Can epigeic invertebrates escape fire?
Christopher Swinstead A * , Paul Nevill B and Philip W. Bateman
A
B
C
Handling Editor: Mike Calver
Abstract
Fire is both a driver of habitat heterogeneity and succession, as well as a destructive and detrimental force, according to the habitat affected and the fire conditions. The responses of many vertebrate taxa to fire have been broadly well-studied, with many taxa seeking shelter, burrowing or fleeing fire fronts to survive.
For invertebrates however, the potential for fleeing fire fronts has only been occasionally observed and has not been adequately quantified, despite invertebrate importance to ecosystem services.
We conducted a series of leaf litter microcosm burn offs during autumn and spring to quantify escape behaviour of epigeic invertebrate fauna compared to their natural abundances.
Controlled burns indicated that very few invertebrates of very few taxonomic groups escape fire. Those that did escape were predominantly made up of Araneae, Collembola, and Isopoda.
Our results indicate that epigeic invertebrates do not survive fire by active escape, implying that recolonisation must take place from outside the burn zone. We cannot, however, be sure that some taxa do not survive in situ through methods such as burrowing, seeking shelter within fire resistant refugia or by being within small unburnt remnant patches in the case of more mosaic pattern burns. Importantly this calls into question the survivability of short-range endemics; as if they cannot escape, then they are at risk of more localised extinctions with more intense burns.
Keywords: arthropod, control burn, fire, mosaic, pyrodiversity, wildfire.
Introduction
Fire in Australia, as in many other parts of the world, is a major driver of change in ecosystems, creating large-scale shifts in habitat, vegetation, and species assemblage and complexity. It does this through burning and removing vegetation in a mosaic, creating habitat heterogeneity, dependent on the fires scale and severity, and creating conditions for broader ecological change within the mosaic (Barclay et al. 2006; Thoresen et al. 2021). Notably, fire itself is only the catalyst for its ecological benefits, which are a result of post-fire recovery (McLauchlan et al. 2020). However, the impact of fires is variable and determined by the habitats and fauna present, burn type, fire intensity, speed, and scale (Friend 1993; Moretti et al. 2006; Pryke and Samways 2012).
Under the right conditions fire can promote ecological diversity and succession but under other conditions it can be detrimental (Driessen and Kirkpatrick 2017; Dickman 2021). Fire can promote habitat variability in multiple landscapes; this increase in habitat variability and subsequent associated species diversity – pyrodiversity – is influenced by the recolonisation pattern of the area and the immigration of fire-adapted species from the surrounding burn mosaic (Swan et al. 2020; Thoresen et al. 2021). It is important to note that not all species that take advantage of a post-fire habitat are advantageous to broad ecological recovery, for instance bark beetles (Ips spp., Scolytidae) increase in number after fire and can kill fire-damaged but still living native pines (Santoro et al. 2001). It is, however, important to highlight that all species recovery is dependent on either movement into or survival within the burnt patch (Jolly et al. 2022).
Fire prevalence and its associated effects vary across biomes and geographical regions. In recent decades, the incidence of fires has burgeoned due to anthropogenically mediated land use changes and environmental factors such as summer droughts, lightning strikes, high combustibility of vegetation, prolonged fire seasons, and a changing climate (Nimmo et al. 2021; Jones et al. 2022). Although all fires can cause species and habitat loss, the greatest risk comes from wildfires – unplanned and uncontrolled fires (Tedim and Leone 2020) of an extent and intensity anomalous to the affected habitat – which cause extensive wholesale damage of environments and loss of fauna, as well as endangering humans and their infrastructure, particularly in biomes not regarded as fire prone (Hrelja et al. 2020; Burke et al. 2021). Landholders and governments therefore employ fire reduction burns alongside other management actions, to reduce the risk of wildfire by reducing fuel load of the burnt area (Burrows and Friend 1998). Such burn-offs aim to make fires more manageable and reduce the risk of wildfires, while also being of lesser intensity, speed, and scale, to promote ecological recovery (Francos and Úbeda 2021; Pereira et al. 2021). Although controlled burn-offs are less destructive than wildfires, they can still potentially negatively affect the environment, although they can be important for the promotion of fire-dependent species and ecological succession (Chambers and Samways 1998; Bogusch et al. 2015).
