Threatened stick-nest rats preferentially eat invasive boxthorn rather than native vegetation on Australia’s Reevesby Island
Annie A. Kraehe



A
B
C
D
Abstract
The incorporation of invasive plants into novel ecosystems often has negative effects, but it can also sometimes enhance ecosystem function. The threatened native rodent species Leporillus conditor (greater stick-nest rat) is extinct on the Australian mainland and now lives primarily on small islands off the coast of southern Australia. Many of these are degraded novel ecosystems invaded by African boxthorn (Lycium ferocissimum), a weed of national significance. However, L. conditor does not appear to be negatively affected by the presence of boxthorn, raising the question of how the two species co-exist.
To understand how L. conditor uses African boxthorn, we evaluated dietary composition of L. conditor on parts of Reevesby Island by comparing consumption of invasive boxthorn with that of native vegetation.
We identified three key vegetation types on the centre of the island and used point-intercept vegetation surveys to estimate relative availability of plant species in each. We then used micro-histological faecal analysis to estimate the proportions of each species in the diet of L. conditor, and quantified plant species selection by using selection ratios (use/availability).
Qualitative evidence of L. conditor activity suggested that it was mostly confined to vegetation with greater abundance of boxthorn than the other vegetation types (13.5%, compared with 5.7% total sampled vegetation). Furthermore, 51.7% of the faecal plant content and 11.8% of total sampled vegetation was African boxthorn, resulting in a selection ration for boxthorn of 4.4. Native species that appeared to be favoured food sources of L. conditor included Olearia axillaris, Myoporum insulare and Enchylaena tomentosa.
Stick-nest rats of Reevesby Island demonstrate a clear selection for African boxthorn, both in terms of diet (tested quantitatively) and nesting (from previous research and our field observations).
The strong selection of stick-nest rats for a declared noxious weed as its main food source and persistence of stick-nest rats on Reevesby Island require consideration with regards to vegetation management on islands where L. conditor occurs. More broadly, it highlights that some elements of novel ecosystems may have unexpected positive impacts on parts of original ecosystems.
Keywords: conservation, Leporillus, Lycium, invasions, island refuges, Manly selectivity, palaeoecology, plant cuticle, selection ratio.
Introduction
Novel ecosystems are an ensemble of organisms that are both self-sustaining and without historical precedent (Hobbs et al. 2013). They develop naturally as a result of biotic or abiotic changes, such as climate change, dispersal events, and the merging of continents. Humans have also caused the development of novel ecosystems, and at an unprecedented rate (Hobbs et al. 2009). Because novel ecosystems are defined by the presence of new (and usually invasive) organisms, they are synonymous with loss of biodiversity and reduced ecosystem value and function. This has certainly been the case in Australia (Carr 1993; Gross 1995; Dickman 1996; Centre for Agriculture and Bioscience International, CABI Compendium: Invasive species, see www.cabi.org/isc), where invasions are known to negatively affect on ecosystem function, with high economic costs (Bradshaw et al. 2021; Carneiro et al. 2024).
Unfortunately, returning ecosystems to their pre-invasion state is not always possible (Hobbs et al. 2009). However, invasive species may be useful to supplement natural ecosystems in cases where functionally equivalent native organisms and processes have been affected (Packer et al. 2016). This is particularly the case where invasive plant species dominate novel ecosystems, yet play important roles in maintaining ecosystem function. Examples of this include the problem of how to manage the invasive tamarisk shrub in the USA. Because this species has assumed an important role in providing habitat to a range of species, biocontrol measures to eradicate it have had negative effects on bird and insect fauna (Carothers et al. 2020). Other examples include the dune-stabilising function of invasive sedges (Wootton et al. 2005) or the documented benefit of invasive plants on pollinating insects (Kovács-Hostyánszki et al. 2022).
Because many invasive plants outcompete the surrounding vegetation through dense growth, they can benefit vertebrates by providing vegetation cover (Dutra et al. 2011). This is also the case in Australia, for example, where dense native but invasively expanding shrublands can be beneficial for small native mammals, possibly because they provide suitable undergrowth cover (Hradsky et al. 2015; Law et al. 2016). Some of these impacts can be restricted to just a single species; an example is the case of the highly invasive blackberry that aids bandicoots and other small mammals by providing a functional equivalent to the vegetation structure present in intact native ecosystems (Packer et al. 2016). Despite the well-known negative effects of invasive weeds to biodiversity and ecosystem values (Williams and West 2000; Pimentel et al. 2005; Pyšek and Richardson 2010; Hoffmann and Broadhurst 2016), it is therefore important to assess whether, and how, an invasive species benefits a native ecosystem or species.
A potential new case of a single species benefiting from a plant invasion is the near-threatened native Australian greater stick-nest rat (Leporillus conditor). L. conditor is the only survivor of two Leporillus species. Both went extinct on the Australian mainland soon after European invasion because of the introduction of feral predators, habitat degradation caused by livestock grazing, and competition with introduced herbivores (Copley 1999a). However, a single natural population of L. conditor was discovered on the Franklin Islands, located in Nuyt’s Archipelago, off the western coast of Eyre Peninsula (Wood Jones 1922). This was subsequently used as a source population for 10 translocation efforts, as part of the stick-nest rat recovery plan (Copley 1995; Short et al. 2019). Successful translocations occurred in novel ecosystems on St Peter Island and Reevesby Island in South Australia, and Salutation Island in Western Australia, which are free from feral predators such as cats and foxes (Copley 1999a). The success of this recovery plan resulted in the down-listing of this species’ conservation status from ‘Endangered’ in 1996 to ‘Vulnerable’ in 2008 to ‘Near Threatened’ in 2016, under the IUCN Red List of Threatened Species (Short et al. 2018).
The St Peter Island and Reevesby Island translocation sites are novel ecosystems that have been changed substantially by the introduction of cats (eradicated on Reevesby Island in the 1980s), over a century of sheep grazing, farming, and introduction of a range of weeds (Robinson et al. 1996a). The change in vegetation and legacy impacts of grazing are a concern for the survival of the species on the island. One of the most successful invasive weeds on Reevesby Island is African boxthorn, Lycium ferocissimum (Robinson et al. 1996a), which is classed as a Weed of National Significance because of its invasiveness, potential for spread, and economic and environmental impacts (Noble et al. 2014). L. ferocissimum forms dense thickets that can block access to water points and provide shelter for invasive animals, such as rabbits and foxes. Additionally, its invasive nature allows it to encroach on the nesting sites of native birds and mammals and displace native plant species that provide essential ecosystem functions (CABI Compendium: Invasive species, see www.cabi.org/isc). It is known to interfere with seal breeding on beaches in the Recherche Archipelago, Western Australia (Gross 1995), as well as penguin breeding on Motunau Island in New Zealand (CABI Compendium: Invasive species, see www.cabi.org/isc). However, the species is also a potential case for benefitting a single vertebrate species because it has been shown to provide better protection for little penguins against cats and foxes than does available native vegetation for (Woehler et al. 2021).
