Enhanced prediction of extreme fire weather conditions in spring using the Hot-Dry-Windy Index in Alberta, Canada
Kyle G. Elliott A * , Mike D. Flannigan B and Cordy Tymstra AA
B
Abstract
Fire weather indices forecast fire behaviour and provide valuable information for wildland fire prevention, preparedness, and suppression. However, these indices do not directly account for atmospheric conditions aloft. The province of Alberta, Canada has experienced extreme fire weather conditions during spring for decades, leading to the continued occurrence of disastrous wildland fires.
We examined the Hot-Dry-Windy Index (HDWI) and spread days over the first 4 days of 80 large wildland fires that started in May 1990–2019 in Alberta.
HDWI values were calculated using ERA5 reanalysis data from the 1000, 975 and 950 hPa levels. Differences between HDWI distributions on spread days and non-spread days were examined using permutation tests. Initial Spread Index was also examined as it is considered an important Fire Weather Index System value for wildland fire spread during spring in Alberta.
Higher median HDWI values were observed on spread days than non-spread days, where median Initial Spread Index values showed little to no difference.
This analysis suggests that HDWI can contribute to the prediction of significant spring wildland fire spread in Alberta.
Forecasted HDWI and HDWI climatologies may provide additional decision support for wildland fire management agencies.
Keywords: Alberta, Canada, fire weather, HDWI, Hot-Dry-Windy Index, prediction, spring wildfires, wildfire spread, wildland fire.
Introduction
Wildland fires are a common and widespread disturbance in Canada, particularly in the boreal/taiga plain and shield ecozones (Stocks et al. 2002). Though wildland fires are a natural and important ecological process (Flannigan et al. 2009), Canada is experiencing a lengthening fire season and increasing occurrence of extreme fires, fire sizes and area burned (Hanes et al. 2018). Western Canada has experienced recent disastrous fire seasons (Tymstra et al. 2021). With expected increases in wildland fire activity due to climate change, and an expanding wildland–urban interface, fire management agencies providing important public safety and protection of assets are encountering more challenging and complex fire conditions (Wotton 2009).
In Alberta, the critical period between snow-melt and green-up accounts for about half of the annual area burned (Tymstra et al. 2021). In longer spring seasons (earlier snow-melt and later green-up), the number of fires increases (Parisien et al. 2023). A decrease in coniferous foliar moisture content, referred to as spring dip, contributes to increased susceptibility to crown fire initiation and spread (Chrosciewicz 1986). Low live fuel moisture content is a primary factor in spring fire behaviour, especially when combined with an abundance of dead forest fuels.
After green-up, the high moisture content of deciduous foliage in the canopy and understorey reduces fire spread potential (Alexander 2010). Since fires occurring before this green-up stage cause nearly all structural loss, an understanding of the fire weather conditions contributing to spring wildland fires is important (Tymstra et al. 2019).
Tymstra et al. (2021) identified critical synoptic weather patterns during spring fire spread days in Alberta. Spread days are associated with cold front passages usually following a blocking ridge breakdown, or persistent strong dry south-southeast winds generated by high pressure systems originating in the Arctic when water bodies are frozen and vegetation evapotranspiration is low.
In Canada, daily wildland fire decision making is primarily based on the Fire Weather Index (FWI) and Fire Behaviour Prediction (FBP) Sub-systems of the Canadian Forest Fire Danger Rating System (CFFDRS) (Lawson and Armitage 2008). CFFDRS produces three fuel moisture codes (Fine Fuel Moisture, Duff Moisture, and Drought Codes) and three fire behaviour indexes (Initial Spread, Buildup, and Fire Weather Indexes). The Initial Spread Index (ISI) estimates spread potential and is an important fire behaviour predictor in the spring. On average, ISI is at or near its peak in the spring, where the Buildup Index (BUI) peaks later in the season (Parisien et al. 2023). Though the FWI System is an effective forecasting tool for predicting fuel conditions and potential fire behaviour, there is a need for additional forecasting and support tools to complement the CFFDRS. In particular, the CFFDRS does not directly account for weather conditions aloft.
Atmospheric indices describing the state of the atmosphere are not readily used as daily indicators of fire behaviour or fire spread potential in Canada. We therefore examined the Hot-Dry-Windy Index (HDWI) and its relationship to spring fire spread in Alberta. Introduced by Srock et al. (2018), this index combines temperature and moisture within the lowest 500 m of the atmosphere into one factor: the Vapour Pressure Deficit (VPD). VPD is the difference between Saturation Vapour Pressure (ES), a variable dependent solely on temperature (T) that represents the maximum amount of moisture the atmosphere can hold at that temperature, and Actual Vapour Pressure (E), a variable dependent solely on absolute moisture content (q). VPD and wind speed (WS) are used in the calculation of HDWI (Srock et al. 2018) (Eqn 1).
HDWI can capture atmospheric moisture, instability, and strong dry wind occurrences, including winds in the lower levels of the atmosphere that mix and quickly alter the surface conditions on a wildland fire. Our objectives were to determine if HDWI can be used to identify spread days during spring fire season, and if so, at what HDWI values did spread days occur. We also aimed to provide context as to what elevated HDWI values were by computing and examining three climatologies.
Materials and methods
Wildland fire data (1990–2019) were obtained from the Alberta Wildfire Management Branch (WMB) (Government of Alberta 2019). This includes fire location coordinates at the time of assessment and burned area size updates. Other information such as fuel type is also available but was excluded due to the objective of assessing a weather-only index. Large fires that started in the month of May were examined. We define a large wildland fire as one that burned 1000 ha or more by the time of extinguishment as used in Tymstra et al. (2021). This resulted in 80 large wildland fires that started in May during this 30-year (1990–2019) study (Fig. 1). The number of May wildland fires >1000 ha and area burned (ha) by year are in Table 1; years with no May wildland fires >1000 ha are not in this table. Of these 80 large wildland fires, ~25% of their total area burned occurred during the first 4 days following ignition (Tymstra et al. 2021). Therefore, HDWI calculations focused on the first 4 days of each fire, with day one being the day it was assessed.
