Effect of vented helmets on heat stress during wildland firefighter simulation
Katherine Christison A , Shae Gurney A and Charles L. Dumke
A School of Integrative Physiology and Athletic Training, University of Montana, Missoula, MT 59812, USA.
B Corresponding author. Email: charles.dumke@umontana.edu
International Journal of Wildland Fire 30(9) 645-651 https://doi.org/10.1071/WF20182
Submitted: 5 December 2020 Accepted: 4 June 2021 Published: 6 July 2021
Journal Compilation © IAWF 2021 Open Access CC BY-NC-ND
Abstract
Uncompensable heat from wildland firefighter personal protective equipment decreases the physiological tolerance while exercising in the heat. Our previous work demonstrated that the standard wildland firefighter helmet significantly increases both perceived and actual head heat. This study compared heat accumulation under simulated working conditions while wearing a standard non-vented helmet versus a vented helmet. Ten male subjects randomly completed two trials separated by a 2-week washout. Subjects walked 180 min (5.6 km h−1, 5% grade) in a heat chamber (35°C, 30% relative humidity) broken into three segments of 50 min of exercise and 10 min rest, followed by a work capacity test to exhaustion. Each trial measured the physiological strain index, perceived head heat, helmet temperature and relative humidity, rating of perceived exertion and heart rate. At the end of the 3-h trial heart rate, physiological strain, perceived exertion, helmet temperature and humidity showed the main effects of time (P < 0.05) but were not different between trials. Work capacity was significantly greater in the vented trial (P = 0.001). End-trial strain and heart rate were significantly related to work performed (r = –0.8, P < 0.001). Elevated work, trends for changes in perceived exertion, helmet microenvironment and perceived head heat suggest greater heat dissipation and comfort with the vented helmet.
Keywords: environmental health, heat-related injuries, interagency hotshot crew, occupational physiology, personal protective equipment, physiological strain index, skin blood flow.
Introduction
The daily workload of wildland firefighters (WLFFs) involves long, strenuous shifts, with the addition of heat stress from personal protective equipment (PPE). Previous studies have demonstrated that PPE contributes to heat accumulation (Montain et al. 1994; Budd et al. 1997), specifically wildland firefighter PPE (Domitrovich 2014; Carballo-Leyenda et al. 2018). Despite evidence that the human head is a major source of heat loss (Rasch et al. 1991; Katsuura et al. 1996), there is a paucity of research on the effect of occupational helmets on heat accumulation and development of heat-related illness. Evidence from sporting helmets suggests an effect on the head microenvironment, blood flow and overall performance. In 2012, Bogdan et al. found that a motorcycle helmet significantly increased head and core temperatures, in addition to thermal sensations (Bogdan et al. 2012). Investigations have shown that the American football helmet increases the rate of core temperature elevation during exercise while decreasing the time to exhaustion (Armstrong et al. 2010) and impairments in cognition have also been observed in cricketers (Neave et al. 2004). It was also found that an unvented aerodynamic bicycle helmet resulted in greater head temperature elevation compared with a vented traditional helmet (Lee et al. 2013). A previous investigation by our group found the WLFF helmet contributes to heat accumulation, perceived head heat and redirection of blood flow to the head compared with not wearing a helmet (Gurney et al. 2021). However, as WLFF are subject to blows to the head, the absence of a helmet is not a consideration in the field (Apuzzo et al. 2020).
The standard WLFF helmet meets the American National Standards Institute (ANSI) Z89.1 requirements for Type I, Class G head protection. Type I helmets are intended to reduce the force of impact resulting from a blow to the top of the head. Class G helmets are intended to reduce the danger of contact with low-voltage conductors. Due to this electrical rating requirement, current WLFF helmets do not have vents. However, a vented helmet is likely to improve the ability to dissipate heat and reduce uncompensable heat strain, a more pertinent problem within the WLFF community. Therefore, the aim of this study was to compare a standard issue WLFF helmet to a ventilated helmet on heat stress factors and a work capacity test while exercising in the heat.
