Future fire events are likely to be worse than climate projections indicate – these are some of the reasons why
Mika Peace
A
B
Abstract
Climate projections signal longer fire seasons and an increase in the number of dangerous fire weather days for much of the world including Australia.
Here we argue that heatwaves, dynamic fire–atmosphere interactions and increased fuel availability caused by drought will amplify potential fire behaviour well beyond projections based on calculations of afternoon forest fire danger derived from climate models.
We review meteorological dynamics contributing to enhanced fire behaviour during heatwaves, drawing on examples of dynamical processes driving fire behaviour during the Australian Black Summer bushfires of 2019–20.
Key dynamical processes identified include: nocturnal low-level jets, deep, unstable planetary boundary layers and fire–atmosphere coupling.
The future scenario we contend is long windows of multi-day fire events where overnight suppression is less effective and fire perimeters will expand continuously and aggressively over multiple days and nights.
Greater overnight fire activity and multi-day events present strategic and tactical challenges for fire management agencies including having to expand resourcing for overnight work, manage personnel fatigue and revise training to identify conditions conducive to unusually active fire behaviour overnight. Effective messaging will be critical to minimise accidental fire ignition during heatwaves and to alert the community to the changing fire environment
Keywords: Australia, Black Summer, boundary layer, climate projections, fire, heatwave, meteorology, plume dynamics.
Introduction
Heatwaves are a sequence of days and nights with unusually high maximum and minimum temperatures. Elevated fire potential and extreme fire behaviour are synonymous with heatwaves due to hot, dry surface conditions and a deep atmospheric boundary layer (or planetary boundary layer (PBL)) supporting organised plume development. Historical records show large impactful fires are often associated with heatwaves, examples include Ash Wednesday 1983 and Black Saturday 2009 (Australia), Pedrόgão Grande 2017 (Portugal), Attica 2018 (Greece), Camp 2018 (USA) and Fort McMurray 2016 (Canada).
In Australia, official heatwave warnings are issued using the Excess Heat Factor described by Nairn and Fawcett (2014). Typically, heatwaves occur under the influence of quasi-stationary upper tropospheric ridges which are seen as maximum geopotential height anomalies at 500 hPa and associated with large amplitude propagating Rossby waves (described by Reeder et al. 2015). The upper features are reflected at the surface by slow-moving or near-stationary synoptic high-pressure systems that can persist for several days, known as blocking highs. Strong lower troposphere subsidence and warming in the high pressure cells can have spatial extents of a few thousand kilometres, therefore heatwaves can affect extensive areas and very large populations (Copernicus Report 2022). Accelerated trends in heat extremes in Western Europe and the links to jet stream dynamics are described by Rousi et al. (2022), who also point out that accurate representation of jet stream dynamics in climate projections is critical (although uncertain) for capturing future heatwave trends. Similarly, Patterson et al. (2019) show that simulation of atmospheric blocking is not captured well in many general circulation models, and that current and future blocking biases are the subject of limited research in the Southern Hemisphere.
The Intergovernmental Panel on Climate Change 6th Assessment Report (IPCC 2023) states the ‘Frequency of extreme fire weather days has increased, and the fire season has become longer since 1950 at many locations. The intensity, frequency and duration of fire weather events are projected to increase throughout Australia. The fire season is generally projected to lengthen from summer into spring and autumn, with some regional variability.’ These IPCC predictions of an increase in the number of dangerous fire weather days and longer fire seasons for Australia are mostly made by calculating the Forest Fire Danger Index (FFDI) of McArthur (1973) from climate model output (e.g. Dowdy 2018; Clarke and Evans 2019; Harris and Lucas 2019).
Normal diurnal cycle of fire activity and vapour pressure deficit (VPD)
Fire weather indices such as FFDI and the Canadian Forest Fire Weather Index (FWI) System (Stocks et al. 1989) are usually calculated to assess daytime conditions at the surface. The Hot–Dry–Windy Index (HDW) of Srock et al. (2018) is calculated from the wind speed and vapour pressure deficit (VPD) within 500 m from the surface and therefore captures boundary layer features to some extent.
For climate projections, fire danger indices are calculated from weather parameters at midday or mid-afternoon when maximum heating is assumed. This approach captures increases in maximum temperatures, changes in precipitation, and surface-based drivers of future fire activity, and is intended to capture the potential maximum afternoon fire spread rate and burn intensity. However, fire danger indices calculated from climate projections in this way do not incorporate multiple dynamical processes that affect fire activity. Understandably, most fire weather climate studies for Australia to date use the FFDI system, which has been replaced by the Australian Fire Danger Rating System (AFDRS, Hollis et al. 2024), for which long-term projections have not yet been undertaken. The HDW index has limited operational use in Australia and does not feature in Australian fire–climate projections. The primary focus of the Canadian system is fire prediction during daytime active burning periods, with a tendency to overpredict nocturnal fire activity. Consequently, operational fire weather indices exhibit biases when applied to climate projections — which is unsurprising as they were not designed for this purpose.
Dowdy (2020) calculated climate change projections for fire weather using Global Climate Models. FFDI was used, with daily maximum temperature at 2:00 pm and mid-afternoon (UTC+06:00) values of relative humidity and wind speed, with drought factor determined using the Keetch–Byram Drought Index (Keetch and Byram 1968). Clarke and Evans (2019) generated an ensemble from four Global Climate Models, which was downscaled using the Weather Research and Forecasting model (WRF), and they similarly calculated FFDI using daily maximum temperature with wind speed and relative humidity at 3:00 pm. Krikken et al. (2021) calculated the FWI for Sweden from sub-daily ERA interim and ERA5 datasets (Dee et al. 2011; Hersbach et al. 2020) using midday temperature, relative humidity and surface wind speed. They make the point that because the climate models show higher temperatures with smaller changes in relative humidity and wind speed, the response of the FWI relates mostly to the changes in precipitation and temperature. Jones et al. (2022) provide a review of current understanding of the impacts of climate change on fire weather. Their comprehensive review and approach of calculating FWI from daily ERA5 surface data underscores through omission that there is no available method for calculating the complex three-dimensional atmospheric processes in the fire environment. Paradoxically, it is these processes that contribute to the most impactful fires.
As pointed out by Balch et al. (2022) a focus on daily (usually afternoon) peak in fire indices highlights factors that promote fire spread, rather than that slow or halt fire growth. Night-time usually provides a critical window for slowing or extinguishing fires owing to lower temperatures and relative humidity recovery. Fire suppression operations often take advantage of night-time conditions when fires activity tends to reduce. Balch et al. (2022) explored global trends in night-time fire activity to assess how the climatological night-time fire season (from 1979 to 2020) has changed as a function of increasing overnight temperatures. Using satellite observations, they showed that globally night-time fire intensity has increased, and they linked this to hotter and drier nights with focus on VPD. They state that ‘with continued night-time warming, we expect to further lose the ‘night brakes’ on fire resulting in a greater number of escaped wildfires’ and conclude that continued warming owing to anthropogenic climate change will promote more intense, longer lasting and larger fires.
Similarly, Chiodi et al. (2021) attribute fires burning longer into the night and fire intensity escalating earlier in the morning due to increases in night-time temperatures and near-surface VPD. The studies of Balch et al. (2022) and Chiodi et al. (2021) both consider meteorological fields at the surface, where an increase in overnight VPD would expect to drive a change in fire activity (e.g. Seager et al. 2015). However, Chiodi et al. (2021) also acknowledge that the available observations show a stronger signal than that suggested by climate projections, thereby indicating there are other mechanisms at play.
Satellite imagery is a data set that can be used objectively to assess trends in fire activity. Luo et al. (2024) and Freeborn et al. (2022) used GOES-R and MODIS satellites, respectively, to explore patterns in overnight fire activity over North America. Both studies showed concerning trends in increasing night-time fire activity.
The shift towards increased nocturnal fire activity in recent years has been also reported in mainstream media. ‘The changing nature of bushfires is leaving emergency services with less relief at night’ appeared as an online Australian Broadcasting Corporation article (24 December 2021) (https://www.abc.net.au/news/2021-12-24/the-changing-nature-of-bushfires-offers-less-reprieve-at-night/100718446). The report pointed to several examples of recent fires in Western Australia that doubled or tripled in size overnight during heatwaves in Northcliffe (2015), Yanchep (2019) and Wooroloo (2021) (see breakout boxes). These fires proved very challenging for tactical and strategic emergency management, first responders and local communities due to in a large part the difficulty of overnight suppression.
Consecutive hot days and nights during heatwaves extend the period of time favourable for continuous fire spread into the evening and overnight, leading to larger fires (Abram et al. 2021; Balch et al. 2022). The frequency and intensity of heatwaves has increased in recent years, and climate projections show that this trend is expected to continue in a warming climate.
In this paper, we contend that the greatest change to fire regimes and consecutive impacts on communities will arise as a consequence of dynamical response to novel heatwave and drought1 regimes, rather than a linear shift in response to global increase in average surface air temperature. We focus on Australia, but the inference is global. We aim to show that key drivers of future fire activity will be (1) fire plumes interacting with the PBL during heatwave events, (2) more multi-day fire events during heatwaves due to limited suppression effectiveness in the overnight period, and (3) that heightened fire activity will be compounded by increased fuel availability of live and dead biomass linked to heatwaves and droughts.
Fire science is highly cross-disciplinary and predicting fire behaviour incorporates complex contributing fields including meteorology, combustion dynamics, plant physiology and hydrological process, as well as human behaviour. The complexity of multiple contributing factors makes it very difficult to predict future fire regimes with a high degree of confidence. However, we contend that the currently published climate outlooks that calculate future fire weather from daily max FFDI or FWI at the surface are likely to consistently underestimate future fire potential. The aim of this paper is to present a narrative that draws on unpublished insights from Australian fire meteorologists and fire behaviour analysts working in predictive services, as well as scientific literature to describe a breadth of factors that influence fire activity. We also examine processes that are likely to drive major fire events in a future climate.
Processes exacerbating future fire weather events beyond responses to increases in near-surface temperature and relative humidity include:
Elevated wind maxima (nocturnal low-level jets) driving overnight fire spread during heatwaves;
Deep, unstable planetary boundary layers supporting plume development and organised convection columns with potential for rotation;
Increased fire-atmosphere coupling due to fires with deep unstable plumes tapping into elevated winds;
Heatwaves leading to extended windows of weather conditions conducive to active fire behaviour throughout the diurnal cycle;
Increased risk of fire-generated thunderstorms;
Greater availability of live and dead fuel in response to drought and heatwaves.