Species recovery after fire is dependent on three key factors: in-situ survival, re-immigration of escaped taxa, and colonisation by taxa from the surrounding matrix. To survive fire, vertebrates utilise a range of tactics largely dependent on the animal’s mobility and speed (Friend 1993; Engstrom 2010; Robinson et al. 2013). These include fleeing and shelter-finding as exhibited by meadow voles (Microtus pennsylvanicus), and reed frogs (Hyperolius nitidulus) (Geluso and Bragg 1986; Grafe et al. 2002). Others, such as montane rattlesnakes (Crotalus willardi obscurus), may survive in situ within burrow or shelter (Smith et al. 2001). Some taxa may instead exhibit complex behavioural responses alongside fleeing; finding refuge such as tree climbing e.g. chimpanzees (Pan troglodytes) (Pruetz and LaDuke 2010) or doubling back into previously burnt habitat e.g. swamp wallabies (Wallabia bicolor) (Garvey et al. 2010). For invertebrates however, there is limited indication that they seek shelter (Uys et al. 2006; Brennan et al. 2011; De Omena et al. 2018) or can flee fire fronts, which could be possibly dependent on mobility (Swengel 2001). This may explain the results of limited previous invertebrate research, which showed predominantly edge-inwards recovery within burns (Zaitsev et al. 2014). Importantly, for short-range endemic invertebrates, only in-situ survival and returning individuals from very close by may be sufficient for population survival (e.g. Mason et al. 2019). Although this suggests that immigration is the primary driver of invertebrate post-fire recovery, it is unclear if this includes the re-migration of invertebrates that initially escaped from fire.
In Australia, the impact of increasing burn frequency, size and intensity, has been shown to be extensive, with over 30% of vertebrate fauna in the areas of the 2019/2020. Australian mega-fires negatively affected by habitat loss (Ward et al. 2020; Nimmo et al. 2021). It is, therefore, important to understand the survival rates and escape tactics of the otherwise broadly disregarded epigeic invertebrate fauna in the face of fires, particularly given their importance to soil health and ecosystem services (Bruyn 1997; Roy et al. 2018; Inkotte et al. 2022). To explore this, we investigated if leaf litter invertebrates typical of the jarrah–marri (Eucalyptus marginata – Corymbia callophyla) woodland ecosystem of Western Australia, showed escape behaviour when confronted with an advancing fire front under a controlled microcosm experiment setting. Response to fire by invertebrates is likely to be varied, dependent on adaptations of the diverse epigeic invertebrate taxa. However for those invertebrates that do not flee fire fronts, as some vertebrate taxa can, this would imply that invertebrate recovery is largely dependent on post-fire immigration and in-situ shelters. An understanding of this can inform how we help rehabilitate post-fire landscapes and carry out fire reduction burns.
Materials and methods
Invertebrate fire escape was investigated in autumn and spring of 2022 using two sets of experimental microcosm burn-offs, five during autumn and seven in spring. For each, 54 L leaf litter samples were collected from the broader Perth metropolitan area, from Baskerville Reserve, Mundijong (32°17′05″S; 115°58′01″E) in autumn, and Mundy Regional Park (31°51′16″S; 116°2′36″E) in spring 2022. Randomised leaf litter samples were collected from areas surrounding trees, i.e. not otherwise bare or from deep leaf litter piles directly under trees. These sites support habitats dominated by Banksia, and wandoo–jarrah–marri respectively.
Alongside each microcosm burn a control collection of leaf litter of equal volume was collected from the same site and run through a Tullgren funnel for 72 h to extract a representative invertebrate control sample. The microcosm burn-offs themselves used a directed fire into an adjacent elongated 1-m-wide pitfall trap, with bounding sheets along the sides, perpendicular to the pitfall traps (Fig. 1a). Bounding walls were used to prevent both invertebrate and fire escape from the microcosm, with a backing shield behind the pitfall to aid in the capture of fleeing invertebrates. All burns were initiated with an intense open flame from a weed burner along the far edge and burnt to the edge of the pitfall trap, leading to predominantly complete burns with moderate smoke, moving at low speeds (8–30 m per hour), with fire temperatures between 200 and 500°C. For the autumn burns, these bounding walls were 2 m long, for spring the length had to be reduced to 1 m due to higher invertebrate abundance. For all sampling, a volume of 52 L of leaf litter per m2, was used, creating a leaf litter depth of 5 cm. Pitfalls were open, with 1 cm of water during fires, which was collected and processed immediately after the fire. During both microcosm burn-offs, any observable invertebrate behaviour was recorded and, where possible, in-situ survivors were collected.
(a) Experimental microcosm setup for invertebrate fire escape, using a modified pitfall setup, with leaf litter to a depth of 5 cm (brown), bound by metal walls 10 cm in height (grey). The pit fall (depressed blue section) is on the opposite side to the burn ignition (flames), with two bounding walls to contain escaping invertebrates, (b) sampled wandoo-jarrah-marri, (c) Banksia habitat respectively.
