Individualised treatment of sexually transmitted infections by rapid molecular assays
Gerald L. Murray A B C * , Emma L. Sweeney D and Catriona S. Bradshaw E F GA
B
C
D
E
F
G
![]() Gerald Murray is a senior research officer in the department of obstetrics, gynaecology and newborn health at The University of Melbourne, based at the Royal Women’s Hospital. His research interests include investigating mechanisms of antimicrobial resistance, studying the human microbiota in health and disease, and human papillomavirus (HPV). |
![]() Emma Sweeney is a senior research fellow at The University of Queensland Centre for Clinical Research. Her research interests include the development of novel molecular tools to detect and characterise pathogens of global health importance, with a particular focus on the sexually transmitted infection (STI) superbug Mycoplasma genitalium. |
![]() Catriona Bradshaw is a clinician researcher at Melbourne Sexual Health Centre, Monash University and Alfred Hospital. Her research interests include translational research to improve the treatment and control of drug resistant and refractory sexually transmitted infections. |
Abstract
Antimicrobial resistance (AMR) is a major problem in the field of sexually transmitted infections (STIs), where escalating levels have coincided with a surge in infections. The individualised treatment of STIs by rapid molecular ‘resistance’ assays is a new approach to patient management that has developed through understanding of the mechanisms of resistance, combined with nucleic acid amplification diagnostics. Mycoplasma genitalium provides an excellent example of this approach because of a restricted repertoire of effective antibiotics, and resistance mediated by single nucleotide polymorphisms. Detection of one of five M. genitalium 23S rRNA gene mutations is highly predictive of treatment failure with macrolides; employing this diagnostically yields >95% cure with azithromycin. Detection of fluoroquinolone resistance mutations in parC (especially affecting serine 83) and gyrA is predicted to have a similar diagnostic value and is currently being evaluated. This work is underpinned by high quality resistance data, emphasising the importance of ongoing surveillance. Despite some limitations, individualised treatment has demonstrated value in antimicrobial stewardship, extending the use of current therapies despite substantial levels of resistance, reducing prescription of ineffective treatments and sparing last-resort antibiotics.
Keywords: antibiotic resistance, diagnostic tests, Mycoplasma genitalium, PCR, sexually transmitted infections, STIs.
Introduction
Antimicrobial resistance (AMR) remains one of the greatest threats to public health and is a particular problem for sexually transmitted infections (STIs). WHO guidance for Neisseria gonorrhoeae indicates a 95% effectiveness and 5% resistance threshold at which to recommend the next antibiotic option.1 However, it is well documented that the antibiotic discovery pipeline is limited; many pathogens have exceeded the above threshold with alternative treatment options either unavailable or reserved for last-resort use. This situation demands new approaches to antimicrobial stewardship and leads to the question – can an alternative approach to therapy preserve the effectiveness of existing treatments in the face of high levels of resistance?
The challenges for treatment for Mycoplasma genitalium are particularly notable. This bacterium has seen a recent upsurge in the levels of AMR, with a focal point in Australia and the Western Pacific region,2,3 and the peculiarities of this organism make it particularly difficult to work with. Mycoplasma genitalium is, however, a good exemplar of the application of resistance-guided therapy.
Mycoplasma genitalium – an unusual pathogen
Mycoplasma genitalium is a common sexually transmitted bacterium that causes urethritis in men, and is associated with cervicitis, pelvic inflammatory disease and pregnancy complications in women.4–6 There has been a gradual evolutionary reduction of the M. genitalium genome to the point where it only contains ~500 genes (compared to ~4400 genes for Escherichia coli).7 This has made the organism highly fastidious and dependent on the host, meaning it is very slow to culture in vitro, often with low success rates. Consequently, routine culture-based antibiotic susceptibility testing is not feasible8,9 and the only practical diagnostic solution to AMR testing is with nucleic acid amplification tests (NAATs).
