A new look at the role of fire-released moisture on the dynamics of atmospheric pyro-convection
Gunnar Luderer A C , Jörg Trentmann B and Meinrat O. Andreae AA Max Planck Institute for Chemistry, Department of Biogeochemistry, PO Box 3060, D-55020 Mainz, Germany.
B Institute for Atmospheric Physics, Johannes Gutenberg University Mainz, Becherweg 21, D-55099 Mainz, Germany.
C Corresponding author. Present address: Potsdam Institute for Climate Impact Research, PO Box 60 12 03, D-14412 Potsdam, Germany. Email: luderer@pik-potsdam.de
International Journal of Wildland Fire 18(5) 554-562 https://doi.org/10.1071/WF07035
Submitted: 16 February 2008 Accepted: 29 September 2008 Published: 10 August 2009
Abstract
We investigate the contribution of the moisture released by wildland fires to the water budget and the convection dynamics of pyro-clouds forming atop fires. Using an approach based on stoichiometric principles and parcel theory of convection, we assess the relative contribution of sensible heat and latent heat to the convection energy. We find that moisture release is of much lesser importance for the fire convection than the release of sensible heat from the combustion. We conclude from theoretical considerations that it is highly unlikely that the decrease of the cloud base of pyro-cumulus compared with that of ambient free convection is due to the fire-released moisture alone, in contrast to what has been suggested previously. In addition to the analytical results, numerical simulations of a specific case study are presented. They show that the fire-released moisture accounts only for a small portion of the total water in the pyro-cumulus cloud. Also, the effect of the fire-released moisture on the convection dynamics and the height of injection is found to be small compared with the effect of the sensible heat release from the fire.
Acknowledgements
We thank B. M. Wotton and J. Goldammer for the discussion on fuel moisture values. G. Luderer was funded by an International Max Planck Research School Fellowship. We thank the Max Planck Society for supporting the present work. We thank two anonymous referees for their constructive comments that helped to improve the paper.
Achtemeier GL (2006) Measurements of moisture in smoldering smoke and implications for fog. International Journal of Wildland Fire 15, 517–525.
| Crossref | GoogleScholarGoogle Scholar |
Clements CB, Potter BE , Zhong S (2006) In situ measurements of water vapor, heat, and CO2 fluxes within a prescribed grass fire. International Journal of Wildland Fire 15, 299–306.
| Crossref | GoogleScholarGoogle Scholar | CAS |
Fromm MD , Servranckx R (2003) Transport of forest fire smoke above the tropopause by supercell convection. Geophysical Research Letters 30(10), 1542.
| Crossref | GoogleScholarGoogle Scholar |
Herzog M, Oberhuber JM , Graf HF (2003) A prognostic turbulence scheme for the non-hydrostatic plume model ATHAM. Journal of the Atmospheric Sciences 60, 2783–2796.
| Crossref | GoogleScholarGoogle Scholar |
Reid JS, Hobbs PV, Rangno AL , Hegg DA (1999) Relationships between cloud droplet effective radius, liquid water content, and droplet concentration for warm clouds in Brazil embedded in biomass smoke. Journal of Geophysical Research 104(D6), 6145–6153.
| Crossref | GoogleScholarGoogle Scholar | CAS |
Rosenfeld D, Fromm M, Trentmann J, Luderer G, Andreae MO , Servranckx R (2007) The Chisholm firestorm: observed microstructure, precipitation and lightning activity of a pyro-Cb. Atmospheric Chemistry and Physics 7, 645–659.
| CAS |
Taylor RJ, Evans ST, King NK, Stephens ET, Packham DR , Vines RG (1973) Convective activity above a large-scale bushfire. Journal of Applied Meteorology 12, 1144–1150.
| Crossref | GoogleScholarGoogle Scholar |
Trentmann J, Andreae MO, Graf HF, Hobbs PV, Ottmar RD , Trautmann T (2002) Simulation of a biomass-burning plume: comparison of model results with observations. Journal of Geophysical Research 107(D2), 4013.
| Crossref | GoogleScholarGoogle Scholar |
Wooster MJ (2002) Small-scale experimental testing of fire radiative energy for quantifying mass combusted in natural vegetation fires. Geophysical Research Letters 29(21),
| Crossref | GoogleScholarGoogle Scholar |

Wooster MJ, Roberts G, Perry GLW , Kaufman YJ (2005) Retrieval of biomass combustion rates and totals from fire radiative power observations: FRP derivation and calibration relationships between biomass consumption and fire radiative energy release. Journal of Geophysical Research 110, D24311.
| Crossref | GoogleScholarGoogle Scholar |

Yokelson R (2008) Interactive comment on ‘Water vapor release from biofuel combustion’ by R. S. Parmar et al. Atmospheric Chemistry and Physics Discussion 8, S1406–S1418.

Appendix
In order to assess the respective effects of temperature and humidity perturbations on the lifting condensation level, we can apply a linearization approach

to Eqn 5:
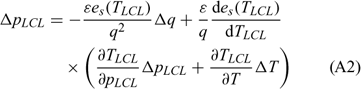
Note that TLCL itself is a function of the temperature at the reference level T and the lifting condensation level pLCL ; hence both its partial derivatives need to be considered as well. From Eqn 6, they can be calculated as


The differential temperature dependence of the saturation water vapor partial pressure is given by the Clausius–Clapeyron equation (e.g. Rogers and Yau 1989)

so we can convert

Substituting Eqns 5, A6, A4, and A3 into Eqn A2 yields

Rearranging results then in the form presented in Eqn 7:
