Balancing act: evaluating non-target risks of feral cat management in conservation reserves
Mark Cowan A E , Michelle Drew B , Brian Macmahon C and Lesley A. Gibson
A
B
C
D
E Present address:
Abstract
Predation by feral cats continues to place substantial pressure on native Australian wildlife, contributing to significant population declines and localised extirpations of susceptible species. In Western Australia (WA), the registration of the poison bait Eradicat® provides a tool to help manage these introduced predators, but only in areas where the risk to non-target species is considered acceptable. The red-tailed phascogale (Phascogale calura), a small carnivorous marsupial now restricted to vegetation remnants in the highly fragmented agricultural zone of south-western WA (i.e. the Wheatbelt), is one species that may be vulnerable to lethal ingestion.
To investigate the impact of repeated Eradicat® baiting, to control feral cats, on the activity levels of the red-tailed phascogale, focusing on populations in two Wheatbelt conservation reserves.
We established a novel approach to monitoring red-tailed phascogales by using tree-mounted camera trap arrays in an area with feral cat management using ground-delivered Eradicat® baits, and two control zones with no feral cat management. We examined changes in activity levels (detection rate and occupancy) based on camera trap detections, before and after Eradicat® application, across two autumn and two spring baiting events. We also investigated non-target bait uptake using camera traps.
Although a small number of baits (7/60) was removed by red-tailed phascogales from the field of view of a camera, our results showed no overall impact of Eradicat® on their activity levels within the study area. Tree-mounted camera traps proved to be highly effective and efficient at detecting red-tailed phascogales. To maximise camera detections, the optimal time for monitoring red-tailed phascogales is during autumn, prior to male die-off.
Our results suggested that the risk posed to red-tailed phascogale populations from the repeated use of Eradicat® baits is likely to be low.
Integrating the application of Eradicat® to control feral cats with existing fox control in conservation reserves that support populations of red-tailed phascogales is likely to pose minimal risk to the species.
Keywords: arboreal camera monitoring, carnivorous marsupial, conservation management, Eradicat® baits, introduced predator control, non-target impacts, red-tailed phascogale, Wheatbelt reserves.
Introduction
As the scourge of the feral cat (Felis catus) continues to affect Australian native wildlife with devastating consequences (Woinarski et al. 2017, 2018, 2020; Murphy et al. 2019), the race is on to develop effective mitigation approaches. Although feral cats are largely ubiquitous in Australian ecosystems (Legge et al. 2017), broadscale control techniques used have met with mixed results across varying climate zones (e.g. Comer et al. 2020; Lohr and Algar 2020; Palmer et al. 2021; Fancourt et al. 2022). In Western Australia (WA), attempts at landscape-scale control of these introduced predators rely largely on the aerial application of the Eradicat® bait (Algar et al. 2013; Comer et al. 2018; Lohr and Algar 2020). Eradicat®, developed and produced by the Department of Biodiversity, Conservation and Attractions (DBCA) specifically for the control of feral cats, is a sausage-style bait that contains 4.5 mg of the toxin, sodium fluoroacetate or 1080 (Algar et al. 2007). Sodium fluoroacetate is a naturally occurring plant metabolite toxin found in high concentrations in many Western Australian plant species (Twigg and King 1991). As such, WA native fauna species that have evolved with the toxin have developed some tolerance to it (Mcilroy 1981; Twigg and King 1991). However, tolerance levels vary; native herbivores tend to tolerate much higher doses than do native predatory species (Twigg and King 1991), and small predatory species may be susceptible to the concentrations of 1080 found in Eradicat® (Hetherington et al. 2007).
One native species that has been considered vulnerable to Eradicat® baiting is the red-tailed phascogale (Phascogale calura), a small (<70 g), semi-arboreal, carnivorous marsupial. Like numerous other small to medium-sized Australian mammal species, the red-tailed phascogale has suffered a severe contraction in its distribution since European colonisation, and it is now primarily restricted to highly fragmented habitat in the southern portion of the WA agricultural zone, i.e. the Wheatbelt (Short et al. 2011). The species is currently listed as ‘Vulnerable’ under the Commonwealth Environment Protection and Biodiversity Conservation Act 1999, and ‘Conservation Dependent’ under the WA Biodiversity Conservation Act 2016. The approximate lethal dose of 1080 to the red-tailed phascogale is 14.1 mg/kg (Martin et al. 2002). Given that a single Eradicat® bait contains 4.5 mg of 1080, theoretically a small red-tailed phascogale (20 g) would need to consume only 6% of the bait to receive a lethal dose or 22% by a large (70 g) individual (Friend et al. 2020).
Early laboratory trials have demonstrated that red-tailed phascogales will consume non-toxic Eradicat® baits, particularly when other food resources are limited (Algar 2006). Trials in the field, also using non-toxic Eradicat® baits but labelled with the biomarker rhodamine B, similarly provided evidence of some consumption of the bait (Algar 2006). Additional field trials undertaken in a number of Wheatbelt reserves, using both non-toxic and toxic Eradicat® baits, showed that although red-tailed phascogales sometimes interacted with the bait, all nine radio-collared individuals survived over the period in which toxic baits were available and/or palatable (Friend et al. 2020). In these trials, of the 62 individuals exposed to rhodamine-labelled non-toxic baits, only one showed any sign of rhodamine ingestion. Although Friend et al. (2020) concluded that the risk to red-tailed phascogales from using Eradicat® to control feral cats was low, they also recommended further trials using repeated baiting events be undertaken to strengthen their case.
