High chytrid prevalence and infection intensities in tadpoles of Mixophyes fleayi
Matthijs Hollanders
A
B
C
D
Abstract
The amphibian chytrid fungus Batrachochytrium dendrobatidis (Bd) has caused catastrophic biodiversity loss globally, but species and life stages within species respond differently to the pathogen. Although tadpoles are often reported to be less vulnerable to disease, they can constitute important infection reservoirs in ecosystems.
We aimed to describe Bd infection patterns of a long-lived tadpole in a species where post-metamorphic animals appear to exhibit limited mortality as a result of chytridiomycosis. We further investigated how oral dekeratinisation can be used as an indicator of infection.
We conducted surveys of tadpoles of Mixophyes fleayi (Fleay’s barred frog) over 2 years, at two rainforest streams on the east coast of Australia, to assess patterns in Bd infection prevalence and intensity. We developed an integrated hierarchical model propagating pathogen-detection errors and incorporating how Bd infections affect oral dekeratinisation.
We found that Bd infection prevalence was strongly associated with lower temperatures and a larger body size, consistent with Bd optimal thermal range and a cumulative risk of exposure for tadpoles. The individual probability of a tadpole being infected with Bd was estimated to be 0.58 [95% HPDI: 0.432, 0.713], the odds of which were approximately eight times greater than for adults at the same sites. Tadpoles infected with Bd were 113 [29, 293] times more likely to have oral dekeratinisation than were uninfected tadpoles, where uninfected individuals were estimated to have a 0.05 [95% HPDI: 0.011, 0.11] probability of having mouthpart loss.
Our results showed that M. fleayi tadpoles are more likely to be infected with Bd than are adults, suggesting that tadpoles could contribute to Bd maintenance in streams. We further showed that sites can be rapidly assessed for Bd by visually checking for oral dekeratinisation.
Long-lived tadpoles, in general, may contribute to Bd maintenance in ecosystems. We suggest continued exploration of Bd immunocompetence across amphibian life stages to further understand the vastly different infection patterns.
Keywords: amphibian, Batrachochytrium dendrobatidis, chytridiomycosis, dekeratinisation, mouthpart loss, pathogen detection, pathogen load, tadpole.
Introduction
The global invasion of the pathogenic amphibian chytrid fungus (Batrachochytrium dendrobatidis, Bd) has strongly affected frog species around the world (Berger et al. 1998; Scheele et al. 2019). However, where some species have been decimated or even driven extinct, others appear to incur fewer costs owing to the fungus. Moreover, different life stages present heterogeneity in vulnerability to mortality; where post-metamorphic stages are frequently cited as being more vulnerable, particularly around metamorphosis itself, larval stages (hereafter interchangeably used with tadpoles) experience fewer detrimental effects (Garner et al. 2009; Ortiz-Santaliestra et al. 2013; Sauer et al. 2020).
Although Bd infections only rarely directly lead to mortality in tadpoles (Blaustein et al. 2005; Arellano et al. 2017), infections are still likely to affect them negatively by limiting feeding ability (Cashins 2009; Venesky et al. 2009; Hagman and Alford 2015; Harjoe et al. 2022), possibly reducing growth and size at metamorphosis (Garner et al. 2009), which may affect individuals post-metamorphosis (Altwegg and Reyer 2003; Garner et al. 2009; Kilpatrick et al. 2010; Sauer et al. 2020). Given the immune system reorganisation that takes place at metamorphosis (Rollins-Smith 1998), highly infected tadpoles may experience considerable costs around this stage, and larval Bd infections may further reduce fitness of these individuals when they metamorphose (Garner et al. 2009; Briggs et al. 2010; Fernández-Loras et al. 2017; Sauer et al. 2020). Because the fungus infects only keratinised body parts, which in tadpoles only occur in mouthparts, dekeratinisation of oral structures (hereafter interchangeably used with mouthpart loss) caused by Bd may be the mechanism that negatively affects larval feeding ability (Marantelli et al. 2004; Cashins 2009; Venesky et al. 2009; Harjoe et al. 2022). For example, torrent-adapted tadpoles in tropical streams were reported to have decreased body condition with increased oral dekeratinisation, with body condition improving on the regrowth of oral structures (Cashins 2009). On metamorphosis, the distribution of keratin shifts from the mouthparts to the skin, which rapidly becomes infected with Bd on development (Marantelli et al. 2004; McMahon and Rohr 2015). Dekeratinisation of the oral structures, in particular when it is observed in the jaw sheaths, has frequently been noted as an indicator of Bd infection in tadpoles (Fellers et al. 2001; Rachowicz 2002; Marantelli et al. 2004; Blaustein et al. 2005; Knapp and Morgan 2006; Drake et al. 2007; Cashins 2009). However, the extent to which dekeratinisation is a good indicator appears to be species-specific (Navarro-Lozano et al. 2018).
In addition to the costs accrued during the larval phase, tadpoles may act as a reservoir for Bd in ecosystems when they occur in permanent water bodies, particularly when tadpoles are long-lived (Rachowicz and Vredenburg 2004; Briggs et al. 2010; Catenazzi et al. 2013; Valencia-Aguilar et al. 2016; Courtois et al. 2017; Sapsford et al. 2018; das Neves-da-Silva et al. 2021). Reservoirs, i.e. hosts that permanently maintain the pathogen and transmit it to other hosts (Haydon et al. 2002), can greatly influence disease impact by increasing the exposure to susceptible individuals (Gog et al. 2002; De Castro and Bolker 2004). When terrestrial habitats seasonally warm up and dry out, becoming unfavourable for Bd growth (Piotrowski et al. 2004), the prevalence and intensity (measured as pathogen load) of Bd infections frequently decreases in adult frogs (Kriger and Hero 2007). However, aquatic environments may maintain lower temperatures and the requisite moisture requirements for Bd growth, facilitating the maintenance of Bd in ecosystems (Sapsford et al. 2018). Tadpoles may therefore remain infected with (and exposed to) Bd under conditions that are favourable to the fungus for long periods of time, and have a higher infection prevalence than do post-metamorphic individuals.
Previous research has found associations between Bd infections, tadpole body size, and temperature (Cashins 2009; Catenazzi et al. 2013; Valencia-Aguilar et al. 2016; Sapsford et al. 2018). Larger tadpoles are reportedly more likely to be infected with Bd, likely owing to the cumulative exposure older tadpoles have accrued to Bd zoospores in the water over time (Valencia-Aguilar et al. 2016; Sapsford et al. 2018). Infections with Bd tend to be more common, and infection intensities higher, with lower water temperatures, likely owing to the thermal preferences of Bd (Sapsford et al. 2018). Although both temperature and body size appear to be influential variables, ambiguity remains about the relative contributions of each on both infection prevalence and intensity in tadpoles (Sapsford et al. 2018; das Neves-da-Silva et al. 2021).
In this study, we assessed the seasonal Bd infection prevalence and infection intensity of tadpoles over 2 years in two independent streams. We focused on tadpoles of Mixophyes fleayi (Fleay’s barred frog), an endangered rainforest species for which the Bd prevalence, intensity, and impacts on mortality have been intensively assessed in both adult and recently metamorphosed cohorts (Hollanders et al. 2023a, 2023b). We built a modified version of a recently developed Bayesian hierarchical model to estimate the effect of temperature, body size, and their interaction on Bd infections. We also assessed to what extent mouthpart loss can be predicted by Bd infection and the uncertainty associated with using mouthpart loss as a proxy for Bd infection. Our results provide valuable insight into Bd dynamics of a long-lived tadpole in subtropical stream environments.