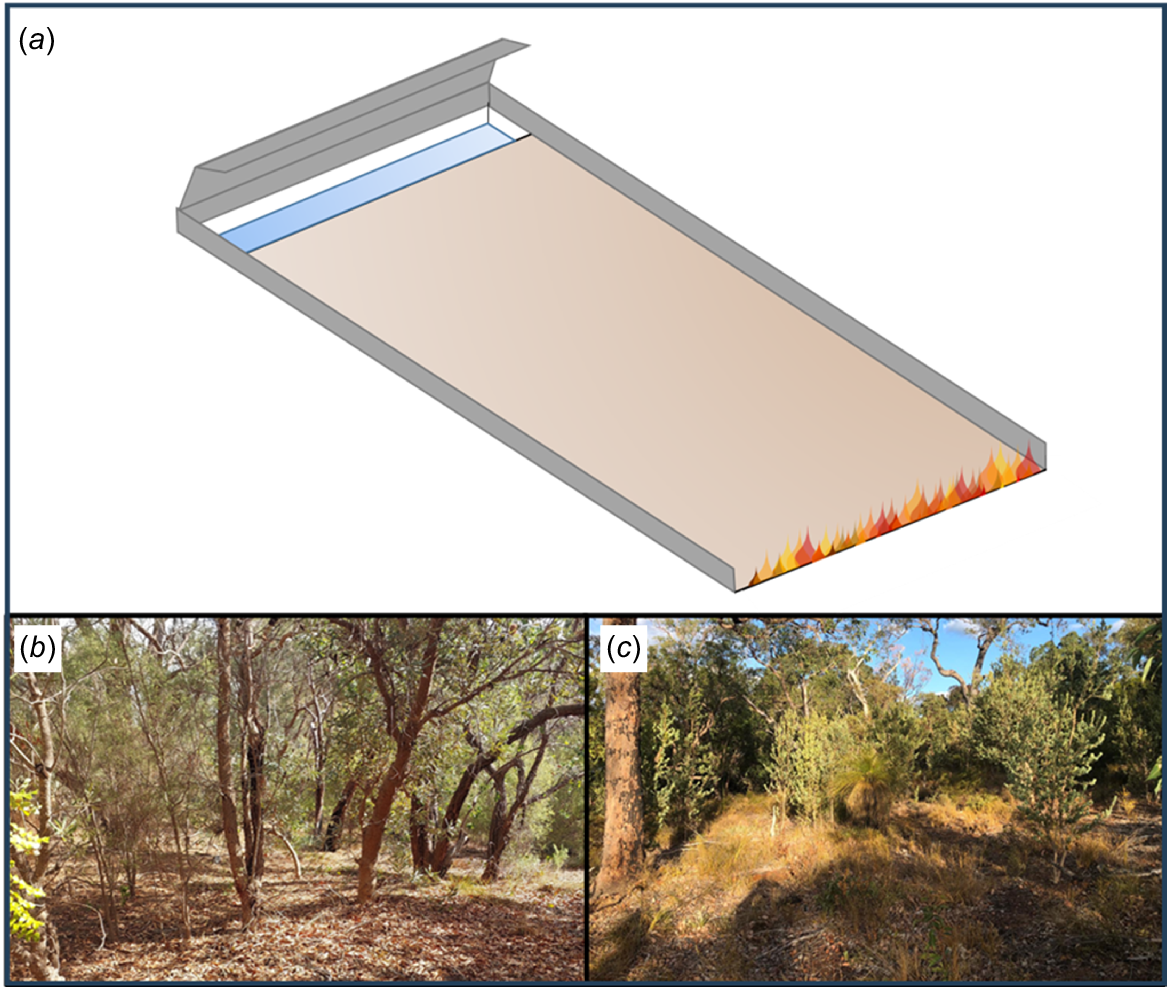
Invertebrate abundance was recorded from control samples, and from the post-fire pitfall samples, with all invertebrates collected initially identified to order and then to Recognisable Taxonomic Units (RTUs) (Oliver and Beattie 1993); larval and immature invertebrates that could not clearly be identified into morphospecies were excluded and tallied separately. This data was then run through a series of multivariate analyses in Primer (Clarke and Gorley 2015) to identify any significant differences in community. Before analysis, all invertebrate morphospecies with fewer than three records were excluded. The resulting data set was log transformed and a Bray–Curtis dissimilarity matrix was created. Initially all data was examined visually using a set of non-metric multidimensional scaling (n-MDS) ordinations. Then each data set was analysed with similarity percentage analysis (SIMPER) and then a permutational analysis of variance (PERMANOVA) to identify which taxa contributed to assemblage differences and to identify any significant differences.
Results
A total of 77 and 87 (0.9% and 1.98% of control abundance) individual invertebrates escaped the fire microcosms in autumn and in spring of 2022 compared to 7637 and 4257 identified from the controls for these periods respectively (8108 and 4384 including larvae), with no escape attempts by volant invertebrates observed. These escapes were predominantly by RTUs within Araneae, Collembola, and Isopoda with isolated escapes from Acarina, Blattodea, Coleoptera, Diptera, Formicidae and Hymenoptera. These escaping taxa represented 15% and 158% of total RTUs, and 25% and 304% of total orders identified from autumn and spring controls respectively.
