Drivers of California’s changing wildfires: a state-of-the-knowledge synthesis
Glen MacDonald A * , Tamara Wall B * , Carolyn A. F. Enquist C * , Sarah R. LeRoy D * , John B. Bradford E , David D. Breshears F , Timothy Brown B , Daniel Cayan G , Chunyu Dong H , Donald A. Falk
A University of California, Los Angeles, Bunche Hall 1152, Los Angeles, CA 90095, USA.
B Desert Research Institute, 2215 Raggio Parkway, Reno, NV 89512, USA. Email: tim.brown@dri.edu
C US Geological Survey, Southwest Climate Adaptation Science Center, 1064 E Lowell Street, Suite N427, University of Arizona, Tucson, AZ 85721, USA.
D Southwest Climate Adaptation Science Center, University of Arizona, 1064 E. Lowell Street, Suite N446, Tucson, AZ 85721, USA.
E Southwest Biological Science Center, US Geological Survey, Flagstaff, AZ 86001, USA.
F School of Natural Resources and the Environment, University of Arizona, Tucson, AZ 85721, USA. Email: mollyhunter@u.arizona.edu
G Scripps Institution of Oceanography, University of California San Diego, CA 92093-0230, USA. Email: dcayan@ucsd.edu, sasha@ucsd.edu
H School of Civil Engineering, Sun Yat-sen University, and Southern Marine Science and Engineering Guangdong Laboratory (Zhuhai), Zhuhai 519082, China. Email: dongchy7@mail.sysu.edu.cn
I College of Earth, Ocean, and Atmospheric Sciences, Oregon State University, Corvallis, OR 97331, USA. Email: erica.fleishman@oregonstate.edu
J Alaska Science Center, US Geological Survey, 4210 University Drive, Anchorage, AK 99508, USA. Email: rloehman@usgs.gov
K Western Ecological Research Center, US Geological Survey, 1655 Heindon Road, Arcata, CA 95521, USA. Email: pvanmantgem@usgs.gov
L Department of Native American Studies, UC Davis, CA 95616, USA. Email: brmiddleton@ucdavis.edu
M Vibrant Planet, Incline Village, NV 89450, USA.
N Department of Environmental Science and Policy, University of California, Davis, CA 95616, USA. Email: hdsafford@ucdavis.edu
O University of California, Davis, 1 Shields Avenue, Davis, CA 95616, USA. Email: Mwschwartz@ucdavis.edu
P Laboratory of Tree-Ring Research, University of Arizona, 1215 E. Lowell Street, Tucson, AZ 85721, USA. Email: trouet@arizona.edu
International Journal of Wildland Fire 32(7) 1039-1058 https://doi.org/10.1071/WF22155
Submitted: 8 July 2022 Accepted: 7 April 2023 Published: 22 May 2023
© 2023 The Author(s) (or their employer(s)). Published by CSIRO Publishing on behalf of IAWF. This is an open access article distributed under the Creative Commons Attribution-NonCommercial-NoDerivatives 4.0 International License (CC BY-NC-ND)
Abstract
Over the past four decades, annual area burned has increased significantly in California and across the western USA. This trend reflects a confluence of intersecting factors that affect wildfire regimes. It is correlated with increasing temperatures and atmospheric vapour pressure deficit. Anthropogenic climate change is the driver behind much of this change, in addition to influencing other climate-related factors, such as compression of the winter wet season. These climatic trends and associated increases in fire activity are projected to continue into the future. Additionally, factors related to the suppression of the Indigenous use of fire, aggressive fire suppression and, in some cases, changes in logging practices or fuel management intensity, collectively have produced large build-ups of vegetative fuels in some ecosystems. Human activities provide the most common ignition source for California’s wildfires. Despite its human toll, fire provides a range of ecological benefits to many California ecosystems. Given the diversity of vegetation types and fire regimes found in the state, addressing California’s wildfire challenges will require multi-faceted and locally targeted responses in terms of fuel management, human-caused ignitions, building regulations and restrictions, integrative urban and ecosystem planning, and collaboration with Tribes to support the reinvigoration of traditional burning regimes.
Keywords: California, climate change, fire suppression, fuel management, ignition sources, Indigenous burning, vegetation, wildfire, wildland fire, wildland–urban Interface.
Introduction
Between 1980 and 2020, western USA and Canada experienced striking increases in the mean annual area burned and the severity of wildfires, leading to increased costs in fire suppression across the region (Miller et al. 2009; LAO (Legislative Analysist Office) 2018; Williams et al. 2019; Parks and Abatzoglou 2020; Natural Resources Canada 2021; Pennick McIver et al. 2021). Wildfire in California played a large role. In a cost analysis of nine western states, it was found that during 2005–2015, the state of California was responsible for an average of 82% of the annual total fire suppression costs (a sum of reimbursed expenditures and state obligations) (Pennick McIver et al. 2021). The 1980s have been identified as an inflection point not only in wildfire regimes in California and western North America, but also in terms of warming and drying of climate (Dennison et al. 2014; Abatzoglou and Williams 2016; Westerling 2016; Holden et al. 2018; Parks and Abatzoglou 2020). The causes, consequences and solutions for increased wildfire risk continue to be widely discussed and debated among both professionals and the public. Such dialogues often underscore the current polarised and politicised nature of public discourse across the nation and state (Hartter et al. 2020). To build broad-based understanding and solutions, it is critical to have informed and credible sources that facilitate the identification and communication of the factors driving increased wildfire risk (Steelman and McCaffrey 2013; Kasperson 2014; Leverkus et al. 2020).
Here, we synthesise and assess the role of climate change and other factors in generating the increasing trend in wildfire size as an evidence-based contribution to the ongoing discourse surrounding California’s current wildfire challenges. The questions addressed in this synthesis are: (1) how has wildfire and land cover changed since 1980? (2) what is the specific role of climate change? (3) what is the role of historical land management (pre-1980)? and (4) what are the effects of land management and ignition sources on California fire regimes since 1980? We explore each question in-depth, in separate sections that end with a summary of takeaway points that readers can readily understand and, we hope, use in relevant decision- and policy-making. We conclude with a general consideration of the future of wildfire challenges and their mitigation in California.
How have wildfire dynamics and land cover changed since 1980?
Wildland fire is an important, ecologically beneficial process in many of California’s ecosystems (Van Wagtendonk et al. 2018). Over 20 different major vegetation types, experiencing a wide range of fire regimes and fire occurrences in individual years, can be recognised in the state (Ornduff et al. 2003; Safford et al. 2022). Fire was used by Indigenous peoples to shape the landscape long before the arrival of Spanish, Mexican, and American settler-colonists in the 18th and 19th centuries (Anderson 2005; Lake 2013; Kane et al. 2019; Knight et al. 2022). In addition to a growing body of Indigenous knowledge (e.g. Knight et al. 2022), early historical records of fire in California come from the diaries of Father Juan Crespi, who accompanied the Portola and Fages expeditions (1769–1772). Crespi describes many instances of burned landscapes and explicitly mentions the use of fire by Indigenous peoples for the purpose of hunting (Bolton 1927). Wildfires have been increasingly well documented over the 20th and 21st centuries. Over the last four decades, many researchers have identified a trend towards larger and more severe wildfires occurring more frequently across California and western USA compared with the earlier 20th century (Westerling et al. 2006; Miller et al. 2009; Williams et al. 2019; Parks and Abatzoglou 2020). Of the 15 states generally included in western USA, California has experienced the most pronounced impacts of these large and severe fires over the past 40 years (Moreira et al. 2020).
We analysed data compiled from state and federal fire jurisdictions by the California Department of Forestry and Fire Protection (CAL FIRE) to examine the trends in fire size over the period 1980–2020 (https://www.fire.ca.gov/stats-events/) (CAL FIRE 2021). The CAL FIRE data used here are annual summary statistics for the whole state. We found a statistically significant upward trend (P < 0.001) in annual area burned since 1980 (Table 1, Fig. 1). Furthermore, 18 of the 20 largest fires in recorded California history have occurred since 2003; four of these occurred in 2021, five in 2020, and another three occurred from 2017 through 2019 (https://34c031f8‐c9fd‐4018‐8c5a‐4159cdff6b0d‐cdn‐endpoint.azureedge.net/‐/media/calfire‐website/our‐impact/fire‐statistics/featured‐items/top20_acres.pdf?rev=be2a6ff85932475e99d70fa9458dca79&hash=A355A978818640DFACE7993C432ABF81). These trends follow a period of lower annual area burned and decrease in large fires in the mid-20th century, concomitant with policies of near complete fire suppression and cooler, moister climate (Keeley and Syphard 2021). The total number of wildfires recorded annually over approximately the post-1980 period shows a statistically significant negative trend (P < 0.001) that is consistent with a trend towards fewer, but larger, individual fires (Table 1, Fig. 1). Both of these data sets also reveal a high amount of interannual variability in addition to the overall trends.
Variable | Tau | Two-sided test P-value |
---|---|---|
Annual area burned 1980–2020 | +0.359 | 0.00099 |
Annual number fires 1987–2020 | −0.451 | 0.00018 |
Annual air temperature 1980–2020 | +0.374 | 0.00072 |
Annual VPD 1980–2020 | +0.478 | 0.00001 |
Positive tau values indicate upwards trends and negative tau values indicate downward trends. The tau values have a range of −1 to 1 and a value of 0 indicates no trend is present. All trends are statistically significant at P < 0.001.
Legend: The model regression line is shown in black. The mean 95% confidence interval is shown as light grey lines. The 95% interval for observations around the modelled regression are shown as dark grey lines. Fig. 1 (a) Annual area burned in California from 1980 to 2020. For illustrative purposes a linear regression line is fitted (R2 = 0.249, P < 0.0001). CAL FIRE estimates for 1987–2020 as of 11 August 2020. Note the annual area burned in 2020 is far above the 95% confidence envelope of the regression model in terms of observations. Data source: (CAL FIRE 2021) (https://www.fire.ca.gov/stats-events/, https://34c031f8‐c9fd‐4018‐8c5a‐4159cdff6b0d‐cdn‐endpoint.azureedge.net/‐/media/calfire‐website/our‐impact/fire‐statistics/2018_redbook_final.pdf?rev=26c8ffc6fef04ea8a77e00f488cb83bd&hash=19B77F82A19D93A7684C7618B6337482). 1980–1986 estimated from CAL FIRE perimeter dataset (https://frap.fire.ca.gov/frap-projects/fire-perimeters/, https://frap.fire.ca.gov/mapping/gis-data/). Fig. 1 (b) Total annual number of fires from 1987 to 2020. For illustrative purposes a linear regression line is fitted (R2 = 0.415, P < 0.0001). Different agencies have different minimum fire sizes for recording fire sizes and perimeters, but typically fires included in the database are 4 ha (10 acres) or greater. Data source: CAL FIRE 2021 (https://www.fire.ca.gov/media/11397/fires-acres-all-agencies-thru-2018.pdf).
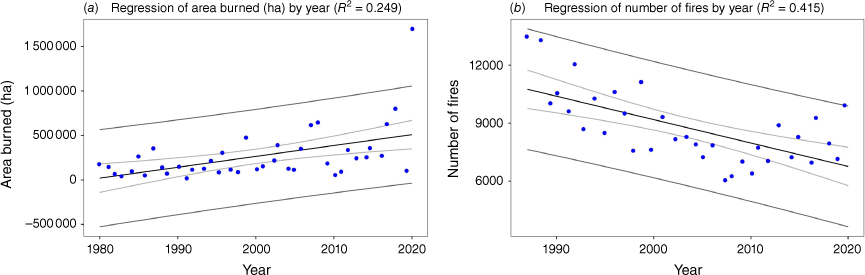
Moreover, since 2002, wildfires in California have destroyed more than 60 000 structures and caused 302 civilian and firefighter fatalities (Safford et al. 2021, 2022). Emblematic of these patterns is the 2018 Camp Fire, which resulted in 85 deaths and the destruction of 18 804 structures (https://www.fire.ca.gov/media/4jandlhh/top20_acres.pdf). The growth of residential development in wildland–urban interface (WUI) areas has placed increasing numbers of structures and people at risk and contributed to the economic toll of wildfires (Hammer et al. 2007; Radeloff et al. 2018; Keeley and Syphard 2019, 2021). WUI areas are found throughout the state, although most structures damaged by California wildfires are in non-forested land cover types (Schwartz and Syphard 2021). The estimated economic cost of the 2018 California wildfires was US$27.7 billion in capital losses, US$32.2 billion in health costs, and US$88.6 billion in indirect losses (Wang et al. 2021). Smoke produced by such wildfires can severely impact human respiratory and cardiovascular health, contributing to increased hospital admissions and emergency room visits (Adetona et al. 2016; Aguilera et al. 2021).
In addition to the area burned by wildfires, the severity of California forest fires, as measured by the degree to which fires have caused overstorey tree mortality and altered soils, has also increased over recent decades (Williams et al. 2023). Since 1980, trends in area burned and fire severity in California resemble the rest of western USA. From the mid-1980s through the mid-2010s, the number of large wildfires (those larger than 405 ha [1000 acres]) increased in nearly all ecoregions in western USA, as did the annual area burned overall (Dennison et al. 2014) and the annual area burned at high severity (Parks and Abatzoglou 2020). Specific to California, in recent decades, increasing area burned and severity has occurred in the Sierra Nevada and southern Cascades (Williams et al. 2023). A notable exception to the increase in fire severity was the California coastal zone (Parks and Abatzoglou 2020).
Although annual area burned is commonly used to estimate trends in wildfire over time, the metric can be misleading or misunderstood. This can lead to misrepresentation of fire dynamics and undermine the effectiveness of vegetation management. Reliance on the annual area burned metric can mask the ecological, social, and economic impacts of fire, which are critical to holistic or multidimensional measures of wildfire impact assessment (Moreira et al. 2020; Safford et al. 2022). Furthermore, what happens within the perimeter of a fire, such as spatial patchiness of fire severity, is often more relevant than the total area burned within the perimeter. For example, in California and western Nevada, the degree and extent of forest fire severity, as measured by the extent of stand-replacing fire, has increased considerably since 1984 (Miller et al. 2009; Miller and Safford 2012; Steel et al. 2015, 2018; Williams et al. 2023). In addition, increases in annual area burned and detrimental impacts are not equally distributed throughout California and its different land cover types (Williams et al. 2019; Parks and Abatzoglou 2020; Schwartz and Syphard 2021). For example, although annual area burned in north-western forests and the forests of the Sierra Nevada is increasing, such trends are not as readily apparent in California coastal vegetation complexes (Williams et al. 2019; Parks and Abatzoglou 2020; Safford et al. 2022). In this regard it is also important to recognise the difference in the often fuel-dominated large fire events in forested regions such as the northern mountain ranges versus the wind-dominated events that often typify large fires in shrubland-dominated vegetation of areas such as southern and coastal California (Keeley and Syphard 2019). Fire severity and vegetation recovery within burned areas is also geographically variable (Alizadeh et al. 2021; Guiterman et al. 2022).