May wildland fires in Alberta (1990–2019) >1000 ha at the time of extinguishment from Tymstra et al. (2021).
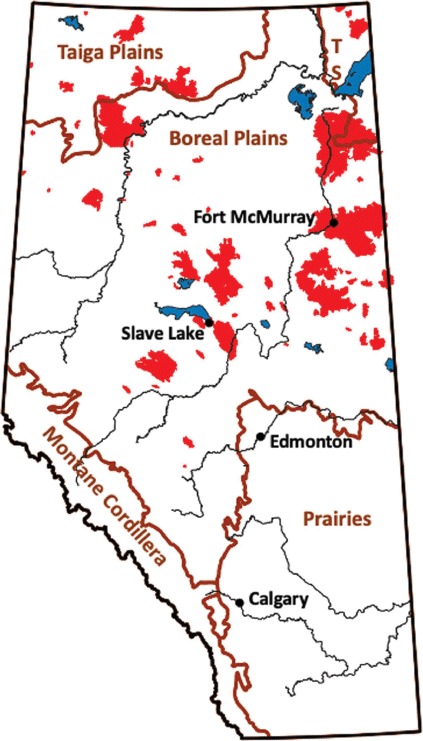
Year | Count | Area burned (ha) | Year | Count | Area burned (ha) | |
---|---|---|---|---|---|---|
1991 | 1 | 1559.1 | 2006 | 1 | 1786.0 | |
1993 | 1 | 4876.1 | 2008 | 1 | 1307.0 | |
1995 | 8 | 328,592.2 | 2011 | 9 | 715,767.2 | |
1998 | 16 | 343,065.1 | 2012 | 3 | 4348.6 | |
1999 | 7 | 31,508.6 | 2013 | 1 | 1303.0 | |
2001 | 6 | 137,055.4 | 2015 | 6 | 43,964.8 | |
2002 | 1 | 238,866.8 | 2016 | 2 | 496,727.6 | |
2003 | 1 | 1310.0 | 2018 | 7 | 28,064.1 | |
2005 | 2 | 10,573.3 | 2019 | 7 | 795,538.6 |
Hourly ERA5 (5th generation) climate reanalysis from the European Centre for Medium-Range Weather Forecasts (ECMWF) data were obtained from the Copernicus Climate Change Service (CS3) Climate Data Store (CDS 2021) for the period 1959–2020 (CS3 Online CDS). ERA5 is a gridded product with a latitude-longitude grid resolution of 0.25° by 0.25°. Four weather variables were used from three levels of the atmosphere (1000, 975, and 950 hPa) to calculate HDWI. Hourly temperature in Kelvin (TK), relative humidity (RH%), U-Component of wind speed (u) and V-Component of wind speed (v) from 11:00 hours to 20:00 hours MDT were obtained for the three pressure levels (1000, 975, and 950 hPa). This timeframe provides coverage during the standard burn period used in Alberta (10:00–18:00 hours MDT). The time of 10:00 hours MDT was excluded due to relatively less favourable burning conditions in the morning. The assessment period was extended to 20:00 hours MDT to account for favourable burning conditions in the early evening that support active fire growth. Each of the 80 fires was manually assigned an ERA5 grid cell based on the coordinates recorded during initial fire assessment. Hourly weather in each assigned grid cell from the three atmospheric levels were recorded for the calculation of hourly HDWI.
Observed weather and daily FWI System values (12:00 hours MST) for weather stations in Alberta were also used from the WMB database. Weather stations closest to individual fires were selected to represent local conditions. We refer to the observed ISI values from the WMB database as ISI.F (ISI FIRES). Tymstra et al. (2021) suggested an ISI adjustment to incorporate the impact of wind gusts on the wind speed. These ISI values were calculated using the ERA5 reanalysis in McElhinny et al. (2020) from 1979 to 2018 (for 1990–2018) in combination with wind gusts recorded from WMB weather stations. To include the 2019 season, Tymstra et al. (2021) calculated FWI System values using ERA5 temperature and dewpoint temperature (°C), relative humidity (RH%), wind speed (km h−1), and 24 h precipitation (mm). To incorporate wind gusts, Tymstra et al. (2021) converted the 10-min average wind speed using a piecewise linear function to a probable maximum of 1 min average wind speed. These adjusted wind speeds were used to recalculate FFMC and ISI values (referred to as ISI.AR).
HDWI addresses how the atmosphere may influence fire behaviour by analysing the lowest 500 m that is below the daytime mixing layer and just above the surface (Srock et al. 2018). The maximum VPD (hPa) and WS (m s−1) from any level in the lowest 500 m from the surface are selected for the calculation of HDWI. These maxima may occur at different pressure levels because near-surface mixing allows for weather variables to have direct impacts on a wildland fire at some time throughout the day (Srock et al. 2018). The units for HDWI values are not expressed as they are not physically significant (Srock et al. 2018).
where U is wind speed (m s−1), VPD (TC, q) is the calculated VPD (hPa) at TC (temperature °C), and q is absolute moisture content.
We calculated Saturation Vapour Pressure (ES) from TC, converted from TK, using Eqn 2 and fitted to Wexler’s formula when TC is between −30 and 35°C (Wexler 1976; Bolton 1980).
where ES is saturation vapour pressure (hPa) and TC is temperature (°C).