A PERMANOVA of invertebrate RTU assemblage showed a significant difference between control and burnt samples (autumn; P = 0.0003, F = 14.317, spring; P = 0.003, F = 10.019). An initial SIMPER analysis indicated that control and burnt samples had an internal similarity of 7457 and 4450% for autumn, with a dissimilarity between these sample types of 9306%. Similarly for Spring, SIMPER showed that the control and burnt samples had internal similarities of 655 and 296%, with a dissimilarity of 9060%. Notably, the SIMPER indicated that Collembola predominantly contributed to this similarity within burnt samples, with a contribution from Isopoda only in spring. These differences between season similarities were then visualised by an n-MDS (Fig. 2). This highlighted the smaller dissimilarity between sample types in autumn burns (Fig. 2a) compared to spring burns (Fig. 2b), as well as the low internal similarity between burnt samples comparatively to control samples in both seasons.
Non-metric multidimensional scaling plot of invertebrate assemblages for fire escape for (a) autumn, with a stress of 0.01 and (b) spring of 2022, with a stress of 0.03. Both plots show a distinct separation of control (purple circles) and burnt (orange diamonds) samples, with a notable clustering in both seasons of control samples, and burnt samples in autumn, with a notable separation of burnt samples in spring.
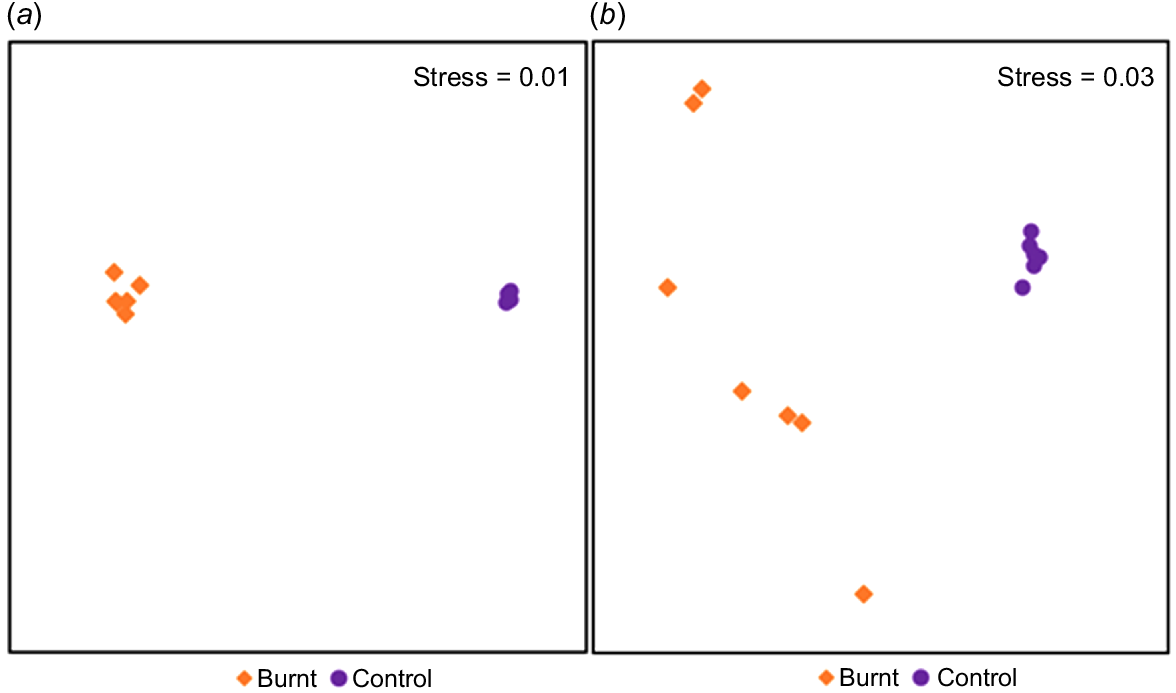
Discussion
We found very little evidence that epigeic invertebrate fauna show specific fire front escape behaviour, unlike that shown by vertebrates (Batista et al. 2023). On average, only 154% of all RTUs identified, and only 144% of the overall taxa abundance recorded in the control samples were observed to have moved away from approaching fire fronts. This indicates that fire escape is a behaviour that is not widely present in epigeic invertebrates. Invertebrates are rarely specifically considered when discussing fire escape – some of the functional traits that make animals more or less susceptible to fire listed by Batista et al. (2023) do, however, apply to our observations here: leaf litter dwelling fauna with limited mobility (i.e. non-volant), poor fire- or smoke-detection ability, and directionless escape are most susceptible to fire. Bonta et al. (2017) has previously described Black Kite (Milvus migrans), Whistling Kite (Haliastur sphenurus), and Brown Falcon (Falco berigora) foraging for fleeing invertebrates and small vertebrates at fire fronts – but only grasshoppers are specifically mentioned. Sticky traps set 2 m up trees in a frequently burned longleaf pine (Pinus palustris) forest caught mostly non-volant and juvenile invertebrates after fires, suggesting that climbing trees allowed these organisms and life stages to find refuge (Dell et al. 2017). When invertebrates are considered as part of fire management, it is usually by comparing assemblages at different times post-fire (e.g. Pryke and Samways 2012), i.e. not the immediate effect of the fire. Some taxa, however, such as mygalomorph spiders – short-range endemics with low dispersal ability post adulthood – are susceptible to local extinction after fires (Mason et al. 2019), and therefore would be missed by such sampling.