The intended audience(s) for this paper are (1) fire management and emergency response personnel to inform future planning, (2) climate scientists to motivate design approaches that interrogate climate predictions and implications for future fire regimes in meaningful ways, and (3) policy makers to inform the development of policies appropriate for future fire environments. The scope of this work is a qualitative description of the influence of heatwaves in future fire regimes. A more quantitative analysis from climate projections is a necessary next step to assess future fire risk, where fire risk is the likelihood of a fire with potential for impact through damage or fatalities.
We address the topic in four parts by:
Examining dynamical processes driving fire behaviour using examples from the Australian Black Summer bushfires of 2019–20;
Reviewing meteorological dynamics contributing to enhanced fire behaviour during heatwaves and meteorological inputs to fire weather prediction;
Describing processes that can affect dead and live fuel availability and landscape dryness in drought and heatwaves;
Discussing the implications of heatwaves and fire regimes in a future climate due to the heightened potential for and consequences of increased diurnal fire activity. This discussion includes considerations for tactical and strategic planning for operational fire management paradigms, resourcing and physical health of firefighters and other incident management personnel, communities, and impacts on critical infrastructure, as well as the interplay and cascade of contributing elements.
Dynamical processes driving fire behaviour using examples from the Australian Black Summer bushfires of 2019–20
During the 2019–20 Black Summer fire season, extended and multiple heatwaves developed across Australia in response to climate drivers including a relatively weak El Niño, a strongly positive Indian Ocean Dipole, a persistent negative Southern Annular Mode and a sudden stratospheric warming event (e.g. Abram et al. 2021; Bureau of Meteorology 2020a; Lim et al. 2021). Numerous new records for both maximum and minimum temperatures were established. The hottest Australian overnight minimum of a stifling 35.9°C was recorded at Noona in inland New South Wales on 18 January 2020. Heatwaves combined with antecedent drought produced a very flammable landscape and favourable meteorological conditions for fire spread that resulted in widespread wildfire activity that persisted for several months. Southern Queensland and northern New South Wales saw serious wildfires in September 2019, while southern New South Wales, eastern Victoria and the Australian Capital Territory experienced wildfires well into January 2020. Numerous fires burned for several weeks, with the most significant fire runs occurring on a smaller number of days. Extreme nocturnal fire activity was a feature of many fires, with fire-fighting resources severely stretched and communities threatened, and in a number of cases severely impacted by fires.
Under heatwave conditions on 30 and 31 December 2019, numerous fires across southern NSW and eastern Victoria made significant runs during the daytime and overnight. Concurrent fires stretched resources day and night as they burned in a complex, heavily-forested terrain under the influence of the same broadscale synoptic weather system. Out of these fires, the Badja Forest, Corryong and Banana Track fires had some of the most significant impacts on communities.
Here, we document the impacts of these fires and then describe the processes contributing to the fire runs, thereby providing compelling examples of extreme fire behaviour during a heatwave. This context leads to subsequent sections which present the expectation for similar future events in a changing climate as more intense droughts and heatwaves are experienced.
Badja Forest fire, New South Wales
The Badja Forest fire ignited from a lightning strike in inaccessible terrain on 27 December 2019. The fire made an overnight run of 36 km in 6 h on 30–31 December (Fig. 1) and impacted the towns of Wandella, Cobargo and Quaama. NSW Rural Fire Service (RFS) Commissioner Shane Fitzsimmons described ‘extraordinary fire overnight, exceeding what was predicted in the given conditions’.
Video still taken from NSW RFS and Australian Capital Territory Government aircraft deployment, showing an overnight fire activity at the Badja Forest at 23:30 pm on 30 December 2019. https://www.news.com.au/national/raw-aerials-show-badja-forest-road-fire/video/6197496f4b6ef02cdb1cc8df6de4d320.
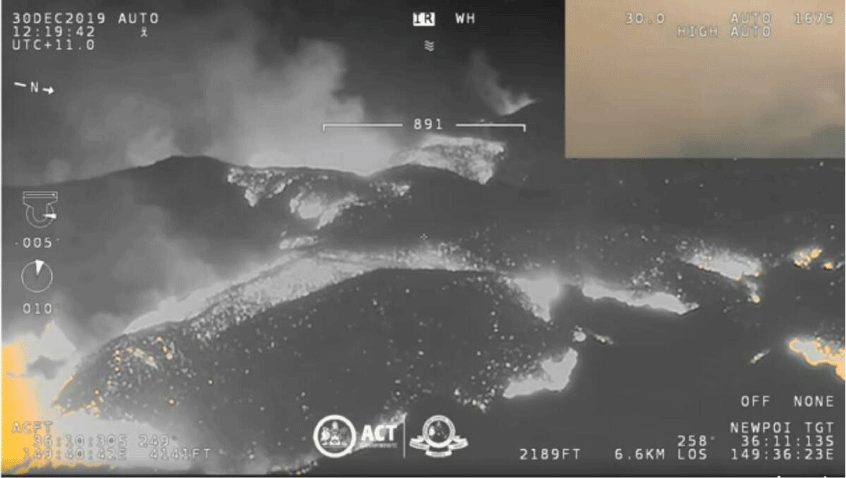
The extreme fire behaviour resulted in two fatalities. A fire generated vortex was documented in Wandella Valley, and a fire-generated thunderstorm was captured on satellite imagery. Catastrophic fire danger conditions (operational FFDI) were recorded by automatic weather stations between 04:00 and 05:00 am. This represented a large discrepancy between the official forecast and observations: the temperature was 10°C greater, relative humidity 30% lower and 10 m wind speed 25 km h−1 higher than forecast.
Banana Track fire, Victoria (also called the Wingan River and Mallacoota fire)
The Banana Track fire was ignited by a lightning strike near Wingan River, 30 km west of Mallacoota on 29 December 2019. The fire ran overnight on 30–31 December exhibiting rapid spread, crown fire and extensive spotting, and produced a nocturnal pyrocumulonimbus (pyroCb, fire generated thunderstorm) cloud. Much of the fire affected area was enveloped with dense smoke which challenged suppression efforts and intelligence gathering (Salkin 2022).
On 30 December the fire ran initially towards the south. A northwest to southwest wind change moved across the fireground on the morning of 31 December driving the fire east-northeast towards the coastal town of Mallacoota, which it impacted early morning on New Years Eve, attracting global media attention. The only access road to Mallacoota was cut by fire and 4,000 people, most of whom were celebrating summer holidays, huddled on the beach at Mallacoota on New Years Eve. A ‘State of Disaster’ was declared and over the following days a dramatic and unprecedented evacuation by the sea was conducted by the Royal Australian Navy.
Corryong fire, Victoria (also called Green Valley and Upper-Murray Walwa fire)
The Corryong fire ignited as the Green Valley fire in NSW on 29 December, then jumped the Murray River into Victoria and made a linear run of approximately 40 km overnight on 30–31 December with a forward rate of spread at night of 3.6 km h−1. New spot fire ignitions from long-distance firebrands contributed to the overnight fire spread, due to a deep plume and strong transport winds extending through the boundary layer. The fire ran a remarkable 78 km between 16:15 pm 30 December and 12:00 pm 31 December (Salkin 2022).
Media reports and photographs show the towns of Corryong and Cudgewa being impacted overnight with evacuations conducted in darkness and roads closed. Fire reports and observations in the early morning noted that firefighters had not previously observed such extreme fire behaviour and that the speed of the fire spread outpaced efforts to contain its spread.
Informal discussions between the authors and experienced fire behaviour analysts (FBANs) identified that the fire behaviour and spread of the Badja Forest, Corryong and Banana Track fires (as well as other major fires during the 2019–20 fire season) did not always reconcile with the standard application of fire spread models using surface-based weather inputs. FBANs remarked that fire spread was underpredicted for both daytime and night-time fire runs when predictions were verified against observed fire perimeters.
The concurrent overnight fire runs at the Badja Forest, Corryong and Banana Track were driven by meteorological processes synonymous with a heatwave over south-eastern Australia, a deep atmospheric boundary layer with hot, dry temperatures, and a nocturnal low-level jet.
Mills et al. (2022) used the VicClim 5 reanalysis dataset to examine meteorological drivers of several of the Victorian Black Summer fires reported on by Salkin (2022). Their description includes examples of low-level inversions overlain by an elevated mixed layer that through fire–atmosphere coupling may influence fire behaviour in a way not represented by surface meteorology. In describing overnight fire runs at Marthavale, Abbeyard, and Mount Burrowa, they state ‘It is likely that the heat release from these fires would have been sufficient to allow the fire to interact with (these) hot, dry, elevated mixed layers, particularly if the fire was at several 100-m elevation.’
Fire–atmosphere interaction processes are discussed in the following section.
Meteorological processes contributing to enhanced fire behaviour during a heatwave
Boundary layer structure
The PBL is the depth of the atmosphere through which turbulent mixing distributes the diurnal heating of the Earth’s surface. Heatwaves are synonymous with an unstable PBL structure that can reach 4–5 km deep. The structure of the PBL layer undergoes relatively slow changes diurnally during a heatwave. In the overnight periods, the near-surface radiation inversion is typically shallow and always overlain by a persistent layer of hot, dry air.
Fire plumes can extend from the surface through the PBL and pioneering fire science papers by Byram (1954), Haines (1988) and McArthur (1967) all describe the importance of atmospheric instability on fire behaviour. Byram (1954) stated that most severe fires (but not all extreme fire behaviour) occur on days when the atmosphere is turbulent and unstable to a height of several thousand feet, with an obvious and well-developed convection column, which may extend high into the atmosphere.
McArthur (1967) recognised that atmospheric instability and atmospheric conditions at a considerable height above a fire are important factors determining energy conversion and convection processes which influence its behaviour. Although atmospheric stability is not included explicitly in McArthur’s fire danger meters, presumably due to the difficulty in obtaining meteorological data at the time of development, the influence of instability is included implicitly through a strong dependence on the temperature and relative humidity inputs in his indices.
Haines (1988) proposed an index based on differentials in air temperature and dryness as a metric to assess how the presence of dry, unstable air can cause wildfires to become large and/or erratic. Although the validity of the original index has been questioned (e.g. Potter 2018; Clabo 2022), the Haines Index and its derivative C-Haines (Mills and McCaw 2010) remain a research contribution recognising the critical influence of PBL stability on fire activity and provide an easily calculated index that can be readily applied in operational fire weather.
