Remote sensing of volatile organic compounds release during prescribed fires in pine forests using open-path Fourier transform infra-red spectroscopy
Cátia Magro



A
B
C
D
E
Abstract
Extreme wildfires have increased in recent decades, yet the consequences of extreme fire behaviour are not fully comprehended. The study of prescribed burning provides opportunities to advance understanding of some overlooked processes in fire behaviour, such as the role of the release of volatile organic compounds (VOC).
The aim of this study was to assess VOC (α-pinene, β-pinene, and limonene), NH3, CO and CO2 emissions during prescribed fires in pine barrens vegetation at the Albany Pine Bush Preserve, USA.
Measurements performed by open-path Fourier transform infra-red spectroscopy (OP-FTIR) quantified VOC concentrations and characterised emissions during four independent prescribed burns.
Combustion products (e.g. CO2, CO, CH4) and VOC exhibited similar emission behaviour during thermal degradation, though VOC concentrations appeared to be independent of the type of biomass burned, unlike those of combustion products; Pinus strobus L. emitted two orders of magnitude higher than Pinus rigida Mill.; VOC and CO are statistically correlated (R2 = 0.84).
These results confirmed that OP-FTIR is a feasible approach for gathering qualitative and quantitative information regarding VOC emission during prescribed fires.
Quantification of VOC concentrations during prescribed fires helps characterise its relationships with greenhouse gas emissions (e.g. CO2 and CO) at different burning conditions (e.g. wind, biomass type), which could be incorporated into existing fire behaviour models to enhance their ability to better predict fire propagation.
Keywords: carbon dioxide, carbon monoxide, OP-FTIR, Pinus rigida Mill., Pinus strobus L., prescribed fires, remote sensing, volatile organic compounds.
Introduction
Extreme wildfire behaviour is related to a number of factors, including high temperature, low humidity and dry fuel accumulation, which are directly tied to climate variability and change (NOAA 2022). There has been an increase in the use of prescribed fires in different parts of the world to remove fuel at significant spatial scales (Harper et al. 2018). Accurate quantification of fuel loads and distribution are imperative to facilitate the management of fires to understand fire spread dynamics, and to develop efficient plans to manage forest fires (Forest Europe 2010). It is important to recognise the potential benefits of prescribed fires in supporting land and ecosystems management (e.g. new growth, greater diversity of the heather structure, recovery habitat), and increasing resilience to wildfires (Knapp et al. 2009). Moreover, prescribed fires have also been employed in many countries to promote more controlled production and dispersion of wildfire smoke, while simultaneously reducing accumulated hazardous fuels. Thus, prescribed fires have the potential to maintain desired conditions in various ecosystem types by promoting vegetation productivity and soil fertility, minimising the spread of pests and diseases, and acclimatising the environment for conservation of endangered native species (Akagi et al. 2014; Greco 2018).
The pine barrens of the Albany Pine Bush Preserve in eastern New York, US are a fire-dependent habitat type. This preserve consists of over 1300 ha of protected land registered as an official National Natural Landmark, New York State Unique Area, Heritage Site, Bird Conservation Area, and National Audubon Society Important Bird Area. This landmark is home to more than 1500 plant and animal species, including 76 wildlife species of greatest conservation need and more than 20 at-risk species. The Albany Pine Bush Commission works with local landowners and the population to lessen the impacts of development and fragmentation of the remaining pine barrens (Bried et al. 2015). The management program of the preserve uses prescribed fires to maintain the open ecosystem of sand dunes with Pinus rigida Mill., Pinus strobus L., and wild blue lupin (Lupinus perennis L.), a host of the endangered Karner blue butterfly (Plebejus melissa samuelis Edwards; Brickle et al. 2013). This fire-dependent habitat requires frequent controlled burns to maintain desired vegetation structure and composition. The main goals of the prescribed fires in the Albany Pine Bush Preserve units are: (1) to expand open patches dominated by warm-season grasses and forbs, including L. perennis, and reduce shrub cover; and (2) to reduced fuel loads and severe wildfire risk.
Even considering advances in fire management, extreme fire behaviour is difficult to predict, especially sudden changes in fire spread and blow-up, eruptive or flashover fires (Cramer et al. 2018; San-Miguel-Ayanz et al. 2019). Thus, it has been suggested that high concentrations of flammable volatiles released by the leaves at high temperatures may help explain erratic fire behaviour (Chetehouna et al. 2009; Rego et al. 2021). The release of large amounts of volatile organic compounds (VOC) combined with specific fuel conditions, topography, and wind can create explosive scenarios, since VOC reach critical flammability thresholds and ignite with very high intensity when provided sufficient oxygen and a potential ignition source (Chatelon et al. 2014). Essential oils and resins in the needles of P. rigida and P. strobus are known to contain high levels of α-pinene, β-pinene, limonene, myrcene and terpinolene (i.e. 5.8–57.8% of total VOC concentration) (Ioannou et al. 2014; Arango-Velez et al. 2018; Hwang et al. 2022). These low density monoterpenoids are highly flammable VOC that may accumulate at ground level (Coudour et al. 2019). The behaviour of flammable volatiles released by vegetation under various environmental conditions has been studied using selectively coated nanosensors, microextraction techniques and chromatographic analysis (Nunes and Pio 2001; Xiong et al. 2003; Winters 2010; Gonçalves 2022; Gonçalves et al. 2022; Magro et al. 2022). However, those high efficient and precise approaches for detecting trace levels of emitted organic compounds require highly specialised equipment that must be operated within controlled laboratory conditions. Development of remote sensing tools has promoted a huge advance in reliable real-time monitoring of atmospheric gas pollutants such as ammonia (NH3) and ethanol (CH3CH2OH), some VOC and greenhouse gases (GHG) such as water vapour, carbon dioxide (CO2), carbon monoxide (CO) and methane (CH4) (Kira et al. 2015; Lin et al. 2019). Open-path Fourier transform infrared spectroscopy (OP-FTIR) is a technique that has been used extensively over the past 50 years for analysis of these types of compounds (Wooster et al. 2011; Akagi et al. 2014; Bai et al. 2014, 2018; Smith et al. 2014; Flesch et al. 2016; Lin et al. 2019; Byrne et al. 2020). Due to the high temporal resolution of real time data acquisition, the OP-FTIR technique allows simultaneous monitoring of multiple molecules at ground-level, both horizontally and vertically (Flesch et al. 2016). In addition to the growing need to assess toxic gaseous pollutants produced during both environmental and industrial events, open-path Fourier transform infra-red spectroscopy (OP-FTIR) methodologies have been used to monitor GHG, especially for ground-level smoke composition studies (Lin et al. 2019). For example, Wooster et al. (2011) used the approach to evaluate the plume released by burning biomass and determined emission ratios and factors for CO2, CO, CH4, formaldehyde and NH3. Similarly, de Castro et al. (2007), measured concentrations of approximately 18, 35, 6 and 2 ppm m−1 of CO2, CO, CH4 and NH3, respectively, that were emitted during burning of Calluna vulgaris (L.) Hull, Chamaespartium tridentatum (L.) P. Gibbs, Erica umbellata L., Ulex europaeus L., and Ulex minor Roth shrubs. Recent studies consider the applicability of other OP-FTIR approaches for the monitoring GHG emissions at farms located in Germany and Australia (Bai et al. 2014, 2018; Ni et al. 2015). Emission concentrations of different terpenoids have been commonly studied by gas chromatography and/or mass spectrometric analysis. For instance, in Scots pine (Pinus sylvestris L.) forests in Sweden, total terpenoid emission concentrations ranged from 3.5 to 56.0 µg m−3, of which limonene, α- and β-pinene comprised 0.81 to 8.10, 1.3–13.05 and 0.45–4.5 µg m−3, respectively (Janson 1992). Other studies conducted in forests, have documented even higher variation of limonene emissions, ranging from 0.9 to 12.2 g m−3 (Antonelli et al. 2020). Emission of other VOC in wildfire smoke sampled in 2016 near Fort Murray, Horse River, Alberta, Canada reached a total peak emission of 852 ng m−3, which was 17-times higher than the emission detected in non-fire conditions (Wentworth et al. 2018). Similarly, another study of VOC in a fire plume detected concentrations ranging from 24.0 to 68.2 µg m−3 (Piel et al. 2021). Nevertheless, studies regarding the application of OP-FTIR methodologies for the assessment of other VOC emissions, such as terpenoids, have yet to be developed, especially during wildfire events.
