The Duff Moisture Code and the limits of sustainable combustion: examining the evidence for a widely used threshold
Mike Wotton A B * and Melanie Wheatley CA
B
C
Abstract
Canadian fire managers rely on the value of the Duff Moisture Code (DMC) for estimating lightning ignition and sustained smouldering in ground fuels. A simple rule used widely operationally suggests that lightning does not ignite fires and smouldering is not sustainable until the DMC >20.
We examine the strength of evidence supporting this simple rule.
We used daily lightning, fire and weather data from 2000 to 2019 to estimate the probability of lightning fire ignition across a number of regions in Canada. We also examined datasets of forest floor consumption from experimental burns carried out in pine forests in Canada.
Neither the 20 years of lightning fire ignition data nor the observed forest floor consumption data reveal consistent signals of an ignition threshold at or around DMC = 20.
Increasing DMC is associated with increasing probability of ignition from lightning and the extent of forest floor consumption, but there is little to no evidence to support the existence of a meaningful threshold across Canada when DMC is ~20. Users of this simple rule, be it for lightning ignition or fire perimeter extinction, should be aware that the data do not support a meaningful threshold around this value.
Keywords: fire extinguishment, fire ignition, fire management, fire occurrence prediction, fuel consumption, generalised additive modelling, lightning, lightning fire, smouldering combustion.
Introduction
Lightning is responsible for approximately 50% of fires in Canada, accounting for over 90% of the total area burned (Hanes et al. 2019). This underscores the significance of understanding the sustainability of ignitions from cloud-to-ground lightning discharges, particularly in areas under intensive fire protection. In the boreal forest, lightning-caused ignitions often ignite in the organic layer near the base of trees and can sometimes sustain themselves underground through smouldering combustion for days or weeks during periods not conducive to flaming spread at the surface. Such ‘holdover’ fires in the organic layer can then serve as the ignition source for flaming combustion once litter moisture and wind conditions become favourable at the surface. Understanding the persistence of active smouldering, or its opposite, the self-extinguishment of active smouldering, is therefore also important from a fire management perspective.
In Canada, the Duff Moisture Code (DMC) output of the Canadian Forest Fire Weather Index (FWI) System (Van Wagner 1987) was developed to track moisture in the upper portion of the duff layer in a typical upland conifer forest (Van Wagner 1970). It provides operational users a reliable indication of lightning fire ignition potential and the depth of burning expected during and after the passage of fire. Many Canadian studies aimed at understanding the factors influencing lightning fire ignition have also found moisture in other fuel layers (i.e. Fine Fuel Moisture Code, FFMC and Drought Code, DC) can also play a role in influencing daily lightning fire occurrence (e.g. Anderson 2002; Wotton and Martell 2005; Wotton et al. 2010; Nadeem et al. 2019); however, in terms of the lightning–fire ignition relationship, organic layer moisture (as indicated by the DMC) is most frequently found to be the most impactful factor. Over the years, a heuristic has been adopted in operational use across the country, suggesting that a DMC value of 20 represents a threshold below which smouldering in the forest floor is not sustainable and will extinguish. This heuristic, or simple variants of it, are widely used in fire management operations to identify when lightning ignitions become possible and when fires will self-extinguish and have been incorporated into guidance provided during operational training (e.g. Alexander 2002; Lawson and Armitage 2008; Zeil 2011, table 3 in Hanes et al. 2021). The threshold has also been utilised in landscape fire growth analyses as an extinction point for active fires and in lightning fire occurrence models (Anderson 2010; Hély et al. 2010; Wang et al. 2014, 2017; Stralberg et al. 2018; Dawe et al. 2022).
Many studies relying on this threshold refer to Anderson and Englefield (2001)’s analysis of lightning fires (in the province of Saskatchewan) as the source of evidence for the threshold; others have pointed to Flannigan and Wotton (1991)’s analysis of lightning fires in Ontario as a source of supporting evidence. Both of these studies specifically examined the partitioning of lightning fire occurrence based on the existing DMC = 20 heuristic but did not explore whether this was indeed the most appropriate threshold. The origin of the rationale for the DMC = 20 threshold as a limit to sustained smouldering combustion and, thus, lightning ignition, seems to have two sources in the citable literature in Canada. The first of these is Van Wagner’s (1972) summary of 12 experimental fires he conducted in eastern Canadian pine forests. Those data (fig. 2 in Van Wagner 1972) suggested DMC must be above the low 20s for there to be measurable duff consumption during a fire. Kourtz (1974), in one of the first studies linking output from lightning strike detectors, lightning fires and fuel moisture, suggested that an area was most likely to have lightning ignitions if the DMC was 20 or greater (and there was significant lightning activity). This dataset consisted of 96 fires and examined weather conditions for 96 days in the province of Ontario; the study did not include a rationale for the choice of the DMC of 20 threshold; it simply stated that for the 96 days studied, it provided a good threshold.