Materials and methods
Field surveys
Mixophyes fleayi (Fleay’s barred frog) is a large (male snout–urostyle lengths [SUL] ± 60 mm, female SUL ± 80 mm), terrestrial, stream-breeding frog endemic to the Gondwanan rainforests of northern New South Wales and South East Queensland, Australia. The tadpoles of M. fleayi are bottom-dwellers that graze the stream substrates, and tadpoles are likely to use their oral disc to adhere to the bottom of fast-flowing stream sections (Anstis 2002). We conducted M. fleayi tadpole surveys at 6-weekly intervals from September 2019 to January 2021 at Brindle Creek, Border Ranges National Park, and Tuntable Creek, Nightcap National Park, New South Wales, Australia. For details on sites, see Hollanders et al. (2023b). At Brindle Creek, we sampled from two adjacent pools separated by a riffle section where adult frogs were known to breed. At Tuntable Creek, we sampled from four pools separated by riffle sections. We used a dipnet (Jonah’s Aquarium The Perfect Dipnet, 1/8” mesh) to sample each pool for 1 min.
Tadpoles were held in a plastic tub and up to 40 individuals were processed (photographed and sampled for Bd) per site per occasion. For 1 year at Brindle Creek, we took a top-down photograph of the full tub of tadpoles (prior to subsampling 40 for processing), so as to measure the length of each tadpole (from the tip of the nose to the base of the tail) by using the Ruler Tool in Adobe Photoshop 2023 to gauge the distribution of size classes (Fig. 1). We handled tadpoles following Cashins (2009), photographed their mouthparts (Nikon D750 and Sigma 105 mm macro at minimum focus), and sampled for Bd by using swabs (five horizontal strokes and five vertical strokes across the mouthparts). For 620 individuals sampled over the 2 years, we photographed mouthparts to identify mouthpart loss associated with Bd and to derive a body size proxy, for which we used the distance between the two mouth corners measured in pixels using the Ruler Tool in Adobe Photoshop 2020. We chose to measure body size indirectly by using this method to reduce handling time of the animals and to get a more precise measurement than when measuring body length in-hand by using calipers. We verified the method by comparing body-length measurements by using calipers with mouthpart widths measured using Photoshop of 136 individuals and found strong agreement between the two measurements (Supplementary material Fig. S1). We identified mouthpart loss by assessing for dekeratinisation of the jaw sheaths from photographs, and scored the amount of dekeratinisation of both the bottom (posterior) and top (anterior) jaw sheath separately on a scale of 1–5, where 1 was barely visible mouthpart loss and 5 was complete dekeratinisation (i.e. no black mouthparts visible) (Fig. 2).
Various stages of oral dekeratinisation in tadpoles of Mixophyes fleayi. The oral dekeratinisation scores of top/bottom (anterior/posterior) jaw sheaths were (a) 0/0, (b) 5/3, (c) 5/3, and (d) 5/4.
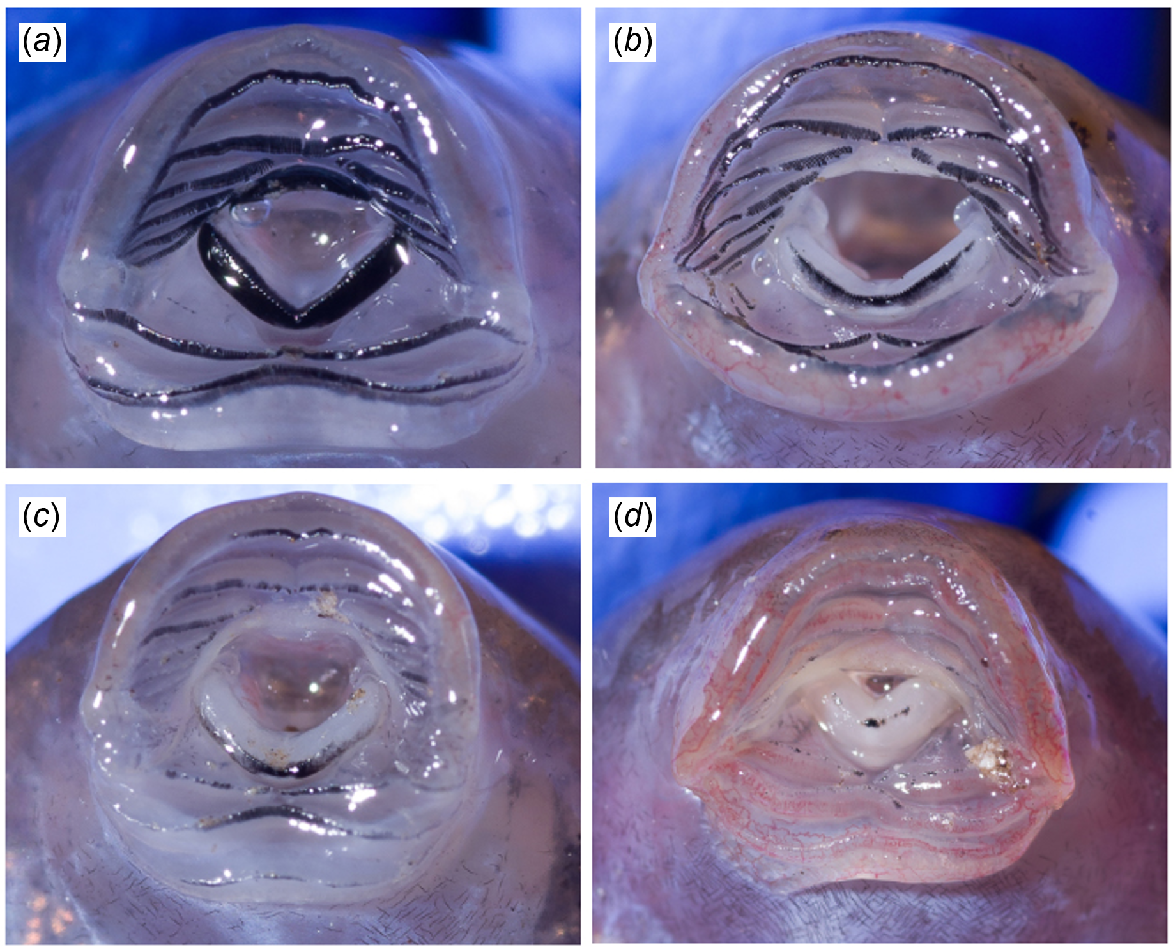
We placed dataloggers (HOBO MX2201) along the edge of the stream to record temperature every 2 h for the duration of the study. We relied on terrestrial dataloggers for our analysis, because dataloggers in the creeks were swept away by floods.
Laboratory work
We extracted and amplified Bd DNA by using standard protocols (Boyle et al. 2004; Hyatt et al. 2007; Brannelly et al. 2020). Briefly, DNA was extracted using Prepman® Ultra (Applied Biosystems) without bead-beating step (Brannelly et al. 2020). Then, sample DNA was amplified using quantitative polymerase chain reaction (qPCR) in duplicate with synthetic gBlocks ITS reference standards (Integrated DNA Technologies) to quantify Bd DNA present on swabs. We considered samples positive when at least one qPCR well returned Bd DNA. Infection intensities are reported as log10 gene copies per swab. For further details on the laboratory process, see Hollanders et al. (2023b).
Statistical analysis
To identify patterns in Bd infection status (infected/uninfected) and infection intensity (pathogen load), we fit a model to swab infection status and (log10) swab infection intensities respectively, correcting for measurement error in the qPCR and the sampling processes (DiRenzo et al. 2018, Fig. S2). The model incorporated imperfect pathogen detection in the qPCR process through both false-negatives and false-positives, and further introduced measurement error in the swab infection intensities by using an informative prior, because infection intensities measured from single samples may not accurately reflect the true infection intensities of individuals (DiRenzo et al. 2018; McElreath 2020; Hollanders and Royle 2022). Note that although infection status was corrected for imperfect detection owing to qPCR, we did not model the false-negatives associated with the swabbing protocol; however, double-swabbing tadpoles would likely not have yielded independent samples (Hollanders and Royle 2022).