To calculate VPD, E is required. E was calculated as:
where E is Actual Vapour Pressure (hPa), RH% is relative humidity expressed as a percentage, and ES is Saturation Vapour Pressure (hPa).
With a calculated ES and E, VPD is calculated as:
where VPD is Vapour Pressure Deficit in hPa, ES is Saturation Vapour Pressure (hPa), and E is Actual Vapour Pressure (hPa).
U-Component of wind (UWS) and V-Component of wind (VWS) were also collected (m s−1). The units for WS for the calculation of HDWI are also m s−1. WS was calculated as:
where WS is wind speed (m s−1), u is the U-Component of wind, and v is the V-component of wind.
These variables were calculated hourly from 11:00 hours to 20:00 hours MDT at the three pressure levels. Hourly maxima of VPD and WS were selected from any of the three levels. That is, the maximum VPD and WS selected did not always come from the same level, but are often a combination. Srock et al. (2018) calculated a daily maximum HDWI value from NCEP’s Climate Forecast System Reanalysis on 6-h outputs for 12:00 hours, 18:00 hours, and 00:00 hours UTC. Due to the availability of hourly ERA5 reanalysis data, we calculated hourly HDWI values. With the hourly maxima selected, the hourly HDWI for 11:00–20:00 hours MDT for the first 4 days of all 80 large May wildland fires were calculated using Eqn 1.
Spread day analysis
A spread day occurs when a fire actively spreads and considerably increases in fire size (Podur and Wotton 2011). Spread days occur when fire behaviour limits the effectiveness of suppression efforts resulting in continued growth of a fire (Wang et al. 2015). A non-spread day is a day where limited fire growth is observed and suppression efforts are likely to succeed. It is hypothesised that HDWI on spread days are significantly greater than HDWI on non-spread days.
There are different approaches to identify spread days and non-spread days, including the use of classified MODIS hotspots (Podur and Wotton 2011), linear spread, and increases in area burned. In our analysis, an area burned mixed approach as in Tymstra et al. (2021) was employed. If a fire was reported as 200 ha or greater on Day 1, it was classified as a spread day. For Days 2, 3, and 4, a spread day occurred if the fire doubled in area burned between two or more updates over a single day (Daily Relative Growth, DRG).
An assessment of the first 4 days of these 80 large wildland fires resulted in a total of 320 possible observations. To negate overnight fire growth impacting the analysis, only those days with at least two fire size updates were used. Due to this approach, days with only one reported fire size were excluded from this analysis. For days with more than two size updates, the first and last updates were selected. This method resulted in 114 observations (206 observations removed, ~64%). For each of the first 4 days, the maximum hourly HDWI that occurred between two reported size updates was selected to represent the growth observed. If a greater HDWI value occurred outside of the size update report times, it was not used as it would not represent the growth observed.
Most fire sizes recorded in the WMB database are ocular assessments of size, especially at the time of assessment and through the first few burn periods. Ocular assessments of size can be difficult due to smoke obscuring visualisation of the fire perimeter, or due to inexperience completing ocular assessments. However, these estimations are acceptable for fire management officials to describe the size and complexity of a fire, thus eliminating the need to interrupt aerial operations by performing a low-level flight of the fire perimeter. Recording an area GPS track is often completed in the early morning or late evening to collect more accurate incident information.
There are known limitations due to the grid sizes with the ERA5 weather data. In particular, the local scale weather variables such as wind speed may be masked due to an averaging effect over a large area. Tymstra et al. (2021) found mean wind speeds were underestimated by 0.8 m s−1 (~2.9 km h−1) in ERA5 reanalysis.
Multiple cases were tested to examine different days since ignition for the 80 wildland fires. HDWI was tested for the first 4 days: Day 1 separated; and Days 2−4 separated. Isolating Day 1 and Days 2–4 was done to determine if different thresholds of HDWI values existed on the day of ignition and for ongoing, active fires. Permutation tests were used to compare HDWI during spread days and non-spread days. The code for permutation testing was written using R statistical software (R Core Team 2020). We conducted a two-tailed test with the null hypothesis that the distributions of HDWI values on spread days was equal to non-spread days. It is expected that all, or a large majority of these 80 large fires were actively suppressed to some degree. Due to the nature of large active wildland fires, it is assumed that suppression would not impact growth on areas with the greatest intensity (i.e. head of the fire).
ISI during spread days and non-spread days was also analysed to compare HDWI performance during spring. Daily ISI values from observed surface weather conditions (ISI.F) as well as those calculated from ERA5 with gusts incorporated into wind speeds (ISI.AR) from Tymstra et al. (2021) were analysed separately. The same spread day classification and permutation test processes were used as in the analysis of HDWI. All statistical analysis was completed using R statistical software (R Core Team 2020), including graphic creation using the ggplot2 package (Wickham 2016).
HDWI forecasting
Srock et al. (2018) calculated HDWI values for 12:00 hours, 18:00 hours and 00:00 hours UTC. Due to diurnal heating, the maximum HDWI is expected to occur around 00:00 hours UTC. Time of occurrence of daily maximum HDWI over the first 4 days of the 80 fires was also recorded (320 possible observations) for the purpose of determining a standard forecast time in May in Alberta. Some of these HDWI values fell outside of the report time used in the earlier spread day analysis. To analyse this, a randomised distribution of the median using a bootstrap was completed on the dataset of peak HDWI time to analyse if theoretical peak burn of 17:00 hours MDT was the time at which daily maximum HDWI occurred.