The moisture codes in the FWI System are designed such that greater numerical values are associated with lower fuel moisture values (i.e. drier conditions) (Van Wagner 1987); a DMC value of 0 implies saturation (300% moisture). The standard conversion between moisture content and DMC (Van Wagner 1987) indicates that a DMC of 20 corresponds to a gravimetric moisture content in the duff layer of 196%. Considerable laboratory work on sustainable smouldering (Fransden 1987, 1997) has shown that, in the decaying material of the upper duff layer (the fibric organic soil layer, often called the F-layer), smouldering combustion becomes unsustainable at moisture above 110% (a DMC equivalent of 49). Lawson et al. (1997) reported similar ignition thresholds (varying from 76% (DMC = 70) to 118% (DMC = 46)) in various F-layer duff types in western Canada. Wotton et al. (2005) felt the drier conditions in the rain-sheltered and less dense (i.e. faster drying) duff layers near the base of overstorey trees might explain the differences between the well-known heuristic and experimental observations. They developed a Sheltered Duff Moisture Code to track moisture in these duff layers.
The objective of this paper is to explore the strength of the evidence behind the DMC of 20 heuristic that is widely used across Canada using additional data sources. Specifically, we explore the relationship of forest floor duff consumption to DMC for several experimental burning studies documented after Van Wagner’s experimental burning. We also use lightning fire, weather and lightning strike location information to explore the empirical probability of a lightning stroke leading to a sustainable ignition as a function of DMC throughout Canada.
Data and methods
Lightning ignition
We assembled datasets of lightning strikes, daily fire weather station observations and lightning fire occurrence for the provinces of British Columbia, Alberta, Saskatchewan, Manitoba and Ontario for the years 2000–2019. These locations were chosen as they represented a good span of the Canadian forest with very significant lightning fire occurrence and were areas with datasets we had available because of ongoing applied research on lightning fire occurrence modelling.
Lightning flash data were from a Canadian Forest Service (CFS) research dataset from the Canadian Lightning Detector Network (CLDN: Kochtubajda and Burrows 2020). These lightning flash data included attributes such as each strike’s location, time of occurrence, multiplicity and average discharge strength. We used multiplicity (which represents the number of individual discharges (strokes) associated with a lightning flash) to summarise the data as lightning stroke counts.
Daily fire weather data were assembled from various agency archives and from Environment Canada records. These daily fire weather data observations included calculated FWI System outputs at each station throughout each fire season (Van Wagner 1987).
Fire occurrence records were assembled from individual fire agencies. These records contained information on the estimated start location of each reported fire, its general cause and the day it was reported to the fire agency. We used only lightning fires. An estimated start date for a fire is sometimes provided in these records. However, Wotton and Martell (2005) found that this start date estimate was unreliable; on ~50% of the estimated start days, there was no lightning active within a 25 km radius of the ignition location. We used the lightning flash data to estimate the likely start date at each fire location. For a 10 km radius around each fire ignition location, lighting stroke counts were summarised for each day, moving backwards in time from the fire report date for 21 days. The first day on or prior to the report date for which there was lightning activity within a 10 km radius of the fire location was assumed to be the start date. In previous lightning fire occurrence modelling work (Wotton and Martell 2005), this was found to provide a better start date estimate than looking for the closest strike. If no lightning was found in the 21 days prior to the report date, the fire was excluded from our analysis.
In provinces where the area under fire management spans major ecological zones, we summarised data and modelled ignition probability separately within these regions (Ecological Stratification Working Group (ESWG) 1996). For provinces with a ‘modified response zone’ (where fires that occur are often intentionally left unsuppressed), we split provinces by these general boundaries as well; in a ‘modified response zone’, detection effort may be lower (Fig. 1). For this analysis, we excluded fires from these modified response zones.
Regions used in the lightning fire ignition analysis. Each province is divided into its major ecological zones (ECWG 1995) and further divided to exclude the portions of modified response zones in the north of some provinces. The delineations of the fire management boundaries shown here are only simple approximations from fire management strategies circa 2000. We use them here to avoid large areas where detection efforts may be significantly different. In practice, such boundaries are not hard lines on a map – response decisions can vary on a fire-by-fire basis; therefore, this map should not be used as a reference for fire management zone boundaries in Canada.
