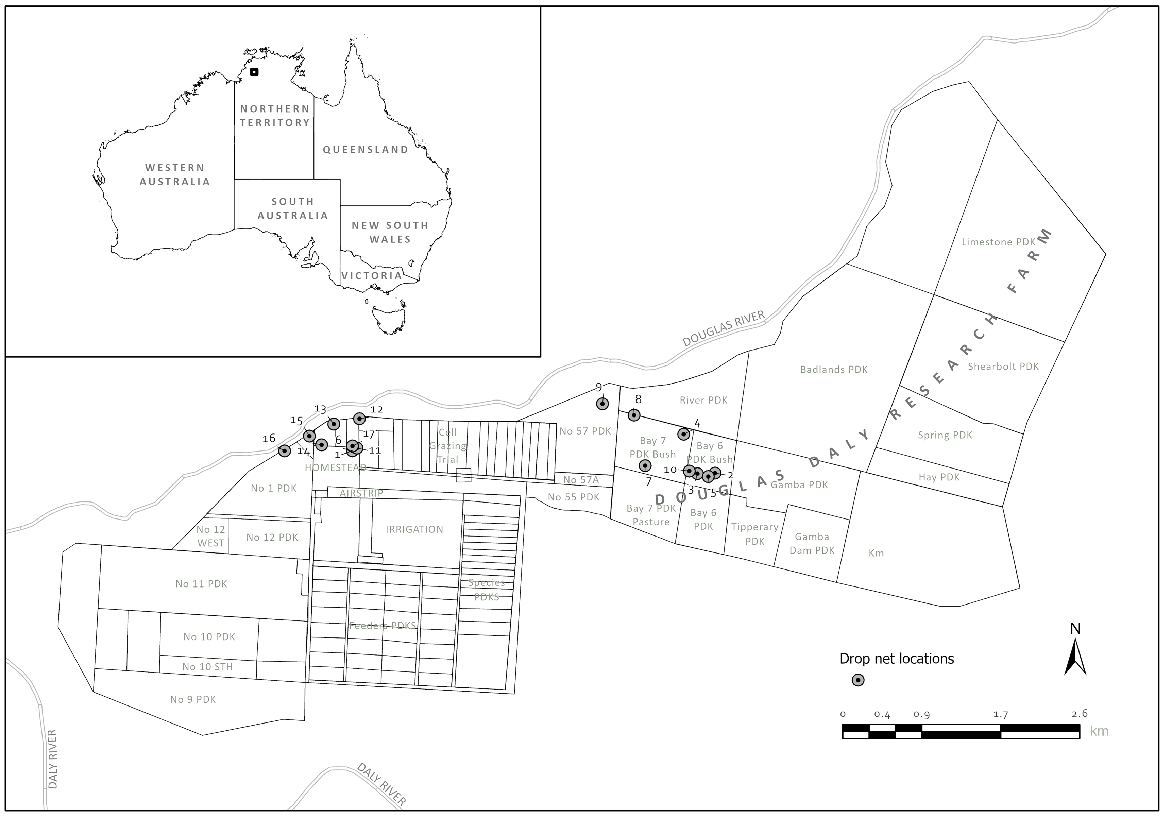
We used a ‘nylon drop-net’ technique originally used to capture ungulates (Kock et al. 1987; Mdetele et al. 2021). The technique was modified by wildlife management practitioners ABS Scrofa Pty Ltd, partners in this study. To our knowledge, only one study describes a nylon drop-net technique for capturing macropods (Lentle et al. 1997). Lentle et al. (1997) describe a method in which operators are required to lower the net manually to ‘close’ the trap, while our method relies on the animal hitting the net and triggering the fall of the net, as described by Mdetele et al. (2021). In our method, we created a ‘funnel’ to guide the animals towards the net, where only one animal per trapping attempt was captured by entanglement. Additionally, in contrast with Mdetele et al. (2021), we did not use vehicles to ‘herd’ the animals towards the net, but instead used people walking at a constant pace to ‘flush’ animals towards the net.
Further details of our method are as follows: The monofilament nylon drop-nets, approximately 20 m long, with 117 mm mesh size and 2 m high, were suspended between 2 m tall fence pickets driven into the ground on a slight angle (70°) in the direction the wallabies were expected to arrive from (Fig. 2a). The fence pickets were placed every 3–4 m across potential wallaby escape routes, e.g. fence lines and obviously used pads (Fig. 2b). Hessian fabric wings (2 m high) were sometimes added to the sides of some of the nets to funnel animals toward the drop-net (Fig. 2c). The top edge filament of the net was attached to the fence picket by light wire hooks. Before the start of a capture session, the bottom of the drop-net was aligned with the bottom of the fence pickets, folding the mesh to form a ‘pocket’ that opened towards where the wallabies were expected to enter the net. Once an animal hits the net, the impact releases the net from the light wire hooks, trapping the animal and allowing other wallabies to jump over the fallen net. In this way, only one wallaby is trapped at a time.
Drop net set up before the catching session. The net in (a), (b) and (d) is completely suspended to prevent animals from getting caught outside a catching session. A hessian fabric wing was used to funnel animals towards the net (c).