Thus, using the Albany Pine Bush Preserve as a proof of concept, the aim of this study was evaluating the potential of OP-FTIR to measure VOC, NH3, CO and CO2 emissions during prescribed fires. By using prescribed fire as experimental tools, this approach will allow to better understand how release of VOC influence fire behaviour. The measurements performed at the preserved pine forests will allow the collection of data in different fuel types, topography, and wind, providing therefore data that, to the best of our knowledge, has yet not been explored.
Materials and methods
Open-path Fourier transform infra-red spectroscopy (OP-FTIR) measurements
Spectral measurements were conducted using a RAM2000 G2 OP-FTIR system (Kassay Field Services Inc., Mohrsville, PA, USA), consisting of a FTIR, two retro-reflectors and a data processing system (Kira et al. 2015, 2016; You et al. 2021). In the most basic depiction of OP-FTIR data processing, field data spectra are collected and processed by an iterative technique that yields a reliable chemical concentration of target chemicals present in the beam path. The beam path is described by the line that the infra-red (IR) signal travels between the spectrometer (transceiver) and retro-reflectors. The IR beam from the RAM2000 G2 OP-FTIR is considered modulated since the high temperature IR source is projected into the interferometer then steered out of the instrument through the telescope mirrors. When the IR signal is returned from the retro-reflectors it goes through a second beam splitter (not the interferometer), which directs the returning IR energy to a cooled detector. In this configuration modulated energy is uniquely attributed to the original IR source propagated from the OP-FTIR. This type of configuration is referred to as ‘mono static.’ Mono static OP-FTIR is a preferred technique since it does not require corrections associated with other configurations (stray, passive or bi-static IR energy) emitted from far IR sources.
The data collection was performed using Remote Molecular Monitor Software, RMMSoft, approved by U.S. Environmental Protection Agency (2000) with the Environmental Technology Verification Program. During on-site measurements, the OP-FTIR spectrometer was placed on a tripod, ensuring that the line of sight was approximately 1.5 m above the ground and aligned with the retro-reflectors (Russwurm and Childers 1999; Jiao et al. 2019). The RMMSoft software has a series of set-up parameters that must be fulfilled to instruct the hardware. The main parameters include the number of co-adds to average together, the amplifier gain, and the path length. Once fed, the software acquisition feature can be started in order to collect the data, analyse it, present the results, and repeat the whole process. The OP-FTIR spectrometer collects an IR spectrum constrained by a user selected time interval, being that the minimum possible time is of 2 s. During this time interval, the OP-FTIR is moving mirrors and the detector, while the data acquisition system completes a cycle. The path lengths used were specific for each case study: 58 m for the test fires and 296–340 m for prescribed fires. The IR spectra were acquired using a resolution of 0.5 cm−1, using a detector gain of 128, which allowed the collection of average voltages of approximately 17 V for test fires and 5–7 V for prescribed fires. Collecting field data using the RAM2000 G2 OP-FTIR is made through a PC-based user interface. The number of co-adds was set to 25 to increase the signal-to-noise ratio (S/N), corresponding approximately to a 1-min acquisition interval for each individual signal (Russwurm and Childers 1999). For each measurement, the data acquisition was initiated prior the fire ignition, being held up until the plume approached and surpassed the OP-FTIR pathway to obtain the compound variation with the fire’s propagation. The range of measured spectra was 700–4500 cm−1; however, due to high water vapour interferences the analysis was restricted to the range of 850–3000 cm−1 to increase the sensitivity. To analyse each data spectrum, on-site background spectra were recorded prior and after each field campaign using the same path as the one used to acquire the data spectrum, preserving therefore, the signal intensity (voltage) and atmospheric characteristics when applying the processing algorithms. Having the same signal intensity results in better data quality and thus lower minimum detection limits (MDL) during processing. Additionally, presenting an identical path for the background allows non-target atmospheric chemicals to be filtered out, to focus on the compound of interest. It is important to note that, since the background measurement was collected pre-burn, and the plume measurements were collected many metres down-wind (ground level) there is no need to apply temperature correction techniques. This strategy was employed to avoid measuring a high temperature plume, which would require matching high temperature reference spectra, as spectral absorption peak features changes with temperature (Smith et al. 2011).
