Grasshopper abundance and offtake increase after prescribed fire in semi-arid grassland
Nicholas Gregory Heimbuch A , Devan Allen McGranahan

A
B
C
Abstract
Fire modulates herbivore dynamics in open ecosystems. While extensive work demonstrates the interaction between fire and vertebrate grazers, less research describes how grasshopper herbivory dynamics respond to fire.
We examined how fire increased grass crude protein content and increased the density of and offtake by grasshoppers relative to unburned mixed-grass prairie.
We deployed grasshopper exclusion cages to determine grasshopper offtake of aboveground plant biomass, counted grasshopper abundance throughout the study period, and measured crude protein content of aboveground grass biomass.
Offtake and density were higher in burned versus unburned plots. Burned plot grasshopper density increased over time, with greater rates of increase in recently burned plots, while density remained constant in unburned locations.
We present a potential mechanism by which fire interacts with grasshoppers in open ecosystems. It is likely that greater grasshopper offtake and density in recently-burned plots is at least partially attributable to higher crude protein content, as grass in these plots has a much higher proportion of recent growth after fire removed senesced material.
Grasshopper herbivory likely acts as a multiplier of livestock herbivory in burned rangeland. Restoring fire regimes can balance direct negative effects of heating against nutritional benefits.
Keywords: fire-grazing interaction, magnet effect, Orthoptera: Acrididae, prescribed fire, pyric herbivory, rangeland forage quality, rangeland pest management.
Introduction
As globally ubiquitous herbivores, grasshoppers (Orthoptera: Acrididae) contribute to ecosystem function around the world. Grasshoppers are particularly important in open ecosystems–rangeland biomes such as grasslands and savannas in which plant communities are regulated by interactive disturbances including fire and herbivory (Bond 2021).
Historically, interest in grasshoppers has generally increased with their local density, as grasshopper outbreaks and locust swarms have wrought economic damage for centuries (Cease et al. 2015). While such outbreaks were long considered to be primarily driven by environmental conditions beyond human control, research has described close interactions between land management and grasshopper dynamics (Le Gall et al. 2019). Although the utility of this broader understanding of grasshoppers and human land use has mostly been realised within the context of pest control (Branson et al. 2006), grasshoppers also contribute to nutrient cycling and plant community composition (Belovsky and Slade 2000; Meyer et al. 2002; Zhang et al. 2011; Kietzka et al. 2021).
Because the nutritive value of vegetation in open ecosystems often varies depending on the time since it last burned, fire likely also affects grasshoppers by modulating their food resources. Perennial, fire-adapted plants resprout using energy stored in organs protected from heat damage, and post-fire plant tissue is typically higher in crude protein and lower in structural carbohydrates than the mature or senescent tissue that was consumed by the fire (McGranahan and Wonkka 2021). Thus, despite overall lower plant biomass on account of the fire, grasshopper abundance on recently-burned areas is often higher than unburned areas, especially for graminivorous (grass-eating) species (Meyer et al. 2002). More broadly, post-disturbance succession and plant nutritive value have been identified as important drivers of grasshopper abundance (Fartmann et al. 2012; Schirmel et al. 2019). Yet explicit examination of the relationships between time-since-fire, plant nutritive value, and grasshopper abundance have not been conducted.
We measured grasshopper abundance and forage consumption, along with grass protein content, in a replicated experiment that created a time-since-fire gradient in temperate grassland. We predicted that more recently burned plots would have both higher protein content, especially in leaves, and greater grasshopper abundance. As such, we predicted a greater degree of vegetation removal by grasshoppers from recently-burned plots, as determined by comparing aboveground plant biomass against that from within grasshopper exclosures.