To prepare and implement more effective management responses to California’s changing wildfire dynamics, it is important to develop a clear understanding of why fires are increasing in size and, in many cases, severity. Land cover change is one such potential driver. In semiarid, mixed-conifer forests, the primary land cover drivers of these trends are: (1) the accumulation of living and dead vegetation biomass (the fuel), reflecting the long-term legacy of fire exclusion and suppression (North et al. 2016, 2022; Safford et al. 2021); and (2) the concomitant increase in the proportion and persistence of dry biomass, exacerbated by climate-induced aridification of these fuels (Restaino and Safford 2018). For example, one analysis suggests that since the beginning of the 20th century, when the observational record began, no fires were recorded in 75% of mixed conifer forests in California (Steel et al. 2015). It should be recognised, however, that small fires in particular may have gone undetected and unaccounted for in historical records, particularly prior to the era of air and satellite remote sensing (Hua and Shao 2017). Nevertheless, large and severe fires were recorded in the early 20th century (Keeley and Syphard 2021). Fire histories, reconstructed from fire-scarred trees, indicate that for centuries before 1850 the same areas tended to burn as frequently as every 5–20 years (Anderson 1994, 1996; Trouet et al. 2010; Safford and Stevens 2017). In fact, estimates suggest that annual area burned totals of over 1.6 million ha (4 million acres) may not have been unusual (Anderson 1994, 2005; Stephens et al. 2007). However, between 2015 and 2020, some of these forests burned intensively in large patches over areas previously subject to decades of suppression, contributing to the state’s significant increases in total annual area burned, especially at high severity.
Land cover in California, including the location and intensity of forestry, agriculture, and urban development, has changed considerably since 1980. These land cover changes will continue to influence the likelihood of large and destructive fires, and fire itself has played a large role in some changes. The density of trees in some forest stands, particularly those in small diameter classes, has increased significantly since the mid-20th century, largely due to fire exclusion (McIntyre et al. 2015; North et al. 2022). Increased stand density has been accompanied by a shift toward a forest composition comprised of species that are less fire resistant than the species they are replacing (e.g. ponderosa pine [Pinus ponderosa] to white fir [Abies concolor]). Although the net percentage of lands used for agriculture has remained relatively stable in recent decades (Hart 2003; Sleeter et al. 2011), the locations of agricultural uses have changed. As suburban housing in the Central Valley of California expanded, nearby agricultural lands were converted to housing and commercial uses, and agricultural operations then shifted into previously uncropped lands in chaparral, woodlands, and forests and in California’s foothills (Sleeter et al. 2011). Expansion of livestock grazing in arid and semiarid regions of California, especially those that overlap with the Great Basin and Mojave Deserts, contributed to expansion of non-native invasive grasses and forbs that are fire-adapted yet highly flammable and, ultimately, can lead to changes in fire dynamics (Williamson et al. 2020). Additionally, as the percentage of developed land increased 37% from 1973 to 2000, the location of these developed lands and the associated loss of open space also influenced societal vulnerability to wildfire (Sleeter et al. 2011). Contrary to popular perception, the great majority of large wildfires during 1992–2019 originated on private or corporate lands and spread onto public lands (Downing et al. 2022).
Fire itself is a major driver of land cover change in California. Changes due to fire can be ephemeral as post-fire succession proceeds, but they can also lead to vegetation conversion (Steel et al. 2018; Guiterman et al. 2022). In California, despite the diversity of vegetation cover types, most changes have occurred in chaparral, oak woodlands, and in many forests of the Sierra Nevada (Safford et al. 2022). Between 1985 and 2021, primarily as a result of fire, forest cover decreased by nearly 7%, whereas shrub, herbaceous, and bare ground cover each increased by about 3% (Wang et al. 2022). Most of the increases in shrub cover occurred as successional (or type-conversion) responses to fire-driven montane forest loss. Even though southern California saw an overall gain in montane shrub cover between 1985 and 2021, there are ongoing losses in lowland shrublands and associated serotinous conifers driven by highly frequent anthropogenic fire (Safford and Van de Water 2014; Keeley and Safford 2016; Safford et al. 2021). Housing development is playing an indirect role in this process because it leads to increased human ignitions and replacement of shrublands by weedy, non-native annual grasses and herbs, which are much more flammable than the shrublands they replace (Syphard et al. 2018a, 2018b). How changes in vegetation will influence future wildfire regimes is an important concern, given that most land cover change (e.g. forest to shrubland and shrubland to grassland) occurs in the direction of vegetation that can carry more frequent, faster-moving fire likely to damage structures (Syphard et al. 2019; Schwartz and Syphard 2021).
In summary, wildfire dynamics and land cover have changed in California since 1980 in the following ways:
The annual area burned by wildfires in California has shown a statistically significant upward trend from 1980 to 2020, where 18 of the 20 largest fires recorded occurred since 2003 (five occurred in 2020 and four occurred in 2021) (https://www.fire.ca.gov/media/4jandlhh/top20_acres.pdf). Nonetheless, average annual area burned during this period is still well below estimates of annual burning before colonial settlement.
The annual number of fires shows a statistically significant downward trend between 1980 and 2020, indicating the growing prominence of large-area fires.
Since 1984, low- and middle-elevation forests in California (and some high-elevation forests) have experienced increasing levels of fire severity, with deleterious environmental and socio-economic consequences.
Land cover changes influence fuels and have both affected, and been affected by, the fire regime.
Human development and associated land cover change in the WUI have increased the economic and human costs of wildfires.
How has climate change affected wildfires in California since 1980?
Changes in California’s climate over past decades are key contributors to trends in fire-related activity. Higher temperatures and decreased precipitation, for example, can accelerate the drying and enhance the flammability of vegetative fuels (Flannigan et al. 2016). Increasing global and regional temperatures of about 1.1°C (~2°F) since 1980 (Fig. 2) are largely attributable to anthropogenic greenhouse gas emissions (Bonfils et al. 2008; Diffenbaugh et al. 2015; Abatzoglou et al. 2016; Williams et al. 2020). The rate of observed global warming has accelerated in recent decades (Smith et al. 2015). The recent and sustained warm annual temperatures are unprecedented over the past 1000 years, when comparing increases in annual or seasonal temperature over the 20th and early 21st century across the Northern Hemisphere with paleoclimatic data (Stevens et al. 2008; Salzer et al. 2009, 2014; Ljungqvist et al. 2012; Landrum et al. 2013; Loisel et al. 2017; de Boer et al. 2019).
Legend: The model regression line is shown in black. The mean 95% confidence interval is shown as light grey lines. The 95% interval for observations around the modelled regression are shown as dark grey lines. Fig. 1 (a) California average annual air temperature from 1980 to 2020. A linear regression line is fitted for illustrative purposes (R2 = 0.330, P < 0.0001). Data source: NOAA National Centers for Environmental Information, Climate at a Glance: Statewide Time Series, published April 2021, retrieved on 5 May 2021 from https://www.ncdc.noaa.gov/cag/. Fig. 1 (b) California average May–October Vapour Pressure Deficit (VPD) from 1980 to 2020. A linear regression line fitted for illustrative purposes shows a significant trend of increasing annual temperature (R2 = 0.433, P < 0.0001). Data source: Williams et al. (2019) and updated by Williams, pers. comm.
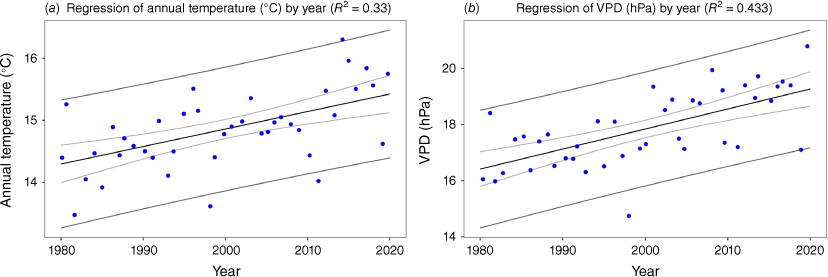
Throughout California precipitation is concentrated in the cool season months and there is a high degree of year-to-year variability. In contrast to temperature trends, there is no evidence of a significant long-term trend in cool-season precipitation (Pierce and Cayan 2013; Diffenbaugh et al. 2015). Higher temperatures do promote aridity, however, by decreasing the relative humidity of the atmosphere, increasing rates of evaporation from land surfaces and sublimation from snow. With warming, the winter precipitation season in California has become temporally compressed over the past six decades, contributing to a lengthened fire season (Goss et al. 2020; Luković et al. 2021). Warming has also accelerated snowmelt in the Sierra Nevada since the mid-20th century (Stewart et al. 2005), and wildfire itself may be contributing to this trend (Hatchett et al. 2023). The trend and impact of increasingly higher temperatures on aridity is highlighted by paleoclimatic studies. Drought conditions in the early 2010s, as measured by the Palmer Drought Severity Index (a measure of soil moisture) in central California and the deficit in snowpack in the Sierra Nevada, were among the most severe over the past 500–1200 years, whereas precipitation deficits over the same period were not anomalous (Griffin and Anchukaitis 2014; Belmecheri et al. 2016; Lepley et al. 2020).
Warmer air temperatures increase saturated water vapour pressure (maximum amount of water vapour that can be in the air), and in the absence of increased atmospheric moisture this causes an increase in the vapour pressure deficit (VPD). VPD is the difference between the water vapour pressure at saturation and the actual water vapour pressure of near-surface air. Globally, VPD has been increasing since the 1990s in tandem with increasing air temperatures (Yuan et al. 2019). VPD has been shown to be an important predictor of wildfire potential in California and the rest of western USA (Seager et al. 2015; Williams et al. 2019; Chen et al. 2021; Dong et al. 2022). High water vapour deficits produce increased evapotranspiration and promote fires through the drying of land surfaces and vegetative fuels (Abatzoglou and Williams 2016). VPD has been increasing in California at a statistically significant rate during the May–October warm season (Fig. 2), a trend that is projected to continue (Williams et al. 2019). Zhuang et al. (2021) estimated that 68% of the upward trend in VPD in western USA between 1979 and 2020 is likely attributable to anthropogenic warming.
The increasing trend in annual area burned in California over the period of 1980–2020 is significantly (P < 0.001) correlated with the observed increases in temperature and associated increases in VPD (Fig. 3). It is estimated that human-caused climate change has caused over half of the documented increases in fuel aridity in western USA since the 1970s, and this doubled the cumulative area of forest burned since the 1980s (Abatzoglou and Williams 2016; Kitzberger et al. 2017). The moisture content of dead vegetative fuel, particularly in spring, is projected to continue decreasing across California in the future, caused mainly by temperature increases (Liu 2016). Live fuel moisture will also decrease and promote greater fire risk in some systems (Ma et al. 2021). Warmer temperatures and earlier spring seasons have led to earlier flammability of fuel vegetation throughout western USA, with the Sierra Nevada experiencing some of the greatest drying related to early spring snowmelt (Westerling et al. 2006; Westerling 2016).
Legend: The model regression line is shown in black. The mean 95% confidence interval is shown as light grey lines. The 95% interval for observations around the modelled regression are shown as dark grey lines. Fig. 1 (a) Regression analysis of annual area burned from 1980 to 2020 and average annual air temperature. There is a significant relationship between annual average temperature and area burned over the time period (P < 0.001). Note the extreme outlier in area burned in 2020. Data source: see Figs 1 and 2. (b) Regression analysis of annual area burned from 1980 to 2020 and VPD. There is a significant relationship between VPD and area burned over the time period (P < 0.0001). Data source: see Figs 1 and 2.
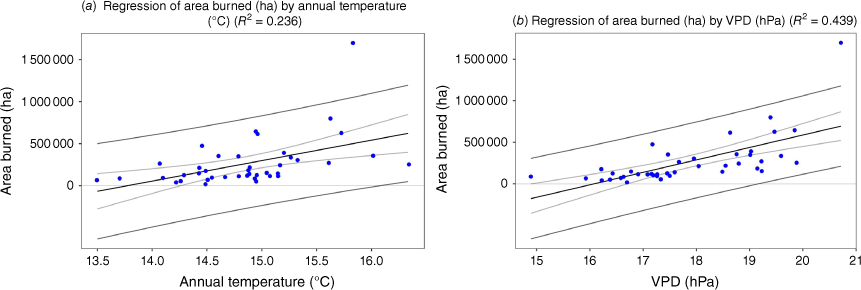
Future projections suggest the upwards trends in temperature, lengthening of the fire season, and increased VPD will continue and increase in signal strength (Pierce et al. 2013; Williams et al. 2019; Swain 2021; Dong et al. 2022). Taken together, these climate trends contribute to increasing fire risk in California (Restaino and Safford 2018). Higher temperatures, compressed precipitation season, and earlier onset of snowmelt result in drier live and dead vegetation, which contributes to increased flammability of large portions of the landscape for longer periods of time (Westerling et al. 2006; Miller et al. 2011; Jolly et al. 2015; Kitzberger et al. 2017; Ma et al. 2021), and exacerbates summer fuel-dominated wildfires (Williams et al. 2019). Furthermore, the effective temporal compression of California’s rainy season, combined with high-intensity weather events such as Santa Ana winds, directly contributes to increasing wildfire risk (Westerling et al. 2004; Swain 2021; Cayan et al. 2022). Increasing temperatures and decreasing precipitation in autumn are linked to increases in extreme fire weather, enhancing the risk of large autumn wildfires (Williams et al. 2019; Goss et al. 2020).
Deficits in summer and winter precipitation have been shown to promote wildfires (Littell et al. 2016; Holden et al. 2018). Higher temperatures exacerbate the fire risks associated with both prolonged and flash droughts, which are associated with the occurrence of synchronous fire years across large parts of western USA (Westerling and Swetnam 2003; Heyerdahl et al. 2008; Littell et al. 2016; Crockett and Westerling 2018; Williams et al. 2020), more large fires (>405 ha [1000 acres]), and an increase in annual burned area (Abatzoglou and Kolden 2013; Riley et al. 2013; Kitzberger et al. 2017). For example, the 2012–2016 drought, intensified by warming, contributed to high biomass of dead and dry vegetation (Williams et al. 2020), providing abundant and flammable fuel for California wildfires during that period.