The majority of epigeic invertebrates that did get captured by pitfall probably constituted incidental migrations during the experimental burning of the substrate rather than deliberate, adaptive, movement away from the fire front. This probably explains our isolated recordings of Acarina, Blattodea, Coleoptera, Diptera, Formicidae and Hymenoptera, many of which were capable of flight, but instead walked into our pitfall trap. In the case of Blattodea, several specimens were observed during the experiment displaying clear movement activity, although this was notably directionless and did not result in a successful escape. For Araneae, Collembola and Isopoda, however, it is less clear, with a small set of RTUs within these orders escaping from all microcosm fire fronts, albeit in small numbers. This behaviour by Araneae is supported by previous observations by Brennan et al. (2011), who noted that other Araneae can show a similar fire escape behaviour. Similarly, it is possible that the records of Collembola could have also been due to an escape response to fire, through uncontrolled jumps. Notably these three orders are all highly mobile or exhibit apparently uncontrolled or random escape movement. However, despite the high RTU escape rates of 22%, 71% and 50% for Araneae, Collembola and Isopoda respectively, their actual escapes only represented a small fraction of their control abundance (27% for Araneae, 11% for Collembola and 31% for Isopoda). This high mortality appears to be in line with other research (Brennan et al. 2011; De Omena et al. 2018), but it is an indication of at least some escape potential by these taxa. However, the low escape rate compared to the abundance of these taxa in the control samples indicates that only a few individuals escape fire fronts.
Overall, the low fire escape potential of epigeic invertebrates is not completely unexpected, as past incidental observations from in-situ test of habitats have observed minimal escape attempts (Swengel 2001; Brennan et al. 2011). This implies that initial recolonisation and recovery of invertebrates post-fire must occur from remnant habitat and fire refuges within the burnt area along with invertebrate immigration from nearby unburnt patches (Swengel 2001). In the case of in-situ fire survival invertebrates have the potential to survive dependent on the fire conditions, in locations such as under bark or moving towards the canopy, in grass trees or other fire-resistant vegetation, or in unburnt leaf litter (either by depth or within an mosaic or patch) (Brennan et al. 2011; Croft et al. 2012; Gongalsky et al. 2012; Robinson et al. 2013; Dell et al. 2017). However, it should be noted that in-situ survival is heavily dependent on the fire’s temperature, extent, depth and speed, with reduced in-situ survival and refuge diversity with increased fire severity (Badejo 1994; Wikars and Schimmel 2001; Brand 2002). This is of particular importance for short-range endemics, which can easily have their entire range burned during uncontrolled burns and wildfires (Mason et al. 2019; Henry et al. 2022).
Additionally, it is important to consider that some taxa may not be readily capable of surviving or migrating to post-fire areas, due to factors such as limited dispersal or dispersal timing, specific reproductive conditions, or have habitat requirements that are not conducive to migrating to or surviving in a post-fire area (Swengel 2001). Post-fire invertebrate immigration on the other hand is largely dependent on the conditions of the post-fire habitat itself, the fire’s size, and the mobility of taxa in question (Zaitsev et al. 2014). Additionally, some taxa do actively migrate to burn patches, to take advantage of the post-fire conditions (Badejo 1994; Brand 2002). However, hotter, less patchy burns and complete burns are more likely to be dependent on post-fire conditions and cross-sectional distance across the burn for invertebrate recovery and immigration to successfully occur, than in more controlled burns. Overall, post-fire recovery of invertebrates appears to mirror that of general post-fire recovery itself, where it is largely dependent on the burn heterogeneity, fire frequency, post-fire resources and conditions, as well as the habitat and its condition (Bradstock et al. 2005; Langlands et al. 2006; Pryke and Samways 2012).