HDWI climatology in May
As with any fuel moisture or fire weather index, information on what constitutes elevated fire behaviour conditions is important. Climatologies provide a framework for fire management officials to use HDWI in an operational setting (McDonald et al. 2018) to identify what are the elevated values, and to detect trends. To provide this context, 31-year climatologies of HDWI for the month of May (1990–2020) were compiled for three locations in Alberta: (1) Fort McMurray (56.75°N, 111.50°W); (2) Slave Lake (55.25°N, 114.75°W); and (3) Peace River (56.25°N, 117.25°W). These climatologies represent three example longitudinal locations across the boreal forest in Alberta. Weather data for the calculation of HDWI was calculated from the same ERA5 source as the spread day analysis. These climatologies extend to 2020 due to available weather data. Maximum daily HDWI values within this time-period were selected to represent that day of May in each year.
A climatology for each of the three locations was constructed to show Maximum Daily HDWI, Mean Daily HDWI, Minimum Daily HDWI, 95th Percentile, 90th Percentile, 75th Percentile and 50th Percentile of HDWI for May (1990–2020). These climatologies were built to provide multiple thresholds of HDWI for indications of moderate to extreme fire weather potential at these specific locations in Alberta.
Results
Spread day analysis
Our analysis determined that the difference between HDWI medians of the two non-normal distributions is statistically significant for the first 4 days on these 80 large wildland fires in May. HDWI values were greater on the spread days than non-spread days (P < 0.01). The 114 observations included 66 spread days and 48 non-spread days. The median HDWI values were 94.5 and 70.9 for spread and non-spread days, respectively.
The distributions of HDWI values on spread days and non-spread days were not significantly different when Day 1 and Days 2–4 were isolated (P = 0.06 and P = 0.13, respectively) (Table 2). There were 69 observations (42 pread days, 27 non-spread days) of a possible 80 on Day 1, and 45 (24 spread days and 21 non-spread days) for Days 2–4, from a total possible 240. The small sample sizes of spread days and non-spread days for these two groups may have impacted results. The maximum HDWI value for non-spread days over days 2–4 was 307.6 (Table 3), which is 325% greater than the median HDWI spread day value. This one sample represents 4.7% of non-spread days for Days 2–4. This fire on this day grew 9000 ha, but did not double in area burned, and therefore was not considered a spread day. This represents a limitation to the methods chosen. Additionally, though it is assumed that these large fires were very difficult to manage, in some instances suppression may have had some impact on overall growth on Days 2–4, in that growth on flanks or near the head was slowed due to suppression, even on elevated HDWI days. The lack of multiple size updates over Days 2–4 may be the result of ongoing escaped fire operations, where providing one report per day is sufficient for gaining an understanding of the changing complexity of a fire. ISI.F and ISI.AR did not have significantly different distributions between spread days and non-spread days as defined in the approach chosen (Table 2). In all cases, ISI was elevated (>10) and had little variation between spread days and non-spread days.
Case | Number of observations | Median | Permutation test | |||
---|---|---|---|---|---|---|
Spread days | Non-spread days | Spread days | Non-spread days | P-value | ||
HDWI all 4 days | 66 | 48 | 94.5 | 70.9 | <0.01 | |
HDWI Day 1 only | 42 | 27 | 98.3 | 74.9 | 0.06 | |
HDWI Days 2–4 | 24 | 21 | 88.4 | 67.8 | 0.13 | |
ISI.F all 4 days | 66 | 48 | 10.2 | 10.7 | 0.65 | |
ISI.F Day 1 only | 42 | 27 | 10.1 | 10.4 | 0.73 | |
ISI.F Days 2–4 | 24 | 21 | 10.3 | 12.4 | 0.14 | |
ISI.AR all 4 days | 66 | 48 | 13.5 | 12.7 | 0.63 | |
ISI.AR Day 1 only | 42 | 27 | 13.5 | 12.0 | 0.26 | |
ISI.AR Days 2–4 | 24 | 21 | 13.6 | 12.7 | 0.85 |
Spread days | Non-spread days | ||||||||||
---|---|---|---|---|---|---|---|---|---|---|---|
25th | 95th | 25th | 95th | ||||||||
Case | Min | Percentile | Median | Percentile | Max | Min | Percentile | Median | Percentile | Max | |
HDWI all days | 25.9 | 71.5 | 94.5 | 199.8 | 304.7 | 3.1 | 53.8 | 70.9 | 159.2 | 307.6 | |
HDWI Day 1 | 29.8 | 73.2 | 98.3 | 240.1 | 304.7 | 3.1 | 50.8 | 74.9 | 159.9 | 186.0 | |
HDWI Days 2–4 | 25.9 | 70.9 | 88.4 | 134.0 | 137.1 | 18.4 | 63.8 | 67.8 | 148.8 | 307.6 |
HDWI and ISI value and percentiles on spread days and non-spread days are in Tables 3 and 4, respectively. ISI values were elevated through both spread days and non-spread days, with the medians, and in most cases even 25th percentiles, being in the very high (8.1–15.0) to extreme (15.1+) ranges as defined by the FWI Code Hazard Levels for Alberta (Government of Alberta 2023) (Table 4). Median ISI.F values were greater on non-spread days than on spread days.