Although fire is a natural process in many of the ecosystems in California, climate change and fire are interacting to alter the structure and function of ecosystems. In ecosystems with sufficient fuels to carry fire, warmer and drier climates are expected to decrease vegetation moisture and increase flammability and wildfire (Gergel et al. 2017; McKenzie and Littell 2017; McEvoy et al. 2020). Tree mortality from causes other than fire (e.g. drought and insects) alters fuel loads and conditions, and also microclimates in ways that can affect fire spread patterns (Ruthrof et al. 2016). Vegetation recovery following wildfire can also be affected by atmospheric demand for moisture (i.e. VPD); for example, pinyon pine (Pinus edulis) and ponderosa pine (Pinus ponderosa) seedling mortality occurs more rapidly with increasing temperature during drought (Adams et al. 2017; Stevens-Rumann et al. 2018), which may be a factor driving recruitment failure (Keeley et al. 2019).
Projections of climate for the 21st century indicate continued occurrence of weather and climate patterns that heighten fire risk (Restaino and Safford 2018). As subtropical high pressure expands due to polar-amplified warming (Johanson and Fu 2009), the wet season in California is projected to become shorter, as is the Santa Ana wind season (Pierce et al. 2013, 2018; Swain et al. 2018; Guzman-Morales and Gershunov 2019; Swain 2021). The frequency of precipitation events is projected to decrease (Polade et al. 2014, 2017), especially during spring and autumn (Pierce et al. 2013, 2018; Swain et al. 2018). Drier springs will add to the stress imposed by increasingly hot summers, including heat waves, which will make vegetation even drier and more flammable. A later start of the rainy season (Pierce et al. 2013) is likely to prolong the period during which vegetation is sufficiently dry to burn and enhance coastal wildfire activity by extending dry fuels into the November ramp-up towards the December peak in downslope wind activity (Guzman-Morales et al. 2016; Syphard et al. 2018a, 2019; Swain 2021; Cayan et al. 2022). VPD will increase as the century progresses which will contribute to substantial increases in the number of days with high fire weather risks and the duration of the fire season (Dong et al. 2022). Although climate projections indicate that Santa Ana winds will prevail as an important phenomenon in future decades, changes in Santa Ana and similar wind regimes appear to be complex (Pierce et al. 2018; Guzman-Morales and Gershunov 2019), and their impact upon future fire risk remains an area of importance for research. In particular, future projections of Santa Ana winds need to resolve the hot and cold flavours of these winds (Gershunov et al. 2021).
Increasing weather variability, which is another component of climate change, also affects wildfire dynamics. In particular, instrumental data have shown (and multiple climate models suggest) that across much of California, the number of days without precipitation and interannual variability in precipitation will continue to increase (Polade et al. 2014; Berg and Hall 2015; Swain et al. 2018; Zamora-Reyes et al. 2022). Furthermore, it is highly likely that enhanced meteorological droughts (defined as evaporative demand minus precipitation) will translate to increases in the severity of ecological droughts (Crausbay et al. 2017; Bradford et al. 2020; Ukkola et al. 2020; McDowell et al. 2022) that decrease soil moisture, increase drying of live vegetation and surface litter fuels, and promote stress and potential mortality of plants (van Mantgem et al. 2020; McDowell et al. 2022).
In summary, since 1980, climate change has played a central role in the changing nature of California wildfires and will likely continue to do so in the following ways:
The climate of California became warmer and more arid from 1980 through 2020, exceeding earlier 20th century conditions and likely those of the past 1000 years. This has increased fuel flammability and the length of the fire season in most of California’s ecosystems. Increasing atmospheric VPD has drawn recent attention as an important metric linking climate to vegetative fuel conditions and fires.
Human-caused increases in greenhouse gases are the leading contributor to climate change and the associated increase in annual area burned in California. It is contributing to warmer temperatures, greater VPD, longer and more severe droughts, a longer fire season, and drier vegetation, all of which contributed to increases in the annual area burned in California. Climatic drivers of fire activity are projected to strengthen during the 21st century given current trends in greenhouse gas emissions. Climate projections to the year 2100 indicate that the impacts of warming, including increased VPD, more-volatile weather, more winter-concentrated precipitation, and elevated probability of extreme hot-dry weather events with high fire risk will push California wildfire activity to even higher levels.
What is the role of historical land management (pre-1980) in California wildfires?
Changing fire regimes and consequences deduced from dendrochronological and historical records
To enhance our understanding of the mechanisms and impacts of shifts in fire regimes and ecosystems over the period of 1980 to the present, and in general over the past 150 years of Euro-American dominated land management practices (Loomis 2017), it is important to frame these changes within a longer temporal context (Falk et al. 2011; Safford et al. 2012; Swetnam et al. 2016; Margolis et al. 2022). Indigenous burning practices have a long history across California (Anderson 1994, 2005; Lightfoot et al. 2013; Aldern and Goode 2014; Long et al. 2021). Historical and contemporaneous applications of these practices demonstrate numerous positive outcomes, both ecologically and culturally. These include the increased prevalence of culturally important species (Aldern and Goode 2014; Long et al. 2016; Goode et al. 2018; Marks-Block et al. 2019), increases in water availability to vegetation (David et al. 2018; Goode et al. 2018), and reductions in the probability of high-severity wildfire (Gumz 2020). Recently, there has been growing attention to, and support for, Indigenous-led cultural burning practices (Harling 2015; Marks-Block et al. 2019; Cowan 2020; Hagemann 2020; Tripp 2020; Adlam et al. 2021; Knight et al. 2022). The relationship between modern prescribed fire strategies, used primarily for reduction of wildfire risk and ecological benefits (Ryan et al. 2013), and the broader cultural and stewardship goals and meanings of Indigenous cultural burning practices, is important and deserving of greater analysis (see for example Kerlin 2020).
Tree-ring records of forest stand ages and fire scars from the coniferous forests of the California mountains document fire regimes and forest conditions prior to European colonisation of North America (Stephens and Fry 2005; Stephens and Gill 2005; Fry and Stephens 2006; Everett 2008; Swetnam et al. 2009; Bernal et al. 2022). In dense areas of higher-elevation forests in northern California (>~7000 ft), pre-colonial fires were relatively infrequent events of mixed or sometimes high severity that usually affected limited areas but could expand during periods of hot, dry, windy weather (Meyer and North 2019). Higher-severity events can be reconstructed by studying stand ages (Brown and Swetnam 1994; Taylor 2000; Beaty and Taylor 2001), in addition to providing a record of scarred trees that show a mosaic of fire severity intensities (Margolis et al. 2011; O’Connor et al. 2014; Heyerdahl et al. 2019). Fire scars in the stems of surviving trees in lower-elevation forests and woodlands (<~2100 m ~7000 ft) across California also show that pre-20th century fire regimes in many ecosystem types consisted primarily of frequent, relatively non-destructive surface and mixed-severity fires (Taylor and Skinner 2003; Taylor et al. 2008, 2016).
Tree survivorship in forests with frequent-fire regimes was highly size-dependent: larger overstorey trees with thick bark, high crowns, and deep root systems tended to survive, but a high proportion of smaller trees (seedlings and saplings), especially of fire-intolerant species like white fir and incense cedar (Calocedrus decurrens), were killed (Safford and Stevens 2017). As a consequence, frequent fire tended to maintain relatively open forests and woodlands (approximately 20–200 trees/acre), allowing for extensive light penetration to the forest floor (Safford and Stevens 2017; Bohlman et al. 2021; Hagmann et al. 2021). Detailed fire scar records of this type of fire regime are especially abundant since AD 1600, but some records, such as those from giant sequoia (Sequoiadendron giganteum), date back more than 2000 years (Swetnam 1993, 2009). In addition to regulating forest stand density, frequent surface fires killed or consumed the abundant smaller woody plant stems (<15 cm diameter) and other surface fuels that are common in forests and serve as the primary carrier of surface fire spread and intensity.
Prior to 20th century fire exclusion, frequent, relatively non-destructive surface and mixed-severity fires occurred at intervals of 5–20 years in seasonally dry forest types (Taylor and Skinner 2003; Taylor and Beaty 2005; Trouet et al. 2010). As stated previously, the approximately 1.6 million ha (4 million acres) burned during the 2020 fire season is likely more typical of pre-colonial area burned than the low annual totals of the mid- to late 20th century (Stephens et al. 2007; Safford et al. 2022). Prior to 1775 and colonial expansion, fire regimes showed inherent natural variability, influenced by factors such as climate and vegetation, and the extensive impacts of Indigenous use of fire (Anderson 1994, 2005; Lake 2013; Lightfoot and Cuthrell 2015; Taylor et al. 2016). Cultural burning practices maintained meadows, raised the water table by decreasing shrub and dense tree growth, and contributed to fresh plant growth for wildlife and cultural uses (Anderson 1994; Huntsinger and McCaffrey 1995; Kimmerer and Lake 2001; Aldern and Goode 2014; Long et al. 2016; Goode et al. 2018). Rapid depopulation of Indigenous peoples due to the introduction of European diseases – as well as oppressive policies at the state (see Johnston-Dodds 2002; Lindsay 2012; Madley 2016) and federal levels (Harley 1918) that attempted to remove Indigenous people and prohibit their traditional land management and cultural practices – resulted in a decline in cultural burning starting in the late 18th century.
The dendrochronological evidence is consistent with historical documents, indicating that after approximately 1850, the reduction in fire activity associated with the decline in Indigenous populations and use of fire was amplified by the widespread introduction of grazing livestock to ecosystems across much of the state and concomitant decrease in spreading fires. The subsequent increase in fire suppression in the 20th century, which along with climatic conditions contributed to low annual area burned totals (Keeley and Syphard 2021), also altered coniferous forest fire regimes and vegetation (Taylor and Skinner 2003; Stephens and Fry 2005; Fry and Stephens 2006; Stephens et al. 2007; Beaty and Taylor 2008; Lorimer et al. 2009; Skinner et al. 2009; Swetnam et al. 2009; Scholl and Taylor 2010; McIntyre et al. 2015; Taylor et al. 2016; Knight et al. 2022). As elaborated in the section below, the alteration of fire regimes and vegetation has increased the fuel load available for fires in many forests and helped to drive the strong increase in forest fire severity seen since the 1980s (Steel et al. 2015; Safford et al. 2022; Williams et al. 2023). In addition, there is evidence that in some ecosystems the loss of Indigenous burning practices has altered the sensitivity of wildfire behaviour to natural climate variability and ongoing climate change (Fry and Stephens 2006; Taylor et al. 2016; Hankins 2021).
Consequences of fire exclusion and timber harvest practices of the past 150 years
Fuel loads have increased across much of the forest land base in western USA due to direct and indirect fire exclusion over the past 150 years (North et al. 2015a, b; Tubbesing et al. 2019). Direct (or active) fire exclusion is exemplified by practices of aggressive fire suppression, which extinguish 98% of all ignitions before they reach approximately 100 ha (250 acres) (Calkin et al. 2015). In most of western USA, fire exclusion became effective at large spatial scales after the Second World War as road and air transport, radio communications, and firefighting technology became more widespread. Annual area burned in forested lands of California and western USA began to decrease in the 1930s, reaching a low point between the 1960s and 1980s (McKelvey and Busse 1996; Littell et al. 2009; Keeley and Syphard 2021). However, fire regimes and fuels were also modified indirectly decades prior to the era of active fire exclusion through the effects of extensive timber logging, road building, agricultural expansion, and livestock grazing (in some locations), and by the severe reduction of Indigenous burning practices.
In forested ecosystems that previously supported frequent, low-severity fire regimes, fire suppression and exclusion caused dramatic changes in forest tree demography and physical structure (Safford and Stevens 2017; Bohlman et al. 2021; Hagmann et al. 2021; Bernal et al. 2022; Knight et al. 2022). Once the surface fires that regulated survivorship of seedlings and small trees had been eliminated, trees in these younger age classes became more abundant (North et al. 2016, 2022; Safford et al. 2021). As a result, stand density (trees/ha) has increased (Figs 4 and 5), leading to increased fuel mass and connectivity (increasing ladder fuels providing pathways for fire to spread from the surface into the forest canopy as well as denser, more connected canopies), and surface fuel loads increased, while spatial heterogeneity (patchiness) and species composition shifted from disturbance-adapted to shade-tolerant species (O’Connor et al. 2017; Safford and Stevens 2017; Bernal et al. 2022). The net result of these changes is the creation of landscape fuel complexes that favour high-intensity, spreading wildfires.
Comparison of the 1911 and 2011 relative stand densities (Stand Density Index – SDI%) from forest inventory data from pine-mixed conifer (Pine MC), xeric-mixed conifer (Xeric MC), and mesic-mixed conifer (Mesic MC) sites in the central and southern Sierra Nevada. The coloured envelopes around each plot are the proportion of the sites (dots) with a given SDI%. The mean SDI% values for each time period is indicated by a horizontal line. The data show the increase in mean and maximum stand density in all three forest types over the 100 years from 1911 to 2011. SDI (%) values over 60% suggest exceedingly high levels of competition and danger of imminent mortality. Source: North et al. (2022) – used by permission.
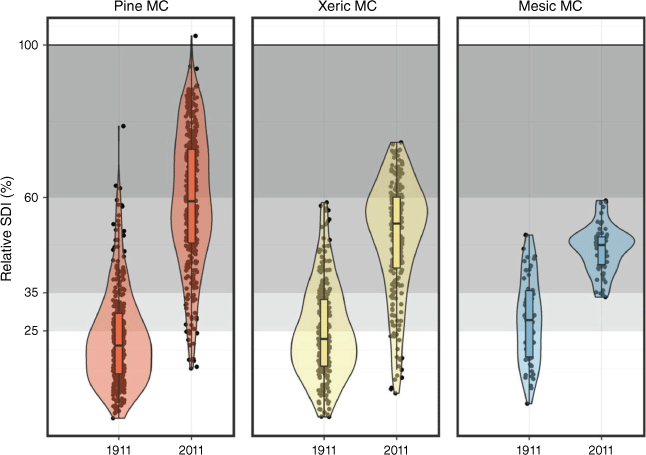
Increasing stand density of vegetative fuels in a Jeffrey pine–white fir stand within Lassen National Park over the period 1925–2008. The stand subsequently burned at high severity in 2012 during the Reading Fire. Source: https://www.psu.edu/news/research/story/fire-operations-prescribed-burning-combo-reduces-wildfire-severity-72/. Photo credit: A. E. Weislander, US Forest Service/Alan H. Taylor, Penn State. Used with permission from Alan H. Taylor.