Materials and methods
Study location & design
Our study was conducted at the USDA Agricultural Research Service (USDA-ARS) Livestock and Range Research Laboratory in Miles City, Montana, USA (46.40 N, 105.95 W). Vegetation is typical mixed-grass prairie, and the study site was dominated by western wheatgrass Pascopyrum smithii. During the study period, the overwhelming majority of grasshoppers on the study site, as determined by mid-season sweep netting and identification at the USDA-ARS Pest Management Research Unit in Sidney, Montana, consisted of the migratory grasshopper Melanoplus sanguinipes, a native species of spur-throated grasshopper in the family Acrididae.
Within a larger prescribed fire experiment, we selected nine rectangular, 300-m2 plots to test three different time-since-fire treatments (n = 3 each): (1) fire the previous autumn; (2) fire the previous spring; and (3) a control treatment left unburned for several years. Livestock were excluded from the entire study area and had been for several years. While the study area was open to wildlife such as deer (Odocoileus spp.), pronghorn Antilocapra americana, and lagomorphs including Sylvilagus floridanus and Lepus spp., we observed no evidence of their presence on any plots during the sampling period. Nor were we aware of other substantial invertebrate herbivores.
Sample collection
To measure the amount of vegetation removed by foraging grasshoppers, we established two pairs of sample points within each plot. Each pair of 0.25-m2 sample points consisted of one full mesh grasshopper exclosure alongside another structure with a similar footprint and shade factor that was open to grasshopper herbivory. Each type of structure consisted of a polyvinyl chloride tube frame with heavy nylon netting, which when fully wrapped and zipped around the frame and weighted down with sand-filled tubes, effectively kept grasshoppers out (Parker and Salzman 1985). Because the mesh reduced sunlight intensity by 400 w m−2 compared to the surrounding area, we designed control structures that remain open on the north and south faces to allow grasshoppers to enter while still producing shade conditions that matched the exclosures during peak photosynthetic activity. These paired structures ensured that shade would not influence grass development, skewing offtake measurements. Structures were monitored at least every 48 h and after any substantial weather event to ensure they remained intact; in the few instances grasshoppers had crawled under the exclosures, they were removed upon discovery.
On all plots, the first pair of structures was established 1 July 2021, and the second pair 1 week later. On 9 August (40 days after the first pair of structures were erected), all aboveground biomass, standing dead and current year’s growth, within each 0.25 m2 frame footprint was clipped to ground level. Within the recently-burned plots, individual grass tiller counts were recorded – because structures were placed randomly and tiller density was observed to be variable, we examined biomass on both a per-tiller basis as well as by area. Clipped biomass was dried at 60°C for 48 h and weighed to the nearest 0.001 g.
We collected forage quality samples on the 26th day of the study, roughly halfway through the study period. For each plot, samples were comprised of 40 western wheatgrass tillers randomly selected by tossing a marker flag in the air and clipping, to ground level, the tiller nearest to where it landed, regardless of phenology or live/dead status. Tillers were separated into leaf blades and stems (which included leaf sheaths) prior to drying at 60°C for 48 h and grinding into fine powder. Protein content was determined with a Thermo Scientific Flash 2000 combustion analyser.
To determine grasshopper density, we employed a standard ring count methodology (Onsager 1977; Joern and Laws 2013). One week after the initial pairs of structures were established, we placed 5, 0.1 m2 rings on the ground in a ×pattern centred on each plot, with rings approximately 1.5 m apart and at least 2 m from plot edges. Nineteen observations were made over the course of the study period, between 9 July and 6 August. All plots were sampled in each round of observations by a single observer (the first author), and all observations were conducted between 10:00 hours and 12:00 hours for consistent solar conditions. Sampling consisted of walking slowly through the plot and agitating the area near each ring with a long stick, and recording the number of grasshoppers that jumped from the ring.
Data analysis
To determine whether accessibility to grasshoppers affected the amount of aboveground vegetation, we subtracted the dried biomass values from control structures from that of their paired grasshopper exclosures and calculated the mean of these two differences for each plot (n = 3 experimental units per treatment). To account for the different lengths of deployments among the two pairs of structures, offtake was expressed as daily rate of removal by dividing the difference in biomass between paired structures by the number of days each structure pair was deployed. We used a linear model with the intercept term removed to test each of the three difference values against 0 (null hypothesis: no difference in standing crop between grasshopper exclosures and control frames) using the lm function in the R statistical environment (R Core Team 2020). We tested pairwise contrasts in standing crop differences across each treatment with a post hoc Tukey test using TukeyHSD.