Spread days | Non-spread days | ||||||||||
---|---|---|---|---|---|---|---|---|---|---|---|
25th | 95th | 25th | 95th | ||||||||
Case name | Min | Percentile | Median | Percentile | Max | Min | Percentile | Median | Percentile | Max | |
ISI.F all 4 days | 1.0 | 6.7 | 10.2 | 32.0 | 40.6 | 2.9 | 8.0 | 10.7 | 23.2 | 46.0 | |
ISI.F Day 1 | 1.0 | 6.3 | 10.1 | 37.2 | 40.6 | 3.0 | 8.3 | 10.4 | 19.3 | 21.0 | |
ISI.F Days 2–4 | 1.4 | 7.4 | 10.3 | 17.3 | 20.0 | 2.9 | 8.0 | 12.4 | 38.5 | 46.0 | |
ISI.AR all 4 days | 0.1 | 8.3 | 13.5 | 45.6 | 68.4 | 1.9 | 9.7 | 12.7 | 39.8 | 50.9 | |
ISI.AR Day 1 | 0.1 | 5.8 | 13.5 | 43.8 | 68.4 | 1.9 | 8.4 | 12.0 | 30.5 | 41.7 | |
ISI.AR Days 2–4 | 0.8 | 9.9 | 13.6 | 43.7 | 49.3 | 5.8 | 10.4 | 12.7 | 50.1 | 50.9 |
HDWI forecasting
The core period of maximum HDWI occurrence of 16:00–19:00 hours MDT (Fig. 2) is consistent with weather observed in Alberta. The randomised distribution of the maximum HDWI using a bootstrap with 10,000 replicates resulted in a mean of 17:01 hours MDT. Our hypothesised time of maximum HDWI (17:00 hours MDT) lies within the bootstrapped 95% confidence interval. Additionally, the median time of maximum HDWI occurrence from the 320 observations was 17:00 hours MDT. This is consistent with the peak burn time of 17:00 hours MDT in Alberta. However, the timeframe of 16:00–19:00 hours MDT where the time of maximum HDWI occurrence peaks is the recommended forecast timeframe for the purpose of capturing wind events over a few hours, instead of using a 1-h time observation.
May HDWI climatology
A 31-year climatology was produced for May for three separate locations in Alberta, Canada (1990–2020). These HDWI climatologies are specific to the ERA5 dataset, a 0.25° × 0.25° grid cell size, and the locations selected (Figs 3, 4, 5, and Table 5). We believe these climatologies can provide valuable information to fire management officials to forecast HDWI and potential dangerous fire behaviour days (McDonald et al. 2018).
HDWI May Climatology (1990–2020) for Fort McMurray, Alberta (56.75°N, 111.50°W) with significant HDWI values and year of occurrence. Notable peaks in maximum HDWI are indicated with the year followed by the observed HDWI value in brackets.
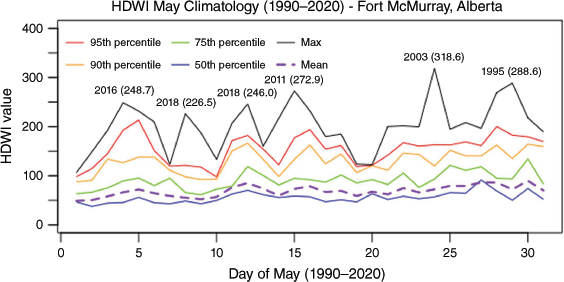
HDWI May Climatology (1990–2020) for Peace River, Alberta (56.25°N, 117.25°W) with significant HDWI values and year of occurrence. Notable peaks in maximum HDWI are indicated with the year followed by the observed HDWI value in brackets.
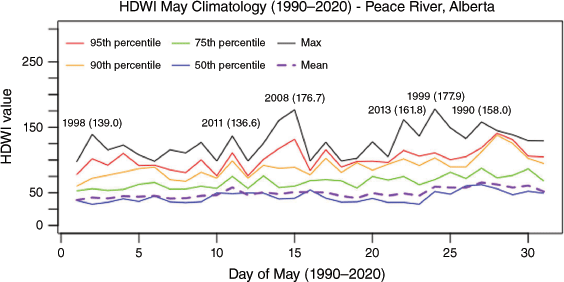
HDWI May Climatology (1990–2020) for Slave Lake, Alberta (55.25°N, 114.75°W) with significant HDWI values and year of occurrence. Notable peaks in maximum HDWI are indicated with the year followed by the observed HDWI value in brackets.
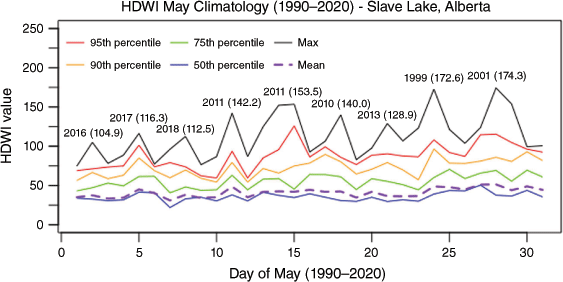
Min | 50p | Mean | 75p | 90p | 95p | Max | ||
---|---|---|---|---|---|---|---|---|
Combined | 0.6 | 44.2 | 53.1 | 69.6 | 101.9 | 127.7 | 318.6 | |
Fort McMurray | 1.4 | 56.3 | 68.9 | 91.2 | 133.7 | 164.4 | 318.6 | |
Peace River | 2.1 | 43.9 | 49.6 | 66.5 | 92.6 | 108.9 | 177.9 | |
Slave Lake | 0.6 | 35.0 | 40.4 | 56.1 | 76.5 | 91.4 | 174.3 | |
Mean | 1.4 | 45.1 | 53.0 | 71.3 | 100.9 | 121.6 | 223.6 |
The values for Fort McMurray are noticeably greater than the other locations (Table 5). Over the 31-year climatology of the maximum wind speed used for HDWI calculation, Fort McMurray had an average wind speed of 4.4 m s−1, where Peace River and Slave Lake were 3.1 and 2.7 m s−1, respectively. With respect to the VPD used in HDWI calculations, values were 11.1, 11.7 and 10.9 hPa for Fort McMurray, Peace River and Slave Lake, respectively. Fort McMurray’s HDWI values were greater due to increased wind speeds in that location. The 90th percentile when all three HDWI climatologies were combined (101.9), as well as the mean of all three locations (100.9), are only slightly higher than the median HDWI value on spread days of 94.5. The median HDWI spread day value of 94.5 are in Table 2 is 78.0% greater than the mean climatology value of 53.1 when all three stations are combined. Additionally, the median HDWI spread day value is 35.8% greater than the climatological 75th percentile value of 69.6 and only 7.8% lower than the climatological 90th percentile value of 101.9.