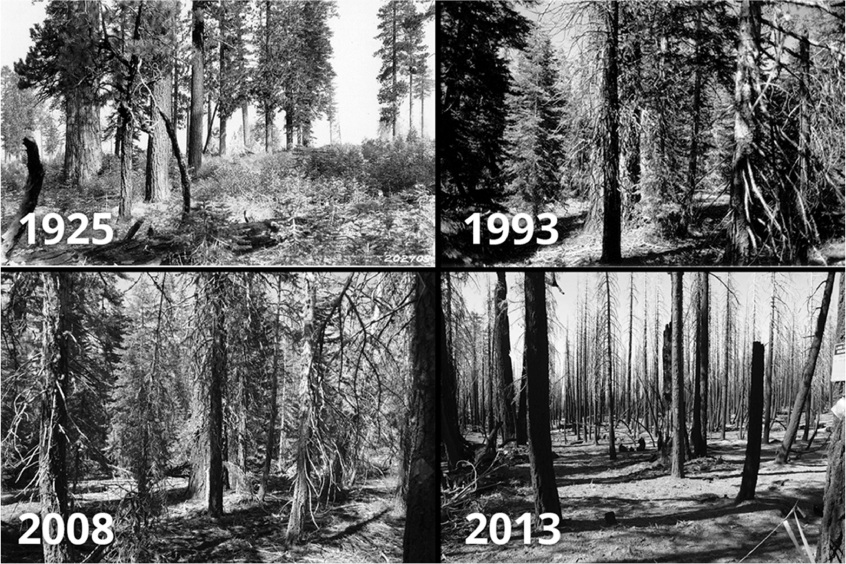
In combination with fire suppression, past timber harvesting practices have also had an impact on the fire risk of some California forests. US Forest Service lands in the Sierra Nevada experienced intensive logging commencing in the early 20th century. Large trees were particularly sought and harvested. This, coupled with fire suppression practices, led to a situation where dense stands of small trees, conducive to the development of crown fires, came to typify large areas (Stephens and Moghaddas 2005; Collins et al. 2011; Miller et al. 2012).
Fire exclusion has a different history in California lowland shrubland ecosystems, especially in coastal central and southern California. Here, lightning ignitions are relatively infrequent, and most burning is caused by human ignitions (Keeley 2002; Anderson and Keeley 2018). The establishment of the California mission system and colonisation of Indigenous peoples in the region led to the suppression of Indigenous burning and major landscape changes, including the transition of many grass-dominated lands to shrublands (Neely 2019). In the late 19th century, Euro–American burning replaced more purposeful Indigenous fire regimes, with accidental ignitions often occurring during the driest, hottest, and windiest periods of the year.
The short, dense canopy of shrubs and high aromatic oil content of leaves in many species leads naturally to intense, rapidly moving fires. Regeneration in chaparral and sage scrub is often fire-cued, and postfire recovery of biomass is rapid (Keeley and Keeley 1981; Bohlman et al. 2018). As a result, these ecosystems can support enough biomass to reburn only a few years after fire (Keeley and Safford 2016). The southern California fire season for shrublands is bimodal: the spring–summer season is characterised by many ignitions, maritime winds, higher fuel moistures, and often fuel-limited fire spread under conditions conducive to traditional fire control; the autumn–early winter ‘Santa Ana’ season is marked by fewer ignitions, critically low fuel moistures, and large fires driven by foehn winds from the interior that often result in high levels of economic loss, home destruction, and human fatalities (Safford et al. 2018). Most ignitions associated with the California coastal fire regime are human-caused, and according to Keeley (2009), fire suppression has been a critical factor in attenuating the high fire frequencies that might otherwise be expected due to human-caused ignitions.
It is hard to generalise the impacts of land management over the past 150 years because across ecosystems, the history of fire regimes has been highly variable in California (Safford et al. 2021). For example, interior chaparral, sagebrush, and pinyon–juniper ecosystems of the Great Basin likely burn today at higher frequencies than under pre-colonial conditions, yet the apparent driver is not more-frequent anthropogenic ignitions but rather the invasion of non-native annual grasses, such as cheatgrass (Bromus tectorum) (Brooks 1999). Higher-elevation forests have not experienced the same degree of fire suppression-driven ecosystem change as the lower-elevation drier forests, simply because their natural fire frequencies are much lower – although the incidence of fire is increasing in high-elevation forests in the Sierra Nevada (Schwartz et al. 2015). Wet coastal forests in northern California support a complex fire regime where lightning ignitions are relatively rare today, but Indigenous ignitions were locally frequent before settler–colonist arrival, leading to uncertainty among managers attempting to restore past, presumably more resilient conditions (Safford et al. 2021). Understanding the full scope of variability in recent fire regimes, its drivers, and potential adaptative responses are important areas of ongoing concern.
To summarise, historical land management over the past 150 years has affected wildfire in California in the following manner:
During the pre-colonisation period, the average annual area burned probably often equaled or exceeded the 1.6 million ha (4 million acre) mark reached in 2020.
The significant reduction of the use of fire by Indigenous peoples following colonisation, the introduction of grazing livestock (which reduced fine fuels and decreased surface fire activity), aggressive fire suppression in the 20th century, and early timber harvest practices (which removed large trees and increased surface fuels) resulted in stand densification by fire-intolerant species and increased fuel loading in some California forests, leading to increasingly severe fires in these ecosystems.
Fire regimes in California are highly ecosystem specific and are prone to differing human land-management influences. Shrublands in the WUI present a different history and challenges from remote coniferous forests, for example.
The need for continued research and targeted adaptive management and paradigm shifts relative to contemporary forest management and other fuel management and fire mitigation practices is critical.
What are the effects of land management and ignition sources on fire regimes since 1980?
Recent changes in fuel conditions related to current and recent management practices
Vegetative fuels (e.g. branches, needles, and other biomass materials) are important drivers of fire behaviour in forested ecosystems (Stephens and Moghaddas 2005). The primary tools for directly managing fuels are mechanical thinning, hand thinning, mastication, and controlled burning (or prescribed fire). Sequential application of these tools is a typical approach, particularly if the management goal is to reduce surface fuels rather than redistribute them (Stephens et al. 2012). Such sequential treatments are designed to disrupt the continuity of live fuels (shrubs, overstorey and small trees, and low branches) and reduce the potential for severe fire, facilitating the return of fire with less destructive behaviour (Agee and Skinner 2005; Ryan et al. 2013).
With the long-term decline in the timber industry, the closure of most of the sawmills across California has created a significant economic barrier to mechanical forest thinning efforts; the collapse in the originally robust bioenergy market has also been problematic in regards to thinning (McIver et al. 2015; Safford et al. 2021). Costs to carry out fuel reduction projects that do not have economic outlets are very high, sometimes exceeding US$5000/acre (US$12 500/ha). Mechanical fuel treatments in areas with the highest wildfire risk (e.g. high levels of biomass, low accessibility, rugged topography) tend to be the most expensive to implement and generate much less revenue than the cost of treatment (Hunter and Taylor 2022). However, the management of forests for both timber resources and fire is not without challenges that require continued research. A recent study of yellow pine and mixed-conifer forests in California found that fire severity on privately managed industrial forest sites was 1.8 times greater than on similar public forest lands (Levine et al. 2022). The authors concluded that current management approaches on the private timberlands may be contributing to the incidences of these high-severity fires. Barriers to specific prescribed fire treatments include operational and environmental constraints such as limited site access, effects on air quality, competing land management regulations, lack of financial and human resources, the existence of extreme conditions that could cause intentional fire to escape or become more severe than intended and, as a direct consequence, increased risk aversion (Williams et al. in press; Quinn-Davidson and Varner 2012; Schultz et al. 2019). These factors have constrained the ability of forest managers to apply prescribed fire with sufficient frequency and extent to mimic historical fire regimes and reduce the risk of high-intensity fire (Van de Water and Safford 2011; Safford and Van de Water 2014).
Patterns of ignition related to human activity
Human-caused ignition was associated with over 90% of recorded fires in most California counties over the period 1919–2016 (Keeley and Syphard 2018). These fires stem from many activities, including arson, debris burning, smoking, recreational activities, equipment operation, vehicles, and power infrastructure (Keeley and Syphard 2018; Short 2021). Human-caused ignitions are particularly dominant in lowland ecosystems west of the Sierra Nevada and along the coast, whereas lightning-caused ignitions are more common in higher-elevation mountain ranges and in desert ecosystems (Fusco et al. 2016; Keeley and Syphard 2018). Eight of the 20 largest wildfires recorded in California history are attributed or partially attributed to human activity (https://www.fire.ca.gov/media/4jandlhh/top20_acres.pdf). Nine have been at least partially attributed to lightning. Human-ignited fires are also responsible for at least 12 of the 20 most destructive fires in terms of structures and lives lost (https://34c031f8‐c9fd‐4018‐8c5a‐4159cdff6b0d‐cdn‐endpoint.azureedge.net/‐/media/calfire‐website/our‐impact/fire‐statistics/featured‐items/top20_destruction.pdf?rev=479d39ded1e248faac4114bdabf49416&hash=33D35B11518F3A681BA613D8C32073FC).
Recent research has shown that human-caused ignitions were responsible for all of the large wind-driven fires related to Santa Ana conditions in southern California (Keeley et al. 2021). Only about 25% of Santa Ana wind days co-occur with fires, and such events are dependent upon an ignition source. Thus, the number of human ignitions was a better predictor of area burned than the Santa Ana wind conditions associated with the time of the fire (Keeley et al. 2021). Human ignitions are most dominant near population centres and along transportation corridors (Chen and Jin 2022). Lightning ignitions are more common in wildlands in northern and northeastern California at higher elevations (Chen and Jin 2022). Although increases in the number of human-caused fires were correlated positively with population growth in California in the early to mid-20th century, the frequency of these wildfires from all human-related causes, except powerlines, has declined since 1980 (Syphard and Keeley 2015; Keeley and Syphard 2019). The decline in most human-caused ignitions in the past 40 years likely reflects a number of factors including increasing efficiency of fire prevention, fire-resistant infrastructure, declining rates of smoking, anti-arson measures, and wide public wildfire messaging and education (Keeley and Syphard 2019). Given these factors, this suggests that the trend of increasing area burned in California over the last four decades cannot simply be explained by increasing numbers of human-caused ignitions. However, human-caused ignitions remain a major contributor to the current wildfire regimes in California and western USA (Fusco et al. 2016; Balch et al. 2017; Keeley and Syphard 2018). Human-caused ignitions are clearly major contributors to the most socio-economically destructive fires (Downing et al. 2022), and due to their timing and location, human-caused ignitions can result in large and severe fires that are highly destructive (Hantson et al. 2022). The growth of the WUI puts humans, human-caused ignition events, and vegetative fuels in close proximity; it also increases human vulnerability to wildfire (Keeley and Syphard 2019). Human ignitions are a key concern in meeting California’s wildfire challenges.
Recent practices in fire management and suppression strategies
The importance of fire, including a mixture of severity levels, in the maintenance and restoration of many California ecosystems is widely recognised (Perry et al. 2011; Meyer 2015; DellaSala et al. 2017). Within some jurisdictions, such as Yosemite and Sequoia & Kings Canyon National Parks, and in adjacent wilderness areas managed by the U.S. Forest Service, resource managers have applied minimal suppression to naturally ignited fires when critical resources are deemed at lower risk of harm (Black et al. 2007). This practice, commonly referred to as ‘managed fire for resource benefit’ (alternative names include ‘prescribed natural fire’ or ‘wildland fire use’), has proven effective in reducing fuels, increasing forest resilience, and minimising the severity of subsequent wildfires (Meyer 2015; Stephens et al. 2021). Yet, five decades after federal agencies formally recognised the importance of fire as an ecological process, both managed wildfire and prescribed fire remain underutilised as management tools in western USA (Kolden 2019; Botti and Nichols 2021; Safford et al. 2022). Revised federal policy guidance enacted in 2009 has expanded opportunities to use wildfire for ecological benefit, allowing for wildfires to be managed for multiple objectives (e.g. full suppression, resource benefit) as they spread across a landscape (Young et al. 2020). This guidance has provided a pathway for returning the important ecological role of fire to many fire-adapted ecosystems. Management of wildfires with multiple objectives has played out within many burn area perimeters in California, including some of the largest wildfires in the state (Kane et al. 2019). Increasingly, the use of less-aggressive wildfire suppression strategies has become important for minimising the negative impacts of wildfire, especially in the context of the operational and legal constraints on mechanical fuel treatments (North et al. 2015a). The application of minimum suppression strategies can and will contribute to increases in annual area burned totals, but when applied in unpopulated areas can contribute to fuel reductions, ecological functioning, and habitat diversity, with little societal cost.
Although the 2009 policy guidance has served to decrease the use of aggressive fire suppression strategies, wildfire management practices in California generally have changed less than those in other western regions (Young et al. 2020), and minimum suppression policies can be suspended during large fire years (e.g. 2020, 2021). Under extreme fire conditions, aggressive suppression tactics that limit fire spread are likely to be reenacted, sometimes in combination with forest closures, to avoid protracted fire events that can strain firefighting resources and pose threats to public safety (Kane et al. 2019). The potential impacts of large and severe wildfires on built infrastructure also have amplified the pressure on federal agencies to institute a more aggressive policy of early fire suppression (Gabbert 2021). However, evidence is lacking for the efficacy of an intensified wildfire suppression strategy that limits opportunities of managing wildfire for resource benefit. For example, some argue that an aggressive approach that minimises fire spread ignores the root of the problem by not directly treating extensive fuel accumulation and stand density-driven tree mortality that could drive more severe megafires in the future (Moreira et al. 2020). Furthermore, most built infrastructure damaged by fire over the past 20 years is found in vegetated areas outside those where modifications in forest management practices could help reduce severe wildfire risk (Schwartz and Syphard 2021).
Over the last several decades our understanding of the role of fire in California ecosystems and the role of humans in driving fire regimes and fire behaviour has clearly improved. Humans influence fire in myriad ways, but our toolbox to manage fire is limited. Costs can be prohibitive, and sometimes competing regulations complicate applications of measures such as prescribed burns, which may be constrained by workforce capacity. In addition, most management tactics are often focused on small geographic areas and short time periods. As an outcome, permanent vegetation type conversions have (and will continue to) become more frequent as previous ecosystems lose resilience and are unable to recover (Coppoletta et al. 2016; Falk et al. 2022; Guiterman et al. 2022). The recent Venado Declaration (2021; https://www.documentcloud.org/documents/21100767-venado-declaration), spearheaded by former California governor Jerry Brown, addresses some of these challenges with suggestions of possible remedies. Yet, without strategic thinking, multi-partner cooperation, and economic investment, fire management tactics stand little chance of making a difference in terms of ecological benefits and risk reduction at adequate spatio-temporal scales. To further complicate finding a path forward, management approaches continue to generate intense debate, particularly in the public arena.