We determined whether crude protein content varied with fire treatment and plant organs (leaf blades vs stems) by fitting each term and their interaction in an ANOVA. Pairwise contrasts among fire treatments were again tested with TukeyHSD.
To determine if there were general linear trends in grasshopper abundance patterns over the course of the study, we conducted a non-parametric test of the Kendall’s tau (τ) statistic fit to the grasshopper count data within each burn treatment using the kendallTrendTest function in the EnvStats package for R (Millard 2013). To compare the relative rates of change over the study period, we plotted the estimated eight slope of the trend for each burn treatment and the associated 95% confidence intervals as returned by kendallTrendTest.
Results
Overall, aboveground plant biomass was lower outside of exclosures in both fire treatments (64 ± 4% less in fall burn plots and 55 ± 9% less in spring burn plots), but did not differ between exclosures and accessible unburned plots (1 ± 8%). Biomass removal by grasshoppers accounted for statistically-significantly lower biomass outside of grasshopper exclosures in both fall and spring burns (t = −7.4, P < 0.001 and t = −5.8, P < 0.001, respectively; Fig. 1). Aboveground biomass was not different between grasshopper exclosures and areas accessible to grasshoppers in unburned plots (t = −0.16, P > 0.05). Offtake was significantly lower in unburned plots than plots burned in both the previous fall and spring (P < 0.01 and P = 0.01, respectively). Over the course of the study period, daily offtake rates averaged 3.9 ± 0.5 kg ha−1 day−1 in fall burns and 2.4 ± 1.3 kg ha−1 day−1 in spring burns, while offtake in unburned plots was not different from 0 (−0.4 ± 1.9 kg ha−1 day−1).
Biomass removal by grasshoppers in control (shaded, unenclosed) frames relative to paired exclosures in plots with three different fire treatments. Standing crop (kg ha−1) was determined by clipping at the end of the 4-week study period and differences attributable to grasshopper removal from control frames are expressed as a percentage of total standing crop in paired exclosures.
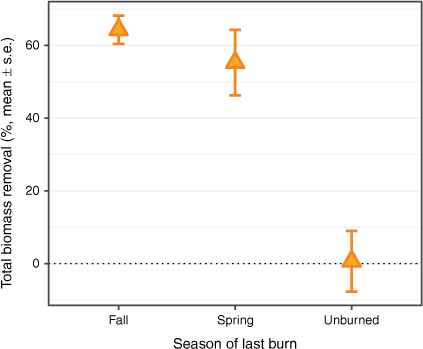
Crude protein content of western wheatgrass tillers varied among the fire treatments (t = 57, P < 0.001; Fig. 2). Crude protein content in fall and spring burns averaged 6.4% ± 0.2 s.e. and did not differ from one another (P > 0.05). But crude protein content in unburned plots, which included a substantial amount of senesced material from previous growing seasons was lower than in both fall and spring burns plots (t = −2.7, P < 0.001 and t = −3.1, P < 0.001, respectively).
Mean protein content of western wheatgrass Pascopyrum smithii sampled from three burn treatments as a percentage of total dry matter. Orange circles indicate the protein content of leaf blades; blue triangles are stems (including leaf sheaths).
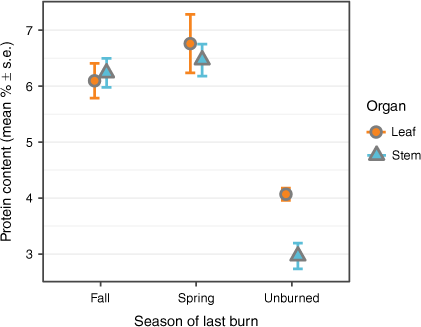
Across all samples, crude protein content did not vary among leaves and stems (t = 2.7, P > 0.05). Despite a trend towards higher crude protein in leaf tissue in unburned plots (Fig. 2), the pattern was not influential enough to create a significant fire treatment × organ interaction (t = 2.1, P > 0.05).