These May climatologies can assist fire weather forecasters, fire management officials and firefighters working on the ground by providing additional information for determining days that may present increased or dangerous spring fire weather conditions in Alberta.
Discussion
From 1990 to 2019, Alberta experienced almost 38,000 wildland fires which burned approximately 5.25 million ha (Government of Alberta 2019). A total of 23.3% started in the month of May and 78.7% of these were caused by human activity (Government of Alberta 2019). Wildland fires that started in May during this time-period accounted for 48.7% of the total annual area burned (Government of Alberta 2019).
Of the main contributors to large wildland fire growth in the spring, FFMC and wind (Tymstra et al. 2019), we chose to focus on the effect of strong, dry winds on the spring fire environment. Though extreme surface winds are not the only prerequisite for large fire growth, these winds increase the likelihood that a fire will escape suppression and grow to large sizes (Beverly et al. 2011). HDWI has shown to represent spread day potential during spring in Alberta and can be a useful tool for prevention, preparedness and suppression planning.
Currently, atmospheric indices are not used as indicators of fire behaviour potential in Alberta. HDWI was developed to capture the effect of hot, dry and windy conditions present in the lower levels of the atmosphere and their impact on fire growth. As outlined in the Flat Top Complex Wildfire Review Committee (2012), the need for improved prediction of high wind and dry air events that result in dangerous fire weather conditions is imperative. HDWI does not examine the impacts of the fuel complex on fire behaviour or spread as it is a weather-only index. Therefore, HDWI is not linked to the FWI System or the FBP System.
The physical drivers of HDWI (WS and VPD) have profound effects on fire behaviour including increasing the rate at which fuels dry and the spread rate of an ongoing fire. Local levels of atmospheric stability are also an important factor in the wildland fire environment, including wind speed and atmospheric mixing in response to instability. It is reasonable to assume that near-surface mixing from late afternoon onward makes it possible that WS and VPD up to 500 m above the surface may affect a fire any point in the day (Srock et al. 2018). The mesoscale weather systems used to calculate HDWI make it difficult to forecast local occurrences such as local mixing, local wind shifts, or the occurrence of a low-level jet. If these local occurrences are deemed possible, they should be examined and incorporated into HDWI calculations to capture growth potential for operational purposes. Calculations using ERA5 data may be misrepresented due to large grid sizes. Actual HDWI values may have been greater than calculated in our analysis due to an averaging effect over large grid cells. Therefore, HDWI thresholds found here may be lower than what will be forecasted or observed. The increased spatial resolution of ERA5-LAND may provide increased accuracies.
ISI was not significant in determining spread days in this analysis, likely due to little variation of values between spread days and non-spread days. Though it was assumed that suppression would not have been effective on main areas of growth, efforts can still restrict overall growth. However, one may expect HDWI to also display these results. Though we would expect ISI to be the primary driver of fire spread in the spring fire environment in Alberta, this suggests that ISI may not be the only indicator of potentially dangerous spring fire behaviour. This supports our initial hypothesis that there is more to spring fire spread potential than fine fuel moisture content and 10 m open wind speed, and that lower levels of the atmosphere may directly impact fire spread. The lack of significant cases with respect to ISI is consistent with Hanes et al. (2020), who found that ISI had a positive relationship with the probability of a fire escaping initial attack and growing to over 4 ha in size, but only in two of four Forest Areas examined in Alberta (1979–2018). ISI values over the first 4 days of these large May fires are elevated (median ISI >10 on spread days and non-spread days). Wind events after 12:00 hours LST are not captured by ISI calculations, whereas HDWI values forecasted/calculated throughout the day may capture these events.
The median ISI.F value for non-spread days was in the very high range (8.1–15.0). One would expect these ISI values to result in significant fire growth that did not occur on the non-spread days. Therefore, it is recommended HDWI be used as an indicator of spring fire growth in conjunction with ISI. For example, a spread day may be expected for a new start (e.g. Grow to 200 ha+ on Day 1) when the HDWI exceeds the median spread day HDWI value of 94.5, and ISI is 8.7 or greater as in Podur and Wotton (2011), 9.0 or greater as in Tymstra et al. (2021) or, though not statistically significant, greater than the median ISI.F on spread days found here of 10.2.
HDWI values for the first 4 days of the 2016 Horse River Wildfire were calculated and compared to the HDWI climatology (Table 6). This fire was the costliest natural disaster in Canadian history to date (MNP 2017). On three of the first 4 days, HDWI values exceeded the daily 95th percentile value, and exceeded the May 90th percentile on Day 2. The observed HDWI values on 1, 3, and 4 May 2016 (Day 1, Day 3, and Day 4, respectively), were the maximum HDWI values recorded in Fort McMurray from 1990 to 2020 on those specific days of May. The only day with a HDWI value below the daily 95th percentile was 2 May 2016 (Day 2). However, the calculated HDWI value (112.8) was above the median spread day value (94.5) from our analysis.