Decoding the complex picture of resistance
A major clinical challenge for M. genitalium is the limited number of treatment options. Many biosynthetic pathways have been lost including antibiotic targets such as peptidoglycan cell-wall synthesis, narrowing the scope of antibiotic susceptibility to a few classes that either inhibit protein synthesis or DNA replication, including macrolides, fluoroquinolones, tetracyclines and streptogramins (Fig. 1).10 Overlaid are the issues of potential significant side effects or limitations in use for a number of these classes (e.g. for fluoroquinolones), contraindications for use in pregnancy (tetracyclines, fluoroquinolones) and escalating resistance.
Summary of current antibiotic treatments for M. genitalium, targets and mechanisms of resistance. Resistance levels are indicated for recent analysis in Australia, but vary by population and risk profile. SNP, single nucleotide polymorphism. Estimates of treatment effectiveness are based on studies using pre-treatment with doxycycline (consistent with Australian treatment guidelines). WT, wild-type.
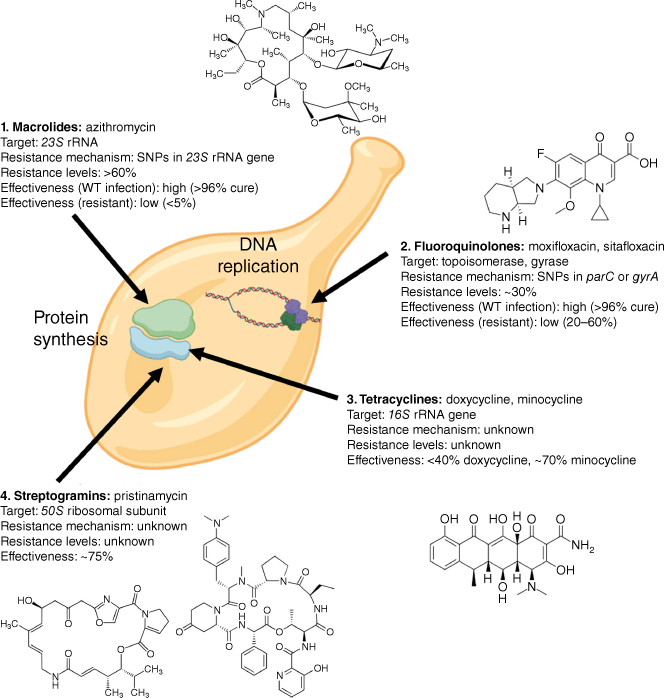
The basis for M. genitalium antibiotic resistance has mainly been pieced together through sequence analysis of clinical samples from patients that have failed or been cured by treatments, and most of these mechanisms have subsequently been confirmed by limited in vitro testing.8,11,12 AMR is typically conveyed by single nucleotide polymorphisms (SNPs) that appear to arise spontaneously with concerning regularity (Fig. 1).
For macrolides (azithromycin), a very high level of resistance is conveyed by one of five changes in the 23S rRNA gene.13 In vitro comparison of sensitive and resistant bacteria illustrates a minimum inhibitory concentration (MIC) difference of ~10,000 fold.14 Resistance to macrolides has developed around the world and is found in >60% of infections in Australia.2
For fluoroquinolones (moxifloxacin and sitafloxacin), our understanding of resistance has recently improved. AMR is primarily the result of SNPs in the DNA topoisomerase gene, parC, affecting a serine at amino acid position 83 or the aspartic acid at position 87. The most potent change is parC G248T (S83I) conferring 40–50% risk of failure of moxifloxacin,3 and a modest change in the in vitro MIC of ~100 fold.14 Although the local prevalence of this mutation varies, a recent meta-analysis shows a global rise from 0% prior to 2012 to 7.6% by 2021.15 The impact of the S83I change in parC is potentiated by subsequent changes in gyrA (affecting methionine 95 or aspartic acid 99),16,17 and, although still uncommon, when these mutations are combined the risk of moxifloxacin failure doubles to >80%.