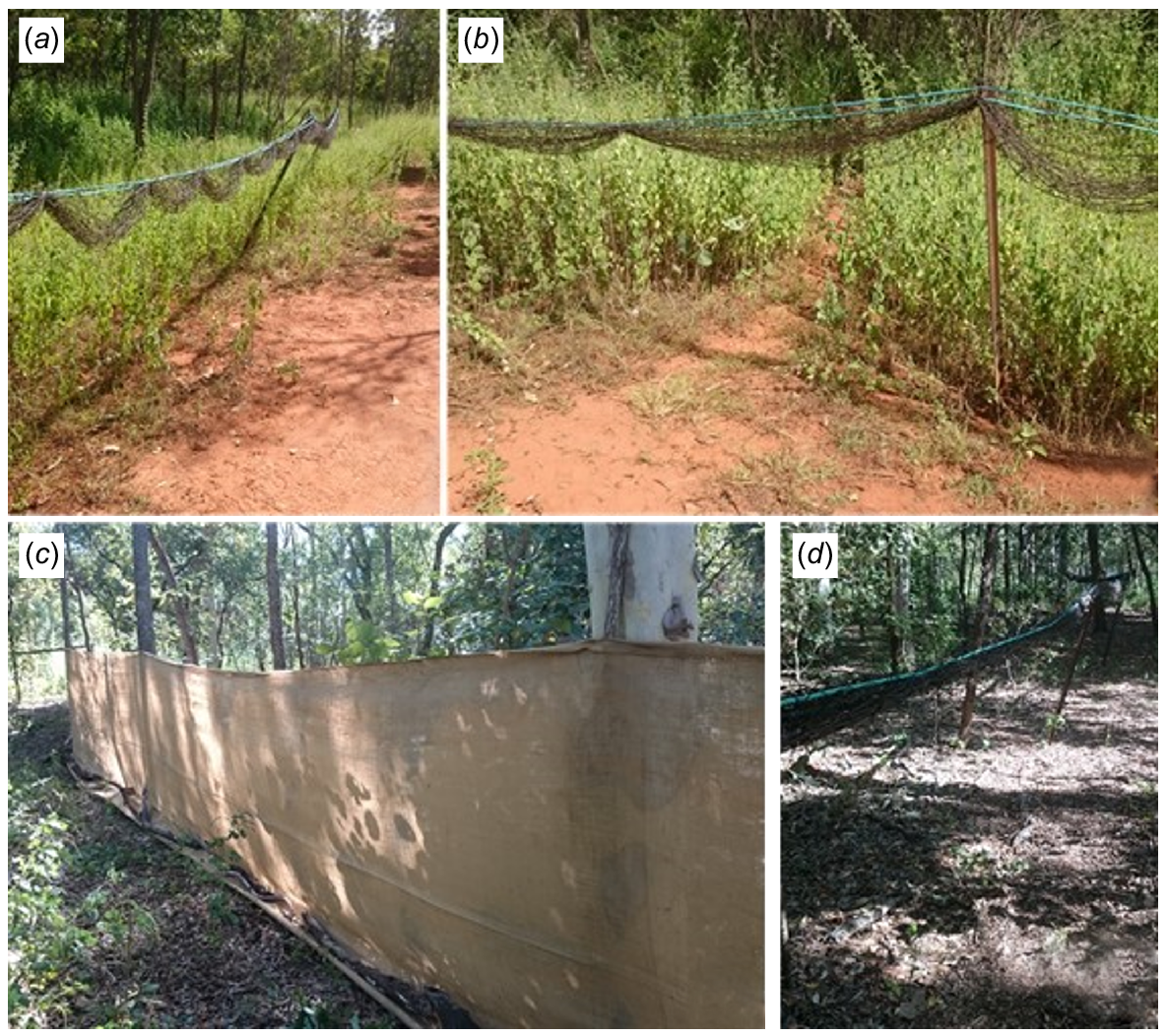
Netting was timed for the early morning and late afternoon when ambient temperatures were lower than 28°C to reduce the risk of hyperthermia. At least two people (handlers) were hidden behind the net during the catching session. Wet hessian bags were also hidden close by. Once the handlers were ready, at least four other people (walkers) walked toward the net in a line parallel to the net. Walkers started from up to 1 km away from the net, depending on where the wallabies were expected to be. During the approach, walkers created disturbance (noise) to ‘flush’ the wallabies towards the net.
Once caught, the handlers approached the animal quickly, placing a wet hessian bag on top of it to calm it down. The muscle relaxant and anxiolytic sedative Diazepam (Ilium Diazepam injection 5 mg/mL) was immediately administered to prevent capture myopathy. A first dose of Diazepam was administered at the point of capture based on visual estimation of body weight in a 5 kg body weight range (Table 1) at 1 mg/kg (Jackson 2007; Sadler 2010). The Diazepam was injected intramuscularly into the epaxial muscle (single-use sterile 22–23 gauge, ¾ inch needles, 3 mL syringe).