The Nowra aerological diagram (Fig. 2) on the morning of the fire runs described above shows a PBL typical of a southern Australian heatwave with a deep dry adiabatic layer to a depth of 4 km. The very shallow near-surface radiation inversion shows strong thermal stratification, with a stable, cooler, moister layer within 200 m of the surface, and calm surface winds with wind speeds of 65 km h−1 at ~600 m elevation. Nowra is on the south coast of NSW, approximately 150 km from Badja and 300 km from Mallacoota and Corryong. Nowra is approximately 15 km from the coast, therefore climatologically the site would experience a maritime influence. However, the dominant mechanism in this case of the offshore wind regime would be a local terrestrial radiation inversion over low lying land.
Aerological diagram at Nowra at 06:00 am on 31 December. Sunrise was at 05:46 am, just earlier than balloon launch. Right side red line show observed temperature. Left side red line show observed dewpoint temperature. Brown diagonal lines are temperature with height. Brown horizontal axis are constant pressure. Black vertical axis shows height in feet (left side) and km (right side).
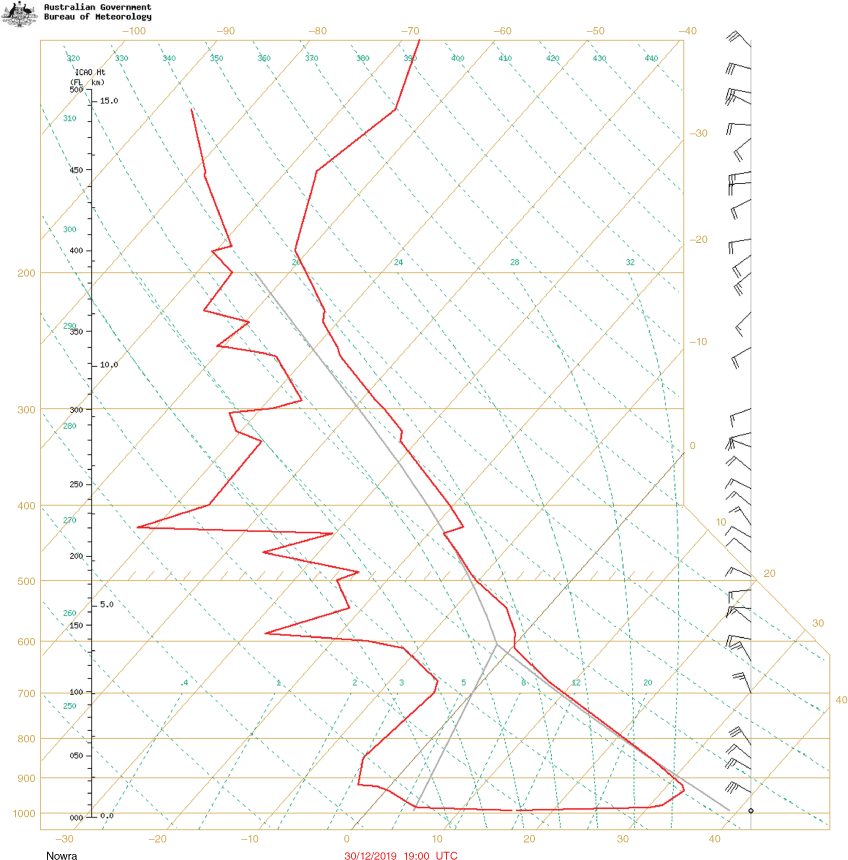
Depending on the inversion strength and the potential energy release from a fire, the PBL structure such as that observed at Nowra can support active fire spread day and night. If sufficient energy is released by a fire, the near-surface inversion will be eroded, or ‘mixed-out’ and the fire activity will be driven by the hotter, drier air and stronger winds above the surface. This is more likely to occur in elevated terrain and in heavy fuels, in comparison to a fire running in grass fuels (lower energy release) on flat terrain (greater nocturnal stability). Overnight fire activity primes conditions for extreme fire behaviour the following day when active, long perimeters are established in the landscape. Sharples (2009) provides a detailed discussion of how terrain, upper winds and elevated temperature inversions can have complex and dynamic influences on active fires. More recent observations and simulations have raised questions and prompted research activity to explore how these processes change when an intense fire plume is present.
Low level jets
Low-level jets are channels of fast wind above the surface that are typically much wider than they are deep. Byram (1954) described low-level jets as a contributing factor for blow-up fires. He uses the term ‘blow-up’ to describe a fire that suddenly and unexpectedly multiplies its rate of energy output many times. The cases he presents include overnight blow-ups driven by a low-level jet near to the surface, with a shallow wind maxima and strong vertical wind shear over complex, mountainous topography in the western USA.
Nocturnal low-level jets in southern Australia are a warm-season PBL phenomenon that often develops during synoptic high-pressure systems and fair weather conditions. Physical mechanisms underpinning the generation of nocturnal low-level jets are described by Blackadar (1957). Low level jets typically develop at around sunset when conditions are conducive to strong radiative cooling. Jets develop in response to diurnal variations in turbulent mixing through the PBL, with the wind strength reaching peak intensity in the pre-dawn hours. Overnight, the increase of the wind speed maximum usually coincides with the top of the nocturnal inversion, which develops not far above the surface.
Key precursors to jet formation are the establishment of a strongly turbulent dry convective boundary layer during the afternoon in response to surface heating, and a rapid cessation of the turbulence in the evening when a nocturnal inversion forms. During the day, there exists a near-balance between the pressure gradient, Coriolis and frictional forces in a deep PBL, and vertical turbulent mass exchange and mixing act to smooth out the maxima and minima of the velocity profile. As an overnight inversion develops, frictional forces at the surface are decoupled and the force imbalance causes an inertial oscillation in the winds, with the adjustment resulting in a super-geostrophic wind.
Markowski and Richardson (2010) observed that the strongest amplification of nocturnal low-level winds occurs following days during which winds in the upper boundary layer were most retarded by surface friction; these are days associated with abundant insolation from deep mixing and deep boundary layers. Simply put, heatwaves are favourable for strong nocturnal low-level jets.
Thermal forcing mechanisms resulting from terrain slope can make a substantial contribution to the amplitude of the diurnal wind oscillation (low-level jet) as shown by Holton (1967) for nocturnal jets over the Great Plains region of the USA. While it is uncertain how applicable such effects are over southeast Australia, similarities in the sloping topography from the Australian Alps to the adjacent southeast coast suggest that enhancement of the low-level jet through thermal forcing is plausible.
Coupled fire-atmosphere simulations of the Corryong fire (Peace et al. 2021) illustrate key processes intersecting low-level jets, deep boundary layers and extreme fire activity in heavy fuels. Simulations made using the coupled fire–atmosphere ACCESS-Fire (described by Toivanen et al. 2019) show that above the Corryong fire, wind speed in the jet maximum exceeded 70 km h−1 within 1,000 m of the surface (Fig. 3). A time series of the wind at 10-min intervals shows short term variability in wind speed consistent with Blackadar (1957), who suggested fluctuations in the strength of the wind and the height of the wind maximum have a period less than 2 h. Along the horizontal profile in Fig. 3, the elevation of the low-level jet is modified by the contours of the topography and lighter winds (shaded yellow) are evident close to the surface; these features would be expected due to friction and overnight decoupling of the near-surface layer. Less intuitive is the extent to which the trajectory of the low-level jet is modified by the fire plume. Coupled simulations show that the jet maximum is strongly perturbed and located very close to the surface due to being drawn down to the surface into the rear of the fire plume adjacent to the active fire front. Ahead of the fire, the jet is diverted upwards to a height of ~5,000 m by the fire plume. Simulations run without fire–atmosphere coupling do not show these processes.
ACCESS-Fire vertical cross-section of wind through a northwest to southeast transect of the Corryong fire perimeter at 04:10 am on 31 December 2019. Wind speed is shaded according to wind strength (km h−1). The meandering jet of stronger winds between 500 and 2,500 m can be seen clearly above the surface topography. Distance along the horizontal axis is approximately 40 km. Pink and yellow bars over the topography indicate the location of the active fire.
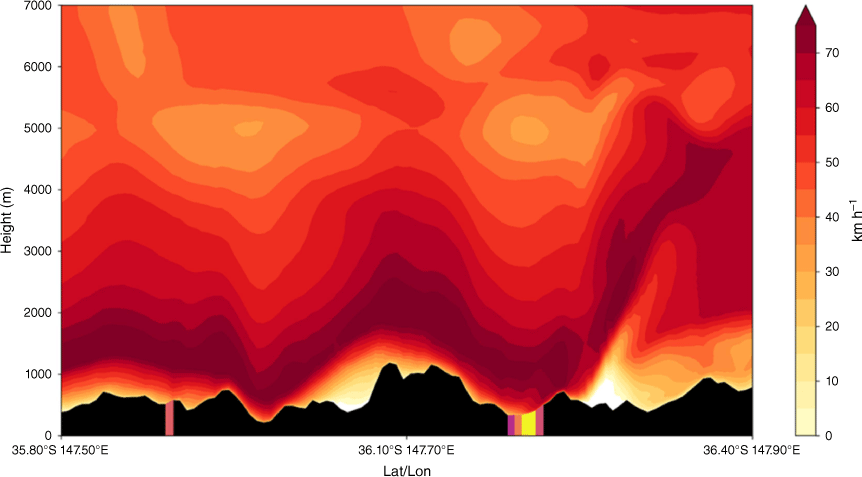
The observed fire spread of 77 km in 27 h, 32 km of which was in the 9-hour window to 01:00 am (Salkin 2022), was due to the fire–atmosphere coupling drawing the stronger elevated winds to the surface to enhance surface fire spread. Simulations show that the depth, elevation and structure of the low-level jet over topography, and the interactions with plume dynamics were critical drivers of overnight fire activity.
Coupled simulations capture the dynamic processes through which fires that generate sufficient energy may develop a plume or convection column that erodes a near-surface inversion and taps into warmer, drier and windier air aloft through mixing and entrainment. Processes observed in the coupled model are consistent with the conceptual model of Potter (2012). The quantum of fuel consumption and heat release ‘sufficient’ to mix through the surface inversion is currently unknown, as are the strength and depth required for an inversion to inhibit mixing. These processes may be investigated further through modelling experiments and lead to techniques suitable for operational application. Understanding the interactions between a fire plume and low-level wind maxima over elevated terrain in deep PBLs is an area of interest to practitioners, and a topic of emerging research in Australia, and it is likely that objective predictive methods will be developed in future.