The model featured a logistic regression component to latent infection status and a linear regression to latent individual infection intensities (see Appendix S2 for details). Both regression models included site, average temperature over the 6-week survey intervals, (log) tadpole size (using the proxy of mouthpart width), all pairwise interactions, the three-way interaction, and correlated random survey effects as predictors. We modelled both the presence (binary) and intensity (ordinal) of mouthpart loss, as determined by the presence and degree of dekeratinisation of the jaw sheath (summed over both top and bottom), as descendant variables of Bd infection. Each component was modelled as a function (logit-linear for status and ordered probit for intensity) of latent estimated Bd infection intensity (and infection status for presence only) and correlated random survey effects. Additionally, we included effects of body size separately for infected and uninfected tadpoles. We conducted posterior predictive checks for the four components of the model and calculated Bayesian P-values for each to assess goodness-of-fit (Gelman et al. 1996; Appendix S2).
We implemented the model with Bayesian methods by using NIMBLE 1.0.1 (de Valpine et al. 2017, 2023) through R 4.3.0 (R Core Team 2023), with weakly informative priors (except for the measurement error of the swabbing process; Table 1, Appendix S2). For predictor variable selection, we used the built-in reversible jump MCMC (RJMCMC, Green 1995) samplers in NIMBLE, which exclude parameters that do not contribute sufficiently to the likelihood (and toggles values to 0 for those iterations where parameters are excluded). Predictors were centred and scaled by two standard deviations to allow direct comparison between binary (site) and continuous (temperature and body size) effects (Gelman et al. 2008). We imputed missing body-size values (n = 244) from a normal distribution parameterised from observed values (n = 621) using MCMC. We ran four chains for 50,000 iterations after discarding 10,000 as burn-in and thinning chains by 10, yielding 20,000 posterior samples that resulted in convergence of all model parameters. We summarised posterior distributions with medians and 95% highest posterior density intervals (HPDIs), included the samples where RJMCMC toggled coefficients to 0 in these summary statistics, report the RJMCMC inclusion probabilities, and considered effects important when 95% HPDIs of the coefficients did not overlap 0. The analysis is fully reproducible on https://github.com/mhollanders/mfleayi-tadpoles.
Function | Parameter | Median | 95% HPDI | RJMCMC | Prior | |
---|---|---|---|---|---|---|
Bd infection status (Ψ) | Intercept | 0.58 | [0.432, 0.713] | Beta (3,3) | ||
Temp A | −3.271 | [−4.616, −1.943] | 1 | t3(0,1) | ||
Body size A | 3.966 | [3.129, 4.891] | 1 | t3(0,1) | ||
Site | 0 | [−1.009, 0.828] | 0.551 | t3(0,1) | ||
Temp × body size | 0 | [−1.609, 0.776] | 0.443 | t3(0,1) | ||
Temp × site | 0 | [−0.658, 1.879] | 0.33 | t3(0,1) | ||
Body size × site | 0 | [−1.142, 0.633] | 0.293 | t3(0,1) | ||
Temp × body size × site | 0 | [−1.46, 0.39] | 0.125 | t3(0,1) | ||
Survey (s.d.) | 1.257 | [0.788, 1.853] | t3+(0,1) | |||
Bd detection probability (r) | 0.619 | [0.567, 0.677] | Beta (6,4) | |||
Bd false-positive (δ21) | 0.016 | [0.003, 0.029] | Beta (1,10) | |||
Bd infection intensity (μ) | Intercept | 3.88 | [3.685, 4.069] | t3(4,1) | ||
Temp | 0 | [−0.444, 0.116] | 0.291 | t3(0,1) | ||
Body size A | 0.45 | [0.222, 0.692] | 0.996 | t3(0,1) | ||
Site | 0 | [−0.417, 0.106] | 0.326 | t3(0,1) | ||
Temp × body size | 0 | [−0.205, 0.117] | 0.104 | t3(0,1) | ||
Temp × site | 0 | [−0.092, 0.228] | 0.083 | t3(0,1) | ||
Body size × site | 0 | [−0.38, 0.017] | 0.122 | t3(0,1) | ||
Temp × body size × site | 0 | [0, 0] | 0.049 | t3(0,1) | ||
Survey (s.d.) | 0.415 | [0.281, 0.571] | t3+(0,1) | |||
Population s.d. (expσμ) | 1.564 | [1.439, 1.687] | t3+(0,1) | |||
Swab error (σswab) | 0.121 | [0, 0.242] | t3+(0.17,0.11) | |||
qPCR error (σqPCR) | 0.632 | [0.593, 0.672] | t3+(0.5,0.05) | |||
Mouthpart loss status (λ) | Intercept | 0.05 | [0.011, 0.11] | Beta (1,10) | ||
Bd infection status A | 4.727 | [3.656, 5.772] | 1 | t3(0,1) | ||
Bd infection intensity A | 2.541 | [1.31, 3.969] | 1 | t3(0,1) | ||
Body size (if Bd+) | 0 | [−0.809, 0.869] | 0.333 | t3(0,1) | ||
Body size (if Bd–) | 0 | [−0.75, 0.934] | 0.35 | t3(0,1) | ||
Survey (s.d.) | 1.826 | [1.088, 2.762] | t3+(0,1) | |||
Mouthpart loss intensity (κ) | Bd infection intensity | 0 | [−0.023, 0.715] | 0.412 | t3(0,1) | |
Body size (if Bd+) | 0 | [−0.329, 0.359] | 0.218 | t3(0,1) | ||
Body size (if Bd–) A | 0.912 | [0, 1.928] | 0.807 | t3(0,1) | ||
Survey (s.d.) | 1.218 | [0.803, 1.742] | t3+(0,1) |
Results
Sampling summary
We captured and swabbed 865 M. fleayi tadpoles (424 at Brindle and 441 at Tuntable) over 25 surveys. Of those, 54% (471) returned Bd DNA in at least one qPCR run, with the median Bd infection intensity being 4.02 log10 gene copies per swab (averaged over duplicate qPCR replicates). Of the 620 tadpoles sampled for mouthpart loss, 39% (243) showed dekeratinisation; of those, 95% (230) returned Bd in at least one qPCR replicate. Because tadpoles were not marked, some individuals may have been sampled more than once over the study period. Tadpoles were present year-round, with pulses of reproduction occurring in Austral spring and autumn (Fig. 1).
Bd infection prevalence
Average Bd prevalence was estimated as 0.58 [95% HPDI: 0.432, 0.713], with no significant site effect (log odds change 0 [95% HPDI: −1.009, 0.828], RJMCMC inclusion 0.551) (Table 1). Individual probability of being infected with Bd was strongly negatively associated with temperature (log odds change −3.271 [95% HPDI: −4.616, −1.943], RJMCMC inclusion 1) and strongly positively with body size (log odds change 3.966 [95% HPDI: 3.129, 4.891], RJMCMC inclusion 1) (Table 1, Fig. 4). We found weak evidence for interaction effects, with low RJMCMC inclusion probabilities and effect estimates overlapping 0 (Table 1). The Bayesian P-value of the prevalence component of the model was 0.293, indicating reasonable fit (Fig. S2, S3a). Model fit improved significantly with the addition of the random survey effect (comparison not shown), which was large (standard deviation [s.d.] of the effect 1.257 [95% HPDI: 0.788, 1.853]), suggesting a considerable amount of unexplained variation among surveys (Fig. 3a). The survey effects were somewhat positively correlated with those of infection intensity (correlation of 0.235 [95% HPDI: −0.307, 0.727]), suggesting that when infections were common the associated infection intensities were also high. The probability of detecting Bd by using qPCR was high (probability of detecting one log10 gene copy in a qPCR replicate estimated as 0.619 [95% HPDI: 0.567, 0.677], yielding a 0.999 [95% HPDI: 0.999, 1] probability of detecting the average load after two qPCR replicates), reflected in that the model estimated that 460 individuals were infected compared with the 471 infections that we observed. The false-positive probability of detecting Bd in the qPCR protocol was estimated to be low (0.016 [95% HPDI: 0.003, 0.029]), but note that Bd detection errors in the swabbing process were not modelled because only single swabs were collected.