Grasshopper abundance was similar across plots at the beginning of the study period (early July) but increased significantly over the next month in fall and spring burn plots (τ = 0.29, P < 0.01 and τ = 0.62, P < 0.001; Fig. 3a). Grasshopper abundance remained constant over the study period in unburned plots (τ = 0.039, P > 0.05). While grasshopper abundance increased in both burn treatments, the rate of increase was approximately three times greater in plots that had been most recently burned in the spring than those that had been burned in the previous fall (Fig. 3b), which represented more than a four-fold increase in density from less than 3–12 grasshoppers m−2 (Fig. 3a).
(a) Observed grasshopper counts per square metre. Orange circles indicate data taken from fall burn treatments, blue triangles from spring burn treatments, and red squares from unburned (control) plots. (b) Data from Kendall’s Tau statistic, which assessed the observed count trendline consistency over time. Tau values were compared against the null hypothesis that there was no trend (slope = 0). 95% confidence intervals show the possible variance in slope for the data over time. Most grasshoppers observed were the migratory grasshopper Melanoplus sanguinipes.
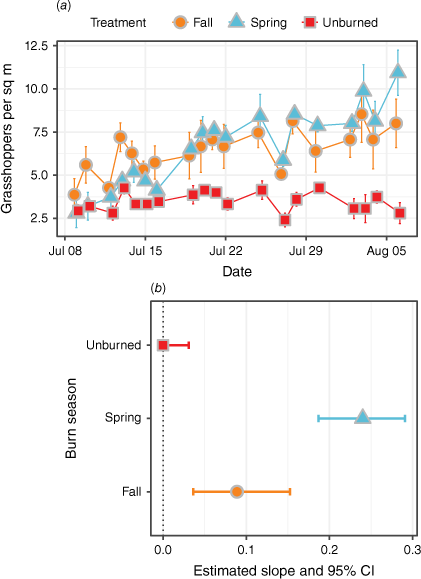
Discussion
Interactions between fire and herbivores drive plant and nutrient dynamics and affect ecosystem service delivery in open ecosystems. Grasshoppers are widely seen as pests in competition with economically-valuable livestock for herbaceous primary productivity (Zhang et al. 2019). Hewitt and Onsager (1983) estimated grasshoppers consume nearly USD400 million (USD1.7 billion, adjusted for inflation) worth of livestock forage per year in the western United States. Fire interacts with grasshoppers via direct and indirect effects, which are variable among species depending on their biology (e.g. Vermeire et al. 2004). In our study of indirect grasshopper responses to fire, grasshoppers removed over half of available aboveground plant biomass in burned plots but had no detectable effect in unburned plots, consistent with other ecosystems in which grasshoppers select burned vegetation (Stein et al. 1992; Lopes and Vasconcelos 2011).
Time since last fire drives spatial and temporal variability in the distribution of plant nutritional quality, which can be a strong determinant of grasshopper abundance and distribution (Joern et al. 2012; White 2012; Ozment et al. 2021). While the effect on grasshopper responses of differing phenological stages when burning occurs have not been specifically evaluated, plant tissues that resprout after fire generally have higher protein content than their mature counterparts on account of having a lower proportion of structural carbohydrates (McGranahan and Wonkka 2021). At the stand level, fire removes low quality, senesced material from previous seasons' growth, allowing high quality regrowth to dominate the sward. This elevated protein content in burned areas can be maintained over longer periods by repeated grazing (Wanchuk et al. 2021), even during drought (Spiess et al. 2020). Higher post-fire nutritional quality attracts herbivores, whose repeated defoliation maintain the high nutritional quality (Archibald et al. 2005; Sensenig et al. 2010; Allred et al. 2011). Previous work shows that the dominant grasshopper species in our plots, M. sanguinipes, prefers current year’s growth and standing dead material makes up only a small proportion of its diet (Anderson and Wright 1952; Mulkern et al. 1962). We suggest that in our study, burned plots with overall higher crude protein content as a result of higher proportions of green, resprouting tissue likely attracted M. sanguinipes.