Fortunately, recent developments in California show reason for optimism. These include the uptick in tribal and non-tribal private interest in traditional prescribed and Indigenous cultural burning (Elassar 2022), the development of new National Forest plans that promote managed wildfire, the passage of the 2021 Bipartisan Infrastructure Law, which includes unprecedented investments for fuels management and firefighting resources, and a number of recent inter-agency and multi-partner agreements related to fire and vegetation management. For example, the 2021 California Wildfire and Forest Resilience Action Plan (https://wildfiretaskforce.org/wp‐content/uploads/2022/04/californiawildfireandforestresilienceactionplan.pdf) sets rigorous targets for vegetation management, fire use, and integration of Indigenous burning practices while also proposing avenues for funding and implementation.
In summary, we found the following trends relative to the combined effects of land management and ignition sources on fire regimes:
Since 1980, some fires in remote forested areas have been allowed to burn to support ecosystem health, effectively increasing the annual area burned in some regions, but also reducing accumulated fuels and promoting ecological services associated with wildfire.
Forest management and fire exclusion practices have changed markedly in recent decades and will continue to change. Some of the fuels and fire regime legacies of 19th and 20th century forest and fire management practices will diminish as the 21st century progresses, particularly as vegetation type conversion (e.g. forest to shrubland) occurs across greater extents. Challenges are present in determining best practices in both the timber and conservation management of forests to address wildfire.
Non-forested landscapes such as chaparral and shrublands are prone to fire, but are often not as amenable to the same management practices (e.g. fuels reduction) as most forest types.
Although the human population of California grew considerably from 1980 to present, there has not been a concomitant increase in the number and overall proportion of human-caused ignitions, except those attributable to powerlines. Only eight of the largest wildfires in the state are wholly or partially attributable to human activity.
Humans remain the most prevalent source of wildfire ignitions in California, particularly in lowland and coastal ecosystems. Human ignitions also play an outsized role in the occurrence of infrastructure-destroying fires. The growth of the WUI increases the risks of ignition, and ignitions in areas likely to impact people most seriously.
Recent progress includes the increasing attempts to integrate lessons from Indigenous cultural burning, development of new National Forest plans that promote management for wildfire, ecosystem resilience and services, recent inter-agency and multi-partner agreements related to fire and vegetation management, and the holistic nature of the 2021 California Wildfire and Forest Resilience Action Plan.
Conclusion: the future of wildfire in California
California’s 21st-century wildfire regimes reflect a confluence of intersecting factors, including climate change, past and present forest management and land use, and the role of human- and lightning-caused ignitions. Past management practices such as fire exclusion and the loss of Indigenous burning will continue to exacerbate the sensitivity of wildfires to climate warming trends. Going forward, it will be important to recognise the diversity within California’s ecosystem types and land uses in the selection, prioritisation, and implementation of fuel and fire management strategies across differing vegetation types (e.g. forest versus shrubland) and across the continuum of urban to less densely developed and populated landscapes. People and infrastructure in many regions across California, particularly in the expanding WUI, will remain vulnerable to high-severity fire under all but the most robust local fuel reduction, defensible space, and fire-hardened building requirements given California’s natural propensity for wildfire, the ongoing accumulation of fuels in some ecosystems due to fire exclusion, and the expectation that temperatures will climb and low-VPD episodes will continue to increase.
In 2009, the U.S. Federal Government developed the National Wildland Fire Cohesive Strategy, followed by a National Action Plan and updated version of the Strategy in 2014. Most recently, the federal response is to incorporate not only greenhouse gas mitigation, but climate adaptation actions that take into account the regional and local differences in ecosystems and human land use. California’s response to its wildfire challenges is complementary to the federal response, especially by providing a solutions-oriented response framework. Indigenous knowledge and management practices are and will continue to be important components of this strategy as it continues to be developed (Long et al. 2021).
Although today we face a particularly challenging confluence of factors driving wildfire, it must also be accepted that wildfires are a long-established, inherent, and inescapable element of California’s and western North America’s natural environment that we need to understand and accept (Moreira et al. 2020; Safford et al. 2022). Contemporary wildfire challenges are very likely to increase over the 21st century, requiring the ongoing evolution of adaptive approaches for ecosystems and developed areas (Schoennagel et al. 2017). This also applies to the necessity of further developing and applying fire-wise building regulations and land-use planning to help buffer human development to minimise loss of human life and structures. California’s Wildfire and Forest Resilience Action Plan (2021) offers a recent example of developing responses to these challenges, with dynamic, multi-pronged approaches based on holistic perspectives. By leveraging the state's innovative human capital and appreciable financial resources, California also has potential to serve as a wildfire resiliency model for diverse wildfire-prone regions across North America and around the globe.
Data availability
All data utilized in this manuscript are fully available via the website links cited in the reference list.
Conflicts of interest
The authors declare no conflicts of interest. Dr. Timothy Brown is an Associate Editor of International Journal of Wildland Fire. To mitigate this potential conflict of interest he was blinded from the peer review process and was not involved at any stage in the editing of this manuscript.
Declaration of funding
This publication was supported by Grant #G18AC00320 from the U.S. Geological Survey (USGS) Southwest Climate Adaptation Science Center. Its contents are solely the responsibility of the authors and do not necessarily represent the views of the Southwest Climate Adaptation Science Center or the USGS.
Acknowledgements
We acknowledge the diverse authorship team of scientists affiliated with the Southwest Climate Adaptation Science Center (SW CASC) for contributing their time and expertise in the development of this synthesis and assessment of the recent scientific literature. We also are grateful to Jon Keeley, Margaret Evans, Stephen Jackson, Malcolm North, and Connie Woodhouse for generously providing their insight and useful comments on an earlier draft of this manuscript.
References
Abatzoglou JT, Kolden CA (2013) Relationships between climate and macroscale area burned in the western United States. International Journal of Wildland Fire 22, 1003-1020.
| Crossref | Google Scholar |
Abatzoglou JT, Williams AP (2016) Impact of anthropogenic climate change on wildfire across western US forests. Proceedings of the National Academy of Sciences 113, 11770-11775.
| Crossref | Google Scholar |
Adams HD, Barron-Gafford GA, Minor RL, Gardea AA, Bentley LP, Law DJ, Breshears DD, McDowell NG, Huxman TE (2017) Temperature response surfaces for mortality risk of tree species with future drought. Environmental Research Letters 12, 115014.
| Crossref | Google Scholar |
Adetona O, Reinhardt TE, Domitrovich J, Broyles G, Adetona AM, Kleinman MT, Ottmar RD, Naeher LP (2016) Review of the health effects of wildland fire smoke on wildland firefighters and the public. Inhalation Toxicology 28, 95-139.
| Crossref | Google Scholar |
Adlam C, Almendariz D, Goode RW, Martinez DJ, Middleton BR (2021) Keepers of the flame: supporting the revitalization of Indigenous cultural burning. Society & Natural Resources 35, 575-590.
| Crossref | Google Scholar |
Agee JK, Skinner CN (2005) Basic principles of forest fuel reduction treatments. Forest Ecology and Management 211, 83-96.
| Crossref | Google Scholar |
Aguilera R, Corringham T, Gershunov A, Benmarhnia T (2021) Wildfire smoke impacts respiratory health more than fine particles from other sources: observational evidence from Southern California. Nature Communications 12, 1493.
| Crossref | Google Scholar |
Aldern JD, Goode RW (2014) The stories hold water: learning and burning in North Fork Mono homelands. Decolonization: Indigeneity, Education, and Society 3(3), 26-51.
| Google Scholar |
Alizadeh MR, Abatzoglou JT, Luce CH, Adamowski JF, Farid A, Sadegh M (2021) Warming enabled upslope advance in western US forest fires. Proceedings of the National Academy of Sciences 118(22), e2009717118.
| Crossref | Google Scholar |
Anderson MK (1994) Prehistoric anthropogenic wildland burning by hunter-gatherer societies in the temperate regions: a net source, sink, or neutral to the global carbon budget? Chemosphere 29, 913-934.
| Crossref | Google Scholar |
Anderson MK (1996) The ethnobotany of deergrass, Muhlenbergia rigens (Poaceae): its uses and fire management by California Indian tribes. Economic Botany 50(4), 409-22.
| Crossref | Google Scholar |
Balch JK, Bradley BA, Abatzoglou JT, Nagy RC, Fusco EJ, Mahood AL (2017) Human-started wildfires expand the fire niche across the United States. Proceedings of the National Academy of Sciences 114, 2946-2951.
| Crossref | Google Scholar |
Beaty RM, Taylor AH (2001) Spatial and temporal variation of fire regimes in a mixed conifer forest landscape, Southern Cascades, California, USA. Journal of Biogeography 28, 955-966.
| Crossref | Google Scholar |
Beaty RM, Taylor AH (2008) Fire history and the structure and dynamics of a mixed conifer forest landscape in the northern Sierra Nevada, Lake Tahoe Basin, California, USA. Forest Ecology and Management 255, 707-719.
| Crossref | Google Scholar |
Belmecheri S, Babst F, Wahl ER, Stahle DW, Trouet V (2016) Multi-century evaluation of Sierra Nevada snowpack. Nature Climate Change 6(1), 2-3.
| Crossref | Google Scholar |
Berg N, Hall A (2015) Increased interannual precipitation extremes over California under climate change. Journal of Climate 28, 6324-6334.
| Crossref | Google Scholar |
Bernal AA, Stephens SL, Collins BM, Battles JJ (2022) Biomass stocks in California’s fire-prone forests: mismatch in ecology and policy. Environmental Research Letters 17(4), 044047.
| Crossref | Google Scholar |
Black A, Williamson M, Doane D (2007) Wildland fire use barriers and facilitators. Fire Management Today 68, 10-14.
| Google Scholar |
Bohlman GN, Underwood EC, Safford HD (2018) Estimating biomass in California’s chaparral and coastal sage scrub shrublands. Madroño 65, 28-46.
| Crossref | Google Scholar |
Bonfils C, Santer BD, Pierce DW, Hidalgo HG, Bala G, Das T, Barnett TP, Cayan DR, Doutriaux C, Wood AW, Mirin A, Nozawa T (2008) Detection and attribution of temperature changes in the mountainous western United States. Journal of Climate 21, 6404-6424.
| Crossref | Google Scholar |
Botti S, Nichols T (2021) National Park Service fire restoration, policies versus results: what went wrong. Parks Stewardship Forum 37, 353-367.
| Crossref | Google Scholar |
Bradford JB, Schlaepfer DR, Lauenroth WK, Palmquist KA (2020) Robust ecological drought projections for drylands in the 21st century. Global Change Biology 26, 3906-3919.
| Crossref | Google Scholar |
Brooks ML (1999) Alien annual grasses and fire in the Mojave Desert. Madroño 46(1), 13-19.
| Google Scholar |
Brown PM, Swetnam TW (1994) A cross-dated fire history from coast redwood near Redwood National Park, California. Canadian Journal of Forest Research 24, 21-31.
| Crossref | Google Scholar |
CAL FIRE (2021) Fire statistics. Available at https://www.fire.ca.gov/stats-events/ [verified 6 September 2021]
California’s Wildfire and Forest Resilience Action Plan: Recommendations of the Governor's Forest Management Task Force (2021) Available at https://wildfiretaskforce.org/wp-content/uploads/2022/04/californiawildfireandforestresilienceactionplan.pdf
Calkin DE, Thompson MP, Finney MA (2015) Negative consequences of positive feedbacks in US wildfire management. Forest Ecosystems 2(1), 9.
| Crossref | Google Scholar |
Cayan DR, DeHaan LL, Gershunov A, Guzman-Morales J, Keeley JE, Mumford J, Syphard AD (2022) Autumn precipitation: the competition with Santa Ana winds in determining fire outcomes in southern California. International Journal of Wildland Fire 31(11), 1056-1067.
| Crossref | Google Scholar |
Chen B, Jin Y (2022) Spatial patterns and drivers for wildfire ignitions in California. Environmental Research Letters 17, 055004.
| Crossref | Google Scholar |
Chen B, Jin Y, Scaduto E, Moritz MA, Goulden ML, Randerson JT (2021) Climate, fuel, and land use shaped the spatial pattern of wildfire in California’s Sierra Nevada. Journal of Geophysical Research: Biogeosciences 126(2), e2020JG005786.
| Crossref | Google Scholar |
Collins BM, Everett RG, Stephens SL (2011) Impacts of fire exclusion and recent managed fire on forest structure in old growth Sierra Nevada mixed‐conifer forests. Ecosphere 2, art51.
| Crossref | Google Scholar |
Coppoletta M, Merriam KE, Collins BM (2016) Post-fire vegetation and fuel development influences fire severity patterns in reburns. Ecological Applications 26(3), 686-699.
| Crossref | Google Scholar |
Cowan J (2020) Alarmed by scope of wildfires, Officials Turn to Native Americans for Help. The New York Times, 7 October 2020. Available at https://www.nytimes.com/2020/10/07/us/native-american-burning-practices-california.html [accessed 24 August 2021]
Crausbay SD, Ramirez AR, Carter SL, Cross MS, Hall KR, Bathke DJ, Betancourt JL, Colt S, Cravens AE, Dalton MS, Dunham JB, Hay LE, Hayes MJ, McEvoy J, McNutt CA, Moritz MA, Nislow KH, Raheem N, Sanford T (2017) Defining ecological drought for the twenty-first century. Bulletin of the American Meteorological Society 98, 2543-2550.
| Crossref | Google Scholar |
Crockett JL, Westerling AL (2018) Greater temperature and precipitation extremes intensify Western US droughts, wildfire severity, and Sierra Nevada tree mortality. Journal of Climate 31, 341-354.
| Crossref | Google Scholar |
David AT, Asarian JE, Lake FK (2018) Wildfire smoke cools summer river and stream water temperatures. Water Resources Research 54, 7273-7290.
| Crossref | Google Scholar |
de Boer HJ, Robertson I, Clisby R, Loader NJ, Gagen M, Young GHF, Wagner-Cremer F, Hipkin CR, McCarroll D (2019) Tree-ring isotopes suggest atmospheric drying limits temperature–growth responses of treeline bristlecone pine. Tree Physiology 39, 983-999.