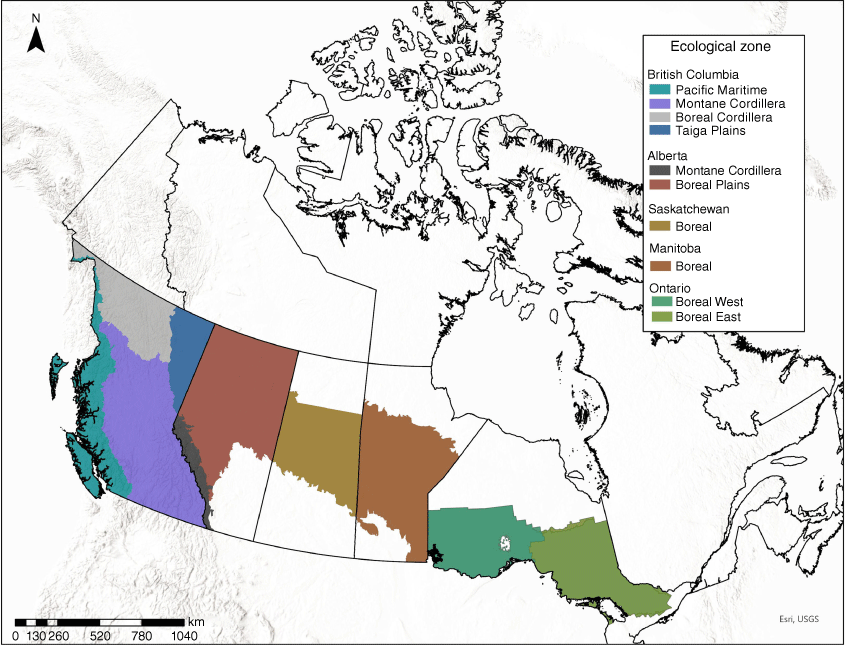
The base daily unit of analysis was a 20 by 20 km cell to account for potential inaccuracies in lightning and fire location (Wotton and Martell 2005). DMC values from the weather stations were interpolated using thin-plate cubic splines to the centre of each grid cell for each day. To capture the core of the fire season in Canada, we only included data from May through August. To demonstrate the effect of season, we also broke this full fire season into a spring period (May only) and a summer period (June through August). Because fire management policies and procedures can vary from province to province, we analysed data from each province separately. After first exploring data visually, we used shape-constrained generalised additive modelling (Wood 2017) to examine the probability of ignition from a single lightning stroke as a function of DMC. Shape-constrained generalised additive models can ensure that relationships between the predictor and response variable follow specific patterns. In this case, it was used to ensure the relationship between lightning ignition and fuel moisture was physically logical; the probability of ignition can only increase as the fuel moisture level decreases. Applying the shape constraints allowed us to reduce model overfitting from the variability in observations. All modelling was performed using the ‘scam’ package (Pya and Wood 2015) in R statistical software (R Core Team 2024).
Forest floor consumption
The CFS, in partnership with different fire management agencies, has continued to carry out large-plot fire behaviour experimentation since the work Van Wagner (1972) summarised. These experimental burning observations have formed the core of the fire behaviour models in the Canadian Forest Fire Behaviour Prediction (FBP) System (Forestry Canada Fire Danger Group (FCFDG) 1992). For the present analysis, we selected datasets from well-documented Canadian burning experiments included in the CFS Fire Behaviour dataset. Only experimental burning projects conducted in fuels with a pine overstorey were included; our goal was to be consistent with the general fuel type in Van Wagner’s original study. The experimental burning projects were: the Darwin Lake Project carried out in mature pine in northern Alberta (Quintilio et al. 1977); the Kenshoe Lake Project carried out in mature pine near White River, Ontario (Stocks 1989); the Sharpsand Creek Project in immature pine north of Thessalon, Ontario (Stocks 1987), and the International Crown Fire Modelling Experiment, carried out in mature pine north of Fort Providence, Northwest Territories (Stocks et al. 2004). Forest floor consumption observations and DMC values from the burn day were analysed similarly to the data presented in Van Wagner (1972, fig. 2 in that report).
Results and discussion
Lightning ignition
Table 1 shows an overall summary of lightning, fire and the occurrence of days above and below the existing DMC threshold of 20 for the portion of each ecozone within each province for the 2000–2019 study period. Overall, 35,343 lightning fires were included across the entire dataset. The percentage of lightning fires occurring at DMC values below 20 (Table 1), while never a majority of fires, reveals that a large number of fires occur at levels below the existing DMC = 20 threshold.