The Wheatbelt region of WA has numerous small, isolated nature reserves (only three are >100,000 ha; most are <5000 ha) surrounded by cleared agricultural land (Department of Biodiversity, Conservation and Attractions 2021). While these reserves are refuges for remnant populations of conservation-significant fauna, such as the red-tailed phascogale, they also harbour introduced predators, such as the feral cat and red fox (Vulpes vulpes). Management of introduced predators in these smaller reserves has been challenging owing to the high reinvasion rates of both foxes and feral cats from the surrounding farmland (Marlow et al. 2015a), and they are impractical to aerial bait because of their size. Ground-based fox baiting, using dried meat baits injected with 4.5 mg of 1080 solution, commenced in these reserves in the 1980s, and even though initial results saw significant recovery of several mammal species (de Tores and Marlow 2012), many of these reserves subsequently experienced major faunal declines in the early 2000s (e.g. Wayne et al. 2013). Although it is difficult to determine the exact cause, meso-predator release of feral cats following the introduction of fox baiting has been implicated (Marlow et al. 2015b). As such, there has been a shift towards integrated control of both feral cats and foxes in WA conservation reserves (Comer et al. 2020).
To provide further assurance to conservation managers intending on integrating feral cat with existing fox control in conservation reserves where red-tailed phascogales persist, a need was identified to better understand the risk of repeated Eradicat® baiting on the species. Here, we established a camera trap monitoring program based on a before–after–control–impact experimental approach focusing on two Wheatbelt reserves with known red-tailed phascogale populations, and routine fox control.
Materials and methods
Study area
The main study area, Tutanning Nature Reserve (~2380 ha), is located 150 km south-east of Perth in the Wheatbelt region of WA and included both a control and treatment zone (Fig. 1). A second control zone was selected in Boyagin Nature Reserve (~4900 ha), situated 40 km to the east of Tutanning (Fig. 1). The area is characterised by Eucalyptus (powder-bark wandoo, brown mallet) and Allocasuarina and Acacia (rock sheoak and jam) woodlands interspersed with patches of kwongan heath and shrublands (Brown and Hopkins 1983). The reserves experience a Mediterranean climate with a mean annual rainfall of ~444 mm (Pingelly Weather Station; www.bom.gov.au; accessed 22 November 2023), and dominant winter rainfall between May and September. Mean long-term maximum and minimum temperatures are 32 and 5.5°C respectively (www.bom.gov.au). Commencing in the mid-1980s, both reserves have been regularly baited for foxes using a dried meat bait injected with 1080 (up to 2010) and then with Pro-bait (2011–2024), a 40 g dry sausage bait containing 3 mg of 1080 also developed and produced by DBCA, which is more inaccessible to small marsupials (Marlow et al. 2015a). Prior to our study, there was no active management of feral cats within either reserve. It is important to note here that our study was not designed to measure the efficacy of Eradicat® baiting on feral cats, or even necessarily to reduce their abundance. We were focused on the impact of Eradicat® baiting on the red-tailed phascogale.
Bait deployment
Eradicat® baits were deployed by hand to approximately half of Tutanning Nature Reserve at a rate of one bait every 100 m along existing vehicle tracks (i.e. treatment zone). In months when Eradicat® was not applied, Pro-bait continued to be delivered at a rate of one bait every 100 m. This resulted in two Eradicat® applications and 10 Pro-bait applications per annum in the treatment area. The remaining half of the reserve continued to receive its existing prescription of Pro-bait (one bait every 100 m) each month and acted as a control zone. A second control zone was established in the western half (~2819 ha) of Boyagin Nature Reserve. This reserve continued to receive Pro-bait at the same prescription as for the control zone in Tutanning.
Baits are typically delivered along ground transects by being thrown from a slow-moving vehicle; however, for these trials, we required that baits be placed in close proximity to the camera array. Prior to deployment, all Eradicat® baits were thawed and placed in direct sunlight to ‘sweat’ the oils and lipid soluble digest material to the surface of the bait. During this process, the baits were sprayed with an ant-deterrent compound (Coopex®) at a concentration of 12.5 g per litre to prevent bait degradation by ants.
Monitoring approach
Red-tailed phascogales were monitored before and after bait delivery by using Reconyx HP2X (Reconyx, Wisconsin, USA) camera traps mounted on 4 cm wide by 0.8 m long wooden platforms attached to tree trunks by right-angle metal brackets approximately 1.6 m above ground level. Camera traps were programmed to high motion-sensor sensitivity, with three rapid fire images taken per trigger event, no delay between trigger events and fast shutter for night-time exposures. Because red-tailed phascogales are primarily nocturnal, camera traps were programmed to trigger only between 1800 hours and 0600 hours. An olfactory lure (PVC container containing peanut butter and oats) was placed in front of each camera trap (except for the last trial, see below). Thirty camera traps at a minimum spacing of 500 m were established in each of the control zones, and within the Eradicat® treatment zone (Supplementary Figs S1, S2), and data collated for a minimum of 16 nights before, and again after, the baiting application. A bait was initially placed within 20 m of the tree with the mounted camera for the first trial, but was subsequently changed to within 5 m for the remaining trials to increase the chance that red-tailed phascogales active on monitored trees would encounter them. All images from the camera traps were uploaded into the program CPW Photo Warehouse (Ivan and Newkirk 2016) for processing. Date- and time-stamp information from each image was captured. For analysis, we used only the first camera trap detection within a 24-h period as an independent detection event, defined as from midday to midday, unless multiple individuals were present in a single image, in which case the number of individuals in that image was used. To investigate seasonal differences, trials were undertaken in autumn and spring 2021, and autumn and spring 2022.