In conclusion, as few epigeic invertebrates can escape from leaf litter fires, the overall survival of invertebrates in burnt regions is likely to be more dependent on refuges and recolonisation from the unburnt mosaic: the response of fauna to fire, whatever it is, helps us understand the biodiversity in fire-prone landscapes (Pausas and Parr 2018). Of concern, however, is that more extreme and extensive burns – wildfires – that are becoming more frequent mean that invertebrate survival in situ will not be possible and recolonisation across a larger burnt, non-mosaic, area will become more difficult and/or take much longer.
Declaration of funding
This study received funding from the Australian Government Research Training Program (RTP) Scholarship.
References
Badejo MA (1994) Effect of accidental fire on soil mite density in a forest reserve in Nigeria. Experimental & Applied Acarology 18, 703-710.
| Crossref | Google Scholar |
Barclay HJ, Chao L, Hawkes B, Benson L (2006) Effects of fire size and frequency and habitat heterogeneity on forest age distribution. Ecological Modelling 197(1–2), 207-220.
| Crossref | Google Scholar |
Batista EKL, Figueira JEC, Solar RRC, de Azevedo CS, Beirão MV, Berlinck CN, Brandão RA, de Castro FS, Costa HC, Costa LM, Feitosa RM, Freitas AVL, Freitas GHS, Galdino CAB, Júnior JES, Leite FS, Lopes L, Ludwig S, do Nascimento MC, Negreiros D, Oki Y, Paprocki H, Perillo LN, Perini FA, Resende FM, Rosa AHB, Salvador LF, Jr, Silva LM, Silveira LF, DeSouza O, Vieira EM, Fernandes GW (2023) In case of fire, escape or die: a trait-based approach for identifying animal species threatened by fire. Fire 6(6), 242.
| Crossref | Google Scholar |
Bogusch P, Blažej L, Trýzna M, Heneberg P (2015) Forgotten role of fires in Central European forests: critical importance of early post-fire successional stages for bees and wasps (Hymenoptera: Aculeata). European Journal of Forest Research 134, 153-166.
| Crossref | Google Scholar |
Bonta M, Gosford R, Eussen D, Ferguson N, Loveless E, Witwer M (2017) Intentional fire-spreading by “firehawk” raptors in Northern Australia. Journal of Ethnobiology 37(4), 700-718.
| Crossref | Google Scholar |
Bradstock RA, Bedward M, Gill AM, Cohn JS (2005) Which mosaic? A landscape ecological approach for evaluating interactions between fire regimes, habitat and animals. Wildlife Research 32(5), 409-423.
| Crossref | Google Scholar |
Brand RH (2002) The effect of prescribed burning on epigeic springtails (Insecta: Collembola) of woodland litter. The American Midland Naturalist 148(2), 383-393.
| Crossref | Google Scholar |
Brennan KEC, Moir ML, Wittkuhn RS (2011) Fire refugia: the mechanism governing animal survivorship within a highly flammable plant. Austral Ecology 36(2), 131-141.
| Crossref | Google Scholar |
Bruyn LALd (1997) The status of soil macrofauna as indicators of soil health to monitor the sustainability of Australian agricultural soils. Ecological Economics 23(2), 167-178.
| Crossref | Google Scholar |
Burke M, Driscoll A, Heft-Neal S, Xue J, Burney J, Wara M (2021) The changing risk and burden of wildfire in the United States. Proceedings of the National Academy of Sciences of the United States of America 118(2), e2011048118.
| Crossref | Google Scholar |
Burrows ND, Friend G (1998) Biological indicators of appropriate fire regimes in southwest Australian ecosystems. In ‘Fire in ecosystem management: shifting the paradigm from suppression to prescription.’ Proceedings of the 20th Tall Timbers Fire Ecology Conference. Tall Timbers Research, Inc, Tallahassee. Available at https://talltimbers.org/wp-content/uploads/2018/09/413-BurrowsandFriend1998_op.pdf
Chambers BQ, Samways MJ (1998) Grasshopper response to a 40-year experimental burning and mowing regime, with recommendations for invertebrate conservation management. Biodiversity and Conservation 7, 985-1012.
| Crossref | Google Scholar |
Croft P, Reid N, Hunter JT (2012) The bark of eucalypt trees: habitat quality for arthropods and impact of fire. Pacific Conservation Biology 18(3), 186-193.
| Crossref | Google Scholar |
De Omena PM, Kersch-Becker MF, Antiqueira PAP, Bernabé TN, Benavides-Gordillo S, Recalde FC, Vieira C, Migliorini GH, Romero GQ (2018) Bromeliads provide shelter against fire to mutualistic spiders in a fire-prone landscape. Ecological Entomology 43(3), 389-393.
| Crossref | Google Scholar |
Dell J, O’Brien J, Doan L, Richards L, Dyer L (2017) An arthropod survival strategy in a frequently burned forest. Ecology 98(11), 2972-2974.