There is some evidence that L. conditor might be another beneficiary of L. ferocissimum infestation on the islands it inhabits. This infestation is so extensive that more stick-nest rats now live in boxthorn-infested novel ecosystems than in areas still dominated by native trees and shrubs (Short et al. 2019). Copley (1999b) found that stick-nests were most numerous around the bases of L. ferocissimum bushes, and, when the population reached its peak in 1997, multiple stick platforms were evident among the higher branches of many of these bushes (Copley 1999b; Short et al. 2019). This may be a strong sign of preference, as raised shelters are likely to be more difficult to build and more exposed to the elements and avian predators. Stick-nest rats may also favour L. ferocissimum on St Peter Island, as a 2006 survey of pitfall traps and Elliott traps at St Peter found that L. conditor was most abundant in localised patches of L. ferocissimum and Atriplex paludosa, despite this being a small minority of the island’s vegetation (Stewart 1996; Copley 1999b; Short et al. 2019).
Even though the extensive benefit of L. ferocissimum to L. conditor as a shelter has been indicated by previous studies, it is important to also assess whether it provides a suitable source of nutrition. This is particularly important in small areas such as Reevesby Island, where food is a critical resource and choices are likely to be limited. Any introduced species of herbivorous animal will need to adapt its diet to take advantage of the plant species available and this may differ considerably from the preferences in its original habitat. Given the uncertainty of the outcome of such introductions, it is therefore important to be able to quantify the food selections made.
In attempting to reconstruct the diet of L. conditor, including the potential use of invasive plant species, microhistological faecal analysis is an ideal method. This is an established technique for rodent diet analysis (McIntire 1989; McMurry et al. 1993; Castleberry et al. 2002; Bergstrom 2013; Newmaster et al. 2013; Soininen et al. 2013; Khanam et al. 2016), which relies on the identification of species-specific characteristics of plant cuticle in faecal samples. Cuticle is the waxy, non-cellular layer that conforms to the surface of a terrestrial leaf epidermis, protecting it from desiccation. Whereas most leaf cells are denatured by the digestion process, much of the cuticle remains, still bearing the imprint of the leaf epidermis (Jones and Krockenberger 2007), which can be diagnostic for the plant material eaten. Microhistological faecal analysis has the benefit of being inexpensive and non-invasive to the animals. It has some risk of bias owing to the differential digestion of plants with cuticles with varying capacities for preservation (Norbury 1988; Jones and Krockenberger 2007). However, it provides strong positive evidence of consumption, and is generally considered to be a reliable technique for most plant species (Todd and Hansen 1973; Anthony and Smith 1974; Norbury 1988; Ellis et al. 1999; Khanam et al. 2016; Pareja et al. 2021).
In this study, we combine point intercept vegetation surveys with microhistological investigations of the degree to which L. ferocissimum is used by the Reevesby Island L. conditor population. This allows us to ask what proportions of native plants and L. ferocissimum are available in areas of L. conditor activity, and whether this availability is reflected in the feeding patterns of L. conditor, and hence to understand the risks, and potential opportunities, of boxthorn infestation on this Vulnerable Australian native.
Materials and methods
Study site and history
Reevesby Island is located at 34.534°S, 136.281°E off the South Australian coast (refer to Fig. 1). It features four rocky lobes linked by narrow sandbars. L. conditor was originally present on the island, but went extinct before its reintroduction (Pedler and Copley 1993). Although the cause and timing of this population’s extinction remain unclear, it is thought to be linked to the introduction of feral cats (Felis catus) in the 1830s (Dickman 1996). The island was used for agricultural purposes and sheep grazing from 1838, until it was designated a conservation park in 1974. By this time, the landscape had been extensively altered (Robinson et al. 1996). Today, Reevesby Island is littered with abandoned farming equipment and populated by invasive plant species, including pasture grasses and Lycium ferocissimum. However, natives such as Myoporum insulare, Atriplex paludosa, and Threlkeldia diffusa are reclaiming the deserted pastures (Robinson et al. 1996). The eradication of the feral cat population in the 1980s facilitated the translocation of a L. conditor population in 1990. Annual monitoring of the population then took place from 1992 to 2000. This showed that the population peaked in 1998, with numbers fluctuating in boom–bust cycles between 1000 and 5000 individuals (Copley 1999b).
Field design
We use the word ‘nest’ to describe any bush (or dense vegetation cluster) showing signs of frequent and sustained use or occupancy by L. conditor (for examples, see Fig. 2). These signs include an abundance of faecal matter and multiple sets of fresh tracks, in addition to signs of gardening, such as the maintenance of an entrance and the construction and maintenance of ‘highways’ (many sets of tracks, following a distinct path that has, in places, been carved, like a tunnel, through the branches of surrounding bushes). Sampling was conducted by A. Kraehe and two volunteers over 6 days in July 2018 under Government of South Australia Permit to Undertake Scientific Research Number A26856-1.
To understand where L. conditor was living, the area 2.3 km north of the old homestead (34.539°S, 136.276°E) was searched for signs of stick-nest rat activity coordinates of all the nests we found were marked (see Fig. 1). This methodology allowed us to rapidly identify the areas that L. conditor favoured and the areas they did not. Leporillus conditor activity was highest on a patch of vegetation sheltered in the sand dunes on the sand bar connecting the southernmost lobes of the island, and a patch of very similar vegetation on the sandbar connecting the southern lobes to the northern lobes. We focused our efforts on the more accessible, densely populated area between the two southern lobes.
Vegetation survey
To assess the vegetation available to L. conditor on Reevesby Island, the island was divided into three distinct vegetation communities by identifying visual differences in satellite imagery. During the survey, it was then confirmed that each vegetation type contained a distinctive subset of plant species (Table 1). The vegetation types were then mapped using satellite imagery by using the labels ‘Vegetation types 1–3’ (see Fig. 1; description of their composition is in the Results). Within each community, vegetation cover was measured as a proxy for availability by using point-intercept plots, based on the Ausplots manual (White et al. 2012) as a guide. GPS points were located and marked using google maps on a Google Pixel 2 mobile device. These data points are accurate to ~5–10 m. Therefore, to ensure accurate lengths and distances of transect lines on the ground, we relied on measuring tape as described below.