Additional processes that favour fire behaviour during heatwaves
Deep PBLs during heatwaves are favourable for organised smoke plumes that can be overlain by deep moist convective cloud. Depending on wind and moisture profiles, plumes can become rotating convection columns, and fire generated vortices and deep pyrocumulus or pyroCb cloud may develop, as documented for the Waroona and Green Valley fires (Peace et al. 2017; unpublished report).
A deep PBL is favourable for daytime and overnight spotting processes, including long distance spotting and turbulence-driven mass spotting. Firebrand transport in hot, dry conditions presents an increased likelihood of sustained ignition from embers overnight, rather than self-extinguishing spots that would be expected on a night when relative humidity increases. Ellis (2015) showed the critical role of fuel moisture content and relative humidity on ignition of spot fires.
Fire activity can be suppressed beneath an inversion overnight, however during a heatwave there is typically rapid and early transition of the PBL to a daytime structure; therefore, fire activity will commence earlier in the day and persist well into the evening and possibly throughout the night. Fire danger indices often reach ‘Extreme’ to ‘Catastrophic’ thresholds earlier in the day and remain elevated for a longer window of hours during heatwaves.
Heatwaves are also typically associated with dry boundary layers, therefore ‘dry’ lightning will occur rather than lightning accompanied by significant rainfall (a threshold of >2 mm is used operationally to categorise ‘dry’ and ‘wet’ thunderstorms). Dry lightning increases the probability of strikes resulting in sustained ignitions rather than self-extinguishing ignitions (Dowdy and Mills 2012). After a sequence of days of fuel pre-conditioning during a heatwave, there is potential for rapid and sustained fire perimeter growth from a new ignition.
Operational fire weather
‘Fire weather’ is the term generally used to describe the meteorological conditions favourable for the ignition and spread of a wildfire.
Brown et al. (2015, 2016) describe development of a high spatial and temporal resolution 40-year climatological data set over Victoria using the WRF model. They emphasised the need for bias correction using quantile mapping (QM) to produce an accurate climatology, and implemented a separate equation for each month and each hour to compensate for diurnal and seasonal variation in fire weather. Brown et al. (2016) describe an improved QM approach to improve bias correction in the tails of the distribution (at high wind speed and low relative humidity), to better represent fire weather extremes. They note the importance of detecting extreme events as these are often of most interest. Although their study calculates FFDI, which has since been superseded operationally by AFDRS, their results show that raw, uncorrected numerical weather prediction (NWP) data (in their case from WRF, but the source model is inconsequential) underpredicts fire potential during extreme events. Similar bias correction against observations or historical datasets is performed in some, but not all projections of fire weather regimes applied to climate modelling studies.
The NWP underprediction bias in extreme fire weather conditions is known (particularly to Australian fire weather forecasters) but is not well documented. NWP models use parameterisation schemes to capture boundary layer turbulence at scales finer than the resolution of the modelling grid. These boundary layer turbulence schemes are not calibrated to optimise the mixing processes that occur in the deep PBLs that characterise many extreme fire weather days in Australia. For example, experiments conducted by Lock (2001) to improve parameterisation of convective boundary layers use a PBL of 2 km deep, whereas PBLs reaching 5 km are typical during Australian heatwaves (conversations between the authors and UK Met Office colleagues suggest a greater mixing depth may result in improved verification on very hot, dry days).
In Australia, operational fire weather forecasts provided to fire agencies use weather parameters from NWP in combination with fuel information to calculate a fire weather or fire behaviour index, either at a point or across a spatial grid. Because existing turbulence parameterisations are known to not effectively mix the elevated wind or dry air to the surface when the PBL is very deep, values of maximum and minimum temperature, relative humidity and wind speed are all adjusted prior to input to fire indices. Predictions provided to fire agencies are modified by (1) a statistical bias correction process and, (2) manually by forecasters based on experience, verification, and comparison with observations. These adjustment processes do not feature in the scientific literature but are described in the Australian Bureau of Meteorology ‘Fire Heatwave and Air Quality’ Operational Documents (‘FHAQ Common GFE Edits’ and ‘Standard Operating Procedures’, both of which are ‘living’ e-documents).
The statistical approach to bias correction for temperature almost always results in an increase on both hot days and hot nights. Meteorologists apply a manual post-processing to wind speed and relative humidity (dewpoint temperature) to ‘mix down’ winds from 900, 950 or 850 hPa elevation to increase the surface wind grids. Similarly, surface dewpoint temperature adjustments produce drier conditions than raw model output.
During Black Summer many of the predictions prepared by FBANs under-estimated potential fire behaviour when generated from weather inputs using ADFD grids (the Australian Digital Forecast Database is made available to fire agencies) and Incident Weather Forecasts (IWFs). Both ADFD and IWFs include surface wind fields that assume the formation of a nocturnal temperature inversion and decoupling of near-surface winds. However, as highlighted by the Black Summer examples earlier, this assumption can be contravened when the scale and intensity of fire in the landscape affects the surrounding atmosphere. Fire danger indices derived from climate projections are likely to have a low bias when compared with the current process for modifying raw NWP to generate operational fire weather forecasts because mixing processes through the PBL (with or without the presence of a fire plume) are not included in projections.
Fuel availability and flammability
The flammability and quantity of fuel available to burn in the landscape is determined by fuel dryness. Meteorological processes at a continuum of timescales from hours to days to seasonal conditions (months or longer) alter the availability of fine, coarse, dead and live fuels (Matthews 2015). Dead fine fuels (<6 mm in diameter) including leaves, twigs, sticks and grass accumulated at the surface are generally considered the available fuel for a bushfire and are the primary fuel load to support fire spread (Sullivan et al. 2012). Live fuels include living foliage and branches that do not always burn in a bushfire, but when they do burn, can contribute to increased flame height and support transition from surface fire behaviour to crowning.
Case 1: A fire that made an overnight run and thereby presented significant challenges to fire-fighting agencies and risk to communities. Yanchep fire, Western Australia, 2019 |
The Yanchep fire spread across the coastal plain north of Perth from 11 to 14 December 2019. Perth city (50 km to the south) recorded a heatwave three consecutive days above 40°C on 13–15 December. |
The Yanchep fire zig-zagged up the coast for 3 days. The fire ran westwards under the influence of easterly winds during the morning and overnight periods, then spread to the northeast in the afternoons under the influence of a southwesterly sea breeze. Local meteorology featured hot, dry continental air from the east descending on the 200 m Darling escarpment parallel with the coast. Dry winds advecting from further inland resulted in limited moisture recovery overnight (see Fig. 4). On the second night of the fire (13 December), the coastal town of Two Rocks came under ember attack around 04:00 am. |
Fig. 4. Perth aerological diagram 12/12/2019 at 07:00 am with very hot and dry low levels, limited surface decoupling of wind, indicative of minimal fuel moisture recovery overnight. Right side red line show observed temperature. Left side red line show observed dewpoint temperature. Brown diagonal lines are temperature with height. Brown horizontal axis are constant pressure. Black vertical axis shows height in feet (left side) and km (right side). ![]() |
The 4-day duration of the fire was unusual given the relatively accessible landscape and level of resources committed. A large contingent of Perth firefighting resources were allocated to the blaze, including 180 firefighters on the first night of the fire. Strong winds and limited moisture recovery resulted in active fire spread overnight, hampering suppression activities. |
Heatwaves and drought increase the amount of fuel in the landscape that is available for consumption because live and dead fuels become more easily ignited and flammable. Live plants become dry and physiologically stressed and are therefore more likely to burn. Dead coarse woody fuels >6 mm in diameter also become dry and increasingly available to burn. The processes through which greater consumption of dry woody fuels contributes to deep and persistent flaming is recognised as an important contributor to energy release during major fires (Sullivan et al. 2002), but it is currently poorly understood and presents an opportunity for further research. The significant increase in quantity of live and dead available fuel during drought and heatwave results in increased intensity of a fire, which favours wind convergence around the fire front and therefore development of deep convection columns.
Heatwaves and drought also increase the connectivity of fuel available in the landscape as transient (soft) containment lines such as gullies and watercourses that act as natural fuel breaks in benign fire seasons become dry and may carry fire unimpeded. Drought reduces the effectiveness of these natural fuel breaks as suppression or containment opportunities and supports landscape fire spread. Caccamo et al. (2012) describe a satellite mapping technique to monitor fuel connectivity across the Sydney Basin as an approach to assess the potential for large fires. The lack of soft containment during the Black Summer fires supported fire spread across natural features that would usually be effective strategic and tactical suppression lines where the fire would be expected to slow and become less intense. Wet sclerophyll and riverine forests in Illawarra (south of Sydney) that would typically act as outright barriers to fire spread were described as supporting intense running fires during December 2019 (unpublished report). A return to effective soft containment was observed the following season (2020–21), following moderate amounts of rainfall (pers. comm. with NSW RFS colleagues).
Heatwaves and fuel availability
Heatwaves affect the diurnal cycle of temperature and relative humidity. Fuel moisture content (FMC) responds to the hot, dry atmosphere, though reduced overnight fuel moisture recovery and cumulative drying can occur over successive hot days and nights (Jyoteeshkumar reddy et al. 2021). During heatwave conditions, the fuel moisture of extinction at which fire propagation ceases is unlikely to be reached overnight.
Sullivan and Matthews (2013) examined spatial and temporal variation in FMC for the Black Saturday Kilmore East fire. They used empirical (point based) models, as no direct measurements of FMC were taken during the fires run. Their fig. 5 shows modelled FMC over the period 6–8 February; the overnight FMC prior to the fire remained below 15% (13–14%) and the afternoon minimum was around 4%. FMC of 5% and below are associated with erratic and severe fire behaviour in eucalypt forests, including crowning and mass spotting (Sullivan et al. 2012). They investigated the impact of the heatwave on FMC by making comparative calculations for a heatwave and for more typical moderate weather. The difference between the two sets of conditions is dramatic (their fig. 9); without a heatwave the overnight FMC is 20–25% and daytime FMC is 10–15%, but with a heatwave, overnight FMC is ~10% and daytime FMC around 5%. The experiments of Sullivan and Matthews (2013) were limited and there is an opportunity for further field measurements of diurnal variation in FMC to validate models in the overnight hours.