| Crossref | Google Scholar | PubMed |
Dickman CR (2021) Ecological consequences of Australia’s “black summer” bushfires: managing for recovery. Integrated Environmental Assessment and Management 17(6), 1162-1167.
| Crossref | Google Scholar | PubMed |
Driessen MM, Kirkpatrick JB (2017) The implications of succession after fire for the conservation management of moorland invertebrate assemblages. Journal of Insect Conservation 21, 15-37.
| Crossref | Google Scholar |
Engstrom RT (2010) First-order fire effects on animals: review and recommendations. Fire Ecology 6, 115-130.
| Crossref | Google Scholar |
Francos M, Úbeda X (2021) Prescribed fire management. Current Opinion in Environmental Science & Health 21, 100250.
| Crossref | Google Scholar |
Friend GR (1993) Impact of fire on small vertebrates in mallee woodlands and heathlands of temperate Australia: a review. Biological Conservation 65(2), 99-114.
| Crossref | Google Scholar |
Garvey N, Ben-Ami D, Ramp D, Croft DB (2010) Survival behaviour of swamp wallabies during prescribed burning and wildfire. Wildlife Research 37(1), 1-12.
| Crossref | Google Scholar |
Geluso KN, Bragg TB (1986) Fire-avoidance behavior of meadow voles (Microtus pennsylvanicus). The American Midland Naturalist 116(1), 202-205.
| Crossref | Google Scholar |
Gongalsky KB, Malmström A, Zaitsev AS, Shakhab SV, Bengtsson J, Persson T (2012) Do burned areas recover from inside? An experiment with soil fauna in a heterogeneous landscape. Applied Soil Ecology 59, 73-86.
| Crossref | Google Scholar |
Grafe TU, Döbler S, Linsenmair KE (2002) Frogs flee from the sound of fire. Proceedings of the Royal Society B: Biological Sciences 269, 999-1003.
| Crossref | Google Scholar |
Henry SC, Kirkpatrick JB, McQuillan PB (2022) The half century impact of fire on invertebrates in fire-sensitive vegetation. Austral Ecology 47(3), 590-602.
| Crossref | Google Scholar |
Hrelja I, Šestak I, Bogunović I (2020) Wildfire impacts on soil physical and chemical properties - a short review of recent studies. Agriculturae Conspectus Scientificus 85(4), 293-301.
| Google Scholar |
Inkotte J, Bomfim B, da Silva SC, Valadão MBX, da Rosa MG, Viana RB, D’Ângelo Rios P, Gatto A, Pereira RS (2022) Linking soil biodiversity and ecosystem function in a Neotropical savanna. Applied Soil Ecology 169, 104209.
| Crossref | Google Scholar |
Jolly CJ, Dickman CR, Doherty TS, Van Eeden LM, Geary WL, Legge SM, Woinarski JCZ, Nimmo DG (2022) Animal mortality during fire. Global Change Biology 28(6), 2053-2065.
| Crossref | Google Scholar | PubMed |
Jones MW, Abatzoglou JT, Veraverbeke S, Andela N, Lasslop G, Forkel M, Smith AJP, Burton C, Betts RA, van der Werf GR, Sitch S, Canadell JG, Santín C, Kolden C, Doerr SH, Quéré CL (2022) Global and regional trends and drivers of fire under climate change. Reviews of Geophysics 60(3), e2020RG000726.
| Crossref | Google Scholar |
Langlands PR, Brennan KEC, Pearson DJ (2006) Spiders, spinifex, rainfall and fire: long-term changes in an arid spider assemblage. Journal of Arid Environments 67(1), 36-59.
| Crossref | Google Scholar |
Mason L, Bateman PW, Miller BP, Wardell-Johnson GW (2019) Ashes to ashes: intense fires extinguish populations of urban short-range endemics. Austral Ecology 44(3), 514-522.
| Crossref | Google Scholar |
McLauchlan KK, Higuera PE, Miesel J, Rogers BM, Schweitzer J, Shuman JK, Tepley AJ, Varner JM, Veblen TT, Adalsteinsson SA, Balch JK, Baker P, Batllori E, Bigio E, Brando P, Cattau M, Chipman ML, Coen J, Crandall R, Crandall R, Daniels L, Enright N, Gross WS, Harvey BJ, Hatten JA, Hermann S, Hewitt RE, Kobziar LN, Landesmann JB, Loranty MM, Maezumi SY, Mearns L, Moritz M, Myers JA, Pausas JG, Pellegrini AFA, Platt WJ, Roozeboom J, Safford H, Santos F, Scheller RM, Sherriff RL, Smith KG, Smith MD, Watts AC (2020) Fire as a fundamental ecological process: research advances and frontiers. Journal of Ecology 108(5), 2047-2069.
| Crossref | Google Scholar |
Moretti M, Duelli P, Obrist MK (2006) Biodiversity and resilience of arthropod communities after fire disturbance in temperate forests. Oecologia 149, 312-327.