Because lures may influence behaviour and this can vary over time, and given that earlier trials had shown that red-tailed phascogales remained active on platforms even without lures, we used Trial 4 to investigate this aspect further. We compared mean detection rate (per 100 trap-nights) for this trial with that of Trial 2 (i.e. same sampling season) at both Tutanning (mean ± s.e., 9.2 ± 1.3 lured, 8.8 ± 1.5 un-lured) and Boyagin (mean ± s.e., 10.6 ± 2.0 lured, 8.3 ± 1.4 un-lured) by using a two-tailed paired Student’s t-test, with no significant difference observed at either site (Tutanning: t-value = 0.33, P = 0.74; Boyagin: t-value = 0.94, P = 0.35).
Eradicat® uptake
To better understand animal interactions with Eradicat® baits, especially those of red-tailed phascogales, and the longevity of baits in the environment, a ground-based camera trap (Reconyx HP2X) mounted on a plastic stake at a height of 40 cm was placed 1.5 m from an Eradicat® bait at each of the 30 monitoring sites in the treatment zone during autumn and spring 2021 (Trials 1 and 2) and autumn 2022 (Trial 3) baiting events. These cameras used the same settings as did the tree mounted cameras, except that they were active both day and night. This resulted in the monitoring of 90 individual Eradicat® baits. At the monitoring sites where the bait was removed, we examined the number of detections on the tree-mounted camera traps for 3 weeks before and after bait removal, to identify the possibility of a negative impact at those sites by using a two-tailed paired Student’s t-test.
Statistical analysis
Changes in red-tailed phascogale activity were modelled using a generalised linear mixed model (GLMM) with a Poisson distribution for each reserve (Tutanning or Boyagin) and each trial separately. The response variable was the total number of independent detections of red-tailed phascogales recorded on each camera trap. For the analysis, only camera detection data from 21 nights pre- and post-baiting were used for all trials and in all zones, but only 16 nights pre- and post-baiting for Trial 4. Camera trap ID was modelled as a random intercept and fixed factors included zone (Tutanning only: control or treatment), session (pre- or post-baiting) and the interaction term (Tutanning only: zone × session). All analyses were performed using R Statistical Software (v4.2.2; R Core Team 2022). GLMMs were fitted using the ‘lme4’ package (v1.1-30; https://cran.r-project.org/web/packages/lme4/index.html; Bates et al. 2015). Post-hoc tests were used to examine pairwise combinations of all variables in the R package ‘emmeans’ (V1.8.0; https://cran.r-project.org/web/packages/emmeans/index.html; Lenth et al. 2020).
Additionally, occupancy from camera trap data was examined for each reserve and each trial, separately, to determine if there was a decrease in occupancy following baiting. Single-season occupancy analysis, without covariates, was employed to assess changes in peak occupancy and probability of detection by only using the seven consecutive day period with the highest occupancy before and after Eradicat® baiting. While we initially examined data for both autumn and spring, a large decrease in detections in spring, and consequently, low overall detection probability, resulted in 95% confidence intervals for occupancy which were too large to make any meaningful interpretations from these data (ranging from close to 0–1; Table S4). Subsequently, we restricted discussion of the occupancy analysis to autumn trials only (Trials 1 and 3).
Results
Across the four trials and all zones, the total number of independent camera detections of red-tailed phascogales was 3373 over 158 nights (0.24 detections per camera trap night). The total number of detections per night for each trial are shown in Table 1. The large difference in the number of detections between spring and autumn trials is likely to be due to male post-breeding mortality, which occurs in July (Bradley 1997).
Camera trap detections | Autumn 2021 | Spring 2021 | Autumn 2022 | Spring 2022 | |
---|---|---|---|---|---|
Total (number of nights) | 1500 (42) | 357 (42) | 1279 (42) | 237 (32) | |
Detections per night | 35.7 | 8.5 | 30.5 | 7.4 |
Changes in detection rate
At Tutanning, the only significant treatment by session interaction observed was in Trial 1 (Fig. 2, Supplementary Table S1). Post hoc tests showed a significant increase in red-tailed phascogale detections in the control zone during the post-baiting period (where Eradicat® baits were not deployed). However, there was no significant difference pre- and post-baiting in the treatment zone (Table S2). The only significant decline in detections in the treatment zone (following baiting) was in Trial 2, but a decline was also observed in the control zone, the treatment by session interaction was not significant (Table S2), and very low detection rates were observed during this trial (Fig. 2). In the Boyagin control zone (again where Eradicat® baits were not deployed), a significant decline in red-tailed phascogale detections was also observed in Trial 2 during the post-baiting period (Table S1, Fig. 2). Post hoc tests similarly showed a significant decline in detections in Trial 3 in the Boyagin control zone during this period (Table S2).
Mean detection rate (per 100 camera trap nights per camera trap site) at (a) the Tutanning treatment and (b) control zones, and (c) Boyagin control zone, prior to (before_bait) and after (post_bait) Eradicat® baiting across four trials (1: autumn 2021; 2: spring 2021; 3: autumn 2022; 4: spring 2022). Error bars represent standard error.