Province | Ecological zone | Number of lightning fires | Number of lightning strokes | Lightning ignition efficiency (no. strokes/no. fires) B | Percentage of fires (DMC ≤ 20) | Percentage of lightning strokes (DMC ≤ 20) | |
---|---|---|---|---|---|---|---|
British Columbia | Pacific Maritime | 784 | 102,678 | 131 | 13 | 31 | |
Boreal Cordillera | 168 | 396,459 | 2360 | 7 | 28 | ||
Taiga Plains | 953 | 1,442,838 | 1514 | 15 | 44 | ||
Montane Cordillera | 12,954 | 2,671,332 | 206 | 8 | 26 | ||
Alberta | Boreal Plains | 8920 | 15,450,164 | 1732 | 29 | 63 | |
Montane Cordillera | 215 | 612,672 | 2850 | 15 | 43 | ||
Saskatchewan | Boreal A | 2461 | 5,293,540 | 2110 | 17 | 60 | |
Manitoba | Boreal A | 2280 | 5,233,108 | 2295 | 17 | 52 | |
Ontario | Boreal East | 2884 | 3,192,791 | 1107 | 17 | 60 | |
Boreal West | 3724 | 3,085,764 | 829 | 25 | 70 |
The percentage of fires when DMC ≤20 represents is calculated using the DMC on the estimated fire start date.
Fig. 2 shows the probability of ignition of a sustainable fire by an individual lightning stroke as a function of DMC from the generalised additive modelling. The term ‘sustainable fire’ is used here to emphasise that our dataset, by definition, contains only fires detected and reported to the agency. Lightning ignitions that self-extinguish before they are ever detected are not included here, nor can their numbers currently be estimated in any reliable way. The data points shown in Fig. 2 are for visualisation and represent empirical estimates of the probability of ignition created by summarising fires and lightning strokes from the observed data into 40 bins, each representing one 2.5 percentile segment of the data (ordered by DMC). The probability of ignition from a lightning stroke can be seen to rise systematically, though non-linearly, with increasing DMC value; there is little indication of a change in the rate of change of the relationship around the DMC value of 20 across the various zones.
Empirical probability estimates and general additive models (GAMs) for each study region: (a) British Columbia, (b) Alberta, (c) Saskatchewan, (d) Manitoba, (e) Ontario. The shaded regions represent the 95% confidence interval from the fitted GAM. The points represent the empirical proportion of observed lightning-caused fires binned into 2.5% intervals of DMC (i.e. partitioning DMC into 40 equal categories). The grey dashed line indicates the currently used DMC of 20 threshold.
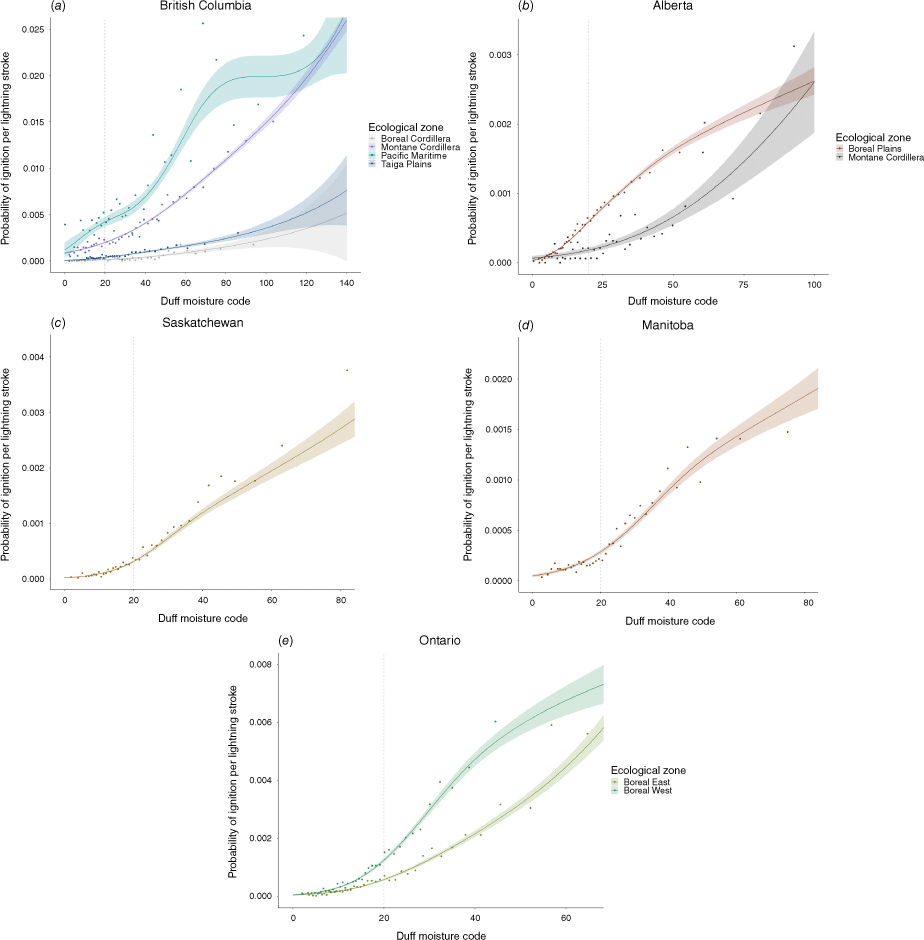
The positive and increasing relationship between DMC and the probability of lightning fire ignition is similar through the intensively managed portions of most of the boreal forest and taiga plains regions studied here (Fig. 2), except for the Northwest region of Ontario (Fig. 2e), where the rate of rise in probability is larger. The Montane Cordillera and Pacific Maritime ecozones in BC provide an interesting contrast to the boreal/taiga zones; the rate of increase in ignition probability with DMC is higher in these regions than seen through most of the boreal forest. The probability data for the Montane Cordillera in BC (in Fig. 2a) suggest