Species | Plot 1 (%) | Plot 2 (%) | Plot 3 (%) | Total sampled vegetation (%) | |
---|---|---|---|---|---|
Atriplex paludosa | 0.54 | 0.00 | 17.53 | 4.08 | |
Carpobrotus rossii | 1.08 | 6.33 | 0.00 | 2.72 | |
Dianella revoluta | 0.54 | 2.53 | 2.06 | 1.59 | |
Enchylaena tomentosa | 1.61 | 0.00 | 10.31 | 2.95 | |
Euphorbia paralias | 0.00 | 0.00 | 0.00 | 0.00 | |
Ficinia nodosa | 2.15 | 0.00 | 0.00 | 0.91 | |
Leucophyta brownii | 5.38 | 0.00 | 1.03 | 2.49 | |
Lycium ferocissimum | 11.83 | 15.19 | 0.00 | 10.43 | |
Muehlenbeckia gunnii | 34.41 | 3.16 | 3.09 | 16.33 | |
Myoporum insulare | 8.60 | 24.05 | 10.31 | 14.51 | |
Nitraria billardierei | 0.00 | 0.63 | 0.00 | 0.23 | |
Olearia axillaris | 7.53 | 12.03 | 13.40 | 10.43 | |
Rhagodia crassifolia | 1.08 | 0.63 | 0.00 | 0.68 | |
Salicornia quinqueflora | 0.00 | 0.00 | 20.62 | 4.54 | |
Senecio lautus | 5.91 | 0.63 | 0.00 | 2.72 | |
Spinifex hirsutus | 1.61 | 1.27 | 0.00 | 1.13 | |
Tetragonia implexicoma | 17.20 | 31.01 | 16.49 | 22.00 | |
Threlkeldia diffusa | 0.54 | 1.90 | 0.00 | 0.91 |
‘Total sampled vegetation’ is the summary of the percentage cover for each plot, divided by the number of plots. The percentages in Plot 1 are the same as the percentages of available vegetation in Fig. 3.
Plot 1 was designed to cover an area of high Leporillus activity. Using Google Maps satellite imagery, we placed a 50 × 50 m plot over this patch of vegetation and generated GPS coordinates for each corner. We then located each corner and marked out the plot by using pegs and a 100 m tape. We then used a 50 m tape to create six parallel transects within this plot, each 50 m long and spaced 10 m apart, oriented in a north–south direction. Note that, although the work was done within the 50 × 50 GPS-based plot, the distances of the transect lines over the ground were shorter because the landscape relief shortened the distances over-ground relative to the two-dimensional satellite image (as visible by the rectangular shape, rather than square shape, of the transect area in Fig. 1). Measurement points or ‘intersects’ were established at 1 m intervals along each transect. At each ‘intersect’, a vertical 5 m staff was positioned, and every plant species touching the staff was recorded. The height at which each species made contact was also noted, recording only the maximum height in instances of multiple contacts by one species. Vegetation cover was calculated by dividing the number of touches for each plant species by the total number of touches.
Plots 2 and 3 contained vegetation communities near Plot 1, but with no evidence of L. conditor activity. These were 20 × 50 m in size, and consisted of three parallel 50 m transects, spaced 10 m apart. Plot 2 ran parallel with the beach in the north–south direction, on the eastern side of Plot 1, for the purpose of sampling Vegetation type 2. Plot 3 ran in an east–west direction, on the southern side of Plot 1, and was selected for the purpose of sampling Vegetation type 3.
Specimen samples
To identify plant cuticle in L. conditor faecal pellets, we created a reference dataset of cuticle morphologies from plants found on Reevesby Island. Plant vouchers for the reference data set were collected, labelled, and pressed according to the vouchering method described in Ausplots (White et al. 2012). Plants were identified using standard identification books (Berkinshaw 2010; Saunders 2018) and a list of plant species on Reevesby Island was generated using NatureMaps (ver. 3.0, Government of South Australia, Department for Environment and Water, see https://data.environment.sa.gov.au/NatureMaps/Pages/default.aspx). Additional tissue samples for each plant species (leaves and stems, as well as fruits and/or flowers where available) were labelled and stored in 100% ethanol in vials at the University of Adelaide. Leaf and flower tissues were processed and made into slides by A. Kraehe using the protocol outlined in Storr (1961).
A minimum of 10 faecal pellets was collected per nest. At each nest, fresh faecal pellets were collected using forceps, labelled with the nest number and location, and stored dry in paper envelopes. Pellets were considered fresh if they were moist, malleable, and dark in appearance. The first step was to wash faecal pellets in cold reverse-osmosis-treated water to minimise contamination. The disaggregation procedure conventionally dissolves the faecal pellets in warm water (Pearson and Dodson 1993; McCarthy et al. 1996). However, this method proved ineffective for fresh faecal pellets, resulting in breakage of cuticle, as well as being slow and labour intensive. Instead, we developed a more reliable protocol that resembled the treatment of the reference cuticles, as follows: faecal pellets were placed into labelled test tubes with a mixture of two parts 35% hydrogen peroxide (w/v) and one part 80% ethanol (v/v) at 90°C until the pellet dissolved. It was often necessary to use forceps to gently pull the pellet apart, breaking the mucous membrane coating. Any remaining aggregation, usually owing to adhesion to mucous membrane, was easily dealt with by using light water pressure and a small brush over a sieve. The remaining material was a combination of plant cuticle, seeds, small pieces of wood and bark, lignin coils, small rocks, and other plant material, such as mesophyll. This was then washed and stained using toluidine blue for 1–2 min and rinsed again. The dissolved scat was then placed in a petri dish to be manually cleaned and sorted under a dissecting microscope. Seeds and woody material were separated, labelled, and placed in long-term storage. Once cleaned and sorted, all cuticle was transferred to microscope slides, smoothed flat with a brush, covered with a drop of phenol glycerine jelly warmed to 60°C and then covered with glass coverslips, and inspected under a AX70 (Olympus, Australia) microscope with a UC50 (Olympus) camera attached, and CellSens (Olympus) image software. Any image stacking was undertaken using Helicon Focus (ver. 7.6.4, see https://www.heliconsoft.com/).
Plant cuticle from the scat material was prepared and identified with reference to plant voucher slides. It was quantified by A. Kraehe by counting square millimetres covered of each species on each slide by using a microscopic grid. This was then calculated into percentage values for further analysis.
Identification of cuticle
To determine what plant species L. conditor was consuming, it was necessary to have a systematic method for comparing the fragments of cuticle in the faecal pellets with the reference cuticle, for identification. Because of the fragmented nature of the digested cuticle, we sought multiple identification characters to ensure that we correctly identified as much of the cuticle as possible. The key identifiers used in this study were differential absorption of stain, unspecialised epidermal cells, stomata, subsidiary cells and trichomes. The methods for identifying cuticle on the basis of differential absorption of stain and unspecialised epidermal cells are outlined in Storr (1961) and Metcalfe and Chalk (1950) respectively. We measured identifiers for each species and created an identification table for key plant species on the island (Supplementary material Table S1).