Overall (n = 40) | Adult ♂ (n = 4) | Adult ♀ (n = 18) | Subadult ♂ (n = 12) | Subadult ♀ (n = 6) | ||
---|---|---|---|---|---|---|
Weight (kg) | 11.19 ± 0.56 | 18.85 ± 1.77 | 11.02 ± 0.34 | 10.73 ± 0.81 | 7.52 ± 0.35 | |
Tail length (cm) | 66.15 ± 1.10 | 77.63 ± 5.04 | 65.54 ± 0.71 | 66.63 ± 2.11 | 59.33 ± 0.90 | |
Tail circumference (cm) | 17.19 ± 0.49 | 22.00 ± 0.74 | 16.34 ± 0.66 | 17.88 ± 0.83 | 15.17 ± 0.69 | |
Tibia length (cm) | 31.60 ± 0.53 | 38.00 ± 0.46 | 31.47 ± 0.43 | 31.42 ± 1.03 | 28.08 ± 0.30 | |
Pes length (cm) | 21.69 ± 0.24 | 24.50 ± 0.35 | 21.18 ± 0.26 | 22.08 ± 0.33 | 20.58 ± 0.49 | |
Health Index | 0.00 ± 0.02 | 0.03 ± 0.08 | 0.04 ± 0.03 | −0.01 ± 0.04 | −0.11 ± 0.03 | |
Testes length (mm) | 40.72 ± 1.97 | 51.23 ± 1.05 | N/A | 37.22 ± 3.61 | N/A | |
Testes width (mm) | 24.14 ± 0.97 | 29.14 ± 0.68 | N/A | 22.32 ± 1.79 | N/A |
Following the administration of the first dose of Diazepam, the animal was removed from the net and placed in a light-proof hessian bag. It was then weighed using a hanging weighing scale, discarding the weight of the bag. If the actual weight was higher than the visual estimate, a second dose of Diazepam was administered. At this point, the wallaby also received a dose of Richtasol (1 mL/25 kg) (Richtasol AD3EC injection Richter – Vitamin A, D3, E and C) by intramuscular injection (single use of a sterile 22–23 gauge, ¾ inch needles, with 1 mL syringe) into the epaxial muscle as per Diazepam. Richtasol is a multivitamin used to prevent and treat capture myopathy (Pierson et al. 2023).
After weighing and measuring (see Supplementary material), each individual was fitted with a VHF collar with an expandable insert as a self-release mechanism (Fig. 3). Animals were tracked during June and July 2016. In addition, each individual was fitted with a custom-made, uniquely colour-coded ear tag. Ear-tagging individuals for subsequent monitoring prolonged the handling time and may have influenced the outcome of the trials. We provide a detailed description of the ear tags as they are of a novel design. Ear tags were constructed from a plastic transparent UV-resistant cable tie (Fig. 4a). A 3 cm section of clear heat shrink tubing (6 mm diameter) was passed over the identification cable tie and fixed by a heat gun to the base (i.e. at the side closest to the locking head) (Fig. 4b, c). This acted as a cushioned surface to prevent irritation of the pierced ear. One piece (1.5 cm) of coloured heat shrink tubing (1.6 mm diameter) was then fixed by a heat gun over the cable tie’s identification tab (Fig. 4d, e). The colours of heat shrink tubing were selected to make 15 unique combinations (Fig. 4f). Two tags of each colour combination were constructed to double the combinations to 30 by placing the tags in the right (female) or left (male) ear.
Fitting of VHF collar to captured agile wallaby. (a) The collar model used; (b) collar fitting process; (c) the elastic insert added to the collar for self-release; and (d) wallaby with the collar fitted.