Survey-specific estimates (medians and 95% HPDIs) of (a) Bd infection prevalence, (b) average Bd infection intensity (log10 gene copies per swab), (c) oral dekeratinisation prevalence, and (d) average oral dekeratinisation score of tadpoles with dekeratinisation. Estimates were averaged over individual-level predictions for each survey.
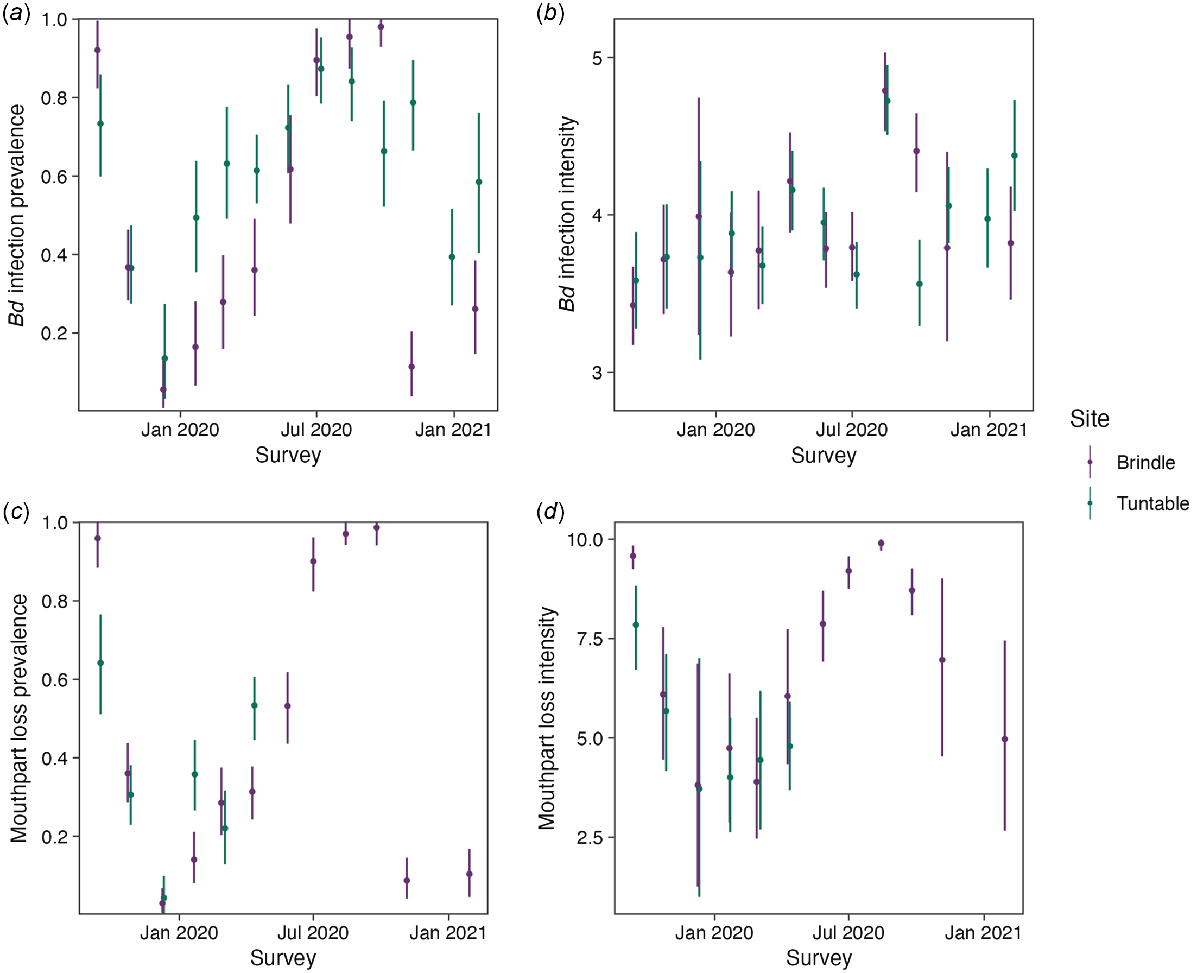
Bd infection intensity
Average Bd infection intensity was estimated as 3.88 [95% HPDI: 3.68, 4.07] log10 gene copies, with no significant differences between sites (RJMCMC inclusion 0.33), with a population s.d. of 1.56 [95% HPDI: 1.44, 1.69] (Table 1). There was a weak positive association between individual Bd infection intensity and body size (log change 0.45 [0.22, 0.69], RJMCMC inclusion 1) (Fig. S2), but no association with temperature (log change 0 [95% HPDI: −0.44, 0.12], RJMCMC inclusion 0.29). We found no evidence for interaction effects, and unexplained variation in the form of survey effects was small (s.d. of survey effect 0.42 [95% HPDI: 0.28, 0.57]) (Table 1, Fig. 3b). There was some measurement error associated with the qPCR process (s.d. of effect 0.63 [95% HPDI: 0.59, 0.67]), and together with the additional swabbing measurement error (0.12 [95% HPDI: 0, 0.24]) incorporated into the model with an informative prior, there were considerable differences between the observed swab infection intensities (computed as the sample mean of positive qPCR replicates) and the estimated latent individual infection intensities (Fig. S1, S2). The Bayesian P-value for this model was 0.76, suggesting decent fit (Fig. S3b).
Mouthpart loss
The presence of mouthpart loss, specifically determined by dekeratinisation of the jaw sheath, was strongly influenced by Bd infection. Uninfected individuals had a probability of 0.05 [95% HPDI: 0.01, 0.11] of having mouthpart loss, whereas infected tadpoles (carrying the average Bd infection intensity) had a 0.85 [95% HPDI: 0.7, 0.97] probability (log odds change 4.73 [95% HPDI: 3.66, 5.77], RJMCMC inclusion 1) (Table 1, Fig. 5a). This implies that individuals infected with Bd had 113 [95% HPDI: 29, 293] times greater odds to have dekeratinisation of the jaw sheath than did uninfected individuals, and that there was a 0.15 [95% HPDI: 0.03, 0.3] probability of tadpoles infected with Bd to not have dekeratinisation. For those individuals that were infected with Bd, higher Bd infection intensities were associated with a considerably higher probability of mouthpart loss (log odds change 2.54 [95% HPDI: 1.31, 3.97], RJMCMC inclusion 1) (Fig. 5b). Body size was not found to have a direct effect on the probability of mouthpart loss. There was a large amount of unexplained survey variation (s.d. of survey effect 1.83 [1.09, 2.76]), which was strongly correlated with those of mouthpart loss intensity (0.7 [95% HPDI: 0.34, 0.95]), showing that when many tadpoles had some degree of mouthpart loss that the intensity of mouthpart loss was high (Fig. 3c, d). The Bayesian P-value of this component was 0.62, suggesting good fit (Fig. S3c).