Materials and methods
Subjects
Ten healthy male subjects (23.8 ± 3.2 years, 180.8 ± 0.9 cm, 77.7 ± 2.2 kg, 59.8 ± 3.6 mL kg−1 min−1 VO2max, 12.1 ± 0.03% body fat) were recruited for participation in the study. Each subject was required to complete a participation readiness questionnaire (PAR-Q+) and signed an informed consent form approved by the University of Montana Institutional Review Board (181-17). Subject inclusion requirements were: male, >63.6 kg, with a VO2max >40 and <65 mL kg−1 min−1.
Experimental design
This study had a randomised crossover design, where subjects exercised for 180 min in a heat chamber with either a WLFF helmet (WH) or a helmet with ventilation (VH) (Fig. 1) on separate occasions. Testing was conducted in Missoula, MT, from October to December 2018. This time period was chosen to avoid heat acclimation to ambient weather. Ten male subjects were recruited and completed both trials in a counterbalanced design. An initial visit was completed to obtain body composition and maximal aerobic capacity testing data. During the two experimental trials, subjects were required to finish a 180-min exercise protocol in a heat chamber (35°C and 30% relative humidity) following an overnight fast, wearing meta-aramid shirt and pants, cotton t-shirt and either a current ANSI Z89.1, Type I, Class G (nonconductive, low-voltage) WH or an ANSI Z89.1, Type I, Class C (conductive), EN-12492 VH.
![]() |
The trials involved 3 × 50 min exercise segments at 5.6 km h−1 and 5% grade with 10 min rest following each segment, followed by a work capacity test to exhaustion (Fig. 2). The intensity was chosen to mimic WLFF ingress hiking conditions as a crew (Sol et al. 2018). Water was provided at a rate of 5 mL kg−1 30 min−1. A 2-week washout period separated the trials to prevent acclimation. Skin blood flow to the neck and forehead (SBFN, SBFH respectively), core temperature (TC), average skin temperature of the chest, scapula and neck (TSK), heart rate (HR), physiological strain index (PSI), rating of perceived exertion (RPE), perceived head heat (PHH), helmet temperature (THELMET) and humidity (HHELMET) and sweat rate (SR) were recorded during trials. American College of Sports Medicine guidelines were followed to determine if an exercise test should be stopped (Riebe et al. 2018).
Body composition
Body composition was measured using a hydrostatic weighing tank with three force transducers and data-collecting software (Exertech) while estimating residual volume from the subject’s height, age and weight. Subjects arrived fasted for ≥ 8 h before body density assessment. Subjects were submerged and weighed repeatedly until consistent measurements were recorded within 0.1 kg of each other. Underwater body weight (BW) was used to calculate body density and predict body fat percentage using the Siri equation (Siri 1956).
Maximal aerobic capacity
A maximal exercise test was administered for each subject. Subjects were instructed to have fasted for ≥3 h before the VO2max test. The standard Bruce Protocol graded exercise test to volitional fatigue was conducted. Every 15 s mixed expired gases were collected via a metabolic cart (Parvomedics, Inc.).
Body weight
Nude BW was measured in private on a calibrated scale (Salter Brecknell) to the nearest 0.01 kg. Weights were taken before and after each trial and were used to calculate percent dehydration and SR:

Heart rate/rating of perceived exertion
HR was measured using a Polar heart rate strap and wrist monitor (Polar Electro Inc., Lake Success, NY, USA). RPE was recorded using the Borg scale (6–20). RPE was recorded every 25 min until the end of the 180-min trial. HR was measured continuously throughout the exercise protocol, but was analysed at 25-min time stamps for consistency with statistical analysis.
Perceived head heat
PHH was recorded using a 15-cm visual analogue scale, with 15 equally spaced dashes. Subjects marked the continuous scale to record how hot their head felt; that mark was then measured using a ruler to give nominal value to PHH. PHH was recorded every 25 min of the protocol until the end of the 180-min trial.