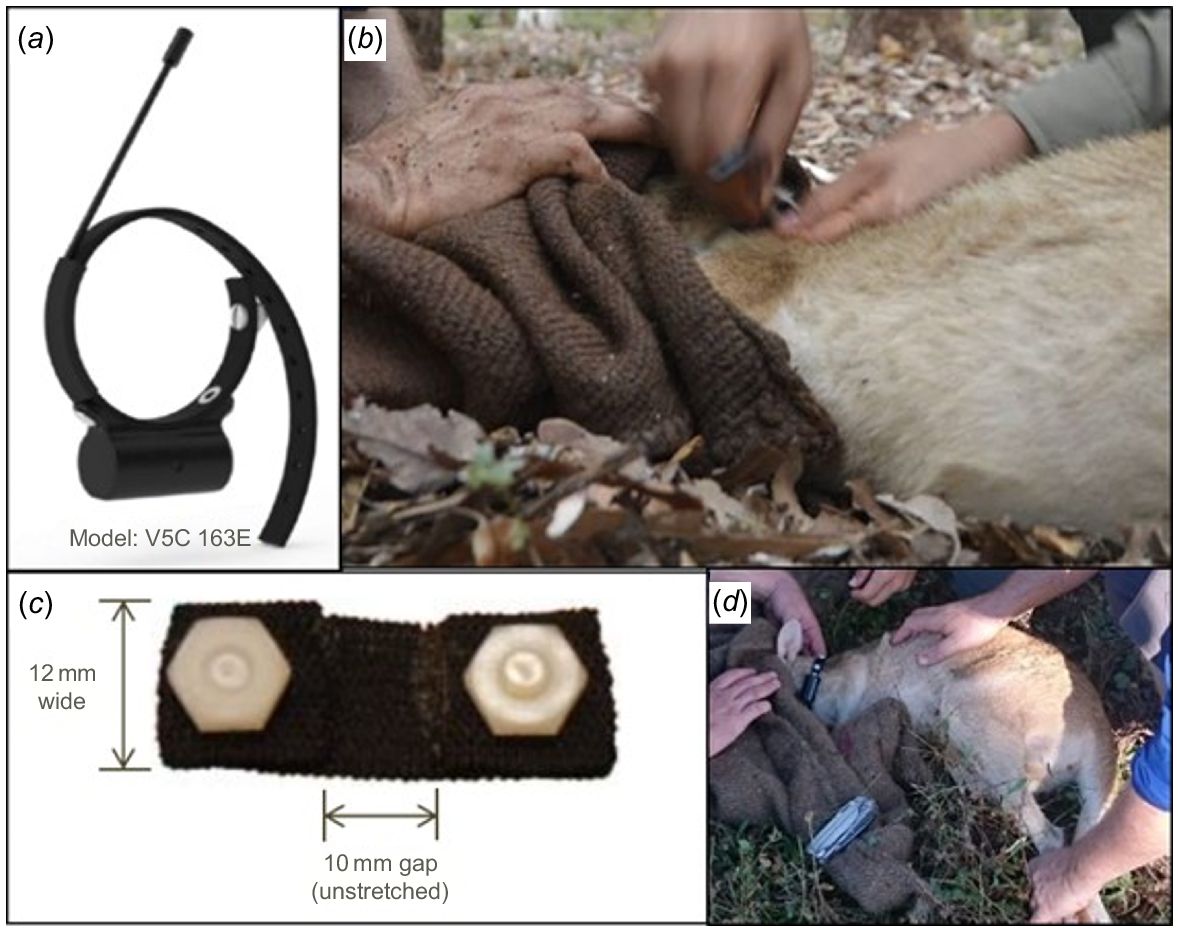
Construction of ear tags for agile wallabies: (a) plastic transparent UV-resistant identification cable tie; (b) 3 cm section of clear heat shrink tubbing (6 mm diameter) next to cable tie; (c) cable tie with clear heat shrink section fixed by heat gun to the base; (d) piece (1.5 cm) of coloured heat shrink tubbing (1.6 mm diameter) next to cable tie; (e) coloured heat shrink tubbing fixed by heat gun, over each side of the cable tie’s identification tab; and (f) each colour combination constructed by pairs.
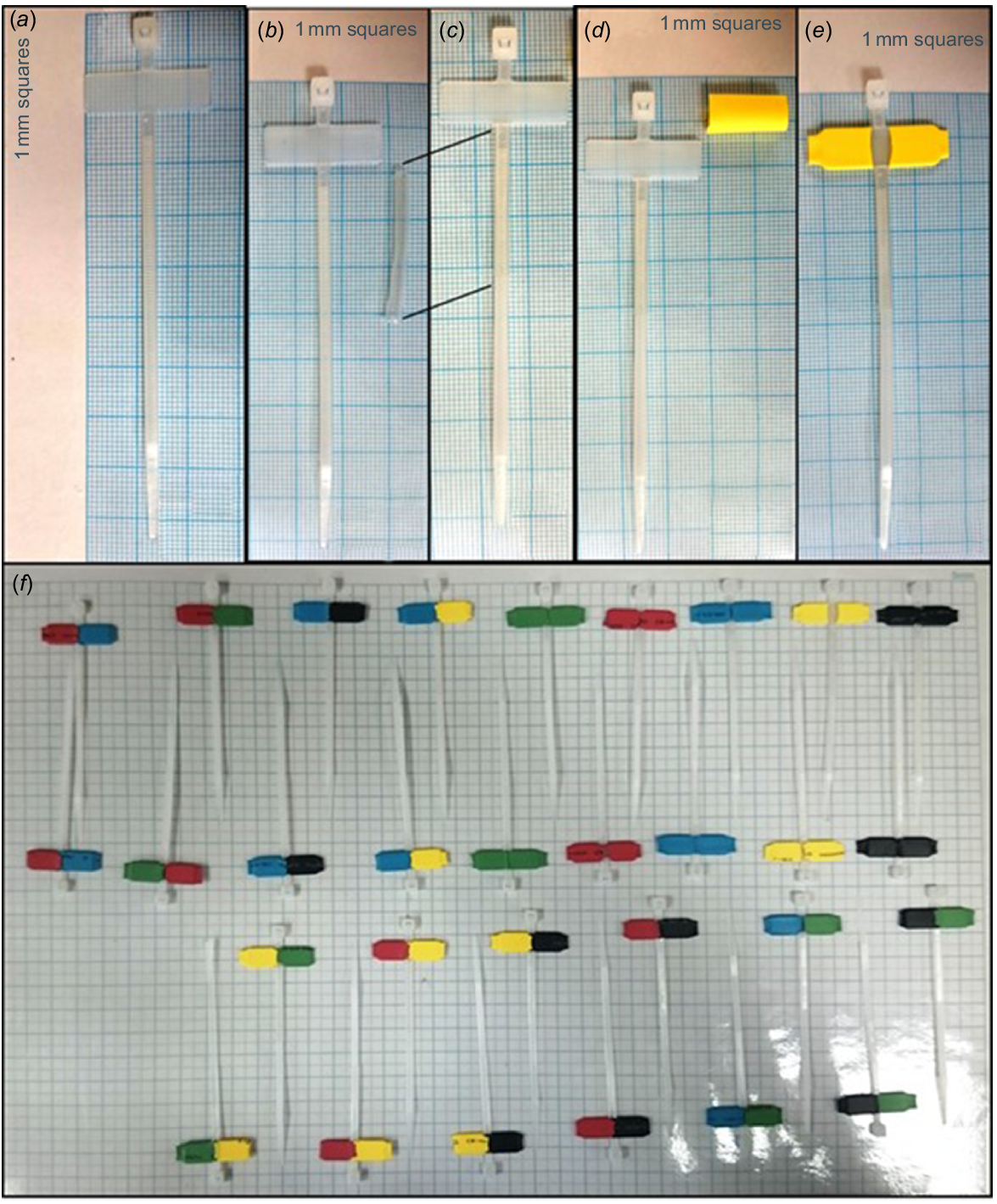
Statistical analyses
A ‘health index’ was calculated using a series of Generalised Linear Models (GLM). Each model combined a morphological measurement related to size, i.e. tibia length or pes length, and a morphological measurement related to fat reserves, i.e. weight or tail circumference. All models included sex as a fixed factor to account for sexual dimorphism. A log-transformation was applied to the models with ‘weight’ as the response variable due to non-normality and overdispersion of residuals. Adjusted R-squares for each model were used to select the model with the best fit, and the residuals were used as the ‘health index’ for each animal. The ‘health index’ values are centred at 0, i.e. the population average, with negative values indicative of animals with lower than average fat reserves adjusted by their size (lower condition) and positive values indicative of animals with higher than average fat reserves adjusted by their size (lower condition).
The factors influencing recovery times, i.e. time from release to flight, were explored by a separate Generalised Linear Model (GLM). The initial model included recovery time as the response variable and sex (male or female), age group (adult or subadult), health index, catch-to-bag time (the time it took from the moment the animal hit the net to when the animal was placed in the bag), and processing time (the time it took to measure, ear tag and collared the animal) as fixed factors. We compared the fit of models using corrected Akaike information criterion (AICc; ΔAIC) and excluded models with ΔAIC > 2 as having substantially less support (Burnham and Anderson 2010). The most parsimonious model of recovery times included only age group and processing time.