Finally, the intensity of mouthpart loss, as determined by the amount of dekeratinisation in the jaw sheaths, was associated with Bd infection status but infection status could not be included as a predictor because of the lack of uninfected individuals with mouthpart loss (Fig. 5c). Infection intensity was weakly positively associated with the degree of oral dekeratinisation (standardised change 0 [−0.02, 0.71], RJMCMC inclusion 0.41). For uninfected tadpoles, body size was positively associated with the degree of oral dekeratinisation (standardised change 0.91 [0, 1.93], RJMCMC inclusion 0.81). There was some amount of unexplained survey variation (s.d. of survey effect 1.22 [0.8, 1.74]). Goodness-of-fit of this component was good (Bayesian P-value of 0.31) (Fig. S3d).
Discussion
We investigated the occurrence of Bd and associated infection intensities in endangered Mixophyes fleayi tadpoles over 2 years, and found that both average infection prevalence (0.58 [95% HPDI: 0.43, 0.71]) and infection intensity (3.88 [3.68, 4.07] log10 gene copies per swab) were high. Compared with adults from the same sites reported in previous studies, which had an average reported prevalence of 0.15 [95% HPDI: 0.07, 0.27] and intensity of 3.036 [95% HPDI: 2.836, 3.234] (Hollanders et al. 2023b), the odds of tadpoles being infected with Bd were approximately eight times greater and average log10 infection intensities were 1.28 times greater. We found evidence of seasonality for Bd infections in tadpoles, where lower temperatures were associated with higher Bd prevalence (but not Bd infection intensity). Additionally, we corroborated evidence for risk of infection increasing cumulatively with age, because we found a strong association between body size and infection prevalence. It is noteworthy that recently metamorphosed individuals of M. fleayi at Brindle Creek have a lower average infection prevalence (estimated at 0.42 [95% HPDI: 0.13, 0.7], after correcting for imperfect detection in the swabbing process) and lower infection intensities (estimated at 2.8 [2.45, 3.08] log10 gene copies per swab) than do the tadpoles (Hollanders et al. 2023a). This discrepancy of infection patterns between larval and post-metamorphic individuals sharing a spatio-temporal space warrants further investigation and suggests considerable differences in immunocompetence, pathogen resistance, exposure history, or other mechanisms of persistence (Brannelly et al. 2021).
Individual Bd infections of tadpoles were associated with lower temperatures and larger body sizes, consistent with results from previous studies (Fig. 4) (Cashins 2009; Catenazzi et al. 2013; Valencia-Aguilar et al. 2016; Sapsford et al. 2018). Low temperatures also correlated with increased infection prevalence with adult amphibians at the same site, consistent with the regional pattern previously reported (Kriger and Hero 2007; Hollanders et al. 2023b). The average 6-weekly temperatures at the sites ranged from 8.6 to 19.8°C, which is within the optimal range for Bd growth; nevertheless, growth is reportedly faster at ~20°C than it is at ~10°C, so Bd physiology may explain the observed pattern of infection prevalence at our sites (Piotrowski et al. 2004; Stevenson et al. 2013). However, thermal optima vary among Bd strains and the performance of the local strain is unknown (Voyles et al. 2017). Alternatively, immune function of tadpoles may be improved with higher temperatures (Cohen et al. 2017). Although tadpole immune response to Bd is poorly understood, previous studies have demonstrated that low-protein diets increase the probability of gaining Bd infections (Venesky et al. 2012) and tadpoles kept at lower temperatures have higher infection intensities (Altman and Raffel 2019), suggesting that resistance to Bd is influenced by external factors. Further, some studies have reported regrowth of oral structures after initial dekeratinisation as a result of Bd infection, suggesting possible tolerance mechanisms after infection (Cashins 2009; Hagman and Alford 2015). However, if tadpoles mounted successful immune responses to Bd infection, lower probability of being infected would be expected with a larger body size, and/or lower infection intensities would be associated with these remaining infections. Contrary to this, we found evidence for infection status being strongly predicted by body size (a proxy for age), in line with previous studies (Valencia-Aguilar et al. 2016; Sapsford et al. 2018). This result is consistent with tadpoles experiencing a cumulative exposure risk and limited immune response to suppress Bd infections in the field.
Prediction curves (medians and 95% HPDIs) with the coefficients of (a) temperature and (b) body size on the probability of Mixophyes fleayi tadpoles being infected with Bd from logistic regression, with the other (scaled) predictors held at their respective means. Points are observed individuals with corresponding (a) temperature and (b) body size at observation, categorised by Bd infection status (infected, top; uninfected, bottom). Body sizes were transformed from mouthpart widths by using an equation (Fig. S2).
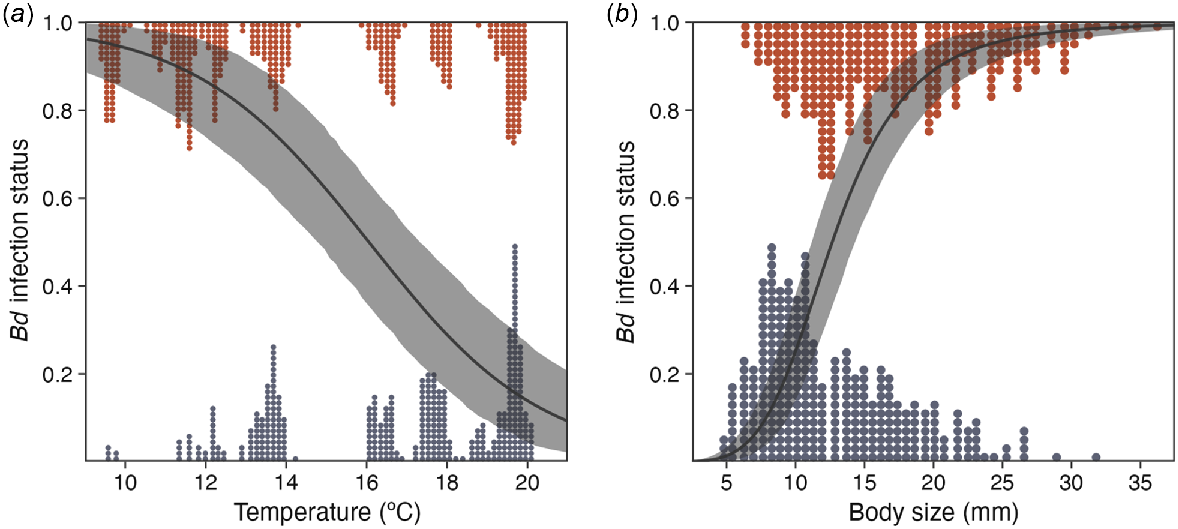
Unlike infection status, we did not find strong predictors influencing infection intensity. RJMCMC excluded all predictors except body size, which was found to have a weakly positive effect on infection intensity (Fig. S2); however, this may simply indicate that larger tadpoles have larger oral structures to swab. The lack of evidence for a temperature effect was noteworthy, given the strength of the effect of temperature on infection status. This may indicate that Bd growth is largely independent of the range of temperatures that occur in these streams once Bd becomes established on a tadpole. Tadpoles did have considerably higher average infection intensities than did adults (median of 3.88 vs 3.0 log10 gene copies), which may in fact underestimate the difference, given that tadpoles have only their oral structures swabbed (10 strokes) compared with the entire ventral surface of post-metamorphic animals (45 strokes, Hollanders et al. 2023b). That tadpoles in populations where adults display limited susceptibility to Bd after initial epidemics have higher infection intensities than do adult frogs has also been found in Rana sierrae/muscosa in the United States (Briggs et al. 2010). One explanation is that tadpoles exist in an environment that is more conducive to Bd growth because of the moisture and lower temperatures (Piotrowski et al. 2004). Alternatively, an increased immune response may be present in adult individuals compared with tadpoles. With relatively fewer deleterious consequences of Bd infections for tadpoles, lower selective pressure would be expected than in post-metamorphic animals experiencing mortality owing to chytridiomycosis. Our results indicated that subtropical stream environments are suitable for high Bd prevalence in tadpoles throughout the year.