2016 | Min | 50p | Mean | 75p | 90p | 95p | Max | ISI.F | ISI.AR | ||
---|---|---|---|---|---|---|---|---|---|---|---|
May | – | 1.4 | 56.3 | 68.9 | 91.2 | 133.7 | 164.4 | 318.6 | – | – | |
1 May | 106.5 | 15.0 | 46.8 | 48.9 | 63.5 | 87.6 | 98.1 | 106.5 | 14.7 | 13.1 | |
2 May | 112.8 | 14.1 | 37.8 | 50.5 | 66.6 | 90.9 | 114.8 | 148.9 | 7.6 | 8.8 | |
3 May | 192.5 | 9.0 | 44.4 | 58.1 | 75.4 | 134.1 | 145.0 | 192.5 | 14.8 | 12.2 | |
4 May | 248.7 | 2.3 | 45.7 | 66.5 | 89.4 | 126.7 | 192.8 | 248.7 | 11.0 | 12.6 |
ISI.F and ISI.AR were in the very high range for all days except 2 May when ISI.F was 7.6 (High). On 2 May, values did not exceed very high ISI or the 95th Percentile HDWI value; however, the spread day threshold of 94.5 was exceeded and resulted in >100% relative growth (spread day). The only day in the first four that was classified as a spread day due to observed growth and not having at least two size updates on Days 3 and 4 was also 2 May.
The primary application of HDWI is to provide additional support for decision making. Forecasting extreme fire weather is the first step in preventing the potential impacts a human-caused fire could have on the public. Wildland fire prevention measures need to be implemented during extreme fire weather and fire behaviour conditions (Flat Top Complex Wildfire Review Committee 2012) for the purpose of mitigating human-caused fires. From a wildland fire prevention standpoint, even if very severe fire weather is forecasted or experienced, there will be no area burned if there is no ignition (Flannigan et al. 2005). If HDWI and ISI thresholds for spread days are forecasted, prevention methods should be employed. Though the prevention system can take time to be implemented, fire prevention methods ‘need to be strategically applied and removed quickly’ (Tymstra et al. 2021, p. 833), therefore, the implementation of these methods also need to be improved. Additionally, lightning caused fires are not preventable and can cause great challenges if ignited under similar conditions to those examined in this analysis.
The recommended daily forecast period for HDWI is 16:00–19:00 hours MDT due to the pronounced peak of maximum HDWI observed for the month of May (1990–2020) (Fig. 2). Forecasting HDWI over this period will ensure abrupt changes in weather such as wind events be captured, rather than only forecasting a 1-h period such as 17:00 hours MDT. To add context to the idea that higher HDWI values result in increased fire behaviour, forecasted HDWI values should be evaluated in reference to the spread day threshold found in this analysis (94.5), May climatologies, or local long-term trends (Killough 2021). It is recommended that HDWI be analysed hourly to identify days and periods of days where fire weather conditions may present dangerous fire behaviour. HDWI climatologies using the Climate Forecast System Reanalysis (CFRS) from NCEP, and forecasts using the Global Ensemble Forecast System (GEFS) are also available from the United States Department of Agriculture (2022) HDWI Real-Time Product Suite website (https://hdwindex.fs2c.usda.gov/abouthdw.html). HDWI is a complementary tool to ISI and outputs of the FBP system for predicting wildland fire spread events in Alberta.
Conclusion
Wildland fire prevention, preparedness and suppression planning in Alberta focuses on the FWI and FBP Systems. These only account for noon surface weather conditions and noon surface weather forecasts. Weather variables and fire weather indices in the lower levels of the atmosphere beyond the surface are not used. In this study on the HDWI in Alberta, the first study of this topic, we examined HDWI on spring wildland fire spread events in Alberta, Canada, to account for atmospheric conditions aloft. We also recommend a forecast timeframe for its application, and provide three climatologies for northern boreal regions.
Higher HDWI values were observed for the first 4 days of 80 large spring wildland fires in Alberta that occurred from 1990 to 2019. A spread day is likely to occur during the first 4 days when HDWI is 94.5 or greater. HDWI provides an enhanced understanding of how other layers of the atmosphere affect fire behaviour. We also examined ISI values as this index is an important output of the FWI System and is the primary value used during spring fire season. ISI value distributions were not significantly different between spread days and non-spread days. This was presumably due to the consistently elevated ISI values over these 80 large spring wildland fires that resulted in little variation between spread days and non-spread days. Though ISI was not significant, we consider this index as an important index to track spring fire spread particularly in the spring.
In this study, we also developed May HDWI climatologies for three locations in Alberta: (1) Fort McMurray; (2) Slave Lake; and (3) Peace River. The three compiled HDWI climatologies provide valuable context information for Alberta fire management staff during in the month of May. A standard forecasting time-period of 16:00–19:00 hours is recommended for daily maximum HDWI observations in May from 1990 to 2020.
Recommendations for future work include the expansion of the spring study period into June, as well as throughout the entirety of the fire season and across Canada. HDWI values that determine fire spread may differ throughout the summer months and across the country. Future work should also include the application of more accurate fire size data, which includes the examination of HDWI using current methods of determining fire size such as MODIS/VIIRS. The higher resolution ERA5-LAND reanalysis data may also provide more accurate weather values. To potentially increase the effectiveness of HDWI at capturing the influence of winds aloft on surface winds, HDWI could include the expansion of the atmospheric level deeper than 500 m above the surface.
Data availability
Historical wildfire data in Alberta is available publicly at https://www.alberta.ca/wildfire-maps-and-data. See references below for availability of ERA5 weather data from the CS3 CDS. Weather and Canadian FWI System indices from the Alberta Forestry Division weather network are available via request to the WMB in Edmonton, Alberta at WF.AWCC-Weather@gov.ab.ca. This paper forms part of the Masters thesis of Elliott (2023).
Conflicts of interest
Mike D. Flannigan is an Associate Editor of the International Journal of Wildland Fire. To mitigate this potential conflict of interest, he had no editor-level access to this manuscript during peer review. The authors declare no other conflicts of interest.
Acknowledgements
We thank Alberta Forestry and Parks, Wildfire Management Branch, for providing wildfire report and weather data.