All analyses were performed using R v 4.4.1 (R Core Team 2024). The function glm from the package ‘MASS’ (Venables and Ripley 2002) was used to construct the GLMs. Assumptions were tested by performing: Shapiro-Wilk tests of normality, using the function shapiro.test from the package ‘stats’ (R Core Team 2024); Levene’s tests for homogeneity of variance (homoscedasticity) using the function leveneTest from the package ‘car’ (Fox and Weisberg 2018), and Pearsonʼs dispersion test by custom function. The function stepAIC from the package ‘MASS’ (Venables and Ripley 2002) was used for model refinement. P-values were generated by the type II Wald chi-square test using the function Anova from the package ‘car’ (Fox and Weisberg 2018).
Results
A total of 40 agile wallabies were caught from 81 net runs. We captured 49% of animals that entered the nets, with 51% either escaping the nets or the trigger release mechanism failing. No animals died during the capture process. Of the 40 animals captured, 34 animals were fitted with a VHF collar. Five of the collars were lost and later recovered. No collared or ear-tagged individuals died during this study.
Sex and age ratios
Of the 40 agile wallabies captured, 55% were adults, and 45% were subadults. Of the captured adults, 82% were female, and only 18% were male. Among captured subadults, 67% were male and 33% were female (Table 1).
Capture and recovery times
Agile wallabies received a Diazepam dose based on their expected weight class (1 mL for every 5 kg increment) (Table 2). Total handling time was kept as short as possible (16:24 ± 00:55 min; , n = 40) (Table 3). Animals were released when they were fully recovered. Four animals delayed their flight after release – one adult male took 11 min from release to flight, and two adult females took 11 and 21 min, respectively. One female took 373 min to recover, although no ill effects were observed following release and during subsequent VHF tracking. Heart rate and body temperature were monitored on this female during recovery, and no obvious cause of the recovery delay was detected. Capture-to-bag time was under 5 min for all animals (Table 3). Although recovery times were most affected by age group (, P = 0.068) or processing time (X = 1.607, P = 0.213), these effects were not significant. The models suggest that adults tend to have longer recovery times.