| Crossref | Google Scholar |
DellaSala DA, Hutto RL, Hanson CT, Bond ML, Ingalsbee T, Odion D, Baker WL (2017) Accommodating mixed-severity fire to restore and maintain ecosystem integrity with a focus on the Sierra Nevada of California, USA. Fire Ecology 13(2), 148-171.
| Crossref | Google Scholar |
Dennison PE, Brewer SC, Arnold JD, Moritz MA (2014) Large wildfire trends in the western United States, 1984–2011. Geophysical Research Letters 41, 2928-2933.
| Crossref | Google Scholar |
Diffenbaugh NS, Swain DL, Touma D (2015) Anthropogenic warming has increased drought risk in California. Proceedings of the National Academy of Sciences 112, 3931-3936.
| Crossref | Google Scholar |
Dong C, Williams AP, Abatzoglou JT, Lin K, Okin GS, Gillespie TW, Long D, Lin Y-H, Hall A, MacDonald GM (2022) The season for large fires in Southern California is projected to lengthen in a changing climate. Communications Earth & Environment 3, 22.
| Crossref | Google Scholar |
Downing WM, Dunn CJ, Thompson MP, Caggiano MD, Short KC (2022) Human ignitions on private lands drive USFS cross-boundary wildfire transmission and community impacts in the western US. Scientific Reports 12, 2624.
| Crossref | Google Scholar |
Elassar A (2022) California once prohibited Native American fire practices. Now, it’s asking tribes to use them to help prevent wildfires. CNN. 3 April 2022. Available at https://www.cnn.com/2022/04/03/us/california-native-american-fire-practitioners-wildfires-climate/index.html [accessed 27 April 2022]
Everett RG (2008) Dendrochronology-based fire history of mixed-conifer forests in the San Jacinto Mountains, California. Forest Ecology and Management 256, 1805-1814.
| Crossref | Google Scholar |
Falk DA, Heyerdahl EK, Brown PM, Farris C, Fulé PZ, McKenzie D, Swetnam TW, Taylor AH, Van Horne ML (2011) Multi-scale controls of historical forest-fire regimes: new insights from fire-scar networks. Frontiers in Ecology and the Environment 9, 446-454.
| Crossref | Google Scholar |
Falk DA, van Mantgem PJ, Keeley JE, Gregg RM, Tepley AJ, Young DJ, Guiterman CH, Marshall LA (2022) Tamm review: mechanisms of forest resilience. Forest Ecology & Management 515, 120129.
| Google Scholar |
Flannigan MD, Wotton BM, Marshall GA, De Groot WJ, Johnston J, Jurko N, Cantin AS (2016) Fuel moisture sensitivity to temperature and precipitation: climate change implications. Climatic Change 134, 59-71.
| Crossref | Google Scholar |
Fry DL, Stephens SL (2006) Influence of humans and climate on the fire history of a ponderosa pine-mixed conifer forest in the southeastern Klamath Mountains, California. Forest Ecology and Management 223, 428-438.
| Crossref | Google Scholar |
Fusco EJ, Abatzoglou JT, Balch JK, Finn JT, Bradley BA (2016) Quantifying the human influence on fire ignition across the western USA. Ecological Applications 26, 2390-2401.
| Crossref | Google Scholar |
Gabbert B (2021) Forest Service Chief says wildfires will be suppressed, rather than “managed”, for now. Wildfire Today, 3 August 2021. Available at https://wildfiretoday.com/2021/08/03/forest-service-chief-says-wildfires-will-be-suppressed-rather-than-managed-for-now/ [accessed 17 November 2021]
Gergel DR, Nijssen B, Abatzoglou JT, Lettenmaier DP, Stumbaugh MR (2017) Effects of climate change on snowpack and fire potential in the western USA. Climatic Change 141, 287-299.
| Crossref | Google Scholar |
Gershunov A, Guzman Morales J, Hatchett B, Guirguis K, Aguilera R, Shulgina T, Abatzoglou JT, Cayan D, Pierce D, Williams P, Small I, Clemesha R, Schwarz L, Benmarhnia T, Tardy A (2021) Hot and cold flavors of southern California’s Santa Ana winds: their causes, trends, and links with wildfire. Climate Dynamics 57(7-8), 2233-2248.
| Crossref | Google Scholar |
Goode R, Gaughen S, Fiero M, Hankins D, Johnson-Reyes K, Middleton BR, Red Owl T, Yonemura R (2018) ‘Summary Report from Tribal and Indigenous Communities within California’. California’s Fourth Climate Change Assessment. Publication number: SUM-CCCA4-2018-010. (California Governor’s Office of Planning and Research) Available at https://www.energy.ca.gov/sites/default/files/2019-11/Statewide_Reports-SUM-CCCA4-2018-010_TribalCommunitySummary_ADA.pdf
Goss M, Swain DL, Abatzoglou JT, Sarhadi A, Kolden CA, Williams AP, Diffenbaugh NS (2020) Climate change is increasing the likelihood of extreme autumn wildfire conditions across California. Environmental Research Letters 15, 094016.
| Crossref | Google Scholar |
Griffin D, Anchukaitis KJ (2014) How unusual is the 2012–2014 California drought? Geophysical Research Letters 41, 9017-9023.
| Crossref | Google Scholar |
Guiterman CH, Gregg RM, Marshall LAE, Beckmann JJ, van Mantgem PJ, Falk DA, Keeley JE, Caprio AC, Coop JD, Fornwalt PJ, Haffey C, Hagmann RK, Jackson ST, Lynch AM, Margolis EQ, Marks C, Meyer MD, Safford H, Syphard AD, Taylor A, Wilcox C, Carril D, Enquist CAF, Huffman D, Iniguez J, Molinari NA, Restaino C, Stevens JT (2022) Vegetation type conversion in the US Southwest: frontline observations and management responses. Fire Ecology 18, 6.
| Crossref | Google Scholar |
Gumz J (2020) Amah Mutsun: When Fire Was a Tool. Times Publishing Group Online Daily, 4 December 2020. Available at https://tpgonlinedaily.com/amah-mutsun-when-fire-was-a-tool/ [accessed 24 August 2021]
Guzman-Morales J, Gershunov A (2019) Climate change suppresses Santa Ana Winds of Southern California and sharpens their seasonality. Geophysical Research Letters 46(5), 2772-2780.
| Crossref | Google Scholar |
Guzman-Morales J, Gershunov A, Theiss J, Li H, Cayan D (2016) Santa Ana Winds of southern California: their climatology, extremes, and behavior spanning six and a half decades. Geophysical Research Letters 43, 2827-2834.
| Crossref | Google Scholar |
Hagemann H (2020) Amah Mutsun Band Reignites Cultural Burning. Santa Cruz Sentinel, 25 November 2020. Available at https://www.santacruzsentinel.com/2020/11/25/amah-mutsun-tribal-band-reignites-cultural-burning/ [accessed 24 August 2021]
Hagmann RK, Hessburg PF, Prichard SJ, Povak NA, Brown PM, Fulé PZ, Keane RE, Knapp EE, Lydersen JM, Metlen KL, Reilly MJ, Sánchez Meador AJ, Stephens SL, Stevens JT, Taylor AH, Yocom LL, Battaglia MA, Churchill DJ, Daniels LD, Falk DA, Henson P, Johnston JD, Krawchuk MA, Levine CR, Meigs GW, Merschel AG, North MP, Safford HD, Swetnam TW, Waltz AEM (2021) Evidence for widespread changes in the structure, composition, and fire regimes of Western North American forests. Ecological Applications 31(8), e02431.
| Crossref | Google Scholar |
Hammer RB, Radeloff VC, Fried JS, Stewart SI (2007) Wildland–urban interface housing growth during the 1990s in California, Oregon, and Washington. International Journal of Wildland Fire 16(3), 255-265.
| Crossref | Google Scholar |
Hankins D (2021) Reading the landscape for fire. Bay Nature, 3 January 2021. Available at https://baynature.org/article/reading-the-landscape-for-fire/ [accessed 24 August 2021]
Hantson S, Andela N, Goulden ML, Randerson JT (2022) Human-ignited fires result in more extreme fire behavior and ecosystem impacts. Nature Communications 13, 2717.
| Crossref | Google Scholar |
Harley FW (1918) Letter to Rider re: Klamath Fires, Klamath National Forest. Available at https://www.karuk.us/images/docs/dnr/FWHarley_USFSRanger_1918_OrleansRD.pdf
Harling W (2015) Learning together burning together. Wildland Fire 24, 26-30.
| Google Scholar |
Hartter J, Hamilton LC, Ducey MJ, Boag AE, Salerno JD, Christoffersen ND, Oester PT, Palace MW, Stevens FR (2020) Finding common ground: agreement on increasing wildfire risk crosses political lines. Environmental Research Letters 15(6), 065002.
| Crossref | Google Scholar |
Hatchett BJ, Koshkin AL, Guirguis K, Rittger K, Nolin AW, Heggli A, Rhoades AM, East AE, Siirila‐Woodburn ER, Brandt WT, Gershunov A, Haleakala K (2023) Midwinter dry spells amplify post‐fire snowpack decline. Geophysical Research Letters 50(3),.
| Crossref | Google Scholar |
Heyerdahl EK, Morgan P, Riser JP (2008) Multi‐season climate synchronized historical fires in dry forests (1650–1900), northern Rockies, USA. Ecology 89, 705-716.
| Crossref | Google Scholar |
Heyerdahl EK, Loehman RA, Falk DA (2019) A multi-century history of fire regimes along a transect of mixed-conifer forests in central Oregon, USA. Canadian Journal of Forest Research 49, 76-86.
| Crossref | Google Scholar |
Holden ZA, Swanson A, Luce CH, Jolly WM, Maneta M, Oyler JW, Warren DA, Parsons R, Affleck D (2018) Decreasing fire season precipitation increased recent western US forest wildfire activity. Proceedings of the National Academy of Sciences 115(36), E8349-E8357.
| Crossref | Google Scholar |
Hua L, Shao G (2017) The progress of operational forest fire monitoring with infrared remote sensing. Journal of Forestry Research 28(2), 215-229.
| Crossref | Google Scholar |
Hunter ME, Taylor MH (2022) The economic value of fuel treatments: a review of the recent literature for fuel treatment planning. Forests 13, 2042.
| Crossref | Google Scholar |
Huntsinger L, McCaffrey S (1995) A forest for the trees: forest management and the Yurok environment, 1850 to 1994. American Indian Culture and Research Journal 19, 155-192.
| Crossref | Google Scholar |
Johanson CM, Fu Q (2009) Hadley Cell widening: model simulations versus observations. Journal of Climate 22, 2713-2725.
| Crossref | Google Scholar |
Jolly WM, Cochrane MA, Freeborn PH, Holden ZA, Brown TJ, Williamson GJ, Bowman DMJS (2015) Climate-induced variations in global wildfire danger from 1979 to 2013. Nature Communications 6, 7537.
| Crossref | Google Scholar |
Kane VR, Bartl-Geller BN, North MP, Kane JT, Lydersen JM, Jeronimo SMA, Collins BM, Monika Moskal L (2019) First-entry wildfire can create opening and tree clump patterns characteristic of resilient forests. Forest Ecology and Management 454, 117659.
| Crossref | Google Scholar |
Kasperson R (2014) Four questions for risk communication. Journal of Risk Research 17(10), 1233-1239.
| Crossref | Google Scholar |
Keeley JE (2002) Native American impacts on fire regimes of the California coastal ranges. Journal of Biogeography 29(3), 303-320.
| Crossref | Google Scholar |
Keeley JE, Keeley SC (1981) Post‐fire regeneration of southern California chaparral. American Journal of Botany 68(4), 524-30.
| Crossref | Google Scholar |
Keeley JE, Syphard AD (2018) Historical patterns of wildfire ignition sources in California ecosystems. International Journal of Wildland Fire 27, 781-799.
| Crossref | Google Scholar |
Keeley JE, Syphard AD (2019) Twenty-first century California, USA, wildfires: fuel-dominated vs. wind-dominated fires. Fire Ecology 15, 24.
| Crossref | Google Scholar |
Keeley JE, Syphard AD (2021) Large California wildfires: 2020 fires in historical context. Fire Ecology 17, 22.
| Crossref | Google Scholar |
Keeley JE, van Mantgem P, Falk DA (2019) Fire, climate and changing forests. Nature Plants 5(8), 774-775.
| Crossref | Google Scholar |
Keeley JE, Guzman-Morales J, Gershunov A, Syphard AD, Cayan D, Pierce DW, Flannigan M, Brown TJ (2021) Ignitions explain more than temperature or precipitation in driving Santa Ana wind fires. Science Advances 7(30), eabh2262.
| Crossref | Google Scholar |
Kerlin K (2020) Rethinking wildfire cultural burning and the art of not fighting fire. UC Davis News. 1 October 2020. Available at https://www.ucdavis.edu/climate/news/rethinking-wildfire [accessed 2 May 2021]
Kimmerer RW, Lake FK (2001) The role of Indigenous burning in land management. Journal of Forestry 99, 36-41.
| Crossref | Google Scholar |
Kitzberger T, Falk DA, Westerling AL, Swetnam TW (2017) Direct and indirect climate controls predict heterogeneous early-mid 21st century wildfire burned area across western and boreal North America. PLoS One 12, e0188486.
| Crossref | Google Scholar |
Knight CA, Anderson L, Bunting MJ, Champagne M, Clayburn RM, Crawford JN, Klimaszewski-Patterson A, Knapp EE, Lake FK, Mensing SA, Wahl D, Wanket J, Watts-Tobi A, Potts MD, Battles JJ (2022) Land management explains major trends in forest structure and composition over the last millennium in California’s Klamath Mountains. Proceedings of the National Academy of Sciences 119, e2116264119.
| Crossref | Google Scholar |
Kolden CA (2019) We’re not doing enough prescribed fire in the western United States to mitigate wildfire risk. Fire 22, 30.
| Crossref | Google Scholar |
Lake FK (2013) Historical and cultural fires, tribal management and research issues in Northern California: trails, fires and tribulations. Occasion: Interdisciplinary Studies in the Humanities 5, 1-22.
| Google Scholar |
Landrum L, Otto-Bliesner BL, Wahl ER, Conley A, Lawrence PJ, Rosenbloom N, Teng H (2013) Last millennium climate and its variability in CCSM4. Journal of Climate 26, 1085-1111.