Other sources of variability likely modulate the ubiquity of the fire–crude protein–grasshopper interaction. Firstly, grasshopper community composition can vary, with variable effects on food resources. While we observed M. sanguinipes to be the dominant species in our single-season study, other work from our research station has shown variability in dominant species. For example, Branson and Vermeire (2016) reported Ageneotettix deorum and Opeia obscura were the two most common species, while Branson and Haferkamp (2014) reported dominance by Phoetaliotes nebrascensis. Further research ought to determine how general the attraction to burned areas is among rangeland grasshopper species; previous fire ecology work has focused on direct effects of heating during fire, not indirect effects of post-fire resource alteration.
Secondly, crude protein is but one component of herbivore nutrition and is therefore not the sole determinant of use, despite being commonly used as a proxy for forage nutritive quality, more broadly. Certainly, diet selection among M. sanguinipes populations varies considerably, especially within temperate regions (Fielding and Defoliart 2008). At the same time, M. sanguinipes responds positively to plant nitrogen content (Branson 2003) and demonstrates higher physiological performance under high-protein diets and their relative diet selection tends to mirror that of what is available on the landscape (Zembrzuski et al. 2021). Furthermore, the pattern of fire and forage extends from crude protein to a broad suite of forage quality components including mineral content (Wanchuk 2022). Thus, it is reasonable to infer that crude protein content likely had a substantial contribution to the correlation between grasshopper density and time-since-fire reported here.
Thirdly, fire altered both vegetation nutritional status and structure in our study plots, making it difficult to parse the relative effect of either. For example, Schirmel et al. (2019) identified vegetation structure as the primary driver of grassland Orthoptera community composition, but found strong evidence for plant nutritive quality as driver as well. Fortunately, Ozment et al. (2021) provided some insight into the relative effects of vegetation structure and nutrient content: not only did they find grasshoppers are generally attracted to high-nutrient grazed areas, but they found the attraction weakened when the nutritional contrast between grazing lawns and surrounding areas lessened during drought. But the structural difference remained, which supports nutritional quality as an important driver.
Regardless of the mechanism, recently burned plots clearly attracted more grasshoppers and subsequently had more aboveground biomass removal than unburned plots, which has potential implications for management.
In terms of fire management, we suggest restoring fire regimes with frequent, spatially-discrete prescribed fire might leverage the direct, negative effects of heating to reduce overall grasshopper populations against the potential nutritional gains post-fire vegetation provides for individual grasshoppers that survive or migrate into recently-burned areas. Improved survival and reproduction resulting from nutrient enhancement in burned vegetation (Branson 2003) could intensify competition between livestock and grasshoppers in burned areas. In contrast, benefits of nutritive enhancement could be offset by negative fire effects, as fire alone can result in short-term reductions in grasshopper abundance by up to 75% (Branson and Vermeire 2016). Direct effects of fire include adult and nymphal mortality (Bock and Bock 1991), and egg mortality due to soil heating (Vermeire et al. 2004; Branson and Vermeire 2013, 2016). Thus, even despite positive nutritional benefits, fire could remain a sustainable low-cost alternative to conventional (chemical) control of economically-damaging grasshopper outbreaks (Branson et al. 2006), which are expensive, unreliable, and have off-target effects on non-pest species (Joern 2000).
Data availability
Data and R script used herein are available under a U.S. Public Domain license at the USDA Ag Data Commons (doi.org/10.15482/USDA.ADC/1528475).