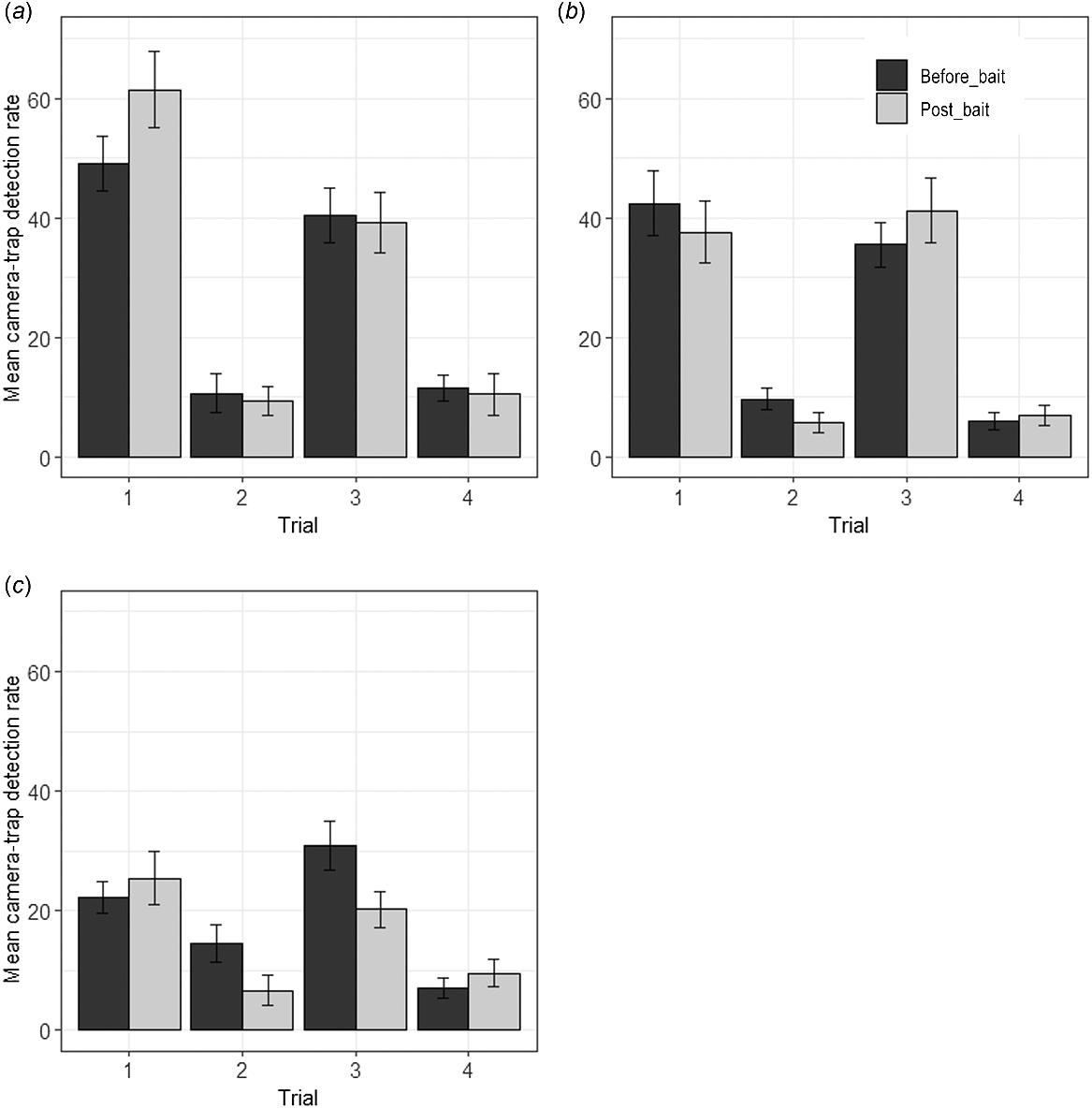
Changes in occupancy
Peak occupancy remained high and stable in all zones and across the two autumn trials (Trials 1 and 3), with slight increases in the Tutanning treatment zone following baiting, and slight decreases or no differences in the two control zones, and considerable overlap of the 95% confidence intervals (Fig. 3, Table S3).
Eradicat® bait uptake
Brush-tailed possums (koomal; Trichosurus vulpecula) were responsible for the majority of Eradicat® bait uptake (37%; 33 baits) with red-tailed phascogales removing 8% (7 baits) (Table 2). After 28 days post-baiting, the maximum length of time camera traps were deployed, the proportion of baits not consumed was 10% (nine baits). These baits had become hard and dry and were likely to be unpalatable and inaccessible to red-tailed phascogales. Baits that were removed or consumed lasted on the ground for an average of 2.8 ± 0.3 days. There were interactions at seven sites where red-tailed phascogales removed the bait from the view of the camera, but none was observed to eat the bait. There were a further eight sites at which red-tailed phascogales either investigated or passed baits but had no other interaction. Where baits were removed, a comparison of the pre-bait (mean ± s.e. = 3.9 ± 1.5) and post-bait (mean ± s.e. = 3.7 ± 1.6) red-tailed phascogale detections on the tree-mounted camera traps showed no significant difference (t-value = 0.28, P = 0.79).