The OP-FTIR data was processed using a multilevel classical least-square (CLS) method, which allows a statistical comparison of the obtained IR spectra with those included in the National Institute of Standards and Technology (NIST) reference library (U.S. Environmental Protection Agency 1999). The target chemicals monitored were α-pinene, β-pinene, limonene, NH3, CH4, methanol (CH3OH), CO, CO2, water vapour and propane (C3H8). The monoterpenoids (α-pinene, β-pinene and limonene) were selected as the major VOC emitted by the vegetation studied (Arango-Velez et al. 2018; Hwang et al. 2022), while propane is a surrogate for linear chain hydrocarbons that absorb in the 2800–3000 cm−1 region of the spectrum. Additionally, NH3 was also monitored as it is one of the main gases released to the atmosphere during wildfires, being highly related to the formation of secondary aerosols in smoke (Lindaas et al. 2021). Other GHG as CO, CO2, and water vapour, which are already highly concentrated in the atmosphere, were considered as interferences during some parts of the prescribed burns in the data processing, as well as the overlaying between terpenoids and other hydrocarbon-type molecules. Therefore, the CO and CO2 values, determined, corresponded to the instances that the OP-FTIR signal was not saturated in the regions used to quantify those target compounds. During data collection, when the number of 25 co-adds was reached the rawest form of the data, known as interferogram, was saved, and then converted to a single-beam data spectrum by application of a Fourier transform. In simple terms, the single-beam data spectrum is divided by the background single beam to create an absorbance spectrum. It is in the absorbance spectrum mode that the CLS algorithm from the analysis method is applied. For each chemical, the analysis method identifies the spectral search region(s) to carry out the CLS statistical comparison between library reference spectrum and the data spectrum. The software also considers other chemicals absorbing within the same analysis regions as interferences within the multichemical analysis region known as matrix. For the analysis, each chemical was analysed in six independent regions, scaled by the absorbance intensity, and then presented as a concentration in µg m−3. The analysis method was reviewed over 16-times to optimise a final method, which was used to reprocess all the final data. Each revision included analytical changes such as region end points, interferences, rejection criterion, references, and other adjustable parameters available in the RMMSoft method setup. The optimised method was able to identify the target chemicals α-pinene, β-pinene, limonene, NH3, methanol, CH4 and CO. When a target chemical is identified and presented by the method is due to the statistical goodness-of-fit established between the field measured data and the library reference has exceeded more than three-times the s.d. of the noise. This value is considered the MDL and can be calculated with a confidence level of 99.7%.
Field campaign at Albany Pine Bush Preserve
Gas emissions were measured during four small test fires at the Albany Pine Bush Preserve on 19 and 20 May 2022. In each burn, fresh branches were constantly added for 15–20 min in order to have tree branches burning simultaneously; this corresponded to 5–10 kg of needle biomass with 100% moisture content. Each needle burned for about 1 min (Rothermel and Deeming 1980).
The temperature and humidity in the test burns were similar to those observed in the days of prescribed fires (maximum temperature 20–24°C; maximum humidity 40–42%). Winds speed was low but variable (≤10 km h−1).
Tree branches and needles of P. rigida and P. strobus emissions were compared in two assays, per species. The purpose of this assay was to optimise the measurement procedure of the VOC emitted by the selected branches and needles during combustion, as well as to identify the specific variations of the volatile compound concentrations. The OP-FTIR equipment was placed and calibrated alongside the test-fire pathway. The data measurements and collection began prior to the beginning of each test fire and prior to the burning of the first set of tree branches. Each experiment lasted from 18 to 26 min, until branch’s combustion was complete.
After the test fires, measurements were made during prescribed fires in four different management units of the Albany Pine Bush Preserve: (1) Dromedary; (2) Dorsum; (3) Cupcake; and (4) Asphalt. The placement of the OP-FTIR and the reflector in each management unit is in Fig. 1. For the open field measurements during the prescribed fires, the data acquisition with the OP-FTIR system was performed similarly as with the test fires. The total data collection time was as follows for the individual fires: 60 min for Dromedary; 90 min for Dorsum; 187 min for Cupcake; and 63 min for Asphalt.
Location of the prescribed fires and the position of the OP-FTIR equipment and reflector in each fire location.
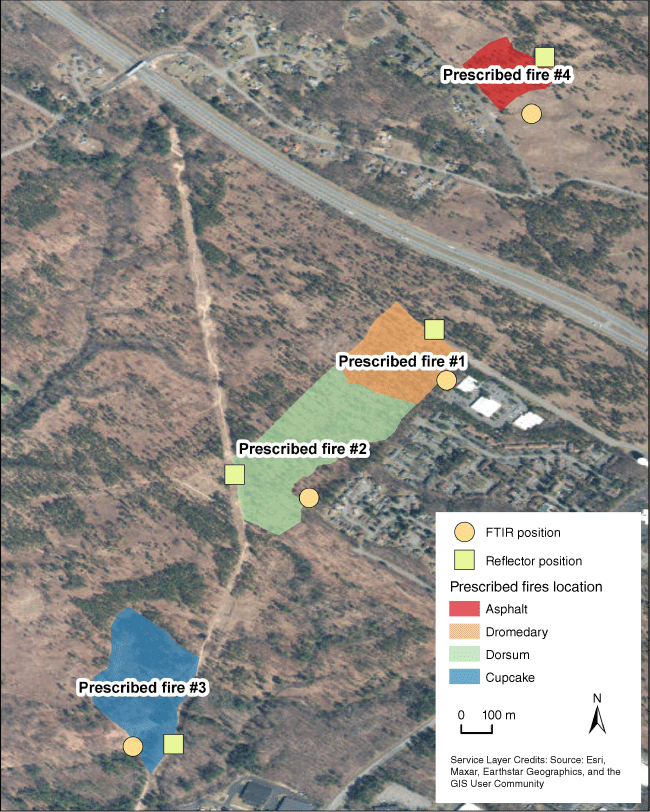
The Dromedary and Dorsum units is characterised by dense forest of P. rigida trees, that was recently thinned to more closely resemble the globally rare, open pitch pine-scrub oak (Quercus ilicifolia Wangenh) barrens stand structure that was historically typical in the area. These units have broad sandy trails and rolling dunes. The Cupcake unit has the most diverse forest communities, with pitch pine-scrub oak barrens, native prairies on rolling sand dunes, and steep slopes and mesic conditions that support dense masses of ferns and other moisture-loving plants. L. perennis is evident in this area in May and June. The Asphalt unit is characterised by sandy open landscape with pitch pine, wild blue lupin, scrub oak and blueberry. A summary of each management unit topography is in Table 1. In spite of local differences all of the burn units had similar fuel load profiles, characterised by a pine litter dominated fuel model (i.e. fuel model two, Anderson 1982).