Their discussion highlights other relevant processes including the heatwave increasing the total amount of fuel available for flaming combustion due to the long-term impact on the moisture content of live fuels (shrubs and other understorey vegetation) as well as coarser dead fuels (logs and branches). To date, climate projections have not considered live fuel moisture content and live to dead fuel ratios, and their contribution as drivers of future fire regimes remains uncertain.
Groom et al. (2004) examined heat damage in mallee-heathland following a heatwave over southern Western Australia in 1991. They monitored death of native shrub and tree species resulting from a combination of severe drought stress and temperatures over 45°C. Plants that had died recently were assumed to have been killed as a result of the heatwave. Their observations capture some of challenges in attributing plant damage and mortality to environmental factors; not all species demonstrate the same degree of leaf damage when subjected to a given period of heat stress; thicker leaves are more heat tolerant than thinner leaves; wind exposure and degree of shading were the most important variables influencing degree of leaf damage; identifying the effects of extreme heat in the field is difficult since a combination of factors may contribute to the observed injury; and damaged and undamaged species may be a result of pre-conditioning, with species in more exposed habitats being pre-adapted to tolerate periods of sustained heat stress.
Gazol and Camarero (2022) identified hotspots of forest mortality in Europe and demonstrated the association between simultaneous occurrence of extreme droughts and heatwaves, with rare compound climate events of hot summers and dry years triggering tree mortality in Europe over the past three decades. Similar to Groom et al. (2004), they note that it is difficult to investigate the contributing drivers, as tree mortality can occur with a lagged response to drought occurrence. Gazol and Camarero (2022) conclude that since summer temperatures and the frequency of droughts are rising, more forests will become vulnerable to compound climate extremes and tree mortality will become a more important driver of forest dynamics. Vegetation responses to heatwave and drought will also be mediated strongly by local site factors linked to soil depth, texture and water-holding capacity (McGrath et al. 2023).
Case 2: A fire that made an overnight run and thereby presented significant challenges to fire-fighting agencies and risk to communities. Wooroloo Fire, Western Australia, 2021 |
The Wooroloo fire burned along the elevated scarp northeast of Perth on 1–5 February 2021 burning 11,000 ha and destroying 86 properties. The final fire perimeter of 122 km was elongated and narrow, consistent with persistent easterly winds over the several days and nights, and no afternoon sea breeze influence. The highest values of FFDI during the event were recorded on the first day of the fire. Fig. 5 shows two FFDI peaks at Pearce on 1 February, one mid-afternoon and a secondary peak late in the evening. This is an example of the diurnal variability that can occur in FFDI. An extended period of hot, dry and windy conditions resulted in 12 h of FFDI >40 and favourable conditions for spot fires. This supported rapid growth of an initial large fire perimeter which then took several days to contain. |
Drought and heatwaves are interrelated processes that increase fuel available for combustion in the landscape. Climatic processes that drive drought also produce conditions favourable for heatwaves. As heatwaves and drought typically occur either simultaneously or sequentially, the respective impacts on fuel availability and fire activity are difficult to resolve, particularly as field observations are difficult to make and costly to collect. In their study of droughts and heatwaves in the Western Mediterranean, Guion et al. (2022) found that heatwaves that occur at the same time as droughts are significantly more intense. The cascading effects of compound heatwave and drought are described by Libonati et al. (2022) for the extreme 2020 fire season in the Pantanal, Brazil. They point out that the soil moisture deficits change sensible heat flux between the surface and the atmosphere, resulting in re-amplification of the near-surface temperatures.
The flammability of drought and heatwave-affected vegetation is a large uncertainty in fuel availability and therefore fire regimes under climate change. Motivated by the need to increase understanding of climate–fire–vegetation interactions, Nolan et al. (2020) provide a detailed discussion on the response of plant physiology and forest flammability to drought, concluding that physiological drought stress and subsequent tree mortality may increase the probability of wildfires through multiple mechanisms including leaf cavitation and shedding, and alterations to forest structure, ratio of dead to live fuels in the canopy and the amount of litter on the forest floor. They point out that drought-induced tree mortality is associated with a more open canopy, which affects the microclimate of the forest floor, increasing temperature and VPD and hence the rate of drying of the understorey and litter fuels. Also, when dead fuels are shed from the canopy, the litter added to the surface bed will initially be uncompacted and well aerated, favouring ease of ignition and rapid combustion. Field observations from eucalypt forests affected by drought and heatwave are consistent with these predictions (Ruthrof et al. 2016).
Although it is well understood that dry fuel conditions create flammable landscapes, predictions from fire spread models used operationally in Australia (Cruz et al. 2015) are less certain under very dry conditions. In part, this is a consequence of the range of conditions experimental burns were conducted in and the difficulty in taking measurements under more severe burning conditions. The challenges are exacerbated by the actuality that events such as Black Summer redefine historical benchmarks of soil and fuel dryness. In order to anticipate fire behaviour under extremely dry conditions, options may include development of a more open-ended drought factor to account for greater consumption of woody fuel (and its contribution to flame zone depth), and consumption of canopy fuels.
Drought impacts are dissimilar across different vegetation regimes; seasonal grass fuel loads tend to be low and often discontinuous due to lack of rainfall. The fire behaviour response in grass fuels can be complex, with fast-moving fire still possible under extreme weather conditions despite low fuel loads.
Research on vegetation changes in Australia under future climates has been limited, a deficit that is understandable due to challenges and complexities linked to climate variability and continental scale (Rifai et al. 2022). Vegetation and land cover as well as representation of fire processes are therefore a source of significant uncertainty in climate simulations (e.g. Rabin et al. 2017 in their description of the Fire Model Intercomparison Project (FireMIP)) and assumptions of current land use persisting are open to question. Li et al. (2022) state that land surface models are often unable to predict the response of vegetation to environmental conditions with sufficient accuracy, especially under stressful conditions such as drought.
Heatwaves in a future climate
Over the coming decades, Australia is projected to experience continued warming, with more extremely hot days and fewer extremely cool days. Temperature thresholds for a heatwave vary with local climatology, but typical Australian conditions are for a period of three or more days with maximum temperatures in the low to mid 40°Cs and very low relative humidity, with dewpoint temperature in low single digits or negative values.
There is no universal definition of atmospheric heatwaves (Perkins-Kirkpatrick et al. 2016) with Perkins and Alexander (2013) describing heatwaves as ambiguous and inconsistent. Most definitions consider both daytime and night-time temperatures and some, but not all incorporate dewpoint temperature (relative humidity). Most focus on summer conditions, while others consider anomalous heat during winter. Irrespective of calculation method, ominous and accelerating trends are clear in climate projections for heatwave duration, frequency, spatial extent and intensity in Australia and globally (Domeisen et al. 2023; Perkins-Kirkpatrick et al. 2016 and references therein). Philip et al. (2022) show that the June 2021 heatwave in the Pacific northwest areas of the USA and Canada shattered temperature records over a very large area and caused over 1,000 heat-related excess deaths. This event was regarded as virtually impossible without human-induced climate change.
Cowan et al. (2014) examined projected changes in Australian heatwaves using CMIP5 for future trends in summer heatwaves and winter warm spells using the heatwave calculation from Pezza et al. (2012). They identified an increase in summer heatwave frequency (number of heatwave days in a summer), increased heatwave duration (number of days and nights the heatwave lasts) and increased heatwave amplitude (the anomaly of the hottest day of the hottest summer heatwave will increase). In summary, the hottest heatwave events will become increasingly hotter by the end of the century, even when referenced to a warming climate.
The analysis of Cowan et al. (2014) indicates that more frequent, hotter, and longer summer heatwaves will occur by the end of the 21st century. For RCP4.5 the heatwave duration will increase by ~2 days and the heatwave frequency will increase by 5–10 days per summer across southern Australia (from a historical 1–2 days per summer). Under the more extreme RCP8.5 scenario, the frequency increases by 10–20 days per summer. They conclude that summer heatwaves and winter warm spells will increase in frequency, duration and amplitude across Australia. This is consistent with Deng et al. (2022), who use the more recent CMIP6 models and summarise their detailed analysis as a ‘warm gets warmed’ pattern over Australia. The warming trend has implications for increased availability of dead fuels and increased flammability of live vegetation, factors that are extremely difficult to test through experimental methods, particularly at a landscape scale.
Drought in a future climate
Delage and Power (2020) use CMIP5 climate models to examine the frequency of droughts. Their analysis shows that the frequency of droughts, including droughts in consecutive winter–spring seasons, is projected to increase in the 21st century under RCP8.5 scenarios. Their analysis focusses on the months of June to November, as the winter–spring precipitation period is important for agricultural production and reservoir management.
In most Australian locations, generally drier conditions are projected to be infrequently punctuated by seasons that are just as wet or wetter than the wettest years experienced during the 20th century. Time in drought in southern Australia is projected to increase, with a decline in mean rainfall, and the nature of droughts is projected to change with a greater frequency of extreme droughts.
During the 20th century, 3-year droughts occurred very infrequently and 5- and 6-year droughts did not occur at all. Projections for the 21st century indicate an increased frequency of 1–4 year droughts and the emergence of 5- and 6- year droughts. The greatest increase in drought frequency is for parts of southwestern Australia and central southern South Australia (fig. 4 of Delage and Power 2020).
Falster et al. (2024) explicitly recognise that the observational drought record is short relative to timescales of hydroclimatic variability. Their 1,000-year simulations showed that the longest Australian droughts in the pre-industrial period are much longer than the longest droughts of the 20th century and that droughts in the period 1900–2000 are within the bounds of pre-industrial natural variability in terms of intensity, severity, and frequency. They conclude that future anthropogenic forcings will lead to significantly longer and more intense droughts than experienced during the 20th century.
The Pacific and Indian Ocean climate variability modes of El Niño and La Niña, the Indian Ocean Dipole and to a lesser extent the Southern Annular Mode are key drivers of rainfall over southern Australia. King et al. (2020) state that there is uncertainty in climate projections resulting from model deficiencies predicting how the Indian Ocean Dipole and El Nino Southern Oscillation (ENSO) will change in the future; these uncertainties flow through to uncertainties in future drought conditions.
There is an ominous consistency from almost all global and regional climate models, that southwestern Australia will tend to be drier in future. Rainfall reconstructions based on tree ring records indicate that over the past 350–700 years there have been multi-decadal periods of quite dry conditions, and some shorter periods of very dry conditions including in the 1880s and 1890s. Rainfall decline is generally identified from the mid-1970s onwards, with an intensification after 2000 (Zheng et al. 2021). In the context of human-induced climate change, this is likely to mean increased rainfall variability, and the likelihood that future dry phases may be amplified in comparison to past events. Amplification of dry periods could trigger vegetation-dependent changes in fuel availability which are not included in current projections.