References
Alexander ME (2010) Surface fire spread potential in trembling aspen during summer in the Boreal Forest Region of Canada. The Forestry Chronicle 86(2), 200-212.
| Crossref | Google Scholar |
Beverly JL, Flannigan MD, Stocks BJ, Bothwell P (2011) The association between Northern Hemisphere climate patterns and interannual variability in Canadian wildfire activity. Canadian Journal of Forest Research 41, 2193-2201.
| Crossref | Google Scholar |
Bolton D (1980) The computation of equivalent potential temperature. Monthly Weather Review 108, 1046-1053.
| Crossref | Google Scholar |
Chrosciewicz Z (1986) Foliar moisture content variations in four coniferous tree species of central Alberta. Canadian Journal of Forest Research 16, 157-162.
| Crossref | Google Scholar |
CDS (Copernicus Climate Data Store) (2021) ERA5 hourly data on pressure levels from 1959 to present. Available at https://cds.climate.copernicus.eu/datasets/reanalysis-era5-pressure-levels?tab=overview
Flannigan MD, Logan KA, Amiro BD, Skinner WR, Stocks BJ (2005) Future area burned in Canada. Climatic Change 72, 1-16.
| Crossref | Google Scholar |
Flannigan MD, Stocks B, Turetsky M, Wotton M (2009) Impacts of climate change on fire activity and fire management in the circumboreal forest. Global Change Biology 15, 549-560.
| Crossref | Google Scholar |
Flat Top Complex Wildfire Review Committee (2012) Flat Top Complex Review. Environment and Sustainable Resource Development, May 2012. Available at https://open.alberta.ca/publications/9781460102732
Government of Alberta (2019) ‘Historical data.’ (Alberta Forestry, Parks and Tourism, Wildfire: Edmonton, AB, Canada) Available at https://www.alberta.ca/wildfire-maps-and-data.aspx#jumplinks-2
Government of Alberta (2023) ‘Fire Weather Index Legend.’ (Alberta Forestry and Parks, Alberta Wildfire: Edmonton, AB, Canada) Available at https://www.alberta.ca/fire-danger
Hanes CC, Wang X, Jain P, Parisien M-A, Little JM, Flannigan MD (2018) Fire-regime changes in Canada over the last half century. Canadian Journal of Forest Research 49, 256-269.
| Crossref | Google Scholar |
Hanes CC, Wotton BM, Woolford DG, Martell DL (2020) Preceding fall drought conditions and overwinter precipitation effects on spring wildland fire activity in Canada. Fire 3, 24.
| Crossref | Google Scholar |
Killough D (2021) Hot-Dry-Windy Index offers improvement for fire prediction. Forestnet.com, TimberWest Magazine, January/February Issue: 34–36. Available at https://issuu.com/forestnet2/docs/01-02.21_tw_issueweb
McDonald JM, Srock AF, Charney JJ (2018) Development and application of a Hot-Dry-Windy Index (HDW) climatology. Atmosphere 9, 285.
| Crossref | Google Scholar |
McElhinny M, Beckers JF, Hanes C, Flannigan MD, Jain P (2020) A high-resolution reanalysis of global fire weather from 1979 to 2018 – overwintering the Drought Code. Earth System Science Data 12, 1823-1833.
| Crossref | Google Scholar |
Parisien M-A, Barber QE, Flannigan MD, Jain P (2023) Broadleaf tree phenology and springtime wildfire occurrence in boreal Canada. Global Change Biology 29, 6106-6119.
| Crossref | Google Scholar | PubMed |
Podur J, Wotton BM (2011) Defining fire spread event days for fire-growth modelling. International Journal of Wildland Fire 20, 497-507.
| Crossref | Google Scholar |
R Core Team (2020) ‘R: A language and environment for statistical computing.’ (R Foundation for Statistical Computing: Vienna, Austria) Available at https://www.R-project.org/
Srock AF, Charney JJ, Potter BE, Goodrick SL (2018) The Hot-Dry-Windy Index: a new fire weather index. Atmosphere 9, 279.
| Crossref | Google Scholar |
Stocks BJ, Mason JA, Todd JB, Bosch EM, Wotton BM, Amiro BD, Flannigan MD, Hirsch KG, Logan KA, Martell DL, Skinner WR (2002) Large forest fires in Canada, 1959-1997. Journal of Geophysical Research 108, 8149 2002.
| Crossref | Google Scholar |
Tymstra C, Woolford DG, Flannigan MD (2019) Statistical surveillance thresholds for enhanced situational awareness of spring wildland fire activity in Alberta, Canada. Journal of Environmental Statistics 9(4), 1-26.
| Google Scholar |
Tymstra C, Jain P, Flannigan MD (2021) Characterisation of initial fire weather conditions for large spring wildfires in Alberta, Canada. International Journal of Wildland Fire 30, 823-835.
| Crossref | Google Scholar |
United States Department of Agriculture (2022) Hot-Dry-Windy Index. Real-Time Product Suite website. Available at https://hdwindex.fs2c.usda.gov/abouthdw.html
Wang X, Thompson DK, Marshall GA, Tymstra C, Carr R, Flannigan MD (2015) Increasing frequency of extreme fire weather in Canada with climate change. Climatic Change 130, 573-586.
| Crossref | Google Scholar |
Wexler A (1976) Vapor pressure formulation for water in range 0 to 100°C. A revision. Journal of Research of the National Bureau of Standards—A. Physics and Chemistry 80A, 775-785.
| Crossref | Google Scholar | PubMed |
Wotton BM (2009) Interpreting and using outputs from the Canadian Forest Fire Danger Rating System in research applications. Environmental Ecology Statistics 16, 107-131.
| Crossref | Google Scholar |