Burn name and location | Date of measurements | Weather | Maximum temperature (°C) | Maximum humidity (%) | Dewpoint (°C) | Transport winds (km h−1) | Burned area (ha) | Dominant species | |
---|---|---|---|---|---|---|---|---|---|
Dromedary; Madison Avenue Pinelands (trailhead #7) | 24 May 2022 | Partly sunny (45–55%) | 20 | 42 | 5.5 | North-east 9.7–12.9 | 5.3 | Pinus rigida; Quercus ilicifolia; Lupinus perennis | |
Dorsum; Madison Avenue Pinelands (trailhead #7) | 10.1 | Pinus rigida; Quercus ilicifolia; Lupinus perennis | |||||||
Cupcake; Great Dune (trailhead #8) | 25 May 2022 | Mostly sunny (40–50%) | 24 | 40 | 8.3 | South 4.8–8.0 | 4.0 | Pinus strobus; Pinus rigida; Quercus coccinea; Quercus rubra; Quercus ilicifolia; Corylus americana; Fagus grandifolia; Acer rubrum; Quercus spp. regeneration | |
Asphalt; (Antelope South) Truax Trail Barrens (trailhead #12) | 26 May 2022 | Partly sunny (55–65%) | 24 | 42 | 6.6 | South 22.5–37.0 | 3.9 | Pinus rigida; Quercus ilicifolia; Lupinus perennis; Vaccinium corymbosum |
Calibration
Standards of (+)-α-pinene (α-pinene; C10H16; 98%; St. Louis, MO, USA), (−)-β-pinene (β-pinene; C10H16; 99%; St. Louis, MO, USA) and R-(+)-limonene (limonene; C10H16; 97%; Toluca, Mexico) were purchased from Sigma-Aldrich. Ultra-pure water was obtained from a Milli-Q water purification system from Merck Millipore (Burlington, MA, USA). The calibration standards were used to prepare individual standard solutions and one mixture of tree terpenoids (α-pinene, β-pinene and limonene), which were used to calibrate the OP-FTIR system in order to acquire their IR spectra and identify possible interferences. Furthermore, library references were also used on the OP-FTIR system for limonene, α- and β-pinene, obtained from the published quantitative Hanst database. To create instrument-specific gas references, an internal 10 m gas cell attachment was installed into the OP-FTIR for qualitative assurance. The new references uniquely match the OP-FTIR for both the resolution, interpolation, and detector-response characteristics. The process to create the instrument-specific references was as follows: (1) pull a vacuum over the acquiesce sample to create a vapour with the target chemical; (2) extract the vapour sample into the 2-L internal gas cell installed into the OP-FTIR, the 10 m cell allows the IR beam to pass through the sample 40 times; (3) analyse the sample by comparison with the Hanst library database reference of known concentration; and (4) install the quantified sampleas a reference library to quantify other field data collected during the trial burns. This process of reference calibration through the use of an real reference standard allows a more accurate field measurement. There is a small unknown amount of quantitation uncertainty if relying exclusively on digital library references. This unknown amount of uncertainty due to the instrument specific parameters is smaller when the concentration of the target chemical measured in the field data is close to the concentration of the library reference. When the concentration of the target chemical is very low (or high) in comparison, those parameters contribute a relatively larger influence over the shape of the absorbance features, which can result in a larger error in the quantified concentration. To reduce this uncertainty, a gas-cell was used taking advantage of strong absorbance features of the library reference to quantify and assign the concentration of the newly created instrument specific reference under more ideal conditions. By flowing the known target chemical into the gas-cell in relatively high concentration abundance, the newly created reference will have spectral absorbance shape features, which include instrument matching parameters. Ultimately, the concentration of that newly created reference is determined by CLS method. The newly created site-specific reference is applied in the same manner as the stored digital library reference, relying on the CLS algorithm to quantify the field data. Note that the CLS technique is applied in the same manner to the newly created reference as was with the original digital reference.
Results and discussion
Assessment of OP-FTIR measurements at Albany Pine Bush Preserve
The behaviour of volatiles emitted during the combustion of P. strobus and P. rigida branches was studied through small test fires set at the Albany Pine Bush Preserve. Volatile emission patterns were unique for each test fire (Fig. 2). With the inclusion of tree branches, rapid combustion ensued, emitting the monitored volatiles, notably evident following branch placement in Fig. 2b and d (marked as ‘i’). Subsequently, tree needles ignited aggressively upon being introduced to the fire, producing a surge of volatiles persisting for 1–4 min.
Concentration of VOC and CO detected in two OP-FTIR assays of test burns conducted using branches and needles of P. strobus L. (a, b) and P. rigida Mill. (c, d). Vertical lines indicate the start (i) and end (ii) of the addition of branches.
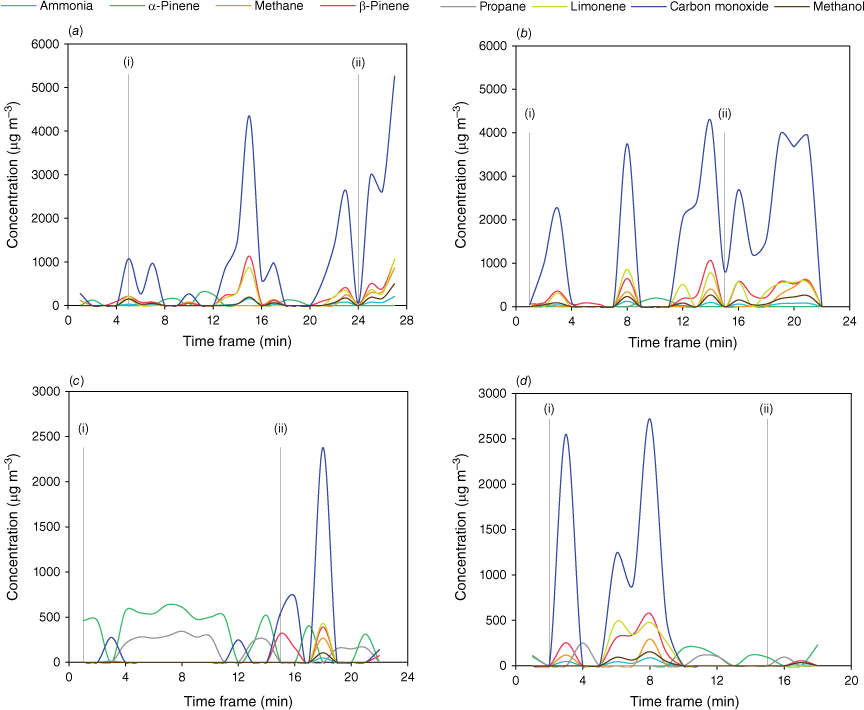
The target volatile compounds tended to increase and decrease in similar patterns with a major emission mid-way through each test burn and a decline towards the end. This suggests that each of the volatiles is being emitted synchronously when the tree branches and needles burn, implying that the biomass was completely burned, and that the major volatiles stored inside the tree leaves and branches were completely emitted. Similar results have been reported for the emission of CO and CO2 during thermal degradation of U. europaeus leaves (de Castro et al. 2007), which suggest that the emission of VOC and GHG during thermal degradation is identical, regardless of vegetation type.