Animal’s weight (kg) | Diazepam dose (mg) | Diazepam volume (mL) | |
---|---|---|---|
0–5 | 5 | 1 | |
5–10 | 10 | 2 | |
10–15 | 15 | 3 | |
15–20 | 20 | 4 | |
20–25 | 25 | 5 |
Task times (minutes) | Overall (n = 40) | Adult ♂ (n = 4) | Adult ♀ (n = 18) | Subadult ♂ (n = 12) | Subadult ♀ (n = 6) | |
---|---|---|---|---|---|---|
Total handling | 16:24 ± 00:55 | 21:15 ± 03:06 | 16:23 ± 01:27 | 14:25 ± 00:58 | 17:10 ± 03:00 | |
Catch to bag | 04:15 ± 00:18 | 03:15 ± 01:19 | 04:30 ± 00:24 | 04:00 ± 00:30 | 04:40 ± 01:09 | |
Processing | 12:09 ± 00:49 | 18:00 ± 02:02 | 11:53 ± 01:12 | 10:25 ± 00:49 | 12:30 ± 03:09 |
Discussion
Capture myopathy is highly prevalent in macropods (Shepherd et al. 1988; Vogelnest and Woods 2008; McMahon et al. 2013; Green-Barber et al. 2018; Thompson et al. 2023). This study recorded no symptoms of capture myopathy or other complications or mortalities during the capture procedure or the post-capture monitoring period. Our integrated method, combining rapid restraint after capture, followed by sedative (Roberts et al. 2010; Thompson et al. 2023) and multivitamin injections (Pierson et al. 2023), represents a valuable improvement to macropods’ safe capture and restraint.
Trapping and chemical restraint
There are many methods for reducing capture myopathy in macropod as well as ungulate (and other) species (Shepherd et al. 1988; Vogelnest and Woods 2008; McMahon et al. 2013; Blumstein et al. 2015; Braud et al. 2019; Breed et al. 2019; Latham et al. 2020). Our method adheres to ‘the three ‘Rs’’ of animal-based research – Replacement, Reduction and Refinement – required to obtain animal ethics clearance. In their strict meaning, ‘replacement’ refers to using another species or captive animals, ‘reduction’ refers to minimising the number of animals handled, and ‘refinement’ refers to building on previously successful methods. Here, we apply these principles, but not always strictly in their original sense.
We replaced the typically used cyclohexamine anaesthetic Ketamine with the benzodiazepine anxiolytic sedative Diazepam. Ketamine is a schedule [S8] controlled substance and not readily available or as safe as Diazepam (Tyner et al. 1997; Posner and Burns 2009; Ferreira et al. 2015). While Ketamine has a wide margin of safety in most species, it does not provide the muscle relaxation needed to prevent capture myopathy in macropods (Fish 2008).
Another alternative used with Macropods including agile wallabies (Stirrat 1997) is a combination of Tiletamine and Zalazepam (Roberts et al. 2010; Mayberry et al. 2014; Thompson et al. 2023). This combination is commonly known as Zoletil 100 or Telazol. Tiletamine is a cyclohexamine related to Ketamine, with dissociative effects, while Zalazepam is a benzodiazepine that can be used as a mild sedative or muscle relaxant, similar to Diazepam; however, it is slightly more potent (Popilskis et al. 2008). Due to its dissociative effects, Tiletamine–Zolazepam is commonly used in darts, where rapid and persistent recumbency is required (Stirrat 1997; Roberts et al. 2010; Thompson et al. 2023). This makes Tiletamine–Zolazepam inappropriate for studies like the one we describe, where quick processing and recovery are paramount.
The sole use of Diazepam as an anxiolytic and light sedative was highly successful. Diazepam provides marked sedation and muscle relaxation but no analgesic effect (Hillyer and Quesenberry 1997; Roberts et al. 2010; Thompson et al. 2023). It has been used to reduce the occurrence of capture myopathy in other macropods, e.g. eastern grey kangaroo (Roberts et al. 2010) and western grey kangaroo (Thompson et al. 2023). Used alone, Diazepam does not provide a true anaesthetic state, and awareness persists with relaxation even at high dosages. Hence, there is a need to use methods of restraint that maintain a quiet capture environment that keeps the animal calm. In our case, animals were placed in a light-proof bag and kept cool by wetting the bag. Midazolam is another benzodiazepine that could replace Diazepam as it is better suited to intramuscular applications (Hillyer and Quesenberry 1997).
While typically, ‘reduction’ in an animal ethics context refers explicitly to reducing the number of animals used, here we show how other relevant ‘reductions’ were applied in this study. We reduced the stress on the animal by keeping handling times short and administering a safe anxiolytic (Diazepam) and a preventative multivitamin (Richtasol). In addition, we conducted captures on cooler days (below 28°C) and in the evenings to reduce the risk of heat stress, which can exacerbate capture myopathy (Meyer et al. 2008a, 2008b; Meyer 2009; Breed et al. 2019; Ali et al. 2023).