Species | Total | Percentage taken | |
---|---|---|---|
Brushtail possum, koomal (Trichosurus vulpecula) | 33 | 36.7 | |
Grey currawong (Strepera versicolor) | 12 | 13.3 | |
Unknown | 9 | 10.0 | |
Uneaten | 9 | 10.0 | |
Red-tailed phascogale (Phascogale calura) | 7 | 7.8 | |
Bobtail (Tiliqua rugosa) | 5 | 5.6 | |
Chuditch (Dasyurus geoffroii) | 3 | 3.3 | |
Australian raven (Corvus coronoides) | 3 | 3.3 | |
Fox (Vulpes vulpes) | 2 | 2.2 | |
Western grey kangaroo (Macropus fuliginosus) | 1 | 1.1 | |
House mouse (Mus domesticus) | 1 | 1.1 | |
Varanid spp. | 1 | 1.1 | |
Australian magpie (Gymnorhina tibicen) | 1 | 1.1 | |
Tammar wallaby (Notamacropus eugenii) | 1 | 1.1 |
Discussion
The registration of Eradicat® poison baits in Western Australia provides a mechanism for the broad-scale management of feral cats; however, potential impacts on non-target species can limit its use. For example, the application of Eradicat® is not currently permitted in areas where the endangered northern quoll (Dasyurus hallucatus) occurs (Cowan et al. 2020). Although some understanding of the sensitivity of a species to the toxin can be achieved from laboratory-derived trials (i.e. LD50), this may differ under field conditions, with a variety of factors influencing susceptibility to lethal ingestion, such as an animal’s ability to find and consume baits and behavioural responses such as bait aversion (Glen et al. 2007). Similarly, palatability trials under laboratory conditions may not be replicated in the wild. On the basis of four bait applications across 2 years, our results showed no overall impact of Eradicat® on activity levels of the red-tailed phascogale population within Tutanning Nature Reserve. The only significant decline in detections in the Eradicat®-baited zone, following baiting, corresponded with a decline in both control zones, indicating the influence of other environmental or behavioural factors, which may be associated with decreased activity of females with young post-breeding.
Arboreal camera-monitoring
In our assessment, the first challenge was to devise a monitoring approach that would provide enough detections of red-tailed phascogales to undertake a robust analysis based on a before–after–control–impact (BACI) design. Red-tailed phascogales can be difficult to trap, and often require a period of trap conditioning whereby the trap mechanism is disabled and traps are pre-baited before attempts to capture them (e.g. Pierson et al. 2023). While red-tailed phascogales are reported to forage on the ground, they are largely arboreal (Short et al. 2011). Our alternative approach of using tree-mounted camera traps proved to be highly effective and efficient at detecting the species, in some cases, up to three individuals were concurrently detected on the same camera, as well as it being a low-impact method from an animal-welfare perspective. Arboreal camera trapping is a recent approach that has shown to be effective elsewhere for monitoring other tree-dwelling species (Moore et al. 2021). A further advantage of using camera traps is that they allow assessments over much larger scales both temporally and spatially than does live-trapping.
The use of a lure is likely to have increased our ability to detect red-tailed phascogales; however, lures can confound results if the effectiveness of the lure diminishes over time (Barcelos et al. 2023). This potential bias can be managed by restricting the duration of the exposure to the lure during each monitoring period and being consistent across treatments. Our examination of lured versus un-lured cameras showed that the mean detection rate did not differ between the spring 2021 (lured) and spring 2022 (un-lured) trials. As such, over extended periods, the detection rate of red-tailed phascogales without the use of a lure may be sufficient to monitor trends, but this requires further validation, given the low number of detections during the spring trials. In terms of maximising detections, results also showed that the optimal time for monitoring red-tailed phascogales using camera traps is during autumn, prior to male die-off.
The disadvantage of relying on camera traps for monitoring trends of red-tailed phascogales is that changes in abundance cannot be readily determined. However, cameras can identify declines in red-tailed phascogale activity over time and trigger the need to undertake more intensive investigations should a decline be observed. In further studies, another approach that could be trialled is the use of remote-logging microchip readers (e.g. Ross et al. 2022). Because red-tailed phascogales readily use the camera trap platform attached to trees (in this case, using lures would be advantageous), a microchip reading antenna could be mounted to these platforms to capture data from microchipped individuals. The only live-trapping effort required would be to microchip as many individuals as possible prior to baiting, and all subsequent monitoring would occur remotely, allowing continuous data capture over extended timeframes.
Susceptibility of red-tailed phascogales to Eradicat®
Our results supported the conclusion of Friend et al. (2020) that the risk posed to red-tailed phascogale populations from the use of Eradicat® baits in areas where the species occurs is likely to be low. Although there is a chance that some individuals may consume enough bait to receive a lethal dose, our results suggested that this may not translate to population-level impacts (e.g. Fancourt et al. 2021). Moreover, the benefit of reducing the impact of predation by feral cats on highly threatened and susceptible fauna populations is likely to outweigh the possible impact on a few individual red-tailed phascogales (Glen et al. 2007). Our study attempted to maximise exposure of red-tailed phascogales to Eradicat® by delivering baits near trees where animals were known to be present; yet, we could not detect changes attributable to the bait application. Operational baiting such as the aerial application of Eradicat® used by the Department of Biodiversity, Conservation and Attraction’s Western Shield Program would be likely to provide less overall exposure to baits than was the case in our study. Nevertheless, a precautionary approach should be taken as population-level impacts may be difficult to determine (Glen et al. 2007). This is particularly the case if the baiting prescription is more intensive, i.e. there is a higher frequency and density of baits in the landscape (than was used in our study) to improve the likelihood of feral cats encountering baits. Long-term monitoring that captures a range of seasonal conditions, and corresponding fluctuations in population size of red-tailed phascogales, including drought periods when natural food resources are likely to be scarce, is likely to be more informative. Although poison-baiting programs may be more effective during dry conditions when feral cats are hungry, non-target species are equally likely to be more vulnerable to toxic ingestion.