Skin temperature/core temperature
Skin and core temperatures were continuously recorded using DASYLab software (Measurement Computing Co.). Skin probes and patches were attached to chest, scapula, and neck sites (Physitemp). Chest temperature probe was placed 5 cm medial and 5 cm superior to the left nipple. Neck temperature probe was placed on the left side of the neck between the sternocleidomastoid and trapezius muscles below the hairline. Scapula temperature probe was placed directly above the inferior angle of the scapula. Core temperature was obtained using a rectal probe placed 10 cm into the rectum (Physitemp). Temperatures were time synchronised and continuously recorded for the duration of the trials using DASYLab software. Skin temperature at the three locations were equally weighted and averaged to obtain mean TSK at each time point:

Skin blood flow
SBF was obtained via laser Doppler flowmetry (Moor Instruments) for 5 min following each 50-min bout of exercise. A wand was placed on the back of the neck and on the forehead, above the right eyebrow, for blood flow measurements. During collection times, subjects were seated in the heat chamber and asked to remain as still and quiet as possible. Time-synchronised SBF data were downloaded by DASYLab software (Measurement Computing Co.). SBF data is expressed as arbitrary units (au).
Chamber environment
Environmental conditions within the chamber were set to 35°C and 30% relative humidity and continuously monitored throughout all laboratory testing sessions. In order to simulate wind, fans were strategically placed in the chamber to mimic air passing over the helmet, as convection is a crucial component to heat dissipation. Wind speed was controlled to match the rate of walking (5.6 km h−1) and verified via a Kestrel 5500 Weather Meter Pro placed directly in front of the helmet (Nielsen-Kellerman Co.).
Helmet microenvironment
Temperature inside the helmet was recorded using DASYLab software (Measurement Computing Co.). The probe was placed inside the helmet, oriented to sit between the helmet and the top of the head. HHELMET was recorded using a Tempi environment sensor (Tahmo Inc.). The Bluetooth Tempi sensor was attached to the webbing inside the helmet with the sensor facing into the helmet space and continuous measurements were taken and recorded using the corresponding smartphone application.
Work capacity test
A work capacity test to volitional fatigue was completed following the final 10-min rest period of the 180-min exercise bout. Speed and grade were set to 5.6 km h−1 and 6.0%, with grade increasing every minute until a maximal 15.0% grade was reached. Once 15% grade was achieved, speed was increased 1.6 km h−1 each minute until exhaustion. Total work performed was converted to kilojoules (KJ).
Physiological strain index
PSI was calculated from resting TC (Tc0) and HR (HR0) compared with a given exercise time point using the equation derived from Moran et al. (1998):

Sweat rate
SR was calculated using pre- and post-exercise body weights (BW) and corrected for urine production, fluid intake, and respiratory water loss (Mitchell et al. 1972). SR is expressed relative to body surface area:
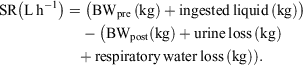
Respiratory water loss:

where Me = rate of evaporative loss (g min−1), and Pa = water vapour pressure (mmHg).
Water pressure was calculated using the following equation:

where T = temperature (°C).
Statistical analysis
Repeated-measures analysis of variance (ANOVA) was used to analyse data. A 2 (treatment) × 6 (time) repeated-measures ANOVA was used to analyse HR, RPE, PHH, PSI, TC, TSK, THELMET and HHELMET. A 2 (treatment) × 3 (time) repeated-measures ANOVA was used to analyse SBFH and SBFN during the trials. A one-way ANOVA was used to assess SR and % dehydration. Two-tailed Pearson correlations were used to analyse relationships between markers of heat stress and performance. Statistical significance was set at a probability of type I errors less than 5% (P < 0.05). All data are presented as mean ± standard error of the mean (s.e.m.). Analyses were conducted using SPSS data analysis software v.22 (SPSS Inc.).
Results
A comparison of the WH and VH helmet characteristics are summarised in Table 1. The 10 subjects completed both WH and VH trials. Wind speed over the helmet remained constant at 6.3 km h−1 and did not differ between the WH and VH trials. Over the course of the 180-min exercise, a significant main effect for time was seen in HR (P = 0.005), TC (P = 0.001) (Table 2) and PSI (P = 0.002; Fig. 3), but neither trial nor time × trial interaction was seen. TSK showed a main effect of time (P = 0.03), with neither trial nor time × trial interaction. Similarly, there was a main effect of time on both TH and HH (P = 0.003 and P < 0.001 respectively), with a trend towards a main effect of trial in HH (P = 0.087) (Table 2).
![]() |
![]() |
RPE showed main effects for time (P = 0.004) with trends towards trial (P = 0.067), while PHH showed a trend towards a main effect of time (P = 0.065), trial (P = 0.064) and time × trial interaction (P = 0.077) (Table 2). A main effect of time was seen in SBFH (P = 0.01), with a similar response seen in SBFN (P = 0.017, Fig. 4a, b). Change in BW (P = 0.75), subjects’ SR (P = 0.92) and percent dehydration (P = 0.70) were not significantly different between trials (Table 3).
![]() |
![]() |
After completion of the 180-min trial, subjects were able to perform greater amount of work during the VH trial compared with WH (P = 0.01) (Fig. 5). At the completion of the work capacity test, there was a significant difference in HHELMET between the WH and VH (P = 0.037, Table 3). Additionally, PHH was significantly elevated in the WH trial compared with the VH (P = 0.049), but there were no significant differences seen in PSI, THELMET or RPE (Table 3). Pearson correlations were run to determine the key relationships. There was a positive correlation between end-trial PHH and end-trial HR (r = 0.67, P = 0.001). There was a negative correlation between the total work performed during the capacity test with end-trial HR (r = –0.87, P < 0.001) and end-trial PSI (r = –0.80, P < 0.001).
![]() |
Discussion
To the authors’ knowledge, this is the first investigation to establish the effect of an ANSI compliant vented helmet on physiological markers of heat stress during a WLFF simulation, as compared with the current standard WH. Our group has previously established that an unvented helmet results in significant elevations in heat accumulation in those exercising in the heat (Gurney et al. 2021). In the current study, significant alterations in PSI, RPE, THELMET, HHELMET and SBFH were seen during the 180-min trial period, suggesting that WLFF accumulate heat during exercise while wearing standard PPE. Trends for a main effect of trial existed for RPE, PHH and HHELMET, indicating that the current WH design appears to contribute to this heat accumulation and resultant redirection of blood flow to the head compared with a vented helmet. This was best illustrated by the significantly greater amount of work performed during the work capacity test in the VH trial.
These results are supported by previous investigations examining the effect of vented helmets on heat dissipation and physiological strain (Holland et al. 2002; Lee et al. 2013). A study examined differences between a traditional vented cycling helmet and a non-vented aerodynamic helmet in highly trained cyclists at ~39°C while doing either low-intensity cycling (30 min at 50% VO2max) or a self-paced 12-km time trial (Lee et al. 2013). In the low-intensity cycling trial, THELMET of the non-vented helmet was ~0.5°C higher than the traditional vented helmet at the end of the 30 min. Additionally, the aerodynamic helmet resulted in an elevated TC (37.9 ± 0.6°C v. 37.7 ± 0.5°C) throughout the 12-km time trial. Although these trials were completed in a higher ambient temperature and exercise intensity, the brief exercise duration resulted in similar absolute TC and THELMET values as our investigation. Similar to the current study, Holland et al. found significant reductions in helmet temperature and humidity with vented helmets while performing simulated forest harvesting (Holland et al. 2002). All told, these studies suggest helmet vents improve the helmet microenvironment, with subsequent amelioration of heat accumulation and improved performance of the helmet wearer.
The head is a crucial element in offloading heat, as two-fifths to half of the body’s heat loss is dissipated from the head (Rasch et al. 1991). The necessity of this heat loss is highlighted during prolonged, strenuous exercise in the heat, as evident in the 12–16 h shifts of WLFFs. Carballo-Leyenda et al. compared the effect of the addition of PPE (specifically helmet, neck shield, gloves and boots) to current personal protective clothing used by Spanish firefighters with a control group wearing sports gear during extended, graded exercise (shorts, cotton t-shirt, underwear and socks). They found the additional PPE significantly increased the temperature at the skin and in the gastrointestinal tract, PSI and RPE compared with the current personal protective clothing and control condition (Carballo-Leyenda et al. 2018). This resulted in the PPE group working a significantly shorter time to exhaustion than the protective clothing group (62.4 v. 115.5 min), suggesting significant implications to WLFFs during their occupational tasks. In fact, the frequency with which PPE is removed during working hours showed significant relationships with the frequency of heat-related illnesses in 674 Korean firefighters (Kim et al. 2019). Specifically, wearing the helmet at rest showed significant effects on the presence of nausea, vomiting, confusion and on the frequency of dizziness. Together, these highlight the importance of maintaining the head microenvironment during occupational tasks to ensure WLFFs safety and performance.
The design of the current study was set to mimic WLFF conditions, as the extended bouts of exercise were completed in common environmental conditions (35°C and 30% relative humidity) at a standardised speed and grade (5.6 km h−1, 5% grade) to mimic the ingress hike of WLFFs (Sol et al. 2018). Elevations in TC, HR, PSI, SBFH and SBFN under both conditions confirmed the helmet’s contribution to heat accumulation and resultant redirection of blood flow during simulated work. Although these factors did not reach significance between the WH and VH trials, trends existed for RPE, PHH and helmet humidity over the 180-min steady-state trials. These trends could be amplified over the duration of WLFF shifts and result in significant difference over time. Furthermore, no significant differences were observed in SR or % dehydration between trials, eliminating hydration status as a potential confounding factor of these data (Table 2).
Importantly, subjects were able to perform ~15% more work (41 s, 13.6 kJ) during the work capacity test while wearing a vented helmet compared with the unvented WH (Fig. 5). This diverges from the results of Lee et al. whose 2013 study found no differences in power output between a vented standard bicycle helmet and an unvented aerodynamic helmet during a 12-km cycling time trial (Lee et al. 2013). Their shorter study duration in combination with higher power outputs (%VO2max) and higher ambient temperatures may account for this discrepancy. The improvement seen during this WLFF simulation could be attributed to the correlative evidence of better maintenance of HHELMET, HR and PSI with the VH during the 180 min of steady-state exercise. These data were corroborated by corresponding alterations in PHH, with a 16% amelioration in scores in the VH trial at the completion of the performance test. Together these findings suggest that a VH better preserves the microenvironment of the head, which may result in lower perceived head heat and perceived effort.
The inability to blind subjects to each trial condition is an obvious and inherent limitation in this study. The subjects examined during this simulated study were fit and might not be representative of the entire WLFF community, as studies have shown maximal aerobic capacities below the current investigation (Carballo-Leyenda et al. 2018; Gaskill et al. 2020). This may have resulted in a protection from alterations in the physiological responses to extended periods of exercise in the heat. Additionally, although the VH was carefully chosen to best mimic the WH, differences in helmet weights were observed (Table 1), which could have affected the physiological burden of the work and resulting work performed, independent of the presence of vents (Taylor et al. 2012). The authors understand that current United States Forest Service recommendations restrict the use of vented helmets from approved PPE garments due to certain ANSI requirements. Nonetheless, together with our previous investigation (Gurney et al. 2021), we have demonstrated the importance of heat dissipation from the head, which may be ameliorated with the use of a vented helmet.
Further investigations of male individuals at the lower end of the VO2max criteria and of females are crucial to further quantify the physiological responses to wearing a VH. Understanding the practicality of a VH in a WLFF field setting would be beneficial to examine the helmet’s efficacy beyond the laboratory setting. Other considerations may be investigations that address the effect of occasional helmet removal on heat accumulation.
Conclusion
This study is the first to examine performance differences following an extended bout of exercise between the standard WH and VH. Improved measures of physiological heat strain and performance indicated that the VH may improve heat dissipation. The current WH may contribute to heat gain, reduced performance and heat-related illnesses that could affect success and safety under field conditions.
Conflicts of interest
The authors declare that they have no competing interests in access to these data or associations with companies involved with products used in this research. The views and conclusions contained herein are those of the authors and should not be interpreted as necessarily representing the official policies or endorsements, either expressed or implied, of the United States Department of Agriculture Forest Service, National Technology and Development Program or the USA government.
Declaration of funding
This study was supported by a grant from the USDA Forest Service National Technology Development Program, 18-CR-11138100–005.
Acknowledgements
The authors thank the subjects for their investment in time and energy. This study would not have been possible without Tristen Burden, Jesse Long, and John Knight as research assistants in data collection.
References
Apuzzo G, Maclay-Schulte D, West M (2020) Wildland firefighting helmet review whitepaper. 2051–2M01–NTDP. White Paper. USDA Forest Service, National Technology and Development Program: 29 (Missoula, MT, USA)Armstrong LE, Johnson EC, Casa DJ, Ganio MS, McDermott BP, Yamamoto LM, Lopez RM, Emmanuel H (2010) The American football uniform: uncompensable heat stress and hyperthermic exhaustion. Journal of Athletic Training 45, 117–127.
| The American football uniform: uncompensable heat stress and hyperthermic exhaustion.Crossref | GoogleScholarGoogle Scholar | 20210615PubMed |
Bogdan A, Sudol-Szopinska I, Luczak A, Konarska M, Pietrowski P (2012) Methods of estimating the effect of integral motorcycle helmets on physiological and psychological performance. International Journal of Occupational Safety and Ergonomics 18, 329–342.
| Methods of estimating the effect of integral motorcycle helmets on physiological and psychological performance.Crossref | GoogleScholarGoogle Scholar | 22995132PubMed |
Budd G, Brotherhood J, Hendrie A, Jeffery S, Beasley F, Costin B, Zhien W, Baker M, Cheney N, Dawson M (1997) Project Aquarius 6. Heat Load From Exertion, Weather, and Fire in Men Suppressing Wildland Fires. International Journal of Wildland Fire 7, 119–131.
| Project Aquarius 6. Heat Load From Exertion, Weather, and Fire in Men Suppressing Wildland Fires.Crossref | GoogleScholarGoogle Scholar |
Carballo-Leyenda B, Villa JG, Lopez-Satue J, Collado PS, Rodriguez-Marroyo JA (2018) Fractional Contribution of Wildland Firefighters’ Personal Protective Equipment on Physiological Strain. Frontiers in Physiology 9, 1139
| Fractional Contribution of Wildland Firefighters’ Personal Protective Equipment on Physiological Strain.Crossref | GoogleScholarGoogle Scholar | 30154736PubMed |
Domitrovich JW (2014) Wildland fire uniform configurations on physiological measures of exercise heat stress – Health and Safety Report. (Missoula Technology and Development Center: Missoula, MT)
Gaskill SE, Dumke CL, Palmer CG, Ruby BC, Domitrovich JW, Sol JA (2020) Seasonal changes in wildland firefighter fitness and body composition. International Journal of Wildland Fire 29, 294–303.
| Seasonal changes in wildland firefighter fitness and body composition.Crossref | GoogleScholarGoogle Scholar |
Gurney S, Christison K, Stenersen T, Dumke CL (2021) Effect of uncompensable heat from the wildland firefighter helmet. International Journal of Wildland Fire
| Effect of uncompensable heat from the wildland firefighter helmet.Crossref | GoogleScholarGoogle Scholar |
Holland EJ, Laing RM, Lemmon TL, Niven BE (2002) Helmet design to facilitate thermoneutrality during forest harvesting. Ergonomics 45, 699–716.
| Helmet design to facilitate thermoneutrality during forest harvesting.Crossref | GoogleScholarGoogle Scholar | 12437853PubMed |
Katsuura T, Tomioka K, Harada H, Iwanaga K, Kikuchi Y (1996) Effects of cooling portions of the head on human thermoregulatory response. Applied Human Science 15, 67–74.
| Effects of cooling portions of the head on human thermoregulatory response.Crossref | GoogleScholarGoogle Scholar | 8739758PubMed |
Kim S, Kim DH, Lee HH, Lee JY (2019) Frequency of firefighters’ heat-related illness and its association with removing personal protective equipment and working hours. Industrial Health 57, 370–380.
| Frequency of firefighters’ heat-related illness and its association with removing personal protective equipment and working hours.Crossref | GoogleScholarGoogle Scholar | 30210098PubMed |
Lee JF, Brown SR, Lange AP, Brothers RM (2013) Effect of an aerodynamic helmet on head temperature, core temperature, and cycling power compared with a traditional helmet. Journal of Strength and Conditioning Research 27, 3402–3411.
| Effect of an aerodynamic helmet on head temperature, core temperature, and cycling power compared with a traditional helmet.Crossref | GoogleScholarGoogle Scholar | 23539083PubMed |
Mitchell JW, Nadel ER, Stolwijk JA (1972) Respiratory weight losses during exercise. Journal of Applied Physiology 32, 474–476.
| Respiratory weight losses during exercise.Crossref | GoogleScholarGoogle Scholar | 5026494PubMed |
Montain S, Sawka MN, Cadarette B, Quigley M, McKay J (1994) Physiological tolerance to uncompensable heat-stress-effects of exercise intensity, protective clothing, and climate. Journal of Applied Physiology 77, 216–222.
| Physiological tolerance to uncompensable heat-stress-effects of exercise intensity, protective clothing, and climate.Crossref | GoogleScholarGoogle Scholar | 7961236PubMed |
Moran DS, Shitzer A, Pandolf KB (1998) A physiological strain index to evaluate heat stress. The American Journal of Physiology 275, R129–R134.
Neave N, Emmett J, Moss M, Ayton R, Scholey A, Wesnes K (2004) The effects of protective helmet use on physiology and cognition in young cricketers. Applied Cognitive Psychology 18, 1181–1193.
| The effects of protective helmet use on physiology and cognition in young cricketers.Crossref | GoogleScholarGoogle Scholar |
Rasch W, Samson P, Cote J, Cabanac M (1991) Heat loss from the human head during exercise. Journal of Applied Physiology 71, 590–595.
| Heat loss from the human head during exercise.Crossref | GoogleScholarGoogle Scholar | 1938732PubMed |
Riebe D, Ehrman JK, Liguori G, Magal M (2018) ‘ACSM’s Guidelines for Exercise Testing and Prescription.’ (Wolters Kluwer: Philadelphia, PA)
Siri WE (1956) ‘Body composition from fluid spaces and density: analysis of methods.’ LBNL Report No. UCRL-3349. (Radiation Laboratory, University of California: Berkeley, CA)
Sol JA, Ruby BC, Gaskill SE, Dumke CL, Domitrovich JW (2018) Metabolic Demand of Hiking in Wildland Firefighting. Wilderness and Environmental Medicine 29, 304–314.
| Metabolic Demand of Hiking in Wildland Firefighting.Crossref | GoogleScholarGoogle Scholar | 29887347PubMed |
Taylor NA, Lewis MC, Notley SR, Peoples GE (2012) A fractionation of the physiological burden of the personal protective equipment worn by firefighters. European Journal of Applied Physiology 112, 2913–2921.
| A fractionation of the physiological burden of the personal protective equipment worn by firefighters.Crossref | GoogleScholarGoogle Scholar | 22143844PubMed |