Acknowledgements
We appreciate the general assistance of D.F. Watson from NPARL in organising field equipment, the assistance of C. Murphy, with protein analysis at LARRL, and N. Davidson, with grasshopper identification at NPARL. D. Strong maintains the field experiment.
References
Allred BW, Fuhlendorf SD, Engle DM, et al. (2011) Ungulate preference for burned patches reveals strength of fire-grazing interaction. Ecology and Evolution 1, 132-144.
| Crossref | Google Scholar | PubMed |
Archibald S, Bond WJ, Stock WD, et al. (2005) Shaping the landscape: Fire–grazer interactions in an African savanna. Ecological Applications 15, 96-109.
| Crossref | Google Scholar |
Belovsky GE, Slade JB (2000) Insect herbivory accelerates nutrient cycling and increases plant production. Proceedings of the National Academy of Sciences 97, 14412-14417.
| Crossref | Google Scholar | PubMed |
Bock CE, Bock JH (1991) Response of grasshoppers (Orthoptera: Acrididae) to wildfire in a Southeastern Arizona grassland. American Midland Naturalist 125, 162-167.
| Crossref | Google Scholar |
Bond WJ (2021) Out of the shadows: Ecology of open ecosystems. Plant Ecology & Diversity 14, 205-222.
| Crossref | Google Scholar |
Branson DH (2003) Reproduction and survival in Melanoplus sanguinipes (Orthoptera: Acrididae) in response to resource availability and population density: The role of exploitative competition. The Canadian Entomologist 135, 415-426.
| Crossref | Google Scholar |
Branson DH, Haferkamp MA (2014) Insect herbivory and vertebrate grazing impact food limitation and grasshopper populations during a severe outbreak. Ecological Entomology 39, 371-381.
| Crossref | Google Scholar |
Branson DH, Vermeire LT (2013) Heat Dosage and Oviposition Depth Influence Egg Mortality of Two Common Rangeland Grasshopper Species. Rangeland Ecology & Management 66, 110-113.
| Crossref | Google Scholar |
Branson DH, Vermeire LT (2016) Grasshopper responses to fire and postfire grazing in the Northern Great Plains vary among species. Rangeland Ecology & Management 69, 144-149.
| Crossref | Google Scholar |
Branson DH, Joern A, Sword GA (2006) Sustainable Management of Insect Herbivores in Grassland Ecosystems: New Perspectives in Grasshopper Control. BioScience 56, 743-755.
| Crossref | Google Scholar |
Cease AJ, Elser JJ, Fenichel EP, et al. (2015) Living With Locusts: Connecting Soil Nitrogen, Locust Outbreaks, Livelihoods, and Livestock Markets. BioScience 65, 551-558.
| Crossref | Google Scholar |
Fartmann T, Krämer B, Stelzner F, et al. (2012) Orthoptera as ecological indicators for succession in steppe grassland. Ecological Indicators 20, 337-344.
| Crossref | Google Scholar |
Fielding DJ, Defoliart LS (2008) Discriminating tastes: Self-selection of macronutrients in two populations of grasshoppers. Physiological Entomology 33, 264-273.
| Crossref | Google Scholar |
Hewitt GB, Onsager JA (1983) Control of grasshoppers on rangeland in the United States: a perspective. Journal of Range Management 36, 202-207.
| Crossref | Google Scholar |
Joern A (2000) What are the consequences of non-linear ecological interactions for grasshopper control strategies? In ‘Grasshoppers and Grassland Health: Managing Grasshopper Outbreaks without Risking Environmental Disaster, NATO Science Series. Vol. 73’. (Eds JA Lockwood, AV Latchininsky, MG Sergeev) pp. 131–144. (Springer: Dordrecht)
Joern A, Laws AN (2013) Ecological Mechanisms Underlying Arthropod Species Diversity in Grasslands. Annual Review of Entomology 58, 19-36.
| Crossref | Google Scholar | PubMed |
Joern A, Provin T, Behmer ST (2012) Not just the usual suspects: Insect herbivore populations and communities are associated with multiple plant nutrients. Ecology 93, 1002-1015.