Bait uptake by non-target species
Examination of bait uptake by red-tailed phascogales supported the results of the arboreal camera-monitoring. Although we recorded a small number of bait removals by red-tailed phascogales, we were unable to detect a difference in tree-mounted camera detection rates at those sites where bait was removed before and after baiting (noting the small sample size). Friend et al. (2020) also documented baits being removed outside the field of view of cameras by red-tailed phascogales, but rhodamine B results indicated little evidence of baits being consumed. Also consistent with Friend et al. (2020), other non-target species were responsible for removing a large proportion of the baits, predominantly brush-tailed possums. Non-target 1080-bait uptake studies in WA have generally failed to demonstrate impacts to populations of native mammals (e.g. Dundas et al. 2014; Comer et al. 2020; Cowan et al. 2020; Bain et al. 2021; Doherty et al. 2022), which is probably not that surprising, given their naturally high tolerance to 1080, particularly in south-western WA (Twigg and King 1991). For example, the northern quoll, in theory, is at risk of lethal poisoning from the consumption of just a single Eradicat® bait. Using radio-collars, Cowan et al. (2020) examined the impact of Eradicat® on the northern quoll in the Pilbara region of WA. Their study demonstrated that there was no detectable risk to northern quolls from aerially delivered Eradicat® baits (applied at 50 per km2) and there were no sublethal effects on reproductive output. Palmer et al. (2021) further demonstrated that ongoing Eradicat® use had a positive effect on the northern quoll population, with evidence of range expansion in the baited area. Similarly, Moseby et al. (2021) reported that none of 37 collared chuditch (western quoll, Dasyurus geoffroii), a species that also occurs in the Wheatbelt region of WA, died as a result of consuming Eradicat® baits (also aerially applied at 50 per km2), despite their bait-uptake study showing that chuditch removed 26% of the baits.
It is also possible that only a small proportion of the bait (if any) is consumed, which may elicit a taste-aversion response, with animals exposed avoiding baits thereafter (Palmer et al. 2021). Learned avoidance of 1080-baits through a sublethal dose has been reported in introduced brush-tailed possums in New Zealand (Moss et al. 1998). However, removal of baits by non-target species is problematic because this reduces the number of baits available to the target species, and thereby may reduce the efficacy of the baiting program. Indeed, we recorded no baits being removed by feral cats, which is consistent with the results of Friend et al. (2020). This is an ongoing problem for introduced-predator control using baits in south-western WA (Dundas et al. 2014; Bain et al. 2021).
Data availability
The data that support this study can be shared upon request to the corresponding author.
Declaration of funding
This project was supported by the Western Australian Department of Biodiversity, Conservation and Attractions (DBCA).
Acknowledgements
We acknowledge and pay our respects to the Noongar people as Traditional Custodians of the lands where our research was conducted. Many thanks go to Ashleigh Johnson who assisted with fieldwork and data management. We are also grateful to Kerri-Ann Hudson, Marie Edgley, Brett Beecham, Peter Lacey and Greg Durell from DBCA’s Wheatbelt Region for supporting the study and providing comment on earlier reports. We thank two anonymous reviewers for their comments on the manuscript.
References
Algar DA (2006) A summary of research undertaken to identify non-target risks in the use of the feral cat bait eradicat and encapsulation of the toxin. Department of Environment and Conservation, Perth, WA. Available at https://library.dbca.wa.gov.au/#record/89316 [accessed 24 November 2023]
Algar D, Angus GJ, Williams MR, Mellican AE (2007) Influence of bait type, weather and prey abundance on bait uptake by feral cats (Felis catus) on Peron Peninsula, Western Australia. Conservation Science Western Australia 6(1), 109-149 Available at https://library.dbca.wa.gov.au/static/Journals/080559/080559-06.005.pdf.
| Google Scholar |
Algar D, Onus M, Hamilton N (2013) Feral cat control as part of rangelands restoration at lorna glen (matuwa), Western Australia: the first seven years. Conservation Science Western Australia 8(3), 367-381.
| Google Scholar |
Bain K, Wayne A, Virgo M (2021) A bait efficacy trial for the management of feral cats (Felis catus) in karri forest areas after timber harvesting activities. Report prepared for forest products commission, Manjimup. Python Ecological Services, Walpole Western Australia. Available at https://library.dbca.wa.gov.au/static/FullTextFiles/072611.pdf [accessed 27 November 2023]
Barcelos DC, Alvarenga GC, Gräbin DM, Baccaro F, Ramalho EE (2023) Divergent effects of lure on multi-species camera-trap detections and quality of photos. Journal for Nature Conservation 71, 126317.
| Crossref | Google Scholar |
Bates D, Mächler M, Bolker B, Walker S (2015) Fitting linear mixed-effects models using lme4. Journal of Statistical Software 67(1), 1-48.
| Crossref | Google Scholar |
Bradley AJ (1997) Reproduction and life history in the red-tailed phascogale, Phascogale calura (marsupialia: dasyuridae): the adaptive-stress senescence hypothesis. Journal of Zoology 241(4), 739-755.