Tadpoles of Mixophyes fleayi may be an important Bd reservoir because of the high infection prevalence and intensity and year-round presence in the streams (Rachowicz and Vredenburg 2004; Cashins 2009; Briggs et al. 2010; Catenazzi et al. 2013; Courtois et al. 2017; Sapsford et al. 2018). However, without an explicit causal or mechanistic model that accounts for transmission (i.e. shedding rates, exposure rates of vulnerable hosts, etc.), we cannot make claims about their status as a reservoir (Wilber et al. 2022). On the basis of the body-size distributions from December 2019 to January 2021, pulses of reproduction occurred in the summer months (Fig. 1), consistent with previous studies (Stratford et al. 2010). Importantly, large tadpoles were present throughout the study period; given that larger tadpoles were more often infected, these individuals are likely to contribute to the maintenance of Bd in the stream environments. Experimental studies have shown that tadpoles readily infect other tadpoles when they share an aquatic environment (Rachowicz and Briggs 2007; Hagman and Alford 2015; Courtois et al. 2017). However, the extent to which adults become infected when entering these aquatic environments is less well understood. In laboratory studies, all post-metamorphic animals housed in an enclosure with infected tadpoles became infected within 3 weeks (Rachowicz and Vredenburg 2004). However, the transmission of tadpoles to terrestrial adults is currently poorly understood. For stream-breeding frogs where breeding congregations occur in and around streams, understanding the risk of exposure from the stream environment is important for understanding infection dynamics. This remains an open area of research across many Bd–amphibian systems.
To our knowledge, our study is the first to assess the effects of both Bd infection status and intensity on the probability of having oral dekeratinisation. Our results confirmed that oral dekeratinisation is a good indicator of Bd infection in Mixophyes fleayi, with a 0.15 [95% HPDI: 0.03, 0.3] probability of an infected tadpole not displaying oral dekeratinisation (Symonds et al. 2007; Navarro-Lozano et al. 2018). We found that infected tadpoles had, on average, 113 times higher odds to have mouthpart loss than did uninfected tadpoles (Fig. 5a). Additionally, we found that among those tadpoles infected with Bd, individuals with a higher infection intensity were significantly more likely to have mouthpart loss (Fig. 5b). Only 13 tadpoles that had oral dekeratinisation returned negative swabs, but some of these were likely to be false-negatives. Our study did not correct for Bd detection errors in the swabbing process, which is predicted to be roughly 0.67 for the average tadpole infection intensity estimated in this study (Hollanders et al. 2023a). Although other causes of oral dekeratinisation exist (Knapp and Morgan 2006), given the prevalence of Bd at our sites and the clear association with Bd, these individuals are likely to have lost their mouthparts as a result of infection. The positive effect of body size on the degree of oral dekeratinisation for uninfected tadpoles may also suggest that prior infections caused the dekeratinisation, and that tadpoles had successfully cleared infection or that our detection protocol failed to detect Bd in these tadpoles. Importantly, the probability of finding two tadpoles with oral dekeratinisation that are not infected with Bd is just 0.0025; consequently, the presence of mouthpart loss in a small sample of tadpoles should allow investigators to conclude the presence of Bd. Unfortunately, the relationship between oral dekeratinisation and Bd infection appears highly species-specific; although the relationship is present for many species, some species show mouthpart loss in the absence of Bd (Navarro-Lozano et al. 2018).
(a) Posterior distributions (with medians and 95% HPDIs) of the probability of having mouthpart loss, as determined by the presence of dekeratinisation of the jaw sheath, for Mixophyes fleayi tadpoles uninfected (left) and infected (right) with Bd. Jittered points are observed individuals, categorised by presence of mouthpart loss (present, top; absent, bottom) and Bd infection status. (b) Prediction curve (median and 95% HPDI) with the coefficient of Bd infection intensity on the probability of having mouthpart loss from logistic regression. Points are observed individuals with corresponding Bd infection intensities estimated by the model (summarised as the median of the posterior distributions) after accounting for measurement error in the sampling (swabbing) and diagnostic (qPCR) processes. (c) Posterior distribution (with median and 95% HPDI) of the average mouthpart loss intensity score for infected (right) tadpoles (there were not enough data to estimate the score of uninfected tadpoles). Points are observed mouthpart loss intensity scores.
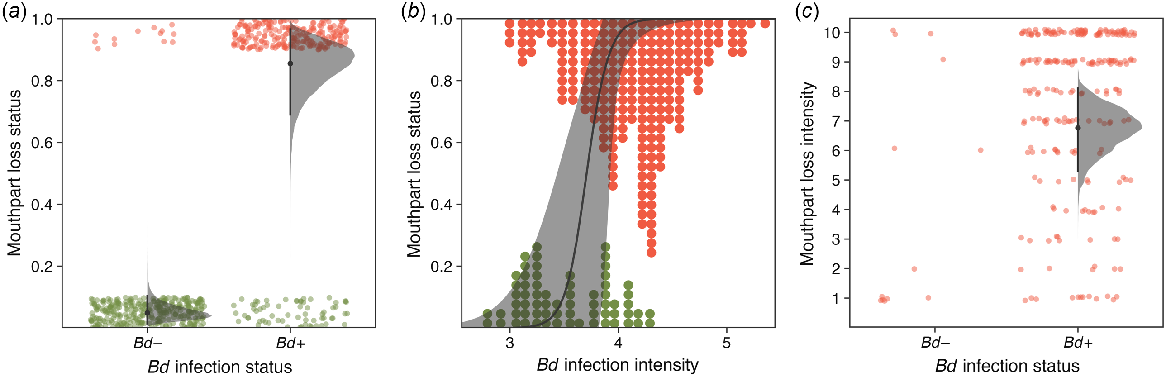
In conclusion, we have shown that Bd infection prevalence and infection intensity in tadpoles are considerably higher than in adults and juveniles at the same sites. Our focal species Mixophyes fleayi declined significantly during the epidemic but has since recovered, having stable populations despite individual mortality occurring with high Bd intensities (Hollanders et al. 2023b). Nevertheless, the year-round presence of tadpoles that are more often infected and infected with higher pathogen loads in the stream environments may constitute a Bd reservoir, both in terms of indirect transmission through the water and directly into the adult population through metamorphosis. However, to establish the role of tadpoles as pathogen reservoirs, future research will need to quantify transmission to more vulnerable hosts. The results of this work suggest fruitful future research into mechanisms of immunocompetence across amphibian life stages.
Data availability
All data, analysis scripts, and posterior samples are available at https://github.com/mhollanders/mfleayi-tadpoles.
Declaration of funding
This project was funded by the New South Wales Government’s Saving our Species Program; specific thanks go to Jill Smith and David Hunter. L. G. was supported by Australian Research Council (ARC) grants DP180101415 and DE200100490. H. M. and D. N. were supported by ARC grant DP180101415.
Acknowledgements
We extend special thanks to New South Wales National Parks and Wildlife Service for allowing us to conduct field work. We thank volunteers Darren McHugh, Liam Bolitho, Madelyn Mangan, and Josephine Humphries for assistance in the field.