Within the tetracycline class, doxycycline is less effective than minocycline (<40 v. 70%).18 Doxycycline is often prescribed as syndromic treatment prior to diagnosis, and can improve the success of subsequent treatment.19 Mechanisms of resistance remain unclear, with a recent study finding no obvious resistance-mediating SNPs in the 16S rRNA gene.20
A template for resistance-guided therapy
Our increasing understanding of AMR mechanisms coupled with NAAT diagnostics has opened the pathway to tailor treatment to the resistance profile of bacteria for each patient (summarised in Fig. 2). Initial studies targeting macrolide resistance used a two-step approach: (i) syndromic treatment with doxycycline while awaiting a diagnostic test result, and (ii) resistance-guided treatment for M. genitalium-positive patients based on detection or absence of five 23S rRNA gene mutations. The initial study found that in a population with a background of 68% macrolide resistance, treatment of macrolide-susceptible infections with azithromycin led to 95% cure, with very low selection of resistance (2.5%).19 The remaining macrolide-resistant infections were triaged to moxifloxacin with 92% cure (in the context of 13% prevalence of parC S83I resistance). This illustrates that effective use of azithromycin, a safer and more-convenient treatment option, is possible even in a background of substantial resistance. This approach has been adopted into Australian, North American, European and British guidelines.
A simplified overview of a resistance guided therapy approach to M. genitalium. A patient presenting with a syndrome or suspicion of infection undergoes syndromic treatment (doxycycline for 7 days) and simultaneous testing for M. genitalium, macrolide resistance (23S rRNA mutations), the primary marker of fluoroquinolone resistance (parC mutation affecting serine 83, especially SNP G248T conferring S83I), and the secondary makers of fluoroquinolone resistance (gyrA mutations affecting methionine 95 or aspartic acid 99). Depending on the diagnostic outcome, a treatment can be prescribed to optimise chance of cure (indicated by percentages in yellow) stratified by four tiers of risk. Notably, resistance-guided therapy for macrolides is currently recommended by guidelines in Australia and elsewhere, while resistance-guided therapy for fluoroquinolones is presented as a conceptual guide. Additionally, there are currently shortages of pristinamycin. FQ, fluoroquinolone; WT, wild-type. This figure has been adapted from Murray et al. (2023).16
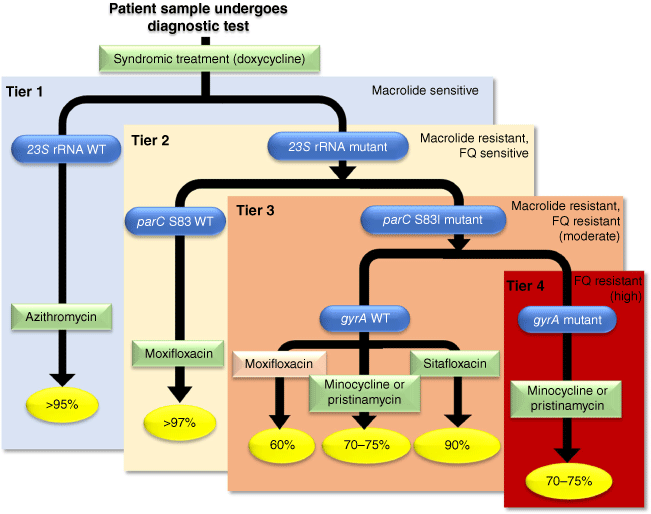
In settings with significant or rising fluoroquinolone resistance, incorporating fluoroquinolone-resistance targets into molecular assays can further improve antibiotic selection. As mentioned above, the major determinant of fluoroquinolone failure is parC S83I mutation. Detection of parC wild-type (WT) or S83I alone prior to treatment could lead to >97% chance of treatment success for patients without parC S83I mutation.21 Testing for gyrA changes could further stratify those with S83I-positivity into moderate or high risk of failure with a fluoroquinolone (Fig. 2).
The approach of combining all of the above resistance or susceptibility targets (macrolide, parC and gyrA) into a sequenced resistance-guided strategy could theoretically achieve ≥95% first-line cure for a high proportion of patients using available antibiotics (Tiers 1 and 2, see Fig. 2).21 Tier 3 patients (parC S83I, gyrA WT) could have a cure of 60–90%, depending on antibiotic choice and availability. The remaining patients with both parC and gyrA resistance mutations could be directed to available alternatives with first line cure for three out of four patients (Tier 4, Fig. 2). A summary of a proposed treatment pathway is shown in Fig. 2 and is currently being evaluated prospectively.