Lastly, we refined the drop-net capture method (Lentle et al. 1997; Mdetele et al. 2021) to capture one wallaby at a time, keeping handling times short and allowing for its proper care and restraint with the minimum of stress. These simple modifications to the drop-net method successfully prevented mortalities, particularly deaths due to capture myopathy, and misadventures (Hampton and Arnemo 2023). Lentle et al. (1997) drop-net technique requires the net to be lowered by an operator, with several animals potentially being enclosed at once; this can increase processing times and stress for the animals. Additionally, by using ‘walkers’ instead of vehicles, as described by Mdetele et al. (2021), we ensure the pace at which the animals are funnelled towards the net is steady and produces minimal flight response stress.
We further refined the management of potential capture myopathy by administering the multivitamin Richtasol (Pierson et al. 2023). We injected all study animals with a combination of vitamins A, D₃ E and C (Richtasol® AD 3EC). The use of vitamin E and selenium combination or an aggregate supplementation is widely recommended for the prevention of capture myopathy in macropods and other species undergoing capture and release (Lapidge 2005; McMahon and Bradshaw 2008; McMahon et al. 2013; Tarszisz et al. 2014).
It is important to acknowledge that the success of the capturing method we describe here, specifically the use of Diazepam and vitamin injections, has limited comparison potential with other methods that do not use these pharmacological interventions. In this study, we did not have control animals that did not receive Diazepam or vitamin injections. However, it is also important to recognise that this kind of study is challenging to perform when we must balance knowledge of current practices while trying to test if new approaches are beneficial. In the light of this, we consider our method to be still a valuable addition to the available repertoire of techniques.
Conclusion
Our modified drop-net and handling/treatment regime prevented capture myopathy in this study. Net modification, Diazepam, and vitamin injections are integral to this successful capture method for medium- and small-sized macropod species, resulting in no mortalities or recorded morbidities.
Data availability
All data supporting this study’s findings can be made available by request to the corresponding author.
Declaration of funding
This research received in-kind support from Douglas Daly Research Farm staff. Funding was provided by Meat and Livestock Australian (BAHE0303) and the Northern Territory Natural Resource Management (NTRM00332).
Acknowledgements
This research was conducted at the Douglas Daly Research Farm. Ethics approval was obtained from the Charles Darwin University Animal Ethics Committee, approval no. A15015. We gratefully acknowledge support from numerous people, including past and present managers of the Douglas Daly Research Farm, Spud Thomas and Cameron Heeb, as well as all the staff working at the farm. We thank Peter Phillips, Rachael Thurlow, our interns Constance Albert, Maxime Quignon, and Lea Lafourniere, as well as numerous volunteers who assisted with catching and tracking wallabies.
References
Ali S, Choudhury B, Basumatary P, Ashraf NVK, Baro D, Barman R, Narayanan A, Chidambaram B (2023) Post-capture management measures to reduce incidences of capture myopathy in Cervids. Veterinary Practitioner 24, 67-70.
| Google Scholar |
Aplin K, Dickman C, Salas L, Woinarski J, Winter J (2016) Macropus agilis. In ‘The IUCN Red List of Threatened Species 2016:. e.T40560A21954106. Available at https://dx.doi.org/10.2305/IUCN.UK.2016-2.RLTS.T40560A21954106.en [Accessed 10 January 2025]
Blumstein DT, Buckner J, Shah S, Patel S, Alfaro ME, Natterson-Horowitz B (2015) The evolution of capture myopathy in hooved mammals: a model for human stress cardiomyopathy? Evolution, Medicine, and Public Health 2015, 195-203.
| Crossref | Google Scholar | PubMed |
Braud C, Mitchell EP, Van der Merwe V, Tordiffe ASW (2019) A veterinary survey of factors associated with capture-related mortalities in cheetahs (Acinonyx jubatus). Journal of the South African Veterinary Association 90, a1723.
| Crossref | Google Scholar |
Breed D, Meyer LCR, Steyl JCA, Goddard A, Burroughs R, Kohn TA (2019) Conserving wildlife in a changing world: understanding capture myopathy – a malignant outcome of stress during capture and translocation. Conservation Physiology 7, coz027.
| Crossref | Google Scholar |
Ferreira JP, Dzikit TB, Zeiler GE, Buck R, Nevill B, Gummow B, Bester L (2015) Anaesthetic induction and recovery characteristics of a diazepam-ketamine combination compared with propofol in dogs. Journal of the South African Veterinary Association 86, a1258.
| Crossref | Google Scholar |
Green-Barber JM, Stannard HJ, Old JM (2018) A suspected case of myopathy in a free-ranging eastern grey kangaroo (Macropus giganteus). Australian Mammalogy 40, 122-126.
| Crossref | Google Scholar |
Hunt W, Bedoya-Perez M, Geesink G, van Den Heuvel A, Mullen J, Frost D (2021) Harvesting Agile Wallabies for human consumption in the Northern Territory of Australia. Ecological Management & Restoration 22, 141-146.
| Crossref | Google Scholar |
Kock MD, Jessup DA, Clark RK, Franti CE, Weaver RA (1987) Capture methods in five subspecies of free-ranging bighorn sheep: an evaluation of drop-net, drive-net, chemical immobilization and the net-gun. Journal of Wildlife Diseases 23, 634-640.