References
Altman KA, Raffel TR (2019) Thermal acclimation has little effect on tadpole resistance to Batrachochytrium dendrobatidis. Diseases of Aquatic Organisms 133, 207-216.
| Crossref | Google Scholar | PubMed |
Altwegg R, Reyer H-U (2003) Patterns of natural selection on size at metamorphosis in water frogs. Evolution 57, 872-882.
| Crossref | Google Scholar | PubMed |
Arellano ML, Natale GS, Grilli PG, Barrasso DA, Steciow MM, Lavilla EO (2017) Host–pathogen relationships between the chytrid fungus Batrachochytrium dendrobatidis and tadpoles of five South American anuran species. Herpetological Journal 27, 33-39.
| Google Scholar |
Berger L, Speare R, Daszak P, Green DE, Cunningham AA, Goggin CL, Slocombe R, Ragan MA, Hyatt AD, McDonald KR, Hines HB, Lips KR, Marantelli G, Parkes H (1998) Chytridiomycosis causes amphibian mortality associated with population declines in the rain forests of Australia and Central America. Proceedings of the National Academy of Sciences 95, 9031-9036.
| Crossref | Google Scholar |
Blaustein AR, Romansic JM, Scheessele EA, Han BA, Pessier AP, Longcore JE (2005) Interspecific variation in susceptibility of frog tadpoles to the pathogenic fungus Batrachochytrium dendrobatidis. Conservation Biology 19, 1460-1468.
| Crossref | Google Scholar |
Boyle DG, Boyle DB, Olsen V, Morgan JAT, Hyatt AD (2004) Rapid quantitative detection of chytridiomycosis (Batrachochytrium dendrobatidis) in amphibian samples using real-time Taqman PCR assay. Diseases of Aquatic Organisms 60, 141-148.
| Crossref | Google Scholar | PubMed |
Brannelly LA, Wetzel DP, West M, Richards-Zawacki CL (2020) Optimized Batrachochytrium dendrobatidis DNA extraction of swab samples results in imperfect detection particularly when infection intensities are low. Diseases of Aquatic Organisms 139, 233-243.
| Crossref | Google Scholar | PubMed |
Brannelly LA, McCallum HI, Grogan LF, Briggs CJ, Puig Ribas M, Hollanders M, Sasso T, Familiar López M, Newell DA, Kilpatrick AM (2021) Mechanisms underlying host persistence following amphibian disease emergence determine appropriate management strategies. Ecology Letters 24, 130-148.
| Crossref | Google Scholar | PubMed |
Briggs CJ, Knapp RA, Vredenburg VT (2010) Enzootic and epizootic dynamics of the chytrid fungal pathogen of amphibians. Proceedings of the National Academy of Sciences 107, 9695-9700.
| Crossref | Google Scholar |
Catenazzi A, von May R, Vredenburg VT (2013) High prevalence of infection in tadpoles increases vulnerability to fungal pathogen in high-Andean amphibians. Biological Conservation 159, 413-421.
| Crossref | Google Scholar |
Cohen JM, Venesky MD, Sauer EL, Civitello DJ, McMahon TA, Roznik EA, Rohr JR (2017) The thermal mismatch hypothesis explains host susceptibility to an emerging infectious disease. Ecology Letters 20, 184-193.
| Crossref | Google Scholar | PubMed |
Courtois EA, Loyau A, Bourgoin M, Schmeller DS (2017) Initiation of Batrachochytrium dendrobatidis infection in the absence of physical contact with infected hosts: a field study in a high altitude lake. Oikos 126, 843-851.
| Crossref | Google Scholar |
das Neves-da-Silva D, Borges-Júnior VNT, Branco CWC, de Carvalho-e-Silva AMPT (2021) Effects of intrinsic and extrinsic factors on the prevalence of the fungus Batrachochytrium dendrobatidis (Chytridiomycota) in stream tadpoles in the Atlantic Forest domain. Aquatic Ecology 55, 891-902.
| Crossref | Google Scholar |
De Castro F, Bolker B (2004) Mechanisms of disease-induced extinction. Ecology Letters 8, 117-126.
| Crossref | Google Scholar |
de Valpine P, Turek D, Paciorek CJ, Anderson-Bergman C, Lang DT, Bodik R (2017) Programming with models: writing statistical algorithms for general model structures with NIMBLE. Journal of Computational and Graphical Statistics 26, 403-413.
| Crossref | Google Scholar |
de Valpine P, Paciorek C, Turek D, Michaud N, Anderson-Bergman C, Obermeyer F, Wehrhahn Cortes C, Rodrìguez A, Temple Lang D, Paganin S (2023) NIMBLE: MCMC, particle filtering, and programmable hierarchical modeling. Manual. Available at https://doi.org/10.5281/zenodo.1211190
DiRenzo GV, Campbell Grant EH, Longo AV, Che-Castaldo C, Zamudio KR, Lips KR (2018) Imperfect pathogen detection from non-invasive skin swabs biases disease inference. Methods in Ecology and Evolution 9, 380-389.
| Crossref | Google Scholar |
Drake DL, Altig R, Grace JB, Walls SC (2007) Occurrence of oral deformities in larval anurans. Copeia 2007, 449-458.
| Crossref | Google Scholar |
Fellers GM, Green DE, Longcore JE (2001) Oral chytridiomycosis in the mountain yellow-legged frog (Rana muscosa). Copeia 2001, 945-953.
| Crossref | Google Scholar |
Fernández-Loras A, Fernández-Beaskoetxea S, Arriero E, Fisher MC, Bosch J (2017) Early exposure to Batrachochytrium dendrobatidis causes profound immunosuppression in amphibians. European Journal of Wildlife Research 63, 99.
| Crossref | Google Scholar |
Garner TWJ, Walker S, Bosch J, Leech S, Marcus Rowcliffe J, Cunningham AA, Fisher MC (2009) Life history tradeoffs influence mortality associated with the amphibian pathogen Batrachochytrium dendrobatidis. Oikos 118, 783-791.
| Crossref | Google Scholar |
Gelman A, Meng X-L, Stern H (1996) Posterior predictive assessment of model fitness via realized discrepancies. Statistica Sinica 6, 733-807.
| Google Scholar |
Gelman A, Jakulin A, Pittau MG, Su Y-S (2008) A weakly informative default prior distribution for logistic and other regression models. The Annals of Applied Statistics 2, 1360-1383.
| Crossref | Google Scholar |
Gog J, Woodroffe R, Swinton J (2002) Disease in endangered metapopulations: the importance of alternative hosts. Proceedings of the Royal Society of London. Series B: Biological Sciences 269, 671-676.
| Crossref | Google Scholar |
Green PJ (1995) Reversible Jump Markov Chain Monte Carlo computation and Bayesian model determination. Biometrika 82, 711-732.
| Crossref | Google Scholar |
Hagman M, Alford RA (2015) Patterns of Batrachochytrium dendrobatidis transmission between tadpoles in a high-elevation rainforest stream in tropical Australia. Diseases of Aquatic Organisms 115, 213-221.
| Crossref | Google Scholar | PubMed |
Harjoe CC, Buck JC, Rohr JR, Roberts CE, Olson DH, Blaustein AR (2022) Pathogenic fungus causes density- and trait-mediated trophic cascades in an aquatic community. Ecosphere 13,.
| Crossref | Google Scholar |
Haydon DT, Cleaveland S, Taylor LH, Laurenson MK (2002) Identifying reservoirs of infection: a conceptual and practical challenge. Emerging Infectious Diseases 8, 1468-1473.
| Crossref | Google Scholar | PubMed |
Hollanders M, Royle JA (2022) Know what you don’t know: Embracing state uncertainty in disease-structured multievent models. Methods in Ecology and Evolution 13, 2827-2837.
| Crossref | Google Scholar |
Hollanders M, Grogan LF, McCallum HI, Brannelly LA, Newell DA (2023a) Limited impact of chytridiomycosis on juvenile frogs in a recovered species. Oecologia 202, 445-454.
| Crossref | Google Scholar |
Hollanders M, Grogan LF, Nock CJ, McCallum HI, Newell DA (2023b) Recovered frog populations coexist with endemic Batrachochytrium dendrobatidis despite load-dependent mortality. Ecological Applications 33, e2724.