PyroCb in a future climate
Peterson et al. (2021) documented the outbreak of pyroCb (Fromm et al. 2006) that developed on 30 and 31 December 2019 and dubbed it the ‘Australian New Year Super Outbreak’. This outbreak represents the largest number of pyroCbs (38) recorded during a fire event (spatially and temporally defined) and included anomalous intense nocturnal pyroCb activity. They characterise the Australian New Year outbreak and the June 2021 event in south-west British Columbia as representing a new class of stratospheric smoke plumes with the potential for significant climate feedback on seasonal and hemispheric scales.
Peterson et al. (2021) state for the future to ‘expect a higher frequency and larger magnitude of pyroCb outbreaks’. They highlight uncertainties in the climate system and that research is required to continue to explore the role of pyroCb activity to consolidate the links between the mechanisms driving extreme pyroCb outbreaks and their potential interactions with a warming climate. Fromm et al. (2022) provide a global overview and identify important directions for further research, but caution against attributing a trend of increased pyroCb activity without robust evidence.
Dowdy et al. (2019) used C-Haines to find an increased risk of dangerous atmospheric conditions associated with extreme fire weather and pyroconvection for many regions of southern Australia in future climate regimes. Di Virgilio et al. (2019) compared the spring and summer periods 2060–79 with 1990–2009 using regional climate models and observations respectively and showed an increase in the number of days with conditions conducive to pyroCb development. Following the studies of Peterson et al. (2021), Di Virgilio et al. (2019) and Dowdy et al. (2019) and noting the uncertainties, pyroCb are more likely with large fires with long perimeters (due to high heat release) and large fires are more likely with heatwaves due to favourable conditions for nocturnal fire activity. Thus, future climates tend towards conditions favourable for greater pyroCb activity during the day, as well as at night.
Managing impacts – fire suppression resources and community messaging
The Black Summer fires posed major challenges for response agencies, governments and affected communities, and these have been documented and analysed at length by post-fire inquiries (Inspector-General for Emergency Management 2020; NSW Bushfire Inquiry 2020). In the following section we briefly examine implications of increased heatwave effects and nocturnal fire activity of operational firefighting and community messaging. Our insights mainly flow from conversations with practitioner colleagues rather than from formal scientific literature.
Nocturnal fire activity presents a hazard to communities as vulnerability increases from day to night. Exposure increases at night as people are more likely to be present in places and settings where they may be adversely affected; during the day people are more likely to be in towns, and children at school. Capacity to respond is diminished at night and warning messages may be less effective if people are asleep and evacuation support is impeded. Community evacuations at night present additional risks through limited visibility and fatigue, as well as the difficulty of managing and relocating animals in the dark, particularly large animals such as horses and cattle. Nocturnal fire activity also has environmental implications for fauna as animals may be unable to escape as fire perimeters expand day and night.
Operational firefighting presents challenges overnight; fire agencies often roster their more experienced incident management teams during the day, when fire activity is expected to be worse, and less experienced teams overnight in anticipation of them dealing with reduced fire activity. The future conundrum is that less experienced teams may be dealing with extreme fire behaviour in the dark when risks are higher due to reduced visibility and communications. In addition, operational staffing is usually reduced overnight, with lessened capacity to provide immediate logistical and tactical support. Aircraft are often unable to operate overnight, especially when winds are strong, restricting the opportunity for airborne mapping to enhance intelligence for aerial fire suppression.
Heatwave impacts on human health will be experienced by firefighters as well as community members. For firefighters, the consequences of fires during heatwaves will be longer days requiring active suppression activities and reduced rest. Physical and physiological response to heatwaves include heat stress and fatigue with impeded physical recovery and impeded sleep from deployments in temporary accommodation with no air-conditioning. These effects may be amplified by the trend towards an aging population of volunteers.
Greater firefighting resources will be required for future fires that burn day and night for consecutive days during heatwaves. Emergency resources will be stretched by episodes of extreme fire behaviour during the day and prolonged multiday campaign events. These future conditions will require changes in planning and preparedness as resource requirements needed to respond to multi-day events and overnight fire activity differ to those for single day events. Other potential considerations during periods of extreme heat may be reduced reliability of radio and other telecommunications and damage to vehicles, generators and other infrastructure through overheating. Heatwaves also impact agricultural activities, can damage roads and rail networks, and increase demand on utilities, particularly electricity.
A surge in research on compound extreme events globally is reported on by Afroz et al. (2023). For Australia, the cascade of risks presented by extreme heatwaves and fires present potential for compounding emergencies. The synoptic weather patterns that are favourable for heatwaves over southern Australia, with a near stationary high-pressure system positioned over the Tasman Sea are also favourable for the development of tropical cyclones over northern Australia. Simultaneous or concurrent fires, heatwaves and tropical cyclones will further stretch the resources of emergency response agencies. The experience of responders during the Black Summer was that the scaling of the resource response was highly non-linear. This is likely to be the case during future events, particularly if more areas (nationally and internationally) are affected sequentially or concurrently, reducing opportunities for resource sharing across jurisdictions.
Concluding comments
This paper has described some of the processes that will drive increased fire activity under likely future climate scenarios. We provide examples, including fires during the Black Summer, to illustrate why projections in the IPCC reports are likely to underestimate fire extent and intensity in the future. Jones et al. (2022) conclude that climate change is exerting globally a pervasive upwards pressure on fire activity through its impacts on fire weather, and this upwards pressure will escalate with each increment of global warming. We have demonstrated that climate projections of future fire regimes calculated using daily maxima of surface-based fire indices are likely to underpredict the extent and impact of future fires, because the approach does not capture fire behaviour response to dynamic factors including: diurnal atmospheric processes during heatwaves, fuel availability and vegetation response to drought and heatwaves, and enhanced fire behaviour driven by interactions between the fire, the PBL and terrain.
A focus of this paper has been the impact of PBL wind on fire spread. Wind maxima associated with low-level jets and other mesoscale or synoptic mechanisms in the PBL including prefrontal jets and baroclinic instability producing thermally driven winds can reach 50–100 km h−1 or more. Elevated winds interacting with a fire plume may drive forward spread rates of 5–15 km h−1 sustained over several hours depending on fuel type. Most response agencies and communities are unprepared for events of this nature.
The response of vegetation to climate change is a topic of considerable uncertainty. However, the assumption made in climate projections, that current conditions will persist, is likely to underestimate fuel availability in the future. More frequent heatwaves will result in extended periods favourable for active fire spread and therefore larger fires. This will be compounded by high dead and live fuel availability over contiguous areas due to intensity and duration of drought and heatwaves. More intense fires will result from fire–atmosphere interactions in deeper boundary layers and heavy fuel loads.
As the climate changes, fire-prone landscapes will expand from historical ranges. This has already been experienced during the Queensland fires in 2018 and 2019, and the Amazon fires in 2019 when rainforests burned following extended drought. In addition to the ecological consequences to non-fire adapted species, expansion of fire ranges means that fires will impact communities with no lived experience of fire and limited resources for mitigating impacts. In the NSW, 2,488 homes burned during the Black Summer, with 14,519 homes saved owing to firefighting protective measures (Black Summer bushfires, NSW, 2019–20 (aidr.org.au)). Fires expanding into communities with no prior fire experience, without the firefighting resources, training and coordination of established fire brigades, and without community and household preparedness, are more likely to result in catastrophic consequences and extensive house loss.
There is benefit in preparing communities and fire practitioners for a changing future. Communities will continue to undergo demographic shifts and are expected to become more vulnerable to hazards. Communities are also expected to experience warning fatigue from multiple hazards. Therefore, contextual and tailored ongoing engagement and education on hazards and risk will assist preparedness and response.
Fire agencies and practitioners will require training on learnings from recent events and scientific investigations, as lived experience may not be an adequate benchmark for future fire behaviour. Training may include emphasis on the hours favourable for spread, in operations and briefings, as well as identifying situations where extreme fire behaviour may be driven by fire–atmosphere interactions.
We need research with outcomes that focus on accurate real-time fire predictions, and leverage verification options and contextual warnings. These may include quantitative methods for overnight fire spread, metrics for assessing wind mixing and plume entrainment, methodologies for predicting fire response over complex terrain, and inclusion of fire dynamics processes in climate projections in addition to attempts to quantify changes and uncertainties of vegetation and fuel structure under future drought and heatwave regimes.
Sharing information, experience and learnings across the global fire community will assist in facing future challenges. The experience from the Black Summer is that current procedures and capabilities do not effectively scale to natural hazards that are amplified by climate change.
Data availability
All data utilised in this manuscript are fully available via the website links and references cited in the reference list.
Conflicts of interest
Dr Lachlan McCaw is an editor of International Journal of Wildland Fire. To mitigate this potential conflict of interest he was blinded from the peer review process and was not involved at any stage in the editing of this manuscript. The authors declare no other conflicts of interest.
Declaration of funding
This research was funded by Australian Climate Services and draws heavily on earlier research funded by Bushfire and Natural Hazards CRC.
Acknowledgements
The authors are grateful to Dr Tim Brown of the Desert Research Institute and Dr Tony Hirst of the Bureau of Meteorology for comments on a draft of the manuscript. We also thank the Associate Editor and two anonymous reviewers for making constructive comments that improved the manuscript.