that, in this region that regularly experiences high DMC values and a lot of lightning fire activity, the increase in the probability of ignition as DMC reaches very high values continues approximately linearly to the extremely dry end of the range of duff moisture. This may provide helpful guidance for modellers and fire managers extrapolating these empirical probability relationships in boreal forest regions beyond the historical range of DMC as the climate changes.
Although the majority of lightning fires in Canada occur in the summer, we also explored the ignition probability–DMC relationship for the spring and summer. Fig. 3 shows both spring and summer models for several of the regions we studied. The full-season model (the black line in Fig. 3) is very similar to the summertime model because the overwhelming majority of data (both fire and lightning) come from the summer period (e.g. the spring period has 7% the number of fires and 3.5% the lightning of the summer period). This much smaller spring period sample size leads to less consistent (and we suggest much less reliable) models of the ignition process, reflected somewhat in the wider confidence bands in the Fig. 3 spring models. There is some inconsistency across the country. For the same DMC value in the western provinces, ignition probability tends to be higher in the spring than summertime values; in Ontario, the opposite is observed. This seasonal exploration (Fig. 3), however, reveals no additional indication of a threshold around a DMC value of 20.
The probability of ignition per lightning stroke from generalised additive modelling for spring and summer periods and for selected regions in our study area: (a) Alberta, (b) Manitoba, (c) northwestern Ontario, and (d) northeastern Ontario. The blue line and associated shaded 95% confidence bands are from the spring data; the red line and associated shaded 95% confidence bands are for the summer period. The solid black line represents the full season (May through August) model from Fig. 2. The grey dashed line indicates the currently used DMC of 20 threshold.
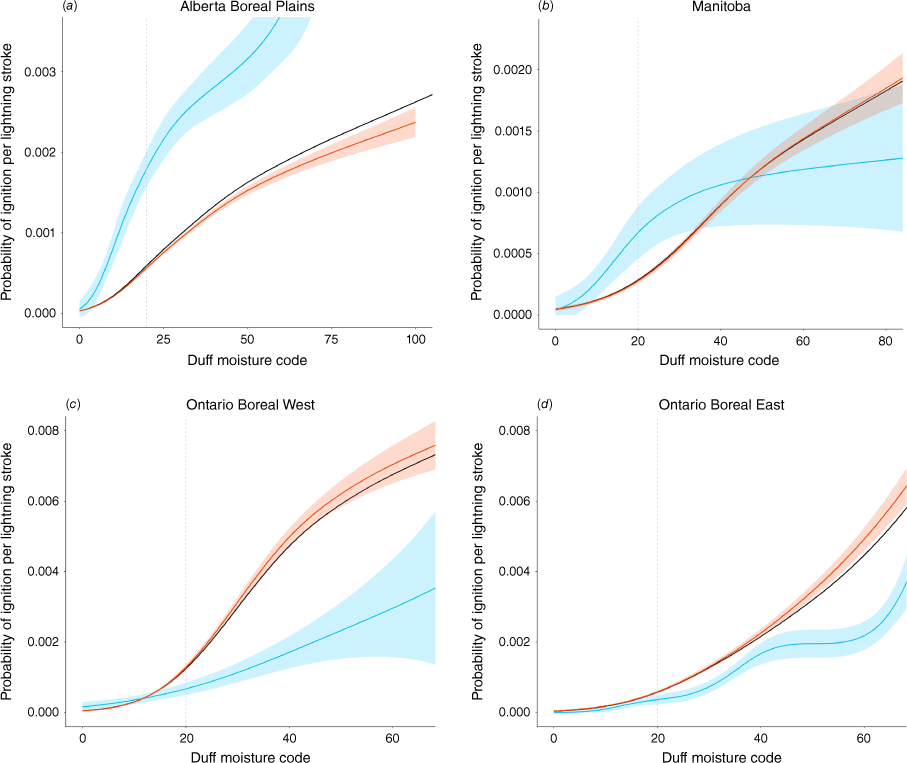
Forest floor consumption
Fig. 4 shows forest floor consumption and DMC values summarised for a series of experimental burning projects carried out in Canada over the last several decades. The observations from Van Wagner’s experimental burns through the 1960s (shown in his original work as fig. 2 in Van Wagner 1972) indeed suggest a threshold for forest floor consumption at a DMC of just over 20 (Fig. 4a); however, none of the data collected on subsequent, similar experimental burning projects show a similar relationship (Fig. 4b–e). A trend of increasing forest floor consumption with increasing DMC value is typically discernable; however, no meaningful or consistent intercept value where forest floor consumption is effectively negligible can be seen. The plotted results from Van Wagner (1972), which have been adopted in recent decades as ‘evidence’ of this threshold for forest floor consumption, seem to be more of an exception than a consistent observation of a meaningful threshold.
Forest floor consumption (kg/m2) observations from experimental fires associated with DMC values: (a) a replotting of data from all fires originally studied in Van Wagner (1972); (b) Darwin Lake Project; (c) Kenshoe Lake Project; (d) Sharpsand Creek Project; (e) International Crown Fire Modelling Experiment (ICFME).
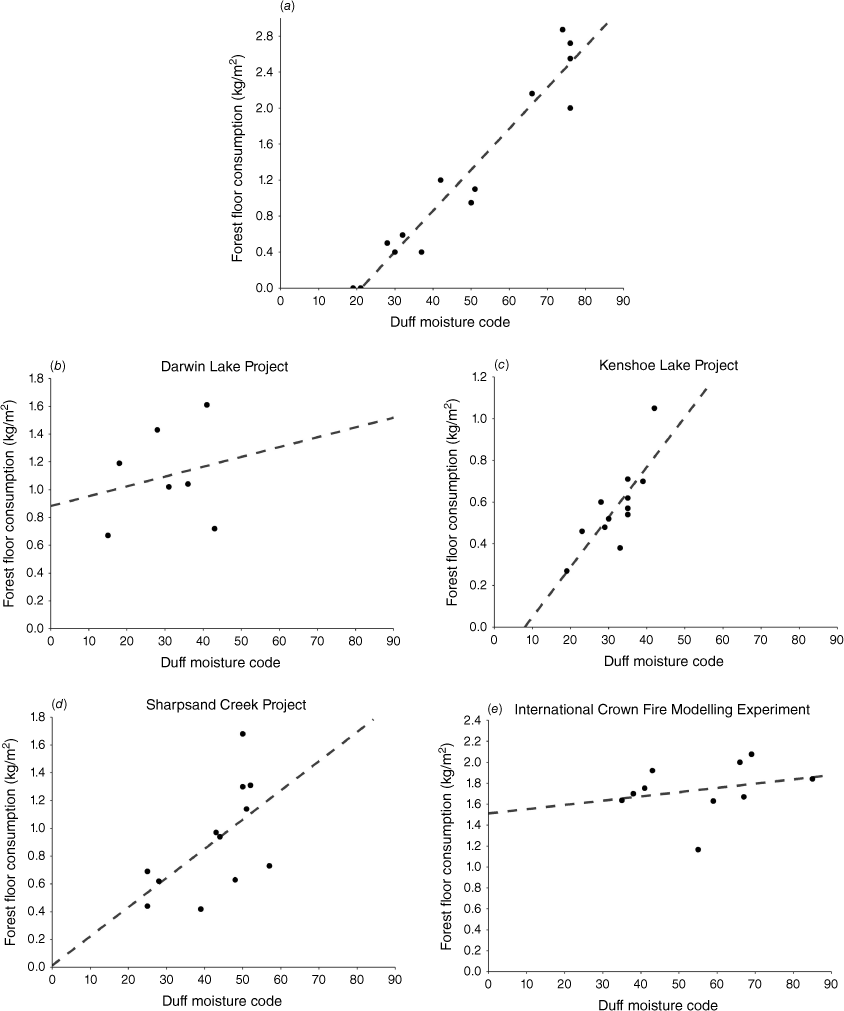
Further discussion and conclusion
The relationships in Fig. 2 show that the DMC is indeed a reasonable predictor of the receptivity of the forest floor to ignition from lightning; indeed, the DMC is used throughout Canada as the primary indicator of whether lightning storms will lead to ignitions. As DMC rises, so does the receptivity of the forest floor to lightning ignition. As such, above a level of DMC of 20, there is indeed a greater tendency for sustainable ignition. There is, however, no strong observational evidence for a threshold around a value of 20 (Figs 2, 3); a threshold of 30 or 10 might equally be considered meaningful. This is not to say that a threshold defined by a DMC of 20 (or some similar value) is not a useful heuristic in some contexts (e.g. providing new crew members with an initial sense of how to interpret the outputs of the FWI System quickly). In considering its use in more advanced decision-making contexts (e.g. intelligence officers predicting expected lightning fire occurrence), it should be understood that no evidence suggests this threshold is a better discriminator of lightning fire occurrence than other DMC values; it is simply a threshold of convenience. The lightning–DMC relationship (Fig. 2) can be used, however, to help provide a physical basis for a region’s adjective rating classifications (i.e. categorizations of conditions as “Low”, “Moderate”, High”, “Extreme”) of the DMC (e.g. Hanes et al. 2021).