Overall, it is possible to observe that VOC emissions (i.e. α-pinene, β-pinene and limonene) from the P. strobus test burns (Fig. 2a, b) were more than 2-times higher than P. rigida (Fig. 2c, d). The maximum emitted concentration of VOC and CO by P. strobus were of 1131 and 5259 µg m−3, respectively, compared to 638 and 2718 µg m−3 emitted from P. rigida. This indicates that P. strobus branches and needles have higher amounts of the target VOC when compared with the P. rigida, and thus might release higher concentrations when ignited. Moreover, P. strobus emissions contained more β-pinene and limonene, while P. rigida pyrolysis had higher α-pinene concentrations (Fig. 2c). The maximum VOC emitted concentration measured are in agreement with the values reported by Antonelli et al. (2020) for P. sylvestris forests (<12.2 mg m−3). In contrast, both species showed relatively low emissions of NH3, CH4, propane and methanol (i.e. maximum values of approximately 207, 861, 350 and 500 µg m−3, respectively). These concentrations are in accordance with those reported by de Castro et al. (2007), especially for NH3. Those authors also reported an emission ratio of NH3 (6%) and CH4 (20%) in comparison to CO. Additionally, it was confirmed that with the exception of CO2, CO was the most abundant GHG emitted during biomass pyrolysis, with an emission ratio of approximately 5:1 for CO2:CO. These correlations show that, even when using different vegetation species, the OP-FTIR analytical approach is consistent with previous studies for most GHG, and is adequate for the study of the selected VOC.
After evaluating OP-FTIR on test fires, measurements during prescribed fires were performed at the Albany Pine Bush Preserve in four different management units (Dromedary, Dorsum, Cupcake, Asphalt). The operations were conducted to reduce litter and duff by 10%, reduce shrub cover by 80%, reduce slash from mechanical fuel reduction treatments and expose mineral soil over 30% of the site. The measurements for CO2 were compromised by its high background concentrations and reduced the sensitivity of the OP-FTIR method. Thus, the analytical thresholds (i.e. MDL) obtained for all the compounds during the prescribed fires ranged from 0.01 to 655.17 µg m−3 (Table 2).
Compound | Limit of detection (µg m−3) | ||||||||
---|---|---|---|---|---|---|---|---|---|
Test fire 1 | Test fire 2 | Test fire 1 | Test fire 2 | Operational prescribed fires | |||||
P. rigida Mill. | P. rigida Mill. | P. strobus L. | P. strobus L. | Dromedary | Dorsum | Cupcake | Asphalt | ||
Carbon dioxide | 655.17 | 655.17 | 341.38 | 379.31 | 140.00 | 227.51 | 23.65 | 11.76 | |
Ammonia | 0.26 | 0.19 | 0.17 | 0.13 | 0.02 | 0.02 | 0.01 | 0.01 | |
α-Pinene | 0.76 | 0.59 | 0.69 | 0.55 | 0.14 | 0.23 | 0.06 | 0.04 | |
Methane | 2.24 | 2.00 | 1.45 | 1.66 | 0.40 | 0.35 | 0.17 | 0.12 | |
β-Pinene | 0.45 | 0.33 | 0.38 | 0.31 | 0.07 | 0.13 | 0.03 | 0.02 | |
Propane | 1.90 | 0.86 | 0.83 | 0.66 | 0.15 | 0.25 | 0.07 | 0.05 | |
Limonene | 0.52 | 0.38 | 0.45 | 0.34 | 0.09 | 0.15 | 0.04 | 0.02 | |
Carbon monoxide | 5.86 | 2.69 | 0.83 | 0.62 | 0.09 | 0.16 | 0.04 | 0.03 | |
Methanol | 0.55 | 0.38 | 0.38 | 0.33 | 0.04 | 0.05 | 0.04 | 0.02 |
The Dromedary prescribed fire was ignited at 19 min (i), when no volatile compounds emissions were detected (Fig. 3a, b). However, after the fire reached the OP-FTIR pathway (ii), VOC started to being detected and the analytical signal increased rapidly, giving a first peak of emission at minute 34 (total volatiles, 1294 µg m−3). A secondary peak was then observed at 51 min (iii; total volatiles, 2713 µg m−3), close to the end of the ignition. Smoke from the fire continued and recordings stopped at minute 60. To take advantage of the favourable weather conditions, the Dorsum fire was ignited almost immediately after the Dromedary unit burn concluded. As observed in Fig. 3c, d, a major emission peak was observed at minute 15 (total volatiles, 4492 µg m−3) suggesting that the fire approached the OP-FTIR pathway shortly after data collection started. The end of the ignitions was around Minute 20, although recordings continued until minute 90, and documented smoke from smouldering combustion. There were no high volatiles emission peaks observed prior to ignition of the Cupcake fire (I; Fig. 3e, f). As in the previous fires, a sudden increase of the volatiles was observed after the fire crossed the OP-FTIR pathway (ii), showing the higher emission peak at 99 min (total volatiles, 6187 µg m−3). Lower amounts of VOC were released as burning abated as recorded until Minute 187. For the Asphalt unit (Fig. 3g, h), concentrations of VOC were lower than the other three units with a major emission peak near the end of the combustion, observed at Minute 41 (iii; total volatiles, 834 µg m−3). This may have resulted from the high wind velocities (22.5–37.0 km h−1) and higher dispersal of volatiles. The fluctuating wind direction may also have contributed to the unique and variable emission pattern. The highly variable wind, fire intensity and smoke dispersal caused the cessation of burning at Minute 41 and termination of recording at Minute 63. In general, both CO and VOC had similar emission behaviour. Nevertheless, the absolute concentration of these two compounds differed between the four fires. Moreover, the VOC concentrations were higher than those previously reported for fire and non-fire conditions (Janson 1992; Wentworth et al. 2018; Antonelli et al. 2020; Piel et al. 2021). It is likely that patterns correspond to distinct dispersion processes where higher wind speeds correspond to higher dispersion and low CO and VOC detection.
Concentrations of individual and total VOC (excluding CO) emitted during the Dromedary (a, b), Dorsum (c, d), Cupcake (e, f), and Asphalt (g, h) prescribed fires. Vertical lines indicate the start of the fire (i), the instant the fire crossed the OP-FTIR pathway (ii) and the end of combustion (iii).