| Crossref | Google Scholar | PubMed |
Kietzka GJ, Lecoq M, Samways MJ (2021) Ecological and Human Diet Value of Locusts in a Changing World. Agronomy 11, 1856.
| Crossref | Google Scholar |
Le Gall M, Overson R, Cease A (2019) A Global Review on Locusts (Orthoptera: Acrididae) and Their Interactions With Livestock Grazing Practices. Frontiers in Ecology and Evolution 7, 263.
| Crossref | Google Scholar |
Lopes CT, Vasconcelos HL (2011) Fire increases insect herbivory in a Neotropical savanna. Biotropica 43, 612-618.
| Crossref | Google Scholar |
Meyer CK, Whiles MR, Charlton RE (2002) Life History, Secondary Production, and Ecosystem Significance of Acridid Grasshoppers in Annually Burned and Unburned Tallgrass Prairie. American Entomologist 48, 52-61.
| Crossref | Google Scholar |
Onsager JA (1977) Comparison of Five Methods for Estimating Density of Rangeland Grasshoppers. Journal of Economic Entomology 70, 187-190.
| Crossref | Google Scholar |
Ozment KA, Welti EAR, Shaffer M, et al. (2021) Tracking nutrients in space and time: Interactions between grazing lawns and drought drive abundances of tallgrass prairie grasshoppers. Ecology and Evolution 11, 5413-5423.
| Crossref | Google Scholar | PubMed |
Parker MA, Salzman AG (1985) Herbivore Exclosure and Competitor Removal: Effects on Juvenile Survivorship and Growth in the Shrub Gutierrezia microcephala. Journal of Ecology 73, 903-913.
| Crossref | Google Scholar |
Schirmel J, Gerlach R, Buhk C (2019) Disentangling the role of management, vegetation structure, and plant quality for Orthoptera in lowland meadows. Insect Science 26, 366-378.
| Crossref | Google Scholar | PubMed |
Sensenig RL, Demment MW, Laca EA (2010) Allometric scaling predicts preferences for burned patches in a guild of East African grazers. Ecology 91, 2898-2907.
| Crossref | Google Scholar | PubMed |
Spiess JW, McGranahan DA, Geaumont B, et al. (2020) Patch-Burning Buffers Forage Resources and Livestock Performance to Mitigate Drought in the Northern Great Plains. Rangeland Ecology & Management 73, 473-481.
| Crossref | Google Scholar |
Stein SJ, Price PW, Abrahamson WG, et al. (1992) The effect of fire on stimulating willow regrowth and subsequent attack by grasshoppers and elk. Oikos 65, 190-196.
| Crossref | Google Scholar |
Vermeire LT, Mitchell RB, Fuhlendorf SD, et al. (2004) Selective control of rangeland grasshoppers with prescribed fire. Journal of Range Management 57, 29-33.
| Crossref | Google Scholar |
Wanchuk MR, McGranahan DA, Sedivec KK, et al. (2021) Contrasts in forage mineral concentration with patch-burn grazing: A preliminary analysis. Translational Animal Science 5, S75-S79.
| Crossref | Google Scholar |
Zembrzuski D, Woller DA, Jech L, et al. (2021) Establishing the nutritional landscape and macronutrient preferences of a major United States rangeland pest, Melanoplus sanguinipes, in field and lab populations. Journal of Orthoptera Research 30, 163-172.
| Crossref | Google Scholar |
Zhang G, Han X, Elser JJ (2011) Rapid top-down regulation of plant C:N:P stoichiometry by grasshoppers in an Inner Mongolia grassland ecosystem. Oecologia 166, 253-264.
| Crossref | Google Scholar | PubMed |
Zhang L, Lecoq M, Latchininsky A, et al. (2019) Locust and grasshopper management. Annual Review of Entomology 64, 15-34.
| Crossref | Google Scholar | PubMed |