Statistical analysis
To understand the selective consumption of plants by L. conditor on Reevesby Island, we used Design I, described by Manly et al. (2002) as a statistical design for quantifying habitat use and availability at the population level. We used the widesI function of the adehabitatHS R package (ver. 0.3.14, C. Calenge and M. Basille, see https://cran.r-project.org/package=adehabitatHS) to determine resource selection by using Pearson’s Chi-Square test (χ2), and a log-likelihood Chi-Square test. Pearson’s Chi-Square test tests a null hypothesis that L. conditor consumes plants in proportion to their availability in the environment (allowing for a degree of error that is dependent on variation and sample size). The log-likelihood Chi-Square test computes the goodness of fit of the null hypothesis versus the alternative hypothesis on the basis of the ratio of their likelihoods for each plant species. If the null hypothesis is supported by the observed data, the two likelihoods should not differ by more than sampling error.
Selection was estimated using the Manly selectivity measure (wi) with Bonferroni confidence intervals proposed by Manly et al. (2002), as follows:
where oi is the proportion of plant species present in scats and πi is the proportion of available plant species in Category i (plant species). The selection ratio for a species is therefore
A value of 1 means that a plant species was consumed in proportion to its availability. Values of >1 imply selection (use greater than availability), whereas values of <1 imply avoidance (use less than availability). The selection ratio (wi) was computed using adehabitatHS (ver. 0.3.14) package with Design I data type in the R software (ver. 3.6.1, R Foundation for Statistical Computing, Vienna, Austria, see https://www.r-project.org/).
Results
For all raw data and codes, refer to Supplementary material. Three vegetation types were present in the areas we surveyed (see Materials and methods).
Vegetation type 1 is the least abundant within the study area. It is located where there is some protection from the strong ocean winds and salt spray. The dominant species are Myoporum insulare and Lycium ferocissimum (as medium to large bushes) and Muehlenbeckia gunnii and Tetragonia implexicoma (as ground cover and in the canopy as creepers/vines). Leporillus faecal pellets and tracks are a common occurrence within patches of this vegetation type, but rarely found outside of them.
Vegetation type 2 is the most prevalent in the study area, occupying the exposed dunes. It is defined by the dominance of Leucophyta brownii and Olearia axillaris (as low shrubs) and Spinifex hirsutus (tufty grass). There is a substantial variation in density within this group; however, dominant species remain a fixed parameter. Because of accessibility and time constraints, this vegetation type was sampled in a low-density area.
Vegetation type 3 occupies low-lying salt and mud flats on the island in areas that are likely to become hypersaline as a result of inundation and subsequent evaporation of seawater. The dominant species are Salicornia quinqueflora (a small succulent shrub), Atriplex paludosa (marsh saltbush), Tetragonia implexicoma (bower spinach) and Olearia axillaris (coastal daisy-bush).
Plant species availability, use and selection by L. conditor
Vegetation availability and use are shown in Fig. 3, and the selection ratio is presented in Fig. 4. L. ferocissimum is by far the most used resource, making up 51.7% of the used material, despite representing only 11.8% of the available vegetation in Plot 1 and 10.43% of total sampled vegetation, as measured in the point-intercept survey (Table 2). This gives it a selection ratio of nearly 4.4 (Table 2).
Percentage of available vegetation (as per relative abundance, see Materials and methods; green) vs use (purple) by Leporillus conditor. Standard errors are shown in black. Data are not shown for species not found in Plot 1.
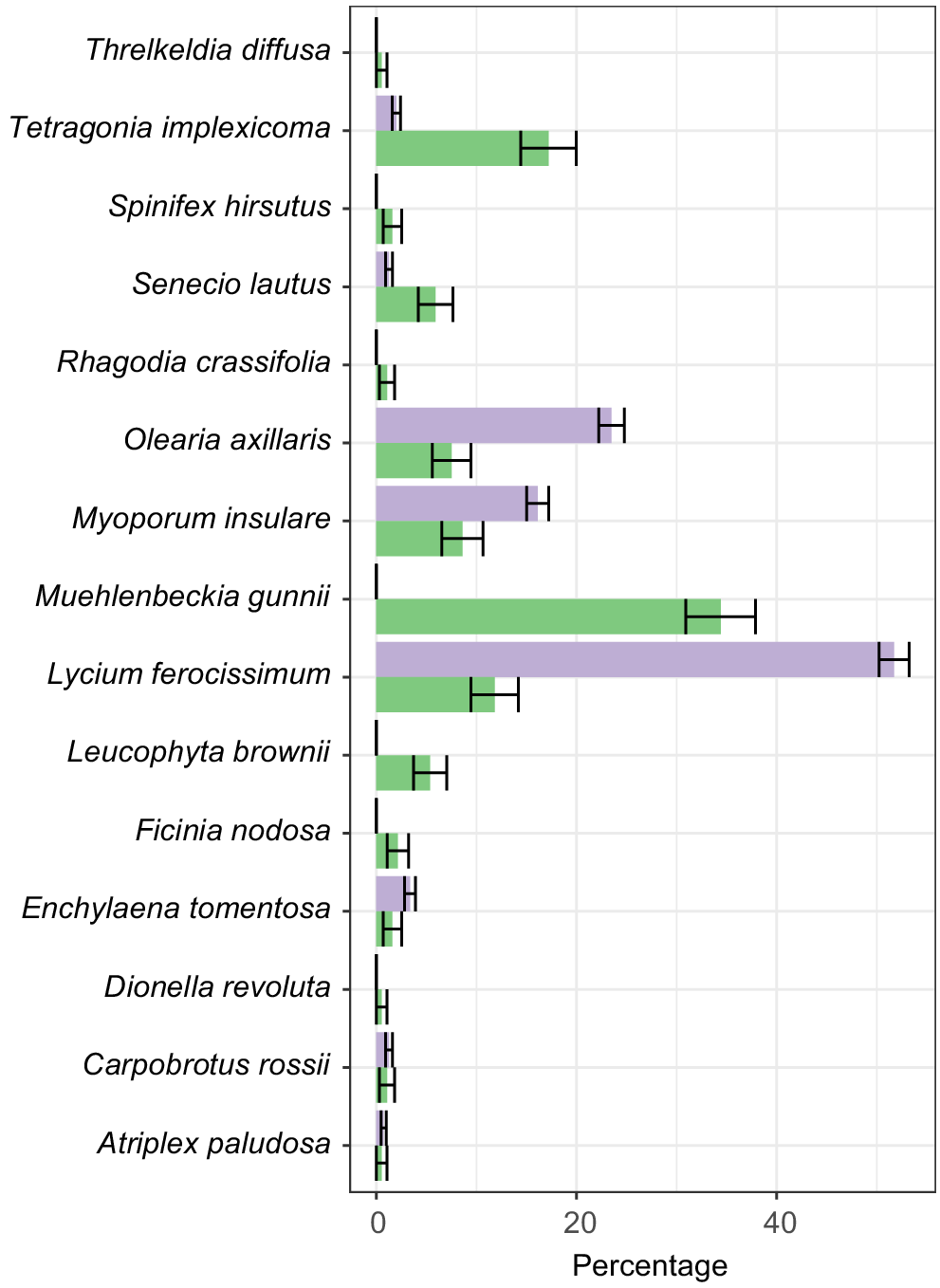
Selective use of plant species by Leporillus conditor in Plot 1. Graph showing selection ratios on the X-axis and plant species on the Y-axis, with 95% confidence intervals as horizontal lines. The dotted vertical line marks a selection ratio of 1. Species on the right-hand side of the dotted line have a selection ratio of >1, which means that they were statistically more used than they are available. This indicates selective use by Leporillus conditor.