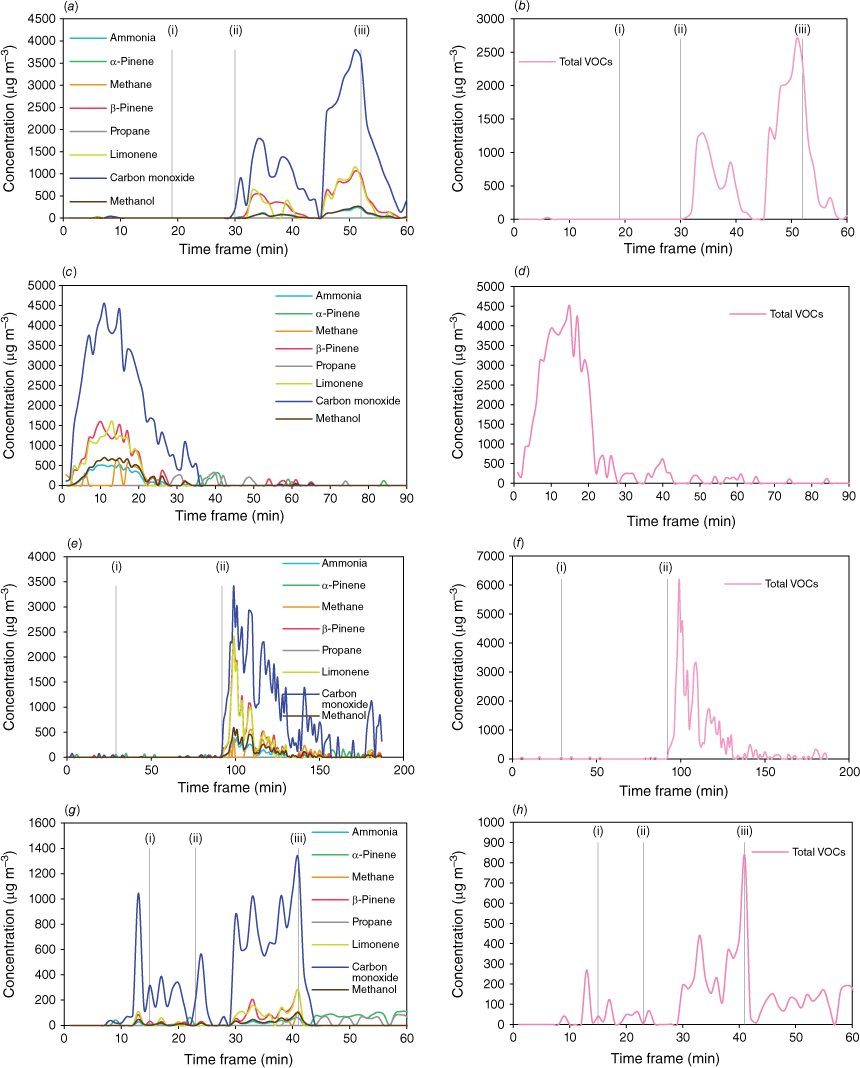
In general, all the prescribed fires featured an initial release boost of volatiles immediately after ignition, with high concentrations occurring when smoke was within the OP-FTIR pathway. Additionally, the volatile behaviour observed during the prescribed fires included high CO emissions (≤4553 µg m−3) as well as β-pinene and limonene (≤1605 µg m−3), followed by medium methanol, NH3, and CH4 emissions (≤698 µg m−3) and, finally, by smaller propane and α-pinene concentrations (≤311 µg m−3). These results agree with those from test fires with pine branches and needles confirming the importance of limonene, α-pinene and β-pinene as the main VOC emitted from fire.
Relations between CO2, CO and VOC
When fuels are completely combusted, CO2 and water vapour are the only end products remaining. However, during most wildfires, combustion is incomplete and they generate a mixture of gases, including CO2, CH4, and CO. These conclusions were reported in the previous sections, where products of incomplete combustion and VOC were as detected by OP-FTIR during prescribed fires. The relationship between CO2 and CO concentrations for the four prescribed fires (Fig. 4) was used as an index of completeness of combustion. Thus, the linear relationship derived from the four prescribed fires, make it possible to predict CO2 concentrations from measured CO concentrations or vice versa. This relationship is estimated by Drysdale (2011) and Ward et al. (1996), which is based on the mole ratio of carbon released as CO2 to the sum of the moles of carbon released as CO2 and CO.
From the analysis of Fig. 4, it seems that CO concentrations are around 1% of CO2 concentrations (obtained by the equation y = 0.0109×; with a R2 = 98%). This percentage indicates a very high completeness of the combustion process where oxygen from wind is not a limiting factor for fire. Wooster et al. (2011), described CO concentrations of around 9–12% of CO2 concentration during open vegetation fires. The present study reported higher emission factors for CO, which indicates that the four case study burns had relatively high degree of combustion processes.
Given the main objective of the study, it was pivotal to establish correlations between CO concentrations, which are commonly measured in prescribed fires, and total volatile concentrations, which are not (Fig. 5). The relation between CO and VOC concentrations measured in open field conditions were well related during the four prescribed fires (~R2 > 0.8) using a second order regression with no intercept (Fig. 5). The lower fit of the Asphalt prescribed fire may have resulted from more variable wind conditions. The capacity to estimate total volatiles from CO measurements has potential assist in modelling the possible effects of VOC release on fire behaviour.
Total VOC concentration (ammonia, α-pinene, methane, β-pinene, propane, limonene and methanol) in relation to CO concentrations during four prescribed fires and all fires combined. All zero values in the y-axis were excluded.
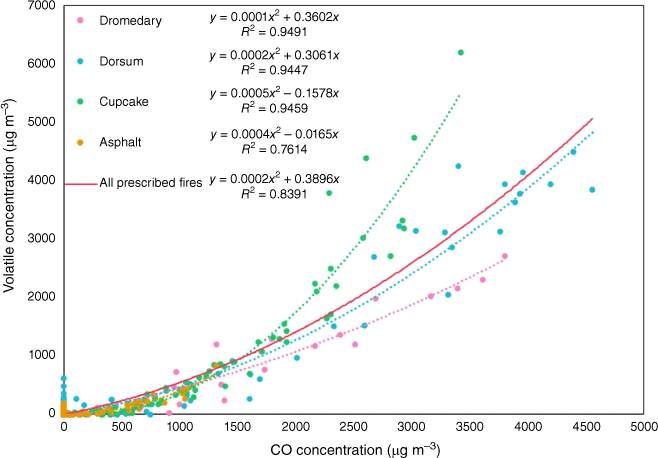
Given the high correlation with products of combustion, the VOC measurements may be included the monitoring and management risk in wildfire-prone forests. In this sense, monitoring of VOC concentrations related to vegetation temperature and its heating may improve the estimations of fire risk as well as the likelihood of wildfire occurrence.
Conclusion
This case study advances the understanding of VOC and GHG emissions during prescribed fires by using OP-FTIR advances as monitoring tool. The site and prescribed burning conditions at Albany Pine Bush Preserve, USA provided insights into how fuel types, topography, and wind influence burning in pine-dominated ecosystems.
The OP-FTIR results demonstrate the potential of the approach by providing qualitative and quantitative information. Analysing the responses of various products’ concentrations (i.e. CO2, CO, CH4, NH3, α-pinene, β-pinene and limonene) to different fire behaviour, site, and fuel conditions, has enabled the establishment of overarching relationships. These findings hold the potential to enhance predictive capabilities concerning extreme fire events. Specifically, the use of OP-FTIR measurements allows us to infer:
Data acquisition by OPS-FTIR (i.e. infra-red spectra and concentrations of selected VOC and GHG) is independent of the type fuel, but dependent on wind and distance to the detector.