Similarly, the DMC is a good indicator of the extent of forest floor consumption; as DMC increases, the expected extent of the burning depth of the forest floor tends to increase as well (e.g. Stocks 1987, 1989). That observation is physically logical, and it is not in dispute. The extended examination of the experimental burn data presented here suggests, however, that there is not a clear or consistent limit to forest floor consumption at a DMC of 20. This implies that there is no reason to infer that there is a smouldering extinction threshold at or around a DMC of 20.
Indeed, simple heuristics (like the DMC of 20 threshold) are valuable for making quick decisions in the dynamic environment of wildland fire management. They can significantly enhance operational efficiency when grounded in reality and easy to apply. However, their utility beyond rapid assessments should be contingent uon their general validity. Like any model, it is crucial to understand a heuristics’ origins, assumptions and limitations to avoid flawed decision-making. Over time, without scrutiny, there is a risk of these rules being institutionally accepted as fact without supporting evidence. Therefore, it is essential for fire scientists and managers to regularly re-examine the evidence supporting or contradicting established heuristics as new data emerge. Equally important is maintaining openness to revising these rules when warranted by evolving knowledge and field experience.
Data availability
The fire and weather datasets used here are obtained from individual provincial fire agencies under the agreement that their data not be shared by us. The lightning flash dataset summarised here is from the federal Department of Environment Canada and Climate Change under a data sharing agreement that prohibits making these data available. Summarised data that support the figures and conclusions will be shared on a reasonable request to the corresponding author; a table of GAM estimates and confidence ranges (Fig. 2) at each integer DMC value are also available from the authors.
Acknowledgements
We want to thank operational personnel at Canadian provincial fire management agencies who shared data with us and discussed the operational use of this lightning ignition threshold.
References
Alexander ME (2002) An overview of systems used for rating fire danger and predicting fire behavior used in Canada. Presentation as part of national S-590 course to National Advanced Resource Technology Center Marana, Arizona March 10–22, 2002 Available at https://www.frames.gov/documents/catalog/alexander_2002_s590-lesson-31_CFFDRS-overview-presentation.pdf
Anderson K (2002) A model to predict lightning-caused fire occurrences. International Journal of Wildland Fire 11(4), 163-172.
| Crossref | Google Scholar |
Anderson KR (2010) A climatologically based long-range fire growth model. International Journal of Wildland Fire 19, 879-894.
| Crossref | Google Scholar |
Dawe DA, Parisien MA, Boulanger Y, Boucher J, Beauchemin A, Arseneault D (2022) Short‐and long‐term wildfire threat when adapting infrastructure for wildlife conservation in the boreal forest. Ecological Applications 32(6), e2606.
| Crossref | Google Scholar | PubMed |
Ecological Stratification Working Group (ESWG) (1996) ‘A national ecological framework for Canada. Agriculture and Agri-Food Canada, Research Branch.’ (Centre for Land and Biological Resources Research and Environment Canada, State of the Environment Directorate: Ottawa, Canada). Available at https://sis.agr.gc.ca/cansis/publications/manuals/1996/A42-65-1996-national-ecological-framework.pdf
Flannigan MD, Wotton BM (1991) Lightning ignited forest fires in northwestern Ontario. Canadian Journal of Forest Research 21(3), 277-287.
| Crossref | Google Scholar |
Fransden WH (1987) The influence of moisture and mineral soil on the combustion limits of smouldering duff. Canadian Journal of Forest Research 17, 1540-1544.
| Crossref | Google Scholar |
Fransden WH (1997) Ignition probability of organic soils. Canadian Journal of Forest Research 27, 1471-1477.