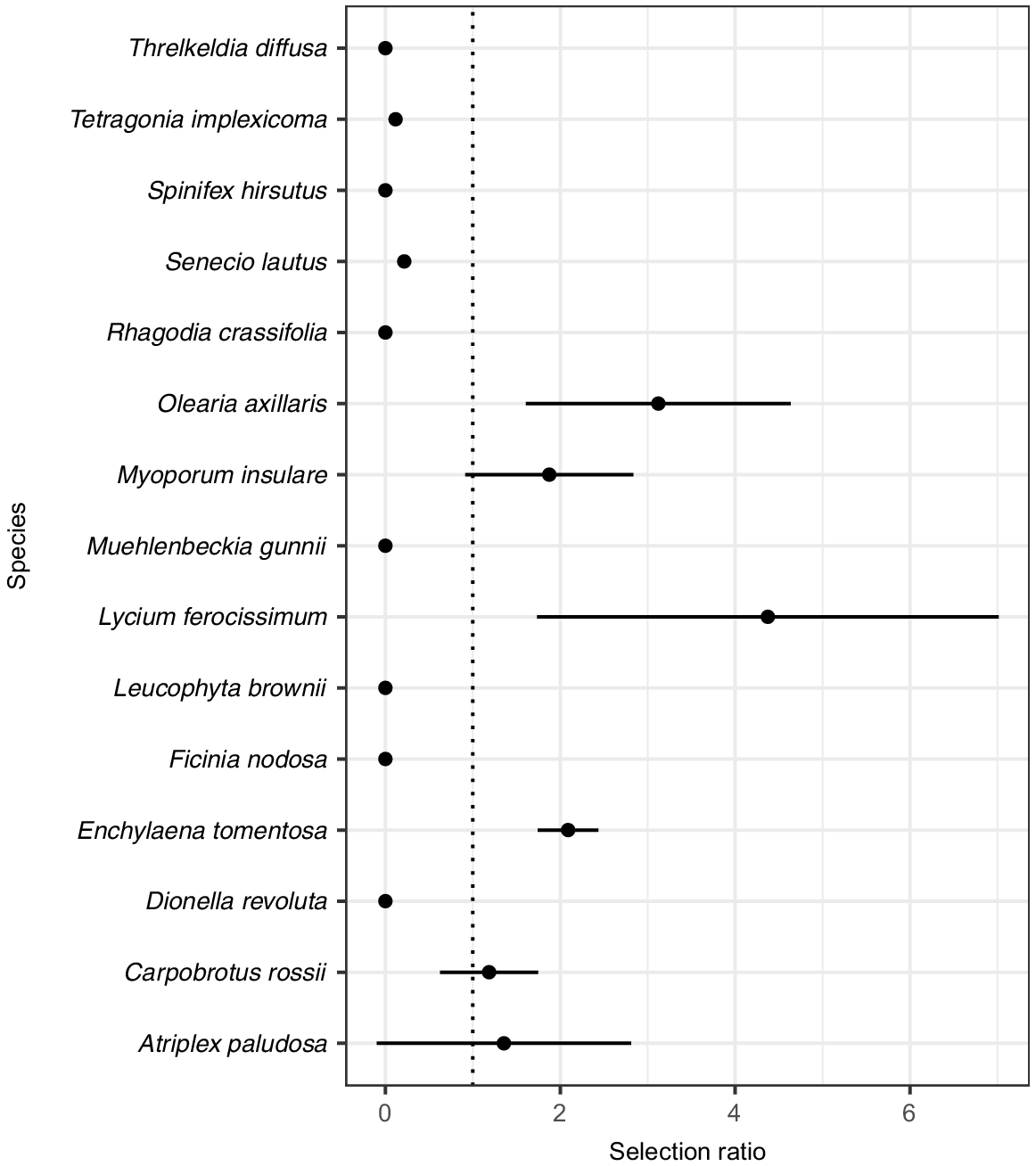
Species | Used | Available | Selection ratio | s.e. | P-value | |
---|---|---|---|---|---|---|
Atriplex paludosa | 0.007 | 0.005 | 1.355 | 1.433 | 0.804 | |
Carpobrotus rossii | 0.013 | 0.011 | 1.186 | 0.891 | 0.835 | |
Dianella revoluta | 0.000 | 0.005 | 0 | – | – | |
Enchylaena tomentosa | 0.034 | 0.016 | 2.089 | 1.243 | 0.381 | |
Ficinia nodosa | 0 | 0.022 | 0 | – | – | |
Leucophyta brownii | 0 | 0.054 | 0 | – | – | |
Lycium ferocissimum | 0.517 | 0.118 | 4.374 | 0.885 | 0.000 | |
Muehlenbeckia gunnii | 0 | 0.344 | 0 | – | – | |
Myoporum insulare | 0.161 | 0.086 | 1.874 | 0.466 | 0.061 | |
Olearia axillaris | 0.235 | 0.075 | 3.122 | 0.82 | 0.01 | |
Rhagodia crassifolia | 0 | 0.011 | 0 | – | – | |
Senecio lautus | 0.013 | 0.059 | 0.216 | 0.085 | 0.000 | |
Spinifex hirsutus | 0 | 0.016 | 0 | – | – | |
Tetragonia implexicoma | 0.02 | 0.172 | 0.116 | 0.031 | 0.000 | |
Threlkeldia diffusa | 0 | 0.005 | 0 | – | – |
‘Used’, total proportion of each species in the total faecal plant material; ‘available’ represents the total proportion of each species in the point-intercept surveys. Selection ratio is the product of ‘used’ divided by ‘available’.
Because of its abundant representation in the scat material, L. ferocissimum was broken into the following two subclasses on the basis of cuticle type: ‘leaf’, and ‘flower’. Leaf cuticle makes up 62.1% (353 out 568 cuticle pieces) of L. ferocissimum cuticle, and the remaining 37.9% (215 out of 568 cuticle pieces) can be attributed to flowers (Table S2).
Atriplex paludosa (marsh saltbush), Carpobrotus rossii (Australian pig face), L. ferocissimum, Enchylaena tomentosa (ruby saltbush), Olearia axillaris (coastal daisy-bush), and Myoporum insulare (native juniper) have selection ratios of >1, although this is not always statistically significant (Fig. 4, Table 2). Tetragonia implexicoma (bower spinach) and Muehlenbeckia gunnii (coastal lignum) are the only species that seem to be selected against. They make up 17.2 and 34.4% of the total available vegetation in Plot 1, but only 2 and 0% of the used resources (Fig. 3).