Combustion products and VOC display similar behaviour during thermal degradation; VOC are emitted as the tree branches and needles are burned, showing major emissions initially that decline and cease when combustion terminates.
Volatile concentrations seem independent of the type of biomass burned.
The VOC profile of P. strobus is two orders of magnitude higher than P. rigida, with CO, β-pinene, and limonene as the main contributors, followed by methanol, NH3, and CH4 emissions and, finally, by smaller propane and α-pinene concentrations.
The strong non-linear correlation between VOC and CO (R2 = 0.84) suggests it may be feasible to amend existing models to consider the impact of VOC on fire behaviour.
Data availability
The simulation data used in this study can be shared upon request to the corresponding author.
Declaration of funding
This research was funded by the project PCIF/GFC/0078/2018-Influence of forest VOC (volatile organic compounds) on extreme fire behaviour from Fundação para a Ciência e a Tecnologia (FCT). Further steps are considered under the project Fire-RES (H2020-LC-GD-2020-3-101037419).
Acknowledgements
The authors thank Rob Crampton for support in the in loco measurements. The authors are grateful to the handling Conservation Director Neil Gifford and the fire management team Tyler Briggs, Todd Compani and David Brickner of Albany Pine Bush Preserve, for the full support that allowed data acquisition to be fully completed, with great efficiency.
References
Akagi SK, Burling IR, Mendoza A, Johnson TJ, Cameron M, Griffith DWT, Paton-Walsh C, Weise DR, Reardon J, Yokelson RJ (2014) Field measurements of trace gases emitted by prescribed fires in southeastern US pine forests using an open-path FTIR system. Atmospheric Chemistry and Physics 14(1), 199-215.
| Crossref | Google Scholar |
Antonelli M, Donelli D, Barbieri G, Valussi M, Maggini V, Firenzuoli F (2020) Forest volatile organic compounds and their effects on human health: a state-of-the-art review. International Journal of Environmental Research and Public Health 17, 6506.
| Crossref | Google Scholar | PubMed |
Arango-Velez A, Chakraborty S, Blascyk K, Phan MT, Barsky J, El Kayal WE (2018) Anatomical and chemical responses of eastern white pine (Pinus strobus L.) to blue-stain (Ophiostoma minus) inoculation. Forests 9(11), 690.
| Crossref | Google Scholar |
Bai M, Suter H, Lam SK, Sun J, Chen D (2014) Use of open-path FTIR and inverse dispersion technique to quantify gaseous nitrogen loss from an intensive vegetable production site. Atmospheric Environment 94, 687-691.
| Crossref | Google Scholar |
Bai M, Suter H, Lam SK, Davies R, Flesch TK, Chen D (2018) Gaseous emissions from an intensive vegetable farm measured with slant-path FTIR technique. Agricultural and Forest Meteorology 258(May 2017), 50-55.
| Crossref | Google Scholar |
Brickle MC, Lookingbill TR, Engelhardt KAM (2013) Proposing new barrens National Natural Landmarks. The George Wright Forum 30(3), 253-260 Available at https://www-jstor-org.esf.idm.oclc.org/stable/43598300.
| Google Scholar |
Bried JT, Gifford NA, Robertson KM (2015) Predicted crown fire risk adds incentive to restore open-canopy pine barrens at the Wildland-Urban Interface. Journal of Sustainable Forestry 34(1–2), 147-167.
| Crossref | Google Scholar |
Byrne B, Strong K, Colebatch O, You Y, Wunch D, Ars S, Jones DBA, Fogal P, Mittermeier RL, Worthy D, Griffith DWT (2020) Monitoring urban greenhouse gases using open-path Fourier transform spectroscopy. Atmosphere-Ocean 58(1), 25-45.
| Crossref | Google Scholar |
Chatelon F-J, Sauvagnargues S, Dusserre G, Balbi J-H (2014) Generalized blaze flash, a “Flashover” behavior for forest fires—analysis from the firefighter’s point of view. Open Journal of Forestry 4(5), 547-557.
| Crossref | Google Scholar |
Chetehouna K, Barboni T, Zarguili I, Leoni E, Simeoni A, Fernandez-Pello AC (2009) Investigation on the emission of volatile organic compounds from heated vegetation and their potential to cause an accelerating forest fire. Combustion Science and Technology 181(10), 1273-1288.
| Crossref | Google Scholar |
Coudour B, Chetehouna K, Lemée L, Bertin P, Garo J-P (2019) Thermal degradation of α-pinene using a Py-GC/MS. Journal of Thermal Analysis and Calorimetry 137, 1315-1328.
| Crossref | Google Scholar |
Cramer W, Guiot J, Fader M, Garrabou J, Gattuso JP, Iglesias A, Lange MA, Lionello P, Llasat MC, Paz S, Peñuelas J, Snoussi M, Toreti A, Tsimplis MN, Xoplaki E (2018) Climate change and interconnected risks to sustainable development in the Mediterranean. Nature Climate Change 8(11), 972-980.
| Crossref | Google Scholar |
de Castro AJ, Lerma AM, López F, Guijarro M, Díez C, Hernando C, Madrigal J (2007) Open-path Fourier transform infrared spectrometry characterization of low temperature combustion gases in biomass fuels. Infrared Physics & Technology 51(1), 21-30.
| Crossref | Google Scholar |
Flesch TK, Baron VS, Wilson JD, Griffith DWT, Basarab JA, Carlson PJ (2016) Agricultural gas emissions during the spring thaw: Applying a new measurement technique. Agricultural and Forest Meteorology 221, 111-121.
| Crossref | Google Scholar |
Forest Europe (2010) Assessment of Forest Fire Risks and Innovative Strategies for Fire Prevention. Available at www.foresteurope.org
Gonçalves OC, Nunes L, Magro C, Neng NR, Rego FC, Vieira P, Nogueira JMF (2022) Evaluación de compuestos orgánicos volátiles emitidos por las hojas de las principales especies de árboles en Portugal a través de metodología por HS-SPME/GC-MS. Ed. Sociedad Española de Ciencias Forestales. In 8º Congreso Forestal Español (8CFE), 1129. ISBN: 978-84-941695-6-4. [in Spanish]
Harper AR, Doerr SH, Santin C, Froyd CA, Sinnadurai P (2018) Prescribed fire and its impacts on ecosystem services in the UK. Science of the Total Environment 624, 691-703.
| Crossref | Google Scholar | PubMed |
Hwang HS, Kim YR, Han JY, Choi YE (2022) Nematicidal properties and chemical composition of Pinus rigida Mill. resin against pinewood nematodes. Forests 13(7), 1131.