| Crossref | Google Scholar |
Brown JM, Hopkins AJM (1983) The kwongan (sclerophyllous shrublands) of Tutanning Nature Reserve, Western Australia. Australian Journal of Ecology 8(1), 63-73.
| Crossref | Google Scholar |
Comer S, Speldewinde P, Tiller C, Clausen L, Pinder J, Cowen S, Algar D (2018) Evaluating the efficacy of a landscape scale feral cat control program using camera traps and occupancy models. Scientific Reports 8, 5335.
| Crossref | Google Scholar | PubMed |
Comer S, Clausen L, Cowen S, Pinder J, Thomas A, Burbidge AH, Tiller C, Algar D, Speldewinde P (2020) Integrating feral cat (Felis catus) control into landscape-scale introduced predator management to improve conservation prospects for threatened fauna: a case study from the south coast of Western Australia. Wildlife Research 47(8), 762-778.
| Crossref | Google Scholar |
Cowan M, Moro D, Anderson H, Angus J, Garretson S, Morris K (2020) Aerial baiting for feral cats is unlikely to affect survivorship of northern quolls in the Pilbara region of Western Australia. Wildlife Research 47(8), 589-598.
| Crossref | Google Scholar |
de Tores PJ, Marlow N (2012) The relative merits of predator-exclusion fencing and repeated fox baiting for protection of native fauna: five case studies from Western Australia. In ‘Fencing for conservation: restriction of evolutionary potential or a riposte to threatening processes?’. (Eds MJ Somers, M Hayward) pp. 21–42. (Springer: New York, NY, USA) doi:10.1007/978-1-4614-0902-1_3
Department of Biodiversity, Conservation and Attractions (2021) Wheatbelt Region parks and reserves management plan 95 2021. Department of Biodiversity, Conservation and Attractions, Perth, WA, Australia. Available at https://nla.gov.au/nla.obj-2992081413 [accessed 24 November 2023]
Doherty TS, Hall ML, Parkhurst B, Westcott V (2022) Experimentally testing the response of feral cats and their prey to poison baiting. Wildlife Research 49(2), 137-146.
| Crossref | Google Scholar |
Dundas SJ, Adams PJ, Fleming PA (2014) First in, first served: uptake of 1080 poison fox baits in south-west Western Australia. Wildlife Research 41, 117-126.
| Crossref | Google Scholar |
Fancourt BA, Augusteyn J, Cremasco P, Nolan B, Richards S, Speed J, Wilson C, Gentle MN (2021) Measuring, evaluating and improving the effectiveness of invasive predator control programs: feral cat baiting as a case study. Journal of Environmental Management 280, 111691.
| Crossref | Google Scholar | PubMed |
Fancourt BA, Harry G, Speed J, Gentle MN (2022) Efficacy and safety of Eradicat® feral cat baits in eastern Australia: population impacts of baiting programmes on feral cats and non-target mammals and birds. Journal of Pest Science 95, 505-522.
| Crossref | Google Scholar |
Friend JA, Hill R, Macmahon B, Bell L, Button T, Mosen C, Hill S (2020) Are red-tailed phascogales (Phascogale calura) at risk from Eradicat® cat baits? Wildlife Research 47(8), 747-761.
| Crossref | Google Scholar |
Glen AS, Gentle MN, Dickman CR (2007) Non-target impacts of poison baiting for predator control in Australia. Mammal Review 37(3), 191-205.
| Crossref | Google Scholar |
Hetherington CA, Algar D, Mills H, Bencini R (2007) Increasing the target-specificity of ERADICAT® for feral cat (Felis catus) control by encapsulating a toxicant. Wildlife Research 34(6), 467-471.
| Crossref | Google Scholar |
Ivan JS, Newkirk ES (2016) CPW photo warehouse: a custom database to facilitate archiving, identifying, summarizing and managing photo data collected from camera traps. Methods in Ecology and Evolution 7(4), 499-504.
| Crossref | Google Scholar |
Legge S, Murphy BP, McGregor H, Woinarski JCZ, Augusteyn J, Ballard G, Baseler M, Buckmaster T, Dickman CR, Doherty T, Edwards G, Eyre T, Fancourt BA, Ferguson D, Forsyth DM, Geary WL, Gentle M, Gillespie G, Greenwood L, Hohnen R, Hume S, Johnson CN, Maxwell M, McDonald PJ, Morris K, Moseby K, Newsome T, Nimmo D, Paltridge R, Ramsey D, Read J, Rendall A, Rich M, Ritchie E, Rowland J, Short J, Stokeld D, Sutherland DR, Wayne AF, Woodford L, Zewe F (2017) Enumerating a continental-scale threat: how many feral cats are in Australia? Biological Conservation 206, 293-303.
| Crossref | Google Scholar |
Lenth R, Singmann H, Love J, Buerkner P, Herve M (2020) emmeans: estimated marginal means. R package version 1.4. 4. The American Statistician 34(4), 216-221.
| Google Scholar |
Lohr CA, Algar D (2020) Managing feral cats through an adaptive framework in an arid landscape. Science of The Total Environment 720, 137631.
| Crossref | Google Scholar | PubMed |
Marlow NJ, Thomas ND, Williams AAE, Macmahon B, Lawson J, Hitchen Y, Angus J, Berry O (2015a) Lethal 1080 baiting continues to reduce European Red Fox (Vulpes vulpes) abundance after more than 25 years of continuous use in south-west Western Australia. Ecological Management & Restoration 16(2), 131-141.