| Crossref | Google Scholar |
LAO (Legislative Analyst’s Office) (2018) The 2018-19 Budget: Fire Recovery Proposals. Available at https://lao.ca.gov/Publications/Report/3767
Lepley K, Touchan R, Meko D, Shamir E, Graham R, Falk D (2020) A multi-century Sierra Nevada snowpack reconstruction modeled using upper-elevation coniferous tree rings (California, USA). The Holocene 30, 1266-1278.
| Crossref | Google Scholar |
Leverkus AB, Thorn S, Lindenmayer DB, Pausas JG (2020) Wildfire debate needs science, not politics. Science 370, 416-417.
| Crossref | Google Scholar |
Levine JI, Collins BM, Steel ZL, de Valpine P, Stephens SL (2022) Higher incidence of high-severity fire in and near industrially managed forests. Frontiers in Ecology and the Environment 20(7), 397-404.
| Crossref | Google Scholar |
Lightfoot KG, Cuthrell RQ (2015) Anthropogenic burning and the Anthropocene in late-Holocene California. The Holocene 25, 1581-1587.
| Crossref | Google Scholar |
Lightfoot KG, Cuthrell RQ, Boone CM, Byrne R, Chavez AS, Collins L, Cowart A, Evett RR, Fine PVA, Gifford-Gonzalez D, Hylkema MG, Lopez V, Misiewicz TM, Reid REB (2013) Anthropogenic burning on the Central California Coast in Late Holocene and early historical times: findings, implications, and future directions. California Archaeology 5, 371-390.
| Crossref | Google Scholar |
Littell JS, McKenzie D, Peterson DL, Westerling AL (2009) Climate and wildfire area burned in western U.S. ecoprovinces, 1916–2003. Ecological Applications 19, 1003-1021.
| Crossref | Google Scholar |
Littell JS, Peterson DL, Riley KL, Liu Y, Luce CH (2016) A review of the relationships between drought and forest fire in the United States. Global Change Biology 22, 2353-2369.
| Crossref | Google Scholar |
Liu Y (2016) Responses of dead forest fuel moisture to climate change. Ecohydrology 10(2), e1760.
| Crossref | Google Scholar |
Ljungqvist FC, Krusic PJ, Brattström G, Sundqvist HS (2012) Northern Hemisphere temperature patterns in the last 12 centuries. Climate of the Past 8(1), 227-249.
| Crossref | Google Scholar |
Loisel J, MacDonald GM, Thomson MJ (2017) Little Ice Age climatic erraticism as an analogue for future enhanced hydroclimatic variability across the American Southwest. PLoS One 12(10), e0186282.
| Crossref | Google Scholar |
Long JW, Lake FK, Goode RW (2021) The Importance of Indigenous cultural burning in forested regions of the Pacific West. Forest Ecology and Management 500, 119597.
| Crossref | Google Scholar |
Loomis, E (2017) ‘Forests and logging in the United States.’ (Oxford Research Encyclopedia of American History) 10.1093/acrefore/9780199329175.013.188
Lorimer CG, Porter DJ, Madej MA, Stuart JD, Veirs Jr SD, Norman SP, O’Hara KL, Libby WJ (2009) Presettlement and modern disturbance regimes in coast redwood forests: implications for the conservation of old-growth stands. Forest Ecology and Management 258, 1038-1054.
| Crossref | Google Scholar |
Luković J, Chiang JCH, Blagojević D, Sekulić A (2021) A later onset of the rainy season in California. Geophysical Research Letters 48, e2020GL090350.
| Crossref | Google Scholar |
Ma W, Zhai L, Pivovaroff A, Shuman J, Buotte P, Ding J, Christoffersen B, Knox R, Moritz M, Fisher RA, Koven CD, Kueppers L, Xu C (2021) Assessing climate change impacts on live fuel moisture and wildfire risk using a hydrodynamic vegetation model. Biogeosciences 18(13), 4005-4020.
| Crossref | Google Scholar |
Margolis EQ, Swetnam TW, Allen CD (2011) Historical stand-replacing fire in upper montane forests of the Madrean Sky Islands and Mogollon Plateau, Southwestern USA. Fire Ecology 7, 88-107.
| Crossref | Google Scholar |
Margolis EQ, Guiterman CH, Chavardès RD, Coop JD, Copes‐Gerbitz K, Dawe DA, Falk DA, Johnston JD, Larson E, Li H, Marschall JM, Naficy CE, Naito AT, Parisien M, Parks SA, Portier J, Poulos HM, Robertson KM, Speer JH, Stambaugh M, Swetnam TW, Tepley AJ, Thapa I, Allen CD, Bergeron Y, Daniels LD, Fulé PZ, Gervais D, Girardin MP, Harley GL, Harvey JE, Hoffman KM, Huffman JM, Hurteau MD, Johnson LB, Lafon CW, Lopez MK, Maxwell RS, Meunier J, North M, Rother MT, Schmidt MR, Sherriff RL, Stachowiak LA, Taylor A, Taylor EJ, Trouet V, Villarreal ML, Yocom LL, Arabas KB, Arizpe AH, Arseneault D, Tarancón AA, Baisan C, Bigio E, Biondi F, Cahalan GD, Caprio A, Cerano‐Paredes J, Collins BM, Dey DC, Drobyshev I, Farris C, Fenwick MA, Flatley W, Floyd ML, Gedalof Z, Holz A, Howard LF, Huffman DW, Iniguez J, Kipfmueller KF, Kitchen SG, Lombardo K, McKenzie D, Merschel AG, Metlen KL, Minor J, O'Connor CD, Platt L, Platt WJ, Saladyga T, Stan AB, Stephens S, Sutheimer C, Touchan R, Weisberg PJ (2022) The North American tree‐ring fire‐scar network. Ecosphere 13(7),.
| Crossref | Google Scholar |
Marks-Block T, Lake FK, Curran LM (2019) Effects of understory fire management treatments on California hazelnut, an ecocultural resource of the Karuk and Yurok Indians in the Pacific Northwest. Forest Ecology and Management 450, 117517.
| Crossref | Google Scholar |
McDowell NG, Sapes G, Pivovaroff A, et al. (2022) Mechanisms of woody-plant mortality under rising drought, CO2 and vapour pressure deficit. Nature Reviews Earth & Environment 3, 294-308.
| Crossref | Google Scholar |
McEvoy DJ, Pierce DW, Kalansky JF, Cayan DR, Abatzoglou JT (2020) Projected changes in reference evapotranspiration in California and Nevada: implications for drought and wildland fire danger. Earth’s Future 8(11), e2020EF001736.
| Crossref | Google Scholar |
McIntyre PJ, Thorne JH, Dolanc CR, Flint AL, Flint LE, Kelly M, Ackerly DD (2015) Twentieth-century shifts in forest structure in California: denser forests, smaller trees, and increased dominance of oaks. Proceedings of the National Academy of Sciences 112, 1458-1463.
| Crossref | Google Scholar |
McKelvey KS, Busse KK (1996) Twentieth-century fire patterns on forest service lands. In ‘Sierra Nevada Ecosystem Project, Final Report to Congress, Vol. II, Assessments and Scientific Basis for Management Options’. Report No. 37. pp. 1119–1138. (University of California, Centers for Water and Wildland Resources: Davis, CA, USA)
McKenzie D, Littell JS (2017) Climate change and the eco‐hydrology of fire: will area burned increase in a warming western USA? Ecological Applications 27, 26-36.
| Crossref | Google Scholar |
Meyer MD (2015) Forest fire severity patterns of resource objective wildfires in the southern Sierra Nevada. Journal of Forestry 113, 49-56.
| Crossref | Google Scholar |
Miller JD, Safford H (2012) Trends in wildfire severity: 1984 to 2010 in the Sierra Nevada, Modoc Plateau, and Southern Cascades, California, U.S.A. Fire Ecology 8, 41-57.
| Crossref | Google Scholar |
Miller JD, Safford HD, Crimmins M, Thode AE (2009) Quantitative evidence for increasing forest fire severity in the Sierra Nevada and Southern Cascade Mountains, California and Nevada, USA. Ecosystems 12, 16-32.
| Crossref | Google Scholar |
Miller JD, Collins BM, Lutz JA, Stephens SL, Van Wagtendonk JW, Yasuda DA (2012) Differences in wildfires among ecoregions and land management agencies in the Sierra Nevada region, California, USA. Ecosphere 3, art80.
| Crossref | Google Scholar |
Moreira F, Ascoli D, Safford HD, et al. (2020) Wildfire management in Mediterranean-type regions: paradigm change needed. Environmental Research Letters 15, 011001.
| Crossref | Google Scholar |
Natural Resources Canada (2021) ‘Cost of wildland fire protection.’ (Natural Resources Canada) Available at https://34c031f8-c9fd-4018-8c5a-4159cdff6b0d-cdn-endpoint.azureedge.net/-/media/calfire-website/our-impact/fire-statistics/featured-items/top20_acres.pdf?rev=be2a6ff85932475e99d70fa9458dca79&hash=A355A978818640DFACE7993C432ABF81 [accessed 8 March 2021]
North M, Brough A, Long J, Collins B, Bowden P, Yasuda D, Miller J, Sugihara N (2015a) Constraints on mechanized treatment significantly limit mechanical fuels reduction extent in the Sierra Nevada. Journal of Forestry 113, 40-48.
| Crossref | Google Scholar |
North MP, Stephens SL, Collins BM, Agee JK, Aplet G, Franklin JF, Fulé PZ (2015b) Reform forest fire management. Science 349, 1280-1281.
| Crossref | Google Scholar |
North MP, Tompkins RE, Bernal AA, Collins BM, Stephens SL, York RA (2022) Operational resilience in western US frequent-fire forests. Forest Ecology and Management 507, 120004.
| Crossref | Google Scholar |
O’Connor CD, Falk DA, Lynch AM, Swetnam TW (2014) Fire severity, size, and climate associations diverge from historical precedent along an ecological gradient in the Pinaleño Mountains, Arizona, USA. Forest Ecology and Management 329, 264-278.
| Crossref | Google Scholar |
O’Connor CD, Falk DA, Lynch AM, Swetnam TW, Wilcox CP (2017) Disturbance and productivity interactions mediate stability of forest composition and structure. Ecological Applications 27, 900-915.
| Crossref | Google Scholar |
Parks SA, Abatzoglou JT (2020) Warmer and drier fire seasons contribute to increases in area burned at high severity in western US forests from 1985 to 2017. Geophysical Research Letters 47(22), e2020GL089858.
| Crossref | Google Scholar |
Pennick McIver C, Cook PS, Becker DR (2021) The fiscal burden of wildfires: State expenditures and funding mechanisms for wildfire suppression in the Western US and implications for federal policy. State and Local Government Review 53(4), 337-351.
| Crossref | Google Scholar |
Perry DA, Hessburg PF, Skinner CN, Spies TA, Stephens SL, Taylor AH, Franklin JF, McComb B, Riegel G (2011) The ecology of mixed severity fire regimes in Washington, Oregon, and Northern California. Forest Ecology and Management 262(5), 703-717.
| Crossref | Google Scholar |
Pierce DW, Cayan DR (2013) The uneven response of different snow measures to human-induced climate warming. Journal of Climate 26, 4148-4167.
| Crossref | Google Scholar |
Pierce DW, Das T, Cayan DR, Maurer EP, Miller NL, Bao Y, Kanamitsu M, Yoshimura K, Snyder MA, Sloan LC, Franco G, Tyree M (2013) Probabilistic estimates of future changes in California temperature and precipitation using statistical and dynamical downscaling. Climate Dynamics 40, 839-856.
| Crossref | Google Scholar |
Polade SD, Pierce DW, Cayan DR, Gershunov A, Dettinger MD (2014) The key role of dry days in changing regional climate and precipitation regimes. Scientific Reports 4, 4364.
| Crossref | Google Scholar |
Polade SD, Gershunov A, Cayan DR, Dettinger MD, Pierce DW (2017) Precipitation in a warming world: Assessing projected hydro-climate changes in California and other Mediterranean climate regions. Scientific Reports 7, 10783.
| Crossref | Google Scholar |
Quinn-Davidson LN, Varner JM (2012) Impediments to prescribed fire across agency, landscape and manager: an example from northern California. International Journal of Wildland Fire 21, 210-218.
| Crossref | Google Scholar |
Radeloff VC, Helmers DP, Kramer HA, Mockrin MH, Alexandre PM, Bar-Massada A, Butsic V, Hawbaker TJ, Martinuzzi S, Syphard AD, Stewart SI (2018) Rapid growth of the US wildland-urban interface raises wildfire risk. Proceedings of the National Academy of Sciences 115(13), 3314-3319.
| Crossref | Google Scholar |
Riley KL, Abatzoglou JT, Grenfell IC, Klene AE, Heinsch FA (2013) The relationship of large fire occurrence with drought and fire danger indices in the western USA, 1984-2008: the role of temporal scale. International Journal of Wildland Fire 22, 894-909.
| Crossref | Google Scholar |
Ruthrof KX, Fontaine JB, Matusick G, Breshears DD, Law DJ, Powell S, Hardy G (2016) How drought-induced forest die-off alters microclimate and increases fuel loadings and fire potentials. International Journal of Wildland Fire 25, 819-830.
| Crossref | Google Scholar |
Ryan KC, Knapp EE, Varner JM (2013) Prescribed fire in North American forests and woodlands: history, current practice, and challenges. Frontiers in Ecology and the Environment 11, e15-e24.
| Crossref | Google Scholar |
Safford HD, Stevens JT (2017) Natural Range of Variation (NRV) for yellow pine and mixed conifer forests in the Sierra Nevada, southern Cascades, and Modoc and Inyo National Forests, California, USA. General Technical Report PSW-GTR-256. 229 p. (USDA Forest Service, Pacific Southwest Research Station: Albany, CA, USA)
Safford HD, Butz RJ, Bohlman GN, Coppoletta M, Estes BL, Gross SE, Merriam KE, Meyer MD, Molinari NA, Wuenschel A (2021) Fire ecology of the North American Mediterranean-climate zone. In ‘Fire ecology and management of US forested ecosystems: past, present, and future. Managing Forest Ecosystems. Vol. 39’. (Eds B Collins, CH Greenberg) (Springer: Cham, Switzerland) 10.1007/978-3-030-73267-7_9
Safford HD, Paulson AK, Steel ZL, Young DJ, Wayman RB (2022) The 2020 California fire season: A year like no other, a return to the past, or a harbinger of the future? Global Ecology and Biogeography 31, 2005-2025.
| Crossref | Google Scholar |
Salzer MW, Hughes MK, Bunn AG, Kipfmueller KF (2009) Recent unprecedented tree-ring growth in bristlecone pine at the highest elevations and possible causes. Proceedings of the National Academy of Sciences 106, 20348-20353.