| Crossref | Google Scholar |
Ioannou E, Koutsaviti A, Tzakou O, Roussis V (2014) The genus Pinus: a comparative study on the needle essential oil composition of 46 pine species. Phytochemistry Reviews 13, 741-768.
| Crossref | Google Scholar |
Janson R (1992) Monoterpene concentrations in and above a forest of Scots pine. Journal of Atmospheric Chemistry 14, 385-394.
| Crossref | Google Scholar |
Jiao L, Guo Y, Chen J, Zhao X, Dong D (2019) Detecting volatile compounds in food by open-path Fourier-transform infrared spectroscopy. Food Research International 119, 968-973.
| Crossref | Google Scholar | PubMed |
Kira O, Dubowski Y, Linker R (2015) Reconstruction of passive open-path FTIR ambient spectra using meteorological measurements and its application for detection of aerosol cloud drift. Optics Express 23(15), A916-A929.
| Crossref | Google Scholar | PubMed |
Kira O, Linker R, Dubowski Y (2016) Detection and quantification of water-based aerosols using active open-path FTIR. Scientific Reports 6(January), 25110.
| Crossref | Google Scholar | PubMed |
Knapp EE, Estes BL, Skinner CN (2009). Ecological effects of prescribed fire season: a literature review and synthesis for managers. General Technical Report PSW-GTR-224. (U.S. Department of Agriculture, Forest Service, Pacific Southwest Research Station: Albany, CA) pp. 1–80. Available at http://books.google.com/books?hl=en&lr=&id=BaOl-7PVLQIC&oi=fnd&pg=PP1&dq=Ecological+Effects+of+Prescribed+Fire+Season+:+A+Literature+Review+and+Synthesis+for+Managers&ots=FwhMmK7uWz&sig=MuiDnNI0CDRsZMjy7stqPXIY29A
Lin CH, Grant RH, Heber AJ, Johnston CT (2019) Application of open-path Fourier transform infrared spectroscopy (OP-FTIR) to measure greenhouse gas concentrations from agricultural fields. Atmospheric Measurement Techniques 12(6), 3403-3415.
| Crossref | Google Scholar |
Lindaas J, Pollack IB, Calahorrano JJ, O’Dell K, Garofalo LA, Pothier MA, Farmer DK, Kreidenweis SM, Campos T, Flocke F, Weinheimer AJ, Montzka DD, Tyndall GS, Apel EC, Hills AJ, Hornbrook RS, Palm BB, Peng Q, Thornton JA, et al. (2021) Empirical insights into the fate of ammonia in Western U.S. wildfire smoke plumes. Journal of Geophysical Research: Atmospheres 126(11), e2020JD033730.
| Crossref | Google Scholar |
Magro C, Gonçalves OC, Morais M, Ribeiro PA, Sério S, Vieira P, Raposo M (2022) Volatile organic compound monitoring during extreme wildfires: assessing the potential of sensors based on LbL and sputtering films. Sensors 22(17), 6677.
| Crossref | Google Scholar | PubMed |
Ni K, Köster JR, Seidel A, Pacholski A (2015) Field measurement of ammonia emissions after nitrogen fertilization – A comparison between micrometeorological and chamber methods. European Journal of Agronomy 71, 115-122.
| Crossref | Google Scholar |
Nunes TV, Pio CA (2001) Emission of volatile organic compounds from Portuguese eucalyptus forests. Chemosphere - Global Change Science 3(3), 239-248.
| Crossref | Google Scholar |
San-Miguel-Ayanz J, Durrant T, Boca R, Libertà G, Branco A, de Rigo D, Ferrari D, Maianti P, Artés Vivancos T, Oom D, Pfeiffer H, Nuijten D, Leray T (2019) Forest Fires in Europe, Middle East and North Africa 2018. Joint Research Centre. Publications Office of the European Union. 10.2760/1128
Smith TEL, Wooster MJ, Tattaris M, Griffith DWT (2011) Absolute accuracy and sensitivity analysis of OP-FTIR retrievals of CO2, CH4 and CO over concentrations representative of “clean air” and “polluted plumes”. Atmospheric Measurement Techniques 4(1), 97-116.
| Crossref | Google Scholar |
Smith TEL, Paton-Walsh C, Meyer CP, Cook GD, Maier SW, Russell-Smith J, Wooster MJ, Yates CP (2014) New emission factors for Australian vegetation fires measured using open-path fourier transform infrared spectroscopy - Part 2: Australian tropical savanna fires. Atmospheric Chemistry and Physics 14(20), 11335-11352.
| Crossref | Google Scholar |
U.S. Environmental Protection Agency (1999) Compendium of Methods for the Determination of Toxic Organic Compounds in Ambient Air. Compendium Method TO-16: Long-Path Open-Path Fourier Transform Infrared Monitoring Of Atmospheric Gases. Available at https://www.epa.gov/sites/default/files/2019-11/documents/to-16r.pdf
U.S. Environmental Protection Agency (2000) Environmental Technology Verification Program. Available at https://archive.epa.gov/nrmrl/archive-etv/web/pdf/01_vr_ail.pdf
Ward DE, Hao WM, Susott RA, Babbitt RE, Shea RW, Kauffman JB, Justice CO (1996) Effect of fuel composition on combustion efficiency and emission factors for African savanna ecosystems. Journal of Geophysical Research: Atmospheres 101, 23569-23576.
| Crossref | Google Scholar |
Wentworth GR, Aklilu Y-a, Landis MS, Hsu Y-M (2018) Impacts of a large boreal wildfire on ground level atmospheric concentrations of PAHs, VOCs and ozone. Atmospheric Environment 178(2017), 19-30.
| Crossref | Google Scholar | PubMed |
Wooster MJ, Freeborn PH, Archibald S, Oppenheimer C, Roberts GJ, Smith TEL, Govender N, Burton M, Palumbo I (2011) Field determination of biomass burning emission ratios and factors via open-path FTIR spectroscopy and fire radiative power assessment: headfire, backfire and residual smouldering combustion in African savannahs. Atmospheric Chemistry and Physics 11(22), 11591-11615.
| Crossref | Google Scholar |
Xiong G, Goodridge C, Wang L, Chen Y, Pawliszyn J (2003) Microwave-assisted headspace solid-phase microextraction for the analysis of bioemissions from Eucalyptus citriodora leaves. Journal of Agricultural and Food Chemistry 51, 7841-7.
| Crossref | Google Scholar | PubMed |
You Y, Moussa SG, Zhang L, Fu L, Beck J, Staebler RM (2021) Quantifying fugitive gas emissions from an oil sands tailings pond with open-path Fourier transform infrared measurements. Atmospheric Measurement Techniques 14(2), 945-959.
| Crossref | Google Scholar |