References
Abram NJ, Henley BJ, Sen Gupta A, Lippmann T, Clarke H, Dowdy A, Sharples J, Nolan R, Zhang T, Wooster M, Wurtzel J, Meissner K, Pitman A, Ukkola A, Murphy B, Tapper N, Boer M (2021) Connections of climate change and variability to large and extreme forest fires in southeast Australia. Communications Earth & Environment 2, 8.
| Crossref | Google Scholar |
Afroz M, Chen G, Anandhi A (2023) Drought and heatwave associated compound extremes: a review of hotspots, variables, parameters, drivers, impacts, and analysis frameworks. Frontiers in Earth Science 10, 914437.
| Crossref | Google Scholar |
AghaKouchak A, Huning LS, Sadegh M, Qin Y, Markonis Y, Vahedifard F, Love CA, Mishra A, Mehran A, Obringer R, Hjelmstad A, Pallickara S, Jiwa S, Hanel M, Zhao Y, Pendergrass AG, Arabi M, Davis SJ, Ward PJ, Svoboda M, Pulwarty R, Kreibich H (2023) Toward impact-based monitoring of drought and its cascading hazards. Nature Reviews Earth & Environment 4(8), 582-595.
| Crossref | Google Scholar |
Balch JK, Abatzoglou JT, Joseph MB, Koontz MJ, Mahood AL, McGlinchy J, Cattau ME, Williams AP (2022) Warming weakens the night-time barrier to global fire. Nature 602, 442-448.
| Crossref | Google Scholar | PubMed |
Blackadar AK (1957) Boundary layer wind maxima and their significance for the growth of nocturnal inversions. Bulletin of the American Meteorological Society 38, 283-290.
| Crossref | Google Scholar |
Brown T, Mills G, Harris S, Podnar D, Reinbold H, Fearon M (2015) A fire weather climatology dataset for Victoria. Final Report to the Department of Environment, Water, Land and Planning. 129 pp. Available at http://cefa.dri.edu/Publications/publications_home.php [verified 9 November 2023]
Brown T, Mills G, Harris S, Podnar D, Reinbold H, Fearon M (2016) A bias corrected WRF mesoscale fire weather dataset for Victoria, Australia 1972-2012. Journal of Southern Hemisphere Earth Systems Science 66, 281-313.
| Crossref | Google Scholar |
Bureau of Meteorology (2020) Special climate statement 73 – extreme heat and fire weather in December 2019 and January 2020. Available at http://www.bom.gov.au/climate/current/statements/scs73.pdf
Caccamo G, Chisholm LA, Bradstock RA, Puotinen ML (2012) Using remotely‐sensed fuel connectivity patterns as a tool for fire danger monitoring. Geophysical Research Letters 39, L01302.
| Crossref | Google Scholar |
Chiodi AM, Potter BE, Larkin NK (2021) Multi-decadal change in Western US nighttime vapor pressure deficit. Geophysical Research Letters 48, e2021GL092830.
| Crossref | Google Scholar |
Clabo D (2022) Why are we still using the Haines Index? Available at https://youtu.be/zpwgxMMzjO4, https://youtu.be/BuiKqm9hP7s at https://wildfiretoday.com/2022/06/10/why-are-we-still-using-the-haines-index [verified 9 November 2023]
Clarke H, Evans JP (2019) Exploring the future change space for fire weather in southeast Australia. Theoretical and Applied Climatology 136, 513-527.
| Crossref | Google Scholar |
Copernicus Report (2022) European State of the Climate Summary 2022. Available at https://climate.copernicus.eu/sites/default/files/custom-uploads/ESOTC2022/PR/ESOTCsummary2022_final.pdf [verified 9 November 2023]
Cowan T, Purich A, Perkins S, Pezza A, Boschat G, Sadler K (2014) More frequent, longer, and hotter heatwaves for Australia in the twenty-first century. Journal of Climate 27, 5851-5871.
| Crossref | Google Scholar |
Cruz MG, Gould JS, Alexander ME, Sullivan AL, McCaw WL, Matthews S (2015) Empirical-based models for predicting head-fire rate of spread in Australian fuel types. Australian Forestry 78, 118-158.
| Crossref | Google Scholar |
Dee DP, Uppala SM, Simmons AJ, Berrisford P, Poli P, Kobayashi S, Andrae U, Balmaseda MA, Balsamo G, Bauer P, Bechtold P, Beljaars ACM, van de Berg L, Bidlot J, Bormann N, Delsol C, Dragani R, Fuentes M, Geer AJ, Haimberger L, Healy SB, Hersbach H, Hólm EV, Isaksen L, Kållberg P, Köhler M, Matricardi M, McNally AP, Monge‐Sanz BM, Morcrette J-J, Park B-K, Peubey C, de Rosnay P, Tavolato C, Thépaut J-N, Vitart F (2011) The ERA-Interim reanalysis: configuration and performance of the data assimilation system. Quarterly Journal of the Royal Meteorological Society 137, 553-597.
| Crossref | Google Scholar |
Delage PD, Power SB (2020) The impact of global warming and the El Niño‑Southern Oscillation on seasonal precipitation extremes in Australia. Climate Dynamics 54, 4367-4377.
| Crossref | Google Scholar |
Deng X, Perkins-Kirkpatrick SE, Alexander LV, Stark C (2022) Projected changes and time of emergence of temperature extremes over Australia in CMIP5 and CMIP6. Earth’s Future 10, e2021EF002645.
| Crossref | Google Scholar |
Di Virgilio G, Evans JP, Blake SAP, Armstrong M, Dowdy AJ, Sharples J, McRae R (2019) Climate change increases the potential for extreme wildfires. Geophysical Research Letters 46, 8517-8526.
| Crossref | Google Scholar |
Domeisen DIV, Eltahir EAB, Fischer EM, Knutti R, Perkins-Kirkpatrick SE, Schär C, Seneviratne SI, Weisheimer A, Wernli H (2023) Prediction and projection of heatwaves. Nature Reviews Earth & Environment 4, 36-50.
| Crossref | Google Scholar |
Dowdy AJ (2018) Climatological variability of fire weather in Australia. Journal of Applied Meteorology and Climatology 57, 221-234.
| Crossref | Google Scholar |
Dowdy AJ (2020) Seamless climate change projections and seasonal predictions for bushfires in Australia. Journal of Southern Hemisphere Earth Systems Science 70, 120-138.
| Crossref | Google Scholar |
Dowdy AJ, Mills GA (2012) Atmospheric and fuel moisture characteristics associated with lightning-attributed fires. Journal of Applied Meteorology and Climatology 51, 2025-2037.
| Crossref | Google Scholar |
Dowdy AJ, Ye H, Pepler A, Thatcher M, Osbrough SL, Evans JP, DiVirgilio G, McCarthy N (2019) Future changes in extreme weather and pyroconvection risk factors for Australian wildfires. Nature. Scientific Reports 9, 10073.
| Crossref | Google Scholar | PubMed |
Ellis PFM (2015) The likelihood of ignition of dry-eucalypt forest litter by firebrands. International Journal of Wildland Fire 24, 225-235.
| Crossref | Google Scholar |
Falster GM, Wright NM, Abram NJ, Ukkola AM, Henley BJ (2024) Potential for historically unprecedented Australian droughts from natural variability and climate change. Hydrology and Earth System Sciences 28, 1383-1401.
| Crossref | Google Scholar |
Freeborn PH, Jolly MW, Cochrane MA, Roberts G (2022) Large wildfire driven increases in nighttime fire activity observed across CONUS from 2003–2020. Remote Sensing of Environment 268, 112777.
| Crossref | Google Scholar |
Fromm MD, Tupper A, Rosenfeld D, Servranckx R, McRae R (2006) Violent pyro-convective storm devastates Australia’s capital and pollutes the stratosphere. Geophysical Research Letters 33, L05815.
| Crossref | Google Scholar |
Fromm M, Servranckx R, Stocks BJ, Peterson DA (2022) Understanding the critical elements of the pyrocumulonimbus storm sparked by high-intensity wildland fire. Communications Earth and Environment 3, 243.
| Crossref | Google Scholar |
Gazol A, Camarero JJ (2022) Compound climate events increase tree drought mortality across European forests. Science of The Total Environment 816, 151604.
| Crossref | Google Scholar | PubMed |
Groom PK, Lamont BB, Leighton S, Leighton P, Burrows C (2004) Heat damage in sclerophylls is influenced by their leaf properties and plant environment. Ecoscience 11, 94-101.
| Crossref | Google Scholar |
Guion A, Turquety S, Polcher J, Pennel R, Bastin S, Arsouze T (2022) Droughts and heatwaves in the Western Mediterranean: impact on vegetation and wildfires using the coupled WRF-ORCHIDEE regional model (RegIPSL). Climate Dynamics 58, 2881-2903.
| Crossref | Google Scholar |
Haines DA (1988) A lower atmospheric severity index for wildland fires. National Weather Digest 13, 23-27.
| Google Scholar |
Harris S, Lucas C (2019) Understanding the variability of Australian fire weather between 1973 and 2017. PLoS One 14, e0222328.
| Crossref | Google Scholar | PubMed |
Hersbach H, Bell B, Berrisford P, Hirahara S, Horányi A, Muñoz-Sabater J, et al. (2020) The ERA5 global reanalysis. Quarterly Journal of the Royal Meteorological Society 146(730), 1999-2049.
| Crossref | Google Scholar |
Hollis JJ, Matthews S, Fox-Hughes P, Grootemaat S, Heemstra S, Kenny BJ, Sauvage S (2024) Introduction to the Australian Fire Danger Rating System. International Journal of Wildland Fire 33, WF23140.
| Crossref | Google Scholar |
Holton JR (1967) The diurnal boundary layer wind oscillation above sloping terrain. Tellus 19, 199-205.
| Crossref | Google Scholar |
Inspector-General for Emergency Management (2020) Inquiry into the 2019–20 Victorian fire season. Phase 1 Community and sector preparedness for and response to the 2019–20 fire season. Available at https://www.igem.vic.gov.au/publications/publications/inquiry-into-the-2019-20-victorian-fire-season-phase-1-reportInspector-General for Emergency Management, Victorian Government [verified 28 December 2023]
IPCC (2023) Climate Change 2023: Synthesis Report. In ‘Contribution of Working Groups I, II and III to the Sixth Assessment Report of the Intergovernmental Panel on Climate Change’. (Eds Core Writing Team, H Lee, J Romero) pp. 35–115. (IPCC: Geneva, Switzerland) 10.59327/IPCC/AR6-9789291691647
Jones MW, Abatzoglou JT, Veraverbeke S, Andela N, Lasslop G, Forkel M, Smith AJP, Burton C, Betts RA, van der Werf GR, Sitch S, Canadell JG, Santín C, Kolden C, Doerr SH, Le Quéré C (2022) Global and regional trends and drivers of fire under climate change. Reviews of Geophysics 60(3), e2020RG000726.
| Crossref | Google Scholar |
Jyoteeshkumar reddy P, Sharples JJ, Lewis SC, Perkins-Kirkpatrick SE (2021) Modulating influence of drought on the synergy between heatwaves and dead fine fuel moisture content of bushfire fuels in the Southeast Australian region. Weather and Climate Extremes 31, 100300.
| Crossref | Google Scholar |
King AD, Pitman AJ, Henley BJ, Ukkola AM, Brown JR (2020) The role of climate variability in Australian drought. Nature Climate Change 10, 177-179.