| Crossref | Google Scholar |
Marlow NJ, Thomas ND, Williams AAE, Macmahon B, Lawson J, Hitchen Y, Angus J, Berry O (2015b) Cats (Felis catus) are more abundant and are the dominant predator of woylies (Bettongia penicillata) after sustained fox (Vulpes vulpes) control. Australian Journal of Zoology 63(1), 18-27.
| Crossref | Google Scholar |
Martin GR, Twigg LE, Marlow NJ, Kirkpatrick WE, King the late DR, Gaikhorst G (2002) The acceptability of three types of predator baits to captive non-target animals. Wildlife Research 29(5), 489-502.
| Crossref | Google Scholar |
Mcilroy JC (1981) The sensitivity of Australian animals to 1080 poison. II. Marsupial and eutherian carnivores. Wildlife Research 8, 385-399.
| Crossref | Google Scholar |
Moore JF, Soanes K, Balbuena D, Beirne C, Bowler M, Carrasco-Rueda F, Cheyne SM, Coutant O, Forget P-M, Haysom JK, Houlihan PR, Olson ER, Lindshield S, Martin J, Tobler M, Whitworth A, Gregory T (2021) The potential and practice of arboreal camera trapping. Methods in Ecology and Evolution 12(10), 1768-1779.
| Crossref | Google Scholar |
Moseby K, Hodgens P, Bannister H, Mooney P, Brandle R, Lynch C, Young C, Jansen J, Jensen M (2021) The ecological costs and benefits of a feral cat poison-baiting programme for protection of reintroduced populations of the western quoll and brushtail possum. Austral Ecology 46(8), 1366-1382.
| Crossref | Google Scholar |
Moss ZN, O’Connor CE, Hickling GJ (1998) Implications of prefeeding for the development of bait aversions in brushtail possums (Trichosurus vulpecula). Wildlife Research 25(2), 133-138.
| Crossref | Google Scholar |
Murphy BP, Woolley L-A, Geyle HM, Legge SM, Palmer R, Dickman CR, Augusteyn J, Brown SC, Comer S, Doherty TS, Eager C, Edwards G, Fordham DA, Harley D, McDonald PJ, McGregor H, Moseby KE, Myers C, Read J, Riley J, Stokeld D, Trewella GJ, Turpin JM, Woinarski JCZ (2019) Introduced cats (Felis catus) eating a continental fauna: the number of mammals killed in Australia. Biological Conservation 237, 28-40.
| Crossref | Google Scholar |
Palmer R, Anderson H, Richards B, Craig MD, Gibson L (2021) Does aerial baiting for controlling feral cats in a heterogeneous landscape confer benefits to a threatened native meso-predator? PLoS ONE 16(5), e0251304.
| Crossref | Google Scholar | PubMed |
Pierson JC, Berry L, Alexander L, Anson J, Birkett M, Kemp L, Pascoe BA, Farquharson KA, Hogg CJ (2023) Adaptive genetic management of a reintroduction program from captive breeding to metapopulation management of an arboreal marsupial. Diversity 15(7), 848.
| Crossref | Google Scholar |
R Core Team (2022). R: A language and environment for statistical computing. R Foundation for Statistical Computing, Vienna, Austria. Available at https://www.R-project.org/
Ross R, Anderson B, Bienvenu B, Scicluna EL, Robert KA (2022) WildTrack: an IoT system for tracking passive-RFID microchipped wildlife for ecology research. Automation 3, 426-438.
| Crossref | Google Scholar |
Short J, Hide A, Stone M (2011) Habitat requirements of the endangered red-tailed phascogale, Phascogale calura. Wildlife Research 38, 359-369.
| Crossref | Google Scholar |
Twigg LE, King DR (1991) The impact of fluoroacetate-bearing vegetation on native Australian fauna: a review. Oikos 61(3), 412-430.
| Crossref | Google Scholar |
Wayne AF, Maxwell MA, Ward CG, Vellios CV, Ward BG, Liddelow GL, Wilson I, Wayne JC, Williams MR (2013) Importance of getting the numbers right: quantifying the rapid and substantial decline of an abundant marsupial, Bettongia penicillata. Wildlife Research 40(3), 169-183.
| Crossref | Google Scholar |
Woinarski JCZ, Murphy BP, Legge SM, Garnett ST, Lawes MJ, Comer S, Dickman CR, Doherty TS, Edwards G, Nankivell A, Paton D, Palmer R, Woolley LA (2017) How many birds are killed by cats in Australia? Biological Conservation 214, 76-87.
| Crossref | Google Scholar |
Woinarski JCZ, Murphy BP, Palmer R, Legge SM, Dickman CR, Doherty TS, Edwards G, Nankivell A, Read JL, Stokeld D (2018) How many reptiles are killed by cats in Australia? Wildlife Research 45(3), 247-266.
| Crossref | Google Scholar |
Woinarski JCZ, Legge SM, Woolley LA, Palmer R, Dickman CR, Augusteyn J, Doherty TS, Edwards G, Geyle H, McGregor H, Riley J, Turpin J, Murphy BP (2020) Predation by introduced cats Felis catus on Australian frogs: compilation of species records and estimation of numbers killed. Wildlife Research 47(8), 580-588.
| Crossref | Google Scholar |