| Crossref | Google Scholar |
Salzer MW, Bunn AG, Graham NE, Hughes MK (2014) Five millennia of paleotemperature from tree-rings in the Great Basin, USA. Climate Dynamics 42, 1517-1526.
| Crossref | Google Scholar |
Schoennagel T, Balch JK, Brenkert-Smith H, Dennison PE, Harvey BJ, Krawchuk MA, Mietkiewicz N, Morgan P, Moritz MA, Rasker R, Turner MG, Whitlock C (2017) Adapt to more wildfire in western North American forests as climate changes. Proceedings of the National Academy of Sciences 114(18), 4582-4590.
| Crossref | Google Scholar |
Scholl AE, Taylor AH (2010) Fire regimes, forest change, and self‐organization in an old‐growth mixed‐conifer forest, Yosemite National Park, USA. Ecological Applications 20, 362-380.
| Crossref | Google Scholar |
Schultz CA, McCaffrey SM, Huber-Stearns HR (2019) Policy barriers and opportunities for prescribed fire application in the western United States. International Journal of Wildland Fire 28, 874-884.
| Crossref | Google Scholar |
Schwartz MW, Syphard AD (2021) Fitting the solutions to the problems in managing extreme wildfire in California. Environmental Research Communications 3, 081005.
| Crossref | Google Scholar |
Schwartz MW, Butt N, Dolanc CR, Holguin A, Moritz MA, North MP, Safford HD, Stephenson NL, Thorne JH, van Mantgem PJ (2015) Increasing elevation of fire in the Sierra Nevada and implications for forest change. Ecosphere 6(7), 121.
| Crossref | Google Scholar |
Seager R, Hooks A, Williams AP, Cook B, Nakamura J, Henderson N (2015) Climatology, variability, and trends in the US vapor pressure deficit, an important fire-related meteorological quantity. Journal of Applied Meteorology and Climatology 54(6), 1121-1141.
| Crossref | Google Scholar |
Short KC (2021) ‘Spatial wildfire occurrence data for the United States, 1992-2018 [FPA_FOD_20210617]’, 5th edn. (Forest Service Research Data Archive: Fort Collins, CO, USA) 10.2737/RDS-2013-0009.5
Skinner CN, Abbott CS, Fry DL, Stephens SL, Taylor AH, Trouet V (2009) Human and climatic influences on fire occurrence in California’s north coast range, USA. Fire Ecology 5, 76-99.
| Crossref | Google Scholar |
Sleeter BM, Wilson TS, Soulard CE, Liu J (2011) Estimation of late twentieth century land-cover change in California. Environmental Monitoring and Assessment 173, 251-266.
| Crossref | Google Scholar |
Smith SJ, Edmonds J, Hartin CA, Mundra A, Calvin K (2015) Near-term acceleration in the rate of temperature change. Nature Climate Change 5, 333-336.
| Crossref | Google Scholar |
Steel ZL, Safford HD, Viers JH (2015) The fire frequency-severity relationship and the legacy of fire suppression in California forests. Ecosphere 6(1), 8.
| Crossref | Google Scholar |
Steel ZL, Koontz MJ, Safford HD (2018) The changing landscape of wildfire: Burn pattern trends and implications for California’s yellow pine and mixed conifer forests. Landscape Ecology 33, 1159-1176.
| Crossref | Google Scholar |
Steelman TA, McCaffrey S (2013) Best practices in risk and crisis communication: implications for natural hazards management. Natural Hazards 65, 683-705.
| Crossref | Google Scholar |
Stephens SL, Fry DL (2005) Fire history in coast redwood stands in the northeastern Santa Cruz Mountains, California. Fire Ecology 1, 2-19.
| Crossref | Google Scholar |
Stephens SL, Gill SJ (2005) Forest structure and mortality in an old-growth Jeffrey pine-mixed conifer forest in north-western Mexico. Forest Ecology and Management 205, 15-28.
| Crossref | Google Scholar |
Stephens SL, Moghaddas JJ (2005) Experimental fuel treatment impacts on forest structure, potential fire behavior, and predicted tree mortality in a California mixed conifer forest. Forest Ecology and Management 215, 21-36.
| Crossref | Google Scholar |
Stephens SL, Martin RE, Clinton NE (2007) Prehistoric fire area and emissions from California’s forests, woodlands, shrublands, and grasslands. Forest Ecology and Management 251, 205-216.
| Crossref | Google Scholar |
Stephens SL, McIver JD, Boerner REJ, Fettig CJ, Fontaine JB, Hartsough BR, Kennedy PL, Schwilk DW (2012) The effects of forest fuel-reduction treatments in the United States. BioScience 62, 549-60.
| Crossref | Google Scholar |
Stephens SL, Thompson S, Boisramé G, Collins BM, Ponisio LC, Rakhmatulina E, Steel ZL, Stevens JT, van Wagtendonk JW, Wilkin K (2021) Fire, water, and biodiversity in the Sierra Nevada: a possible triple win. Environmental Research Communications 3(8), 081004.
| Crossref | Google Scholar |
Stevens MB, González‐Rouco JF, Beltrami H (2008) North American climate of the last millennium: underground temperatures and model comparison. Journal of Geophysical Research: Earth Surface 113, F01008.
| Crossref | Google Scholar |
Stevens-Rumann CS, Kemp KB, Higuera PE, Harvey BJ, Rother MT, Donato DC, Morgan P, Veblen TT (2018) Evidence for declining forest resilience to wildfires under climate change. Ecology Letters 21, 243-252.
| Crossref | Google Scholar |
Stewart IT, Cayan DR, Dettinger MD (2005) Changes toward earlier streamflow timing across western North America. Journal of Climate 18, 1136-1155.
| Crossref | Google Scholar |
Swain DL (2021) A shorter, sharper rainy season amplifies California wildfire risk. Geophysical Research Letters 48(5), e2021GL092843.
| Crossref | Google Scholar |
Swain DL, Langenbrunner B, Neelin JD, Hall A (2018) Increasing precipitation volatility in twenty-first-century California. Nature Climate Change 8, 427-433.
| Crossref | Google Scholar |
Swetnam TW (1993) Fire history and climate change in giant sequoia groves. Science 262, 885-889.
| Crossref | Google Scholar |
Swetnam TW, Baisan CH, Caprio AC, Brown PM, Touchan R, Anderson RS, Hallett DJ (2009) Multi-millennial fire history of the giant forest, Sequoia National Park, California, USA. Fire Ecology 5, 120-150.
| Crossref | Google Scholar |
Swetnam TW, Farella J, Roos CI, Liebmann MJ, Falk DA, Allen CD (2016) Multiscale perspectives of fire, climate and humans in western North America and the Jemez Mountains, USA. Philosophical Transactions of the Royal Society B: Biological Sciences 371, 20150168.
| Crossref | Google Scholar |
Syphard AD, Keeley JE (2015) Location, timing and extent of wildfire vary by cause of ignition. International Journal of Wildland Fire 24, 37-47.
| Crossref | Google Scholar |
Syphard AD, Gershunov A, Lawson DM, Rivera Huerta H, Guzman-Morales J, Jennings MK (2018a) San Diego Wildfires: Drivers of Change and Future Outlook. In ‘San Diego County ecosystems: ecological impacts of climate change on a biodiversity hotspot’. California’s Fourth Climate Change Assessment. Publication number: EXT-CCC4A-2018-010. (Eds MK Jennings, D Cayan, J Kalansky, AD Pairis, et al.) pp. 49–69. (California Energy Commission)
Syphard AD, Brennan TJ, Keeley JE (2019) Extent and drivers of vegetation type conversion in Southern California chaparral. Ecosphere 10, e02796.
| Crossref | Google Scholar |
Taylor AH (2000) Fire regimes and forest changes in mid and upper montane forests of the southern Cascades, Lassen Volcanic National Park, California, USA. Journal of Biogeography 27, 87-104.
| Crossref | Google Scholar |
Taylor AH, Beaty RM (2005) Climatic influences on fire regimes in the northern Sierra Nevada mountains, Lake Tahoe Basin, Nevada, USA. Journal of Biogeography 32, 425-438.
| Crossref | Google Scholar |
Taylor AH, Skinner CN (2003) Spatial patterns and controls on historical fire regimes and forest structure in the Klamath Mountains. Ecological Applications 13, 704-719.
| Crossref | Google Scholar |
Taylor AH, Trouet V, Skinner CN (2008) Climatic influences on fire regimes in montane forests of the southern Cascades, California, USA. International Journal of Wildland Fire 17, 60-71.
| Crossref | Google Scholar |
Taylor AH, Trouet V, Skinner CN, Stephens S (2016) Socioecological transitions trigger fire regime shifts and modulate fire–climate interactions in the Sierra Nevada, USA, 1600–2015 CE. Proceedings of the National Academy of Sciences 113, 13684-13689.
| Crossref | Google Scholar |
Tripp B (2020) Our land was taken. But we still hold the knowledge of how to stop mega-fires. The Guardian, 16 September 2020. Available at https://www.theguardian.com/commentisfree/2020/sep/16/california-wildfires-cultural-burns-indigenous-people [accessed 24 August 2021]
Trouet V, Taylor AH, Wahl ER, Skinner CN, Stephens SL (2010) Fire‐climate interactions in the American West since 1400 CE. Geophysical Research Letters 37(4), L04702.
| Crossref | Google Scholar |
Tubbesing CL, Fry DL, Roller GB, Collins BM, Fedorova VA, Stephens SL, Battles JJ (2019) Strategically placed landscape fuel treatments decrease fire severity and promote recovery in the northern Sierra Nevada. Forest Ecology and Management 436, 45-55.
| Crossref | Google Scholar |
Ukkola AM, De Kauwe MG, Roderick ML, Abramowitz G, Pitman AJ (2020) Robust future changes in meteorological drought in CMIP6 projections despite uncertainty in precipitation. Geophysical Research Letters 47, e2020GL087820.
| Crossref | Google Scholar |
Van de Water KM, Safford HD (2011) A summary of fire frequency estimates for California vegetation before Euroamerican settlement. Fire Ecology 7(3), 26-58.
| Crossref | Google Scholar |
van Mantgem PJ, Falk DA, Williams EC, Das AJ, Stephenson NL (2020) The influence of pre-fire growth patterns on post-fire tree mortality for common conifers in western US parks. International Journal of Wildland Fire 29(6), 513-518.
| Crossref | Google Scholar |
Wang D, Guan D, Zhu S, Kinnon MM, Geng G, Zhang Q, Zheng H, Lei T, Shao S, Gong P, Davis SJ (2021) Economic footprint of California wildfires in 2018. Nature Sustainability 4(3), 252-260.
| Crossref | Google Scholar |
Wang JA, Randerson JT, Goulden ML, Knight CA, Battles JJ (2022) Losses of tree cover in California driven by increasing fire disturbance and climate stress. AGU Advances 3(4), e2021AV000654.
| Crossref | Google Scholar |
Westerling AL (2016) Increasing western US forest wildfire activity: sensitivity to changes in the timing of spring. Philosophical Transactions of the Royal Society B: Biological Sciences 371, 20150178.
| Crossref | Google Scholar |
Westerling AL, Swetnam TW (2003) Interannual to decadal drought and wildfire in the western United States. EOS, Transactions American Geophysical Union 84, 545-555.
| Crossref | Google Scholar |
Westerling AL, Cayan DR, Brown TJ, Hall BL, Riddle LG (2004) Climate, Santa Ana winds and autumn wildfires in southern California. EOS, Transactions American Geophysical Union 85, 289-296.
| Crossref | Google Scholar |
Westerling AL, Hidalgo HG, Cayan DR, Swetnam TW (2006) Warming and earlier spring increase western U.S. forest wildfire activity. Science 313, 940-943.
| Crossref | Google Scholar |
Williams AP, Abatzoglou JT, Gershunov A, Guzman-Morales J, Bishop DA, Balch JK, Lettenmaier DP (2019) Observed impacts of anthropogenic climate change on wildfire in California. Earth’s Future 7(8), 892-910.
| Crossref | Google Scholar |
Williams AP, Cook ER, Smerdon JE, Cook BI, Abatzoglou JT, Bolles K, Baek SH, Badger AM, Livneh B (2020) Large contribution from anthropogenic warming to an emerging North American megadrought. Science 368, 314-318.
| Crossref | Google Scholar |
Williams JN, Safford HD, Enstice N, Steel ZL, Paulson AK (2023) High-severity burned area and proportion exceed historic conditions in Sierra Nevada, California, and adjacent ranges. Ecosphere 14(1), e4397.
| Crossref | Google Scholar |
Williams JN, Quinn-Davidson L, Safford HD, et al. (in press) Overcoming barriers to prescribed fire in the North American Mediterranean Climate Zone. Frontiers in Ecology and the Environment
| Google Scholar |
Williamson MA, Fleishman E, Mac Nally RC, Chambers JC, Bradley BA, Dobkin DS, Board DI, Fogarty FA, Horning N, Leu M, Wohlfeil Zillig M (2020) Fire, livestock grazing, topography, and precipitation affect occurrence and prevalence of cheatgrass (Bromus tectorum) in the central Great Basin, USA. Biological Invasions 22, 663-680.
| Crossref | Google Scholar |
Young JD, Evans AM, Iniguez JM, Thode A, Meyer MD, Hedwall SJ, McCaffrey S, Shin P, Huang CH (2020) Effects of policy change on wildland fire management strategies: evidence for a paradigm shift in the western US? International Journal of Wildland Fire 29, 857-877.
| Crossref | Google Scholar |
Yuan W, Zheng Y, Piao S, Ciais P, Lombardozzi D, Wang Y, Ryu Y, Chen G, Dong W, Hu Z, Jain AK, Jiang C, Kato E, Li S, Lienert S, Liu S, Nabel JEMS, Qin Z, Quine T, Sitch S, Smith WK, Wang F, Wu C, Xiao Z, Yang S (2019) Increased atmospheric vapor pressure deficit reduces global vegetation growth. Science Advances 5(8), eaax1396.
| Crossref | Google Scholar |
Zamora-Reyes D, Black B, Trouet V (2022) Enhanced winter, spring, and summer hydroclimate variability across California from 1940 to 2019. International Journal of Climatology 42(9), 4940-4952.
| Crossref | Google Scholar |
Zhuang Y, Fu R, Santer BD, Dickinson RE, Hall A (2021) Quantifying contributions of natural variability and anthropogenic forcings on increased fire weather risk over the western United States. Proceedings of the National Academy of Sciences 118(45),.
| Crossref | Google Scholar |