Of all the available species, the succulents have the most fragile cuticle, and are the most susceptible to damage during digestion and laboratory processes. As such, it may be reasonable to class the ‘used’ data for these species as missing, or incomplete. However, studies have shown that the effects of differential preservation are usually minor (Todd and Hansen 1973; Anthony and Smith 1974; Norbury 1988; Ellis et al. 1999; Khanam et al. 2016; Pareja et al. 2021) and, as the other plant cuticles are more robust, they are unlikely to be affected by this secondary selective process.
Discussion
We found clear evidence that L. conditor (stick-nest rat) selected the invasive Lycium ferocissimum (African boxthorn) as a food resource. Although it was not quantified, the density of L. conditor activity in vegetation dominated by L. ferocissimum suggests that it is also the preferred habitat for L. conditor, as already shown by previous research (Copley 1995; Short et al. 2019). Importantly, L. ferocissimum was the most strongly selected plant species and preferred even over succulent species such as the ruby saltbush, which form the majority of L. conditor’s diet in the comparatively natural or restored habitats such as the Franklin Islands and the Arid Recovery native-mammal re-introduction site of northern South Australia (Read 1984; Robinson et al. 1996a; Copley 1999a; Ryan et al. 2003).
It is possible that flowers are more palatable than other parts of the plant and could have increased selective use of L. ferocissimum. Flowers were abundant despite the collection period occurring in winter, outside the species’ usual summer-flowering period (Erkelenz 1993). However, it seems more likely that this preference is driven by the habitat choice of L. conditor, because the species seems to be a dietary generalist and consumes a variety of different plants, depending on location and season (Read 1984).
The benefit of L. ferocissimum as a food plant is possibly accompanied by the further preference as a shelter by L. conditor, although this was not quantified here. At the Arid Recovery native-mammal re-introduction site (Moseby et al. 2011), L. conditor demonstrated a preference for low, dense shrubs as the base for nest sites (O’Neill 1999; Short et al. 2019), likely owing to the protection they offer against predators, especially avian predators (Copley 1988; O’Neill 1999). Even though L. ferocissimum provides L. conditor with low, dense cover on Reevesby Island, so could other plants that grow within the same microhabitat. However, L. conditor may favour L. ferocissimum bushes because the large thorns provide more protection from predators than do the plants that are native to the animal’s offshore island refuges. A preference for thorns and spines by L. conditor is supported by Short et al. (2018), who found that very prickly Acacia tetragonophylla bushes were the primary nesting shrubs used by L. conditor at Heirisson Prong (introduced in 1999, last sighting in 2007), in Shark Bay, Western Australia.
Despite the successful adaptation of L. conditor to boxthorn invasion, it is difficult to judge the degree to which L. ferocissimum is beneficial in replacing pre-disturbance structural complexity and food resources on Reevesby Island, because there is no historical reference for pre-colonial vegetation on the island. However, the location of the only natural Leporillus population, on the Franklin Islands, offers an interesting comparison. Descriptions of the vegetation there appear to be very similar to those on Reevesby Island, except that they do not contain L. ferocissimum (Osborn 1922; Read 1984; Robinson et al. 1996a). Interestingly, on the Franklin Islands, stick-nest rats also show a clear preference for a habitat that is not part of the native vegetation, as population density of L. conditor appears to be highest among the granite slabs along the coastline, rather than more heavily vegetated areas (Read 1984). Areas of dense vegetation cover are also used, but appear to have lower population densities (Read 1984). It therefore remains unclear whether L. conditor’s preference for nesting in L. ferocissimum thickets is due to its efficiency as a shelter, as it is for other vertebrates (Dutra et al. 2011; Hradsky et al. 2015; Law et al. 2016; Packer et al. 2016), or whether it relates to a strong selection for L. ferocissimum as a food source.
Evidence of stick-nest rats using native vegetation for food and habitat on Reevesby Island and the Franklin Islands (Read 1984) suggests that L. conditor on Reevesby Island are unlikely to require boxthorn for their survival. However, the fact that they appear to favour introduced vegetation as habitat at both locations emphasises the lack of information we have on this species. Part of the issue is that all translocation sites, and remnant natural populations, are located on the very fringe of L. conditor’s former distribution (Copley 1999a) and are unlikely to represent their optimal environment. In addition, there is no evidence of a preference for thorned plants preserved in fossilised stick-nest rat middens, suggesting that more research is required as to the original habitat preferences of this species (Pearson and Dodson 1993; McCarthy et al. 1996; McCarthy 1999; Pearson 1999; Allen et al. 2000; McCarthy and Head 2001; Pearson et al. 2001; Pearson and Betancourt 2002; Webeck and Pearson 2005). Lack of good understanding of habitat preference is an important issue in translocations, particularly in Australia and surrounding Oceanian areas where translocation sites are often subject to extensive weed invasions (Morris et al. 2021). Another recent Australian example is the single remaining population of Shark Bay mouse (Pseudomys gouldi), which also has a broader range of suitable habitats than was previously assumed (Palmer et al. 2024).
The apparent beneficial effects of African boxthorn infestation on stick-nest rats are consistent with other observations of beneficial interactions between invasives and native ecosystem components (Azmi and Jennings 2013; Packer et al. 2016; Carothers et al. 2020). This is an important consideration when assessing the economic impact of invasions and their control, particularly when expensive but inefficient measures result in a removal of beneficial effects (Boltovskoy et al. 2022). In the case of stick-nest rats, this might be a particular issue in times of warming climates, which makes it particularly important for the species to have sufficient cover (Onley et al. 2022). However, the beneficial effects of improved plant cover from invasive species (Dutra et al. 2011) need to be balanced against the otherwise detrimental effect of these often very destructive species. It is also not clear whether the benefits will persist when a novel ecosystem has reached a stable state. African boxthorn is highly invasive and, if left unchecked, may continue to spread, displacing native vegetation, and resulting in decreased ecosystem diversity and function that might ultimately also affect stick-nest rats themselves. It is even possible that the feeding preferences of L. conditor facilitate the invasion of boxthorn because of its potential to spread the seed through its faeces, similar to observations that re-introductions of burrowing mammals can aid the spread of invasive plants (Palmer et al. 2020). This highlights the importance of considering the potential negative outcomes of translocation efforts in cases where translocated species interact with novel ecosystems in a way that is detrimental to the overall ecosystem function (Palmer et al. 2020).