| Crossref | Google Scholar |
Hanes CC, Wang X, Jain P, Parisien MA, Little JM, Flannigan MD (2019) Fire-regime changes in Canada over the last half-century. Canadian Journal of Forest Research 49(3), 256-269.
| Crossref | Google Scholar |
Hanes CC, Wotton BM, McFayden C, Jurko N (2021) ‘An approach for defining physically based Fire Weather Index System classes for Ontario.’ Information Report GLC-X-29. 35 p. (Natural Resources Canada, Canadian Forest Service, Great Lakes Forestry Centre). Available at https://cfs.nrcan.gc.ca/publications/download-pdf/40501
Hély C, Fortin M-J, Anderson KR, Bergeron Y (2010) Landscape composition influences local pattern of fire size in the eastern Canadian boreal forest: role of weather and landscape mosaic on fire size distribution in mixedwood boreal forest using the Prescribed Fire Analysis System. International Journal of Wildland Fire 19, 1099-1109.
| Crossref | Google Scholar |
Kochtubajda B, Burrows WR (2020) Cloud-to-ground lightning in Canada: 20 years of CLDN data. Atmosphere-Ocean 58(5), 316-332.
| Crossref | Google Scholar |
Nadeem K, Taylor SW, Woolford DG, Dean CB (2019) Mesoscale spatiotemporal predictive models of daily human-and lightning-caused wildland fire occurrence in British Columbia. International Journal of Wildland Fire 29(1), 11-27.
| Crossref | Google Scholar |
Pya N, Wood SN (2015) Shape constrained additive models (R package version 1.2-10). Retrieved from https://CRAN.R-project.org/package=scam
R Core Team (2024) R: A language and environment for statistical computing. (R Foundation for Statistical Computing: Vienna, Austria). Available at https://www.r-project.org/ [verified 11 September 2024]
Stocks BJ (1987) Fire behaviour in immature jack pine. Canadian Journal of Forest Research 17, 80-86.
| Crossref | Google Scholar |
Stocks BJ (1989) Fire behaviour in mature jack pine. Canadian Journal of Forest Research 19, 783-190.
| Crossref | Google Scholar |
Stocks BJ, Alexander ME, Wotton BM, Stefner CN, Flannigan MD, Taylor SW, Lavoie N, Mason JA, Hartley GR, Maffey ME, Dalrymple GN, Blake TW, Cruz MG, Lanoville RA (2004) Crown fire behaviour in a northern jack pine-black spruce forest. Canadian Journal of Forest Research 34, 1548-1560.
| Crossref | Google Scholar |
Stralberg D, Wang X, Parisien MA, Robinne FN, Sólymos P, Mahon CL, Nielsen SE, Bayne EM (2018) Wildfire‐mediated vegetation change in boreal forests of Alberta, Canada. Ecosphere 9(3), e02156.
| Crossref | Google Scholar |
Van Wagner CE (1972) Duff consumption by fire in eastern pine stands. Canadian Journal of Forest Research 2, 34-39.
| Crossref | Google Scholar |
Wang X, Parisien MA, Flannigan MD, Parks SA, Anderson KR, Little JM, Taylor SW (2014) The potential and realized spread of wildfires across Canada. Global Change Biology 20(8), 2518-2530.
| Crossref | Google Scholar | PubMed |
Wang X, Parisien MA, Taylor SW, Candau JN, Stralberg D, Marshall GA, Little JM, Flannigan MD (2017) Projected changes in daily fire spread across Canada over the next century. Environmental Research Letters 12(2), 025005.
| Crossref | Google Scholar |
Wierzchowski J, Heathcott M, Flannigan MD (2002) Lightning and lightning fire, Central Cordillera, Canada. International Journal of Wildland Fire 11(1), 41-51.
| Crossref | Google Scholar |
Wotton BM, Martell DL (2005) A lightning fire occurrence model for Ontario. Canadian Journal of Forest Research 35(6), 1389-1401.
| Crossref | Google Scholar |
Wotton BM, Stocks BJ, Martell DL (2005) An index for tracking sheltered forest floor moisture within the Canadian Forest Fire Weather Index System. International Journal of Wildland Fire 14(2), 169-182.
| Crossref | Google Scholar |
Wotton BM, Nock CA, Flannigan MD (2010) Forest fire occurrence and climate change in Canada. International Journal of Wildland Fire 19(3), 253-271.
| Crossref | Google Scholar |
Zeil R (2011) Working with the Great Lakes Fire and Fuels Information System Tools in Lake States Fire Management. Lake States Fire Science Network Technical Guide 11-1. Available at http://lakestatesfiresci.net/docs/LSFSC_TG_11-1.pdf