| Crossref | Google Scholar |
Krikken F, Lehner F, Haustein K, Drobyshev I, van Oldenborgh GJ (2021) Attribution of the role of climate change in the forest fires in Sweden 2018. Natural Hazards Earth Systems Science 21, 2169-2179.
| Crossref | Google Scholar |
Li X, Xi B, Wu X, Choat B, Feng J, Jiang M, Tissue D (2022) Unlocking drought-Induced tree mortality: physiological mechanisms to modeling. Frontiers in Plant Science 13, 835921.
| Crossref | Google Scholar | PubMed |
Libonati R, Geirinhas JL, Silva PS, et al. (2022) Assessing the role of compound drought and heatwave events on unprecedented 2020 wildfires in the Pantanal. Environmental Research Letters 17, 015005.
| Crossref | Google Scholar |
Lim E, Hendon H, Butler A, Thompson D, Lawrence Z, Scaife A, Shepherd T, Polichtchouk I, Nakamura H, Kobayashi C, Comer R, Coy L, Dowdy A, Garreaud R, Newman P, Wang G (2021) The 2019 Southern Hemisphere stratospheric polar vortex weakening and its impacts. Bulletin of the American Meteorological Society 102, E1150-E1171.
| Crossref | Google Scholar |
Lock AP (2001) The numerical representation of entrainment in parameterizations of boundary layer turbulent mixing. Monthly Weather Review 129, 1148-1163.
| Crossref | Google Scholar |
Luo K, Wang X, de Jong M, Flannigan M (2024) Drought triggers and sustains overnight fires in North America. Nature 627, 321-327.
| Crossref | Google Scholar | PubMed |
Markowski P, Richardson Y (2010) The nocturnal low-level wind maximum. In ‘Mesoscale Meteorology in Midlatitudes’. pp. 73–114. Chapter 4. eBook Academic Collection. (EBSCO Publishing, John Wiley & Sons, Ltd) 10.1002/9780470682104.ch4
Matthews S (2015) Dead fuel moisture research: 1991–2012. International Journal of Wildland Fire 23, 78-92.
| Crossref | Google Scholar |
McGrath G, Fontaine JB, Van Dongen R, Hyde J, Leopold M, Matusick G, Ruthrof KX (2023) Geophysics reveals forest vulnerability to drought. Ecohydrology 16, e2596.
| Crossref | Google Scholar |
Mills G, Salkin O, Fearon M, Harris S, Brown T, Reinbold H (2022) Meteorological drivers of the eastern Victorian Black Summer (2019–2020) fires. Journal of Southern Hemisphere Earth Systems Science 72, 139-163.
| Crossref | Google Scholar |
Nairn J, Fawcett R (2014) The excess heat factor: a metric for heatwave intensity and its use in classifying heatwave severity. International Journal of Environmental Research and Public Health 12, 227-53.
| Crossref | Google Scholar | PubMed |
Nolan RH, Blackman CJ, de Dios VR, Choat B, Medlyn BE, Li X, Bradstock RA, Boer MM (2020) Linking forest flammability and plant vulnerability to drought. Forests 11, 779.
| Crossref | Google Scholar |
NSW Bushfire Inquiry (2020) Final report of the NSW Bushfire Inquiry. Government of New South Wales. Available at https://www.nsw.gov.au/sites/default/files/noindex/2023-06/Final-Report-of-the-NSW-Bushfire-Inquiry.pdf [verified 28 December 2023]
Patterson M, Bracegirdle T, Woollings T (2019) Southern Hemisphere atmospheric blocking in CMIP5 and future changes in the Australia-New Zealand Sector. Geophysical Research Letters 46, 9281-9290.
| Crossref | Google Scholar |
Peace M, McCaw L, Santos B, Kepert JD, Burrows N, Fawcett RJB (2017) Meteorological drivers of extreme fire behaviour during the Waroona bushfire, Western Australia, January 2016. Journal of Southern Hemisphere Earth Systems Science 67(2), 79-106.
| Crossref | Google Scholar |
Peace M, Hanstrum B, Greenslade J, Zovko-Rajak D, Santra A, Kepert J, Fox-Hughes P, Ye H, Shermin T, Jones J (2021) Coupled fire-atmosphere simulations of five Black Summer fires using the ACCESS-Fire model - Black Summer final report. (Bushfire and Natural Hazards Cooperative Research Centre: Melbourne, Vic.)
Perkins SE, Alexander LV (2013) On the measurement of heatwaves. Journal of Climate 26, 4500-4517.
| Crossref | Google Scholar |
Perkins-Kirkpatrick SE, White CJ, Alexander LV, Argüeso D, Boschat G, Cowan T, Evans JP, Ekström M, Oliver ECJ, Phatak A, Purich A (2016) Natural hazards in Australia: heatwaves. Climatic Change 139, 101-114.
| Crossref | Google Scholar |
Peterson DA, Fromm MD, McRae RHD, Campbell JR, Hyer EJ, Taha G, Camacho CP, Kablick GP, Schmidt CC, DeLand MT (2021) Australia’s Black Summer pyrocumulonimbus super outbreak reveals potential for increasingly extreme stratospheric smoke events. Climate Dynamics 54, 4367-4377.
| Crossref | Google Scholar |
Pezza AB, van Rensch P, Cai W (2012) Severe heatwaves in southern Australia: Synoptic climatology and large scale connections. Climate Dynamics 38, 209-224.
| Crossref | Google Scholar |
Philip S, Kew S, van Oldenborgh G, Anslow F, Seneviratne S, Vautard R, et al. (2022) Rapid attribution analysis of the extraordinary heatwave on the Pacific coast of the US and Canada in June 2021. Earth System Dynamics 13, 1689-1713.
| Crossref | Google Scholar |
Potter BE (2012) Atmospheric interactions with wildland fire behaviour – II. Plume and vortex dynamics. International Journal of Wildland Fire 21, 802-817.
| Crossref | Google Scholar |
Potter B (2018) The Haines Index – it’s time to revise it or replace it. International Journal of Wildland Fire 27, 437-440.
| Crossref | Google Scholar |
Rabin SS, Melton JR, Lasslop G, Bachelet D, Forrest M, Hantson S, Kaplan JO, Li F, Mangeon S, Ward DS, Yue C, Arora VK, Hickler T, Kloster S, Knorr W, Nieradzik L, Spessa A, Folberth GA, Sheehan T, Voulgarakis A, Kelley DI, Prentice IC, Sitch S, Harrison S, Arneth A (2017) The Fire Modeling Intercomparison Project (FireMIP), phase 1: experimental and analytical protocols with detailed model descriptions. Geoscientific Model Development 10, 1175-1197.
| Crossref | Google Scholar |
Reeder MJ, Spengler T, Musgrave R (2015) Rossby waves, extreme fronts, and wildfires in southeastern Australia. Geophysical Research Letters 42, 2015-2023.
| Crossref | Google Scholar |
Rifai SW, De Kauwe MG, Ukkola AM, Cernusak LA, Meir P, Medlyn BE, Pitman AJ (2022) Thirty-eight years of CO2 fertilization has outpaced growing aridity to drive greening of Australian woody ecosystems. Biogeosciences 19, 491-515.
| Crossref | Google Scholar |
Rousi E, Kornhuber K, Beobide-Arsuaga G, Luo F, Coumou D (2022) Accelerated western European heatwave trends linked to more-persistent double jets over Eurasia. Nature Communications 13, 3851.
| Crossref | Google Scholar | PubMed |
Ruthrof KX, Fontaine JB, Matusick G, Breshears DB, Law DJ, Powell S, Hardy G (2016) How drought-induced forest die-off alters microclimate and increases fuel loadings and fire potentials. International Journal of Wildland Fire 25, 819-830.
| Crossref | Google Scholar |
Seager R, Hooks A, Williams AP, Cook B, Nakamura J, Henderson N (2015) Climatology, variability, and trends in the U.S. vapor pressure deficit, an important fire-related meteorological quantity. Journal of Applied Meteorology and Climatology 54, 1121-1141.
| Crossref | Google Scholar |
Sharples JJ (2009) An overview of mountain meteorological effects relevant to fire behaviour and bushfire risk. International Journal of Wildland Fire 18, 737-754.
| Crossref | Google Scholar |
Srock AF, Charney JJ, Potter BE, Goodrick SL (2018) The Hot-Dry-Windy Index: a new fire weather index. Atmosphere 9, 279.
| Crossref | Google Scholar |
Stocks BJ, Lawson BD, Alexander ME, Van Wagner CE, McAlpine RS, Lynham TJ, Dube DE (1989) The Canadian Forest Fire Danger Rating System: an overview. The Forestry Chronicle 65, 450-457.
| Crossref | Google Scholar |
Sullivan AL, Matthews S (2013) Determining landscape fine fuel moisture content of the Kilmore East ‘Black Saturday’ wildfire using spatially-extended point-based models. Environmental Modelling & Software 40, 98-108.
| Crossref | Google Scholar |
Sullivan AL, Knight IK, Cheney NP (2002) Predicting the radiant heat flux from burning logs following a fire. Australian Forestry 65, 59-67.
| Crossref | Google Scholar |
Sullivan AL, McCaw WL, Cruz MG, Matthews S, Ellis PF (2012) Fuel, fire weather and fire behaviour in Australian ecosystems. In ‘Flammable Australia: fire regimes, biodiversity and ecosystems in a changing world’. (Eds RA Bradstock, AM Gill, RJ Williams) pp. 51–78. (CSIRO Publishing: Melbourne, Vic., Australia)
Toivanen J, Engel CB, Reeder MJ, Lane TP, Davies L, Webster S, Wales S (2019) Coupled atmosphere‐fire simulations of the Black Saturday Kilmore East wildfires with the unified model. Journal of Advances in Modeling Earth Systems 11, 210-230.
| Crossref | Google Scholar |
Zheng Y, Jong LM, Phipps SJ, Roberts JL, Moy AD, Curran MAJ, van Ommen TD (2021) Extending and understanding the South West Western Australian rainfall record using a snowfall reconstruction from Law Dome, East Antarctica. Climate of the Past 17, 1973-1987.
| Crossref | Google Scholar |
Footnotes
1 Drought definitions vary with region and different time periods. Here, we do not aim to delve into the differences but acknowledge the spectrum of multi-year and flash drought time scales can affect fuel availability. AghaKouchak et al. (2023) provide a description of drought indicators as well as the cascading factors contributing to drought impacts.