Our study has shown an unexpected selection for invasive L. ferocissimum on Reevesby Island. Together with the species’ apparent preference for granite slabs on Franklin Island (Read 1984), this has provided important clues as to the importance of vegetation type, terrain, and geology in identifying potential future translocation sites. For example, the ability of L. conditor to switch to a new food plant suggests substantial dietary flexibility as long as suitable succulent vegetation and possibly spiny shelter plants are present. The preference for granite slabs exhibited on Franklin Island is aligned with their historical use of rock overhangs as shelters, which is reflected by the presence of rock-shelter middens across Australia (e.g. Copley 1988). Similar geological landscape features might therefore be an additional predictor of mainland re-introduction success. Thus, despite insights gained from recent studies, our lack of understanding of the historical habitat of stick-nest rats suggests that assessments of the pre-colonial landscapes of previous occurrence sites (such as including midden sites and record maps) will be important for future translocation success.
Data availability
The raw vegetation survey and cuticle identification data for this paper, and the code to replicate Figs 3 and 4 and Table 2, are available in the supplementary material. A preprint version of this article is available in Kraehe et al. (2024).
Declaration of funding
V. Weisbecker was funded by the Australian Research Council Centre of Excellence for Australian Biodiversity and Heritage (CE170100015) and Future Fellowship (FT180100634).
Acknowledgements
We thank field volunteers Baillie Trenwith, Holly Kraehe, Czes Klopotowski, and two anonymous reviewers.
References
Allen V, Head L, Medlin G, Witter D (2000) Palaeo-ecology of the Gap and Coturaundee Ranges, western New South Wales, using stick-nest rat (Leporillus spp.) (Muridae) middens. Austral Ecology 25, 333-343.
| Crossref | Google Scholar |
Anthony RG, Smith NS (1974) Comparison of rumen and fecal analysis to describe deer diets. The Journal of Wildlife Management 535-540.
| Crossref | Google Scholar |
Azmi WA, Jennings J (2013) The impact of management practices of exotic willows (Salix spp.) on aquatic invertebrate communities in South Australian freshwater streams. Journal of Sustainability Science and Management 8, 43-52.
| Google Scholar |
Bergstrom BJ (2013) Would East African savanna rodents inhibit woody encroachment? Evidence from stable isotopes and microhistological analysis of feces. Journal of Mammalogy 94, 436-447.
| Crossref | Google Scholar |
Boltovskoy D, Guiaşu R, Burlakova L, Karatayev A, Schlaepfer MA, Correa N (2022) Misleading estimates of economic impacts of biological invasions: including the costs but not the benefits. Ambio 51, 1786-1799.
| Crossref | Google Scholar | PubMed |
Bradshaw CJA, Hoskins AJ, Haubrock PJ, Cuthbert RN, Diagne C, Leroy B, Andrews L, Page B, Cassey P, Sheppard AW, Courchamp F (2021) Detailed assessment of the reported economic costs of invasive species in Australia. NeoBiota 67, 511-550.
| Crossref | Google Scholar |
Carneiro L, Hulme PE, Cuthbert RN, Kourantidou M, Bang A, Haubrock PJ, Bradshaw CJA, Balzani P, Bacher S, Latombe G, Bodey TW, Probert AF, Quilodrán CS, Courchamp F (2024) Benefits do not balance costs of biological invasions. BioScience 7, 340-344.
| Crossref | Google Scholar |
Carothers SW, Johnson RR, Kingsley KJ (2020) A naturalized riparian ecosystem: consequences of Tamarisk leaf beetle (Diorhabda spp.) biocontrol. In ‘Riparian research and management: past, present, future. Vol. 2’. (Eds SW Carothers, RR Johnson, DM Finch, KJ Kingsley, RH Hamre) pp. 18–46. (US Department of Agriculture, Forest Service, Rocky Mountain Research Station: Fort Collins, CO, USA)
Castleberry NL, Castleberry SB, Ford WM, Wood PB, Mengak MT (2002) Allegheny woodrat (Neotoma magister) food habits in the central Appalachians. The American Midland Naturalist 147, 80-92.
| Crossref | Google Scholar |
Copley P (1999a) Natural histories of Australia’s stick-nest rats, genus Leporillus (Rodentia: Muridae). Wildlife Research 26, 513-539.
| Crossref | Google Scholar |
Dutra HP, Barnett K, Reinhardt JR, Marquis RJ, Orrock JL (2011) Invasive plant species alters consumer behavior by providing refuge from predation. Oecologia 166, 649-657.
| Crossref | Google Scholar | PubMed |
Ellis W, Carrick F, Lundgren P, Veary A, Cohen B (1999) The use of faecal cuticle examination to determine the dietary composition of koalas. Australian Zoologist 31, 127-133.
| Crossref | Google Scholar |
Gross C (1995) Conservation biology in Australia and Oceania. Pacific Conservation Biology 2, 200-201.
| Crossref | Google Scholar |
Hobbs RJ, Higgs E, Harris JA (2009) Novel ecosystems: implications for conservation and restoration. Trends in Ecology & Evolution 24, 599-605.
| Crossref | Google Scholar | PubMed |
Hoffmann BD, Broadhurst LM (2016) The economic cost of managing invasive species in Australia. NeoBiota 31, 1-18.
| Crossref | Google Scholar |
Hradsky BA, Loschiavo J, Hradsky M, Di Stefano J (2015) Shrub expansion alters forest structure but has little impact on native mammal occurrence. Austral Ecology 40, 611-624.
| Crossref | Google Scholar |
Jones KMW, Krockenberger AK (2007) Determining the diet of cryptic folivores: an assessment of diet analysis techniques using the green ringtail possum (Pseudochirops archeri) as a case study. Wildlife Research 34, 352-358.
| Crossref | Google Scholar |
Khanam S, Howitt R, Mushtaq M, Russell JC (2016) Diet analysis of small mammal pests: a comparison of molecular and microhistological methods. Integrative Zoology 11, 98-110.
| Crossref | Google Scholar |
Kovács-Hostyánszki A, Szigeti V, Miholcsa Z, Sándor D, Soltész Z, Török E, Fenesi A (2022) Threats and benefits of invasive alien plant species on pollinators. Basic and Applied Ecology 64, 89-102.
| Crossref | Google Scholar |
Kraehe AG, Weisbecker V, Hill R, Hill K (2024) Threatened stick-nest rats preferentially eat invasive boxthorn rather than native vegetation on Australia’s Reevesby Island. ResearchSquare 2024, version 2. [Preprint, posted 23 May 2024].
| Crossref | Google Scholar |
Law B, Brassil T, Gonsalves L (2016) Recent decline of an endangered, endemic rodent: does exclusion of disturbance play a role for Hastings River mouse (Pseudomys oralis)? Wildlife Research 43, 482-491.
| Crossref | Google Scholar |
McCarthy L, Head L (2001) Holocene variability in semi-arid vegetation: new evidence from Leporillus middens from the Flinders Ranges, South Australia. The Holocene 11, 681-689.