| Crossref | Google Scholar | PubMed |
Hyatt AD, Boyle DG, Olsen V, Boyle DB, Berger L, Obendorf D, Dalton A, Kriger K, Hero M, Hines H, Phillott R, Campbell R, Marantelli G, Gleason F, Colling A (2007) Diagnostic assays and sampling protocols for the detection of Batrachochytrium dendrobatidis. Diseases of Aquatic Organisms 73, 175-192.
| Crossref | Google Scholar | PubMed |
Kilpatrick AM, Briggs CJ, Daszak P (2010) The ecology and impact of chytridiomycosis: an emerging disease of amphibians. Trends in Ecology & Evolution 25, 109-118.
| Crossref | Google Scholar | PubMed |
Knapp RA, Morgan JAT (2006) Tadpole mouthpart depigmentation as an accurate indicator of chytridiomycosis, an emerging disease of amphibians. Copeia 2006, 188-197.
| Crossref | Google Scholar |
Kriger KM, Hero J-M (2007) Large-scale seasonal variation in the prevalence and severity of chytridiomycosis. Journal of Zoology 271, 352-359.
| Crossref | Google Scholar |
Marantelli G, Berger L, Speare R, Keegan L (2004) Distribution of the amphibian chytrid Batrachochytrium dendrobatidis and keratin during tadpole development. Pacific Conservation Biology 10, 173-179.
| Crossref | Google Scholar |
McElreath R (2020) Chapter 15. Missing data and other opportunities. In ‘Statistical rethinking: a Bayesian course with examples in R and Stan. CRC texts in statistical science’. (Ed. R McElreath) pp. 489–524. (Taylor and Francis, CRC Press: Boca Raton, FL, USA) https://doi.org/10.1201/9780429029608
McMahon TA, Rohr JR (2015) Transition of chytrid fungus infection from mouthparts to hind limbs during amphibian metamorphosis. EcoHealth 12, 188-193.
| Crossref | Google Scholar | PubMed |
Navarro-Lozano A, Sánchez-Domene D, Rossa-Feres DC, Bosch J, Sawaya RJ (2018) Are oral deformities in tadpoles accurate indicators of anuran chytridiomycosis? PLoS ONE 13, e0190955.
| Crossref | Google Scholar | PubMed |
Ortiz-Santaliestra ME, Rittenhouse TAG, Cary TL, Karasov WH (2013) Interspecific and postmetamorphic variation in susceptibility of three North American anurans to Batrachochytrium dendrobatidis. Journal of Herpetology 47, 286-292.
| Crossref | Google Scholar |
Piotrowski JS, Annis SL, Longcore JE (2004) Physiology of Batrachochytrium dendrobatidis, a chytrid pathogen of amphibians. Mycologia 96, 9-15.
| Crossref | Google Scholar | PubMed |
Rachowicz LJ (2002) Mouthpart pigmentation in Rana muscosa tadpoles: seasonal changes without chytridiomycosis. Herpetological Review 33, 263-265.
| Google Scholar |
Rachowicz LJ, Briggs CJ (2007) Quantifying the disease transmission function: effects of density on Batrachochytrium dendrobatidis transmission in the mountain yellow-legged frog Rana muscosa. Journal of Animal Ecology 76, 711-721.
| Crossref | Google Scholar | PubMed |
Rachowicz LJ, Vredenburg VT (2004) Transmission of Batrachochytrium dendrobatidis within and between amphibian life stages. Diseases of Aquatic Organisms 61, 75-83.
| Crossref | Google Scholar | PubMed |
R Core Team (2023) ‘R: a language and environment for statistical computing.’ Manual. (R Foundation for Statistical Computing: Vienna, Austria) Available at https://www.R-project.org/
Rollins-Smith LA (1998) Metamorphosis and the amphibian immune system. Immunological Reviews 166, 221-230.
| Crossref | Google Scholar | PubMed |
Sapsford SJ, Alford RA, Schwarzkopf L (2018) Disentangling causes of seasonal infection prevalence patterns: tropical tadpoles and chytridiomycosis as a model system. Diseases of Aquatic Organisms 130, 83-93.
| Crossref | Google Scholar | PubMed |
Sauer EL, Cohen JM, Lajeunesse MJ, McMahon TA, Civitello DJ, Knutie SA, Nguyen K, Roznik EA, Sears BF, Bessler S, Delius BK, Halstead N, Ortega N, Venesky MD, Young S, Rohr JR (2020) A meta-analysis reveals temperature, dose, life stage, and taxonomy influence host susceptibility to a fungal parasite. Ecology 101, e02979.
| Crossref | Google Scholar |
Scheele BC, Pasmans F, Skerratt LF, Berger L, Martel A, Beukema W, Acevedo AA, Burrowes PA, Carvalho T, Catenazzi A, De la Riva I, Fisher MC, Flechas SV, Foster CN, Frías-Álvarez P, Garner TWJ, Gratwicke B, Guayasamin JM, Hirschfeld M, Kolby JE, Kosch TA, La Marca E, Lindenmayer DB, Lips KR, Longo AV, Maneyro R, McDonald CA, Mendelson J, III, Palacios-Rodriguez P, Parra-Olea G, Richards-Zawacki CL, Rödel M-O, Rovito SM, Soto-Azat C, Toledo LF, Voyles J, Weldon C, Whitfield SM, Wilkinson M, Zamudio KR, Canessa S (2019) Amphibian fungal panzootic causes catastrophic and ongoing loss of biodiversity. Science 363, 1459-1463.
| Crossref | Google Scholar | PubMed |
Stevenson LA, Alford RA, Bell SC, Roznik EA, Berger L, Pike DA (2013) Variation in thermal performance of a widespread pathogen, the amphibian chytrid fungus Batrachochytrium dendrobatidis. PLoS ONE 8, e73830.
| Crossref | Google Scholar | PubMed |
Stratford D, Grigg G, McCallum H, Hines H (2010) Breeding ecology and phenology of two stream breeding myobatrachid frogs (Mixophyes fleayi and M. fasciolatus) in south-east Queensland. Australian Zoologist 35, 189-197.
| Crossref | Google Scholar |
Symonds EP, Hines HB, Bird PS, Morton JM, Mills PC (2007) Surveillance for Batrachochytrium dendrobatidis using Mixophyes (Anura: Myobatrachidae) larvae. Journal of Wildlife Diseases 43, 48-60.
| Crossref | Google Scholar | PubMed |
Valencia-Aguilar A, Toledo LF, Vital MVC, Mott T (2016) Seasonality, environmental factors, and host behavior linked to disease risk in stream-dwelling tadpoles. Herpetologica 72, 98-106.
| Crossref | Google Scholar |
Venesky MD, Parris MJ, Storfer A (2009) Impacts of Batrachochytrium dendrobatidis infection on tadpole foraging performance. EcoHealth 6, 565-575.
| Crossref | Google Scholar | PubMed |
Venesky MD, Wilcoxen TE, Rensel MA, Rollins-Smith L, Kerby JL, Parris MJ (2012) Dietary protein restriction impairs growth, immunity, and disease resistance in southern leopard frog tadpoles. Oecologia 169, 23-31.
| Crossref | Google Scholar | PubMed |
Voyles J, Johnson LR, Rohr J, Kelly R, Barron C, Miller D, Minster J, Rosenblum EB (2017) Diversity in growth patterns among strains of the lethal fungal pathogen Batrachochytrium dendrobatidis across extended thermal optima. Oecologia 184, 363-373.
| Crossref | Google Scholar | PubMed |
Wilber MQ, DeMarchi J, Fefferman NH, Silk MJ (2022) High prevalence does not necessarily equal maintenance species: avoiding biased claims of disease reservoirs when using surveillance data. Journal of Animal Ecology 91, 1740-1754.
| Crossref | Google Scholar |