Resistance-guided therapy for other STIs
Important advances have also been made in resistance-guided therapy for N. gonorrhoeae. Recent data indicate 63% of N. gonorrhoeae isolates in Australia are ciprofloxacin resistant, with many countries reporting >90% resistance.22,23 Commercial tests are now available for detection of ciprofloxacin resistance, targeting the principal resistance mutation (serine to phenylalanine mutation at amino acid position 91 of the GyrA protein), as well as WT gyrA sequence. Mutation detection is highly predictive of resistance to ciprofloxacin (~99%), whereas detection of WT S91 is strongly associated with susceptibility to ciprofloxacin (≥99%).24 This provides a possible means to extend the use of oral antimicrobials for gonorrhoea, although a recent surge in ciprofloxacin resistance may limit the long-term utility of this approach.22 Nevertheless, a recent proof-of-principle study demonstrated the utility of expanded resistance-guided treatment approaches for sparing use of ceftriaxone in gonorrhoea, but stressed the importance that assay use is informed by the non-random nature of co-resistances.25
Considerations and potential downsides to this approach
Although resistance-guided therapy is showing great promise, there are potential downsides:
Tailored treatment is predicated on high quality evidence of AMR mechanisms, but that information is not always available, e.g. for tetracycline resistance in M. genitalium.
It is important to be wary of studies reporting mutations as ‘mediating-resistance’ without sufficient phenotypic and clinical evidence.
Resistance mechanisms may change or evolve over time, e.g. through the horizontal gene transfer. Diagnostic tests must keep up with such changes.
Diagnostic-assay design can be complicated by the presence of multiple different mechanisms of resistance to the same antibiotic and evolving resistance mutations. This requires more targets to be integrated into an assay, e.g. the suite of mutations in parC and gyrA that contribute to fluroquinolone resistance in M. genitalium. Use of diagnostic direct sequencing (metagenomics) may help resolve these issues in the future.
Although yet to be properly documented for M. genitalium, heteroresistance (the presence of subpopulations of resistant bacteria in a predominantly WT background) may lead to a false-negative test for resistance, leading to inappropriate treatment and treatment failure.
This approach is cost effective for M. genitalium in a high-income country26; however, cost effectiveness should be further determined for different populations and settings
Summary, and future perspectives
In summary, resistance-guided therapy can be highly effective, facilitating continued use of preferred treatments in the face of high levels of resistance, while reducing the futile prescription of antibiotics when there is little chance of treatment success. This approach can spare reserved or specific-purpose antibiotics such as pristinamycin (the only safe option for macrolide-resistant M. genitalium in pregnancy).
Future research is clearly needed to support this approach more broadly in the field of STIs, including discovery of mechanisms of resistance for other antibiotics. The transfer of tests across to rapid-diagnostic platforms for beside use will accelerate the timely delivery of treatments and reduced loss to follow up. Finally, surveillance remains essential to monitor the AMR landscape, both to identify new issues as they arise, and to assess the impact of interventions.
Data availability
Data sharing is not applicable as no new data were generated or analysed during this study.
Conflicts of interest
G. L. Murray declares having previously receiving diagnostic kits from SpeeDx Pty Ltd and TIB-MOLBIOL, unrelated to this work. E. L. Sweeney reports previous research funding from SpeeDx Pty Ltd, including a previous Researcher Exchange and Development with Industry (REDI) fellowship working directly with SpeeDx. C. S. Bradshaw previously receiving diagnostic kits from SpeeDx Pty Ltd and Cepheid Pty Ltd unrelated to this work. SpeeDx played no role in the conception or preparation of this manuscript. The authors have no further conflicts of interest to declare.
Declaration of funding
Gerald L. Murray and Catriona S. Bradshaw received funding for this work from the Australian Research Council Industrial Transformation Research Hub for Antimicrobial Resistance (IH190100021).
References
1