| Crossref | Google Scholar |
McCarthy L, Head L, Quade J (1996) Holocene palaeoecology of the northern Flinders Ranges, South Australia, based on stick-nest rat (Leporillus spp.) middens: a preliminary overview. Palaeogeography, Palaeoclimatology, Palaeoecology 123, 205-218.
| Crossref | Google Scholar |
McMurry ST, Lochmiller RL, Boggs JF, Leslie DM, Jr, Engle DM (1993) Opportunistic foraging of eastern woodrats (Neotoma floridana) in manipulated habitats. American Midland Naturalist 325-337.
| Crossref | Google Scholar |
Morris SD, Brook BW, Moseby KE, Johnson CN (2021) Factors affecting success of conservation translocations of terrestrial vertebrates: a global systematic review. Global Ecology and Conservation 28, e01630.
| Crossref | Google Scholar |
Moseby KE, Read JL, Paton DC, Copley P, Hill BM, Crisp HA (2011) Predation determines the outcome of 10 reintroduction attempts in arid South Australia. Biological Conservation 144, 2863-2872.
| Crossref | Google Scholar |
Newmaster SG, Thompson ID, Steeves RAD, Rodgers AR, Fazekas AJ, Maloles JR, McMullin RT, Fryxell JM (2013) Examination of two new technologies to assess the diet of woodland caribou: video recorders attached to collars and DNA barcoding. Canadian Journal of Forest Research 43, 897-900.
| Crossref | Google Scholar |
Norbury GL (1988) Microscopic analysis of herbivore diets – a problem and a solution. Wildlife Research 15, 51-57.
| Crossref | Google Scholar |
Onley IR, Austin JJ, Mitchell KJ, Moseby KE (2022) Understanding dispersal patterns can inform future translocation strategies: a case study of the threatened greater stick-nest rat (Leporillus conditor). Austral Ecology 47, 203-215.
| Crossref | Google Scholar |
Osborn TGB (1922) The flora and fauna of Nuyt’s Archipelago and the Investigator Group. No. 3. A sketch of the ecology of the Franklin Islands. Transactions of the Royal Society of South Australia 3, 194-206.
| Google Scholar |
Packer JG, Delean S, Kueffer C, Prider J, Abley K, Facelli JM, Carthew SMJB (2016) Native faunal communities depend on habitat from non-native plants in novel but not in natural ecosystems. Biodiversity and Conservation 25, 503-523.
| Crossref | Google Scholar |
Palmer BJ, Valentine LE, Page M, Hobbs RJ (2020) Translocations of digging mammals and their potential for ecosystem restoration: a review of goals and monitoring programmes. Mammal Review 50, 382-398.
| Crossref | Google Scholar |
Palmer BJ, Cowen SJ, Bourne AR (2024) Not so fussy after all: Shark Bay mouse (Pseudomys gouldii) recorded using a range of habitat types on Faure Island. Australian Mammalogy 46, AM23038.
| Crossref | Google Scholar |
Pareja J, Espunya C, Baraza E, Bartolomé J (2021) Complementarity between microhistological analysis and PCR-capillary electrophoresis in diet analysis of goats and cattle using faecal samples. Animal 15, 100145.
| Crossref | Google Scholar | PubMed |
Pearson S (1999) Late Holocene biological records from the middens of stick-nest rats in the central Australian arid zone. Quaternary International 59, 39-46.
| Crossref | Google Scholar |
Pearson S, Betancourt JL (2002) Understanding arid environments using fossil rodent middens. Journal of Arid Environments 50, 499-511.
| Crossref | Google Scholar |
Pearson S, Dodson JR (1993) Stick-nest rat middens as sources of paleoecological data in Australian deserts. Quaternary Research 39, 347-354.
| Crossref | Google Scholar |
Pearson SG, Baynes A, Triggs BE (2001) The record of fauna, and accumulating agents of hair and bone, found in middens of stick-nest rats (Genus Leporillus) (Rodentia: Muridae). Wildlife Research 28, 435-444.
| Crossref | Google Scholar |
Pimentel D, Zuniga R, Morrison D (2005) Update on the environmental and economic costs associated with alien-invasive species in the United States. Ecological Economics 52, 273-288.
| Crossref | Google Scholar |
Pyšek P, Richardson DM (2010) Invasive species, environmental change and management, and health. Annual Review of Environment and Resources 35, 25-55.
| Crossref | Google Scholar |
Ryan SA, Moseby KE, Paton DC (2003) Comparative foraging preferences of the greater stick-nest rat Leporillus conditor and the European rabbit Oryctolagus cuniculus: implications for regeneration of arid lands. Australian Mammalogy 25, 135-146.
| Crossref | Google Scholar |
Short J, Richards JD, O’Neill S (2018) Reintroduction of the greater stick-nest rat (Leporillus conditor) to Heirisson Prong, Shark Bay: an unsuccessful attempt to establish a mainland population. Australian Mammalogy 40, 269-280.
| Crossref | Google Scholar |
Short J, Copley P, Ruykys L, Morris K, Read J, Moseby K (2019) Review of translocations of the greater stick-nest rat (Leporillus conditor): lessons learnt to facilitate ongoing recovery. Wildlife Research 46, 455-475.
| Crossref | Google Scholar |
Soininen EM, Zinger L, Gielly L, Bellemain E, Bråthen KA, Brochmann C, Epp LS, Gussarova G, Hassel K, Henden J-A, Killengreen ST, Rämä T, Stenøien HK, Yoccoz NG, Ims RA (2013) Shedding new light on the diet of Norwegian lemmings: DNA metabarcoding of stomach content. Polar Biology 36, 1069-1076.
| Crossref | Google Scholar |
Storr GM (1961) Microscopic analysis of faeces, a technique for ascertaining the diet of herbivorous, mammals. Australian Journal of Biological Sciences 14, 157-164.
| Crossref | Google Scholar |
Todd JW, Hansen RM (1973) Plant fragments in the feces of bighorns as indicators of food habits. The Journal of Wildlife Management 37, 363-366.
| Crossref | Google Scholar |
Webeck K, Pearson S (2005) Stick-nest rat middens and a late-Holocene record of White Range, central Australia. The Holocene 15, 466-471.
| Crossref | Google Scholar |
Williams JA, West CJ (2000) Environmental weeds in Australia and New Zealand: issues and approaches to management. Austral Ecology 25, 425-444.
| Crossref | Google Scholar |
Wood Jones F (1922) The flora and fauna of Nuyt’s archipelago and the Investigator Group. No. 2. - The monodelphian mammals. Transactions of the Royal Society of South Australia 46, 181-194.
| Google Scholar |
Wootton LS, Halsey SD, Bevaart K, McGough A, Ondreicka J, Patel P (2005) When invasive species have benefits as well as costs: managing Carex kobomugi (Asiatic sand sedge) in New Jersey’s coastal dunes. Biological Invasions 7, 1017-1027.
| Crossref | Google Scholar |