| Crossref | Google Scholar | PubMed |
Lapidge SJ (2005) Reintroduction increased vitamin E and condition in captive-bred yellow-footed rock wallabies Petrogale xanthopus. Oryx 39, 56-64.
| Crossref | Google Scholar |
Latham ADM, Davidson B, Warburton B, Yockney I, Hampton JO (2020) Efficacy and animal welfare impacts of novel capture methods for two species of invasive wild mammals in New Zealand. Animals 10, 44.
| Crossref | Google Scholar |
Lentle RG, Potter MA, Springett BP, Stafford KJ (1997) A trapping and immobilisation technique for small macropods. Wildlife Research 24, 373-377.
| Crossref | Google Scholar |
Mayberry C, Bencini R, Mawson PR, Maloney SK (2014) Sedation of western grey kangaroos (Macropus fuliginosus ocydromus) with tiletamine-zolazepam. Animal Welfare 23, 141-144.
| Crossref | Google Scholar |
McMahon CR, Bradshaw CJA (2008) To catch a buffalo: field immobilisation of Asian swamp buffalo using etorphine and xylazine. Australian Veterinary Journal 86, 235-241.
| Crossref | Google Scholar | PubMed |
McMahon CR, Wiggins NL, French V, McCallum HI, Bowman DMJS (2013) A report of capture myopathy in the Tasmanian pademelon (Thylogale billardierii). Animal Welfare 22, 1-4.
| Crossref | Google Scholar |
Mdetele D, Misinzo G, Komba E, Shemanche J, Eblate E, Mdaki M, Keyyu J, Kock R (2021) Modified netting technique for capturing gazelles in Serengeti, Ngorongoro and Loliondo, Tanzania. African Journal of Ecology 59, 152-158.
| Crossref | Google Scholar |
Meyer LCR, Fick L, Matthee A, Mitchell D, Fuller A (2008a) Hyperthermia in captured impala (Aepyceros melampus): a fright not flight response. Journal of Wildlife Diseases 44, 404-416.
| Crossref | Google Scholar | PubMed |
Meyer LCR, Hetem RS, Fick LG, Matthee A, Mitchell D, Fuller A (2008b) Thermal, cardiorespiratory and cortisol responses of impala (Aepyceros melampus) to chemical immobilisation with 4 different drug combinations. Journal of the South African Veterinary Association 79, 121-129.
| Crossref | Google Scholar | PubMed |
Phillips BE, Cannizzo SA, Godfrey MH, Stacy BA, Harms CA (2015) Exertional myopathy in a juvenile Green Sea Turtle (Chelonia mydas) entangled in a large mesh gillnet. Case Reports in Veterinary Medicine 2015, 604320.
| Crossref | Google Scholar |
Pierson JC, Berry L, Alexander L, Anson J, Birkett M, Kemp L, Pascoe BA, Farquharson KA, Hogg CJ (2023) Adaptive genetic management of a reintroduction program from captive breeding to metapopulation management of an arboreal marsupial. Diversity 15, 848.
| Crossref | Google Scholar |
Rogers DI, Battley PF, Sparrow J, Koolhaas A, Hassell CJ (2004) Treatment of capture myopathy in shorebirds: a successful trial in northwestern Australia/(Tratamiento de Miopatía en playeros: casos exitosos en la parte noroeste de Australia). Journal of Field Ornithology 75, 157-164.
| Crossref | Google Scholar |
Shepherd NC, Hopwood PR, Dostine PL (1988) Capture myopathy - 2 techniques for estimating its prevalence and severity in red kangaroos, Macropus-Rufus. Australian Wildlife Research 15, 83-90.
| Crossref | Google Scholar |
Stirrat SC (1997) Behavioural responses of agile wallabies (Macropus agilis) to darting and immobilisation with tiletamine hydrochloride and zolazepam hydrochloride. Wildlife Research 24, 89-95.
| Crossref | Google Scholar |
Stirrat SC (2008) Age structure, mortality and breeding in a population of agile wallabies (Macropus agilis). Australian Journal of Zoology 56, 431-439.
| Crossref | Google Scholar |
Tarszisz E, Dickman CR, Munn AJ (2014) Physiology in conservation translocations. Conservation Physiology 2, cou054.
| Crossref | Google Scholar |
Thompson GG, Thompson SA, Pusey A (2023) Poor welfare outcomes resulting from poor management decisions in a translocation of western grey kangaroos (Macropus fuliginosus). Pacific Conservation Biology 29, 130-140.
| Crossref | Google Scholar |
Tyner CL, Woody BJ, Reid JS, Chafetz EP, Lederer HA, Norton JF, Keefe TJ, Jöchle W (1997) Multicenter clinical comparison of sedative and analgesic effects of medetomidine and xylazine in dogs. Journal of the American Veterinary Medical Association 211, 1413-1417.
| Crossref | Google Scholar | PubMed |
Wallace RS, Bush M, Montali RJ (1987) Deaths from exertional myopathy at the National Zoological Park from 1975 to 1985. Journal of Wildlife Diseases 23, 454-462.
| Crossref | Google Scholar | PubMed |