| Crossref | Google Scholar | PubMed |
Nimmo DG, Carthey AJR, Jolly CJ, Blumstein DT (2021) Welcome to the Pyrocene: animal survival in the age of megafire. Global Change Biology 27(22), 5684-5693.
| Crossref | Google Scholar | PubMed |
Oliver I, Beattie AJ (1993) A possible method for the rapid assessment of biodiversity. Conservation Biology 7(3), 562-568.
| Crossref | Google Scholar |
Pausas JG, Parr CL (2018) Towards an understanding of the evolutionary role of fire in animals. Evolutionary Ecology 32(2), 113-125.
| Crossref | Google Scholar |
Pereira P, Bogunovic I, Zhao W, Barcelo D (2021) Short-term effect of wildfires and prescribed fires on ecosystem services. Current Opinion in Environmental Science & Health 22, 100266.
| Crossref | Google Scholar |
Pruetz JD, LaDuke TC (2010) Brief communication: reaction to fire by savanna chimpanzees (Pan troglodytes verus) at Fongoli, Senegal: conceptualization of “fire behavior” and the case for a chimpanzee model. American Journal of Physical Anthropology 141(4), 646-650.
| Crossref | Google Scholar | PubMed |
Pryke JS, Samways MJ (2012) Differential resilience of invertebrates to fire. Austral Ecology 37(4), 460-469.
| Crossref | Google Scholar |
Robinson NM, Leonard SWJ, Ritchie EG, Bassett M, Chia EK, Buckingham S, Gibb H, Bennett AF, Clarke MF (2013) REVIEW: refuges for fauna in fire-prone landscapes: their ecological function and importance. Journal of Applied Ecology 50(6), 1321-1329.
| Crossref | Google Scholar |
Roy S, Roy MM, Jaiswal AK, Baitha A (2018) Soil arthropods in maintaining soil health: thrust areas for sugarcane production systems. Sugar Tech 20, 376-391.
| Crossref | Google Scholar |
Santoro AE, Lombardero MJ, Ayres MP, Ruel JJ (2001) Interactions between fire and bark beetles in an old growth pine forest. Forest Ecology and Management 144(1–3), 245-254.
| Crossref | Google Scholar |
Smith LJ, Holycross AT, Painter CW, Douglas ME (2001) Montane rattlesnakes and prescribed fire. The Southwestern Naturalist 46(1), 54-61.
| Crossref | Google Scholar |
Swan M, Christie F, Steel E, Sitters H, York A, Di Stefano J (2020) Ground-dwelling mammal diversity responds positively to productivity and habitat heterogeneity in a fire-prone region. Ecosphere 11(9), e03248.
| Crossref | Google Scholar |
Swengel AB (2001) A literature review of insect responses to fire, compared to other conservation managements of open habitat. Biodiversity and Conservation 10, 1141-1169.
| Crossref | Google Scholar |
Tedim F, Leone V (2020) The dilemma of wildfire definition: what it reveals and what it implies. Frontiers in Forests and Global Change 3, 553116.
| Crossref | Google Scholar |
Thoresen J, Vermeire M-L, Venter Z, Wolfaard G, Krumins JA, Cramer M, Hawkins H-J (2021) Fire and herbivory shape soil arthropod communities through habitat heterogeneity and nutrient cycling in savannas. Global Ecology and Conservation 25, e01413.
| Crossref | Google Scholar |
Uys C, Hamer M, Slotow R (2006) Effect of burn area on invertebrate recolonization in grasslands in the Drakensberg, South Africa. African Zoology 41(1), 51-65.
| Crossref | Google Scholar |
Ward M, Tulloch AIT, Radford JQ, Williams BA, Reside AE, Macdonald SL, Mayfield HJ, Maron M, Possingham HP, Vine SJ, O’Connor JL, Massingham EJ, Greenville AC, Woinarski JCZ, Garnett ST, Lintermans M, Scheele BC, Carwardine J, Nimmo DG, Lindenmayer DB, Kooyman RM, Simmonds JS, Sonter LJ, Watson JEM (2020) Impact of 2019–2020 mega-fires on Australian fauna habitat. Nature Ecology & Evolution 4, 1321-1326.
| Crossref | Google Scholar | PubMed |
Wikars L-O, Schimmel J (2001) Immediate effects of fire-severity on soil invertebrates in cut and uncut pine forests. Forest Ecology and Management 141(3), 189-200.
| Crossref | Google Scholar |
Zaitsev A, Gongalsky KB, Persson T, Bengtsson J (2014) Connectivity of litter islands remaining after a fire and unburnt forest determines the recovery of soil fauna. Applied Soil Ecology 83, 101-108.
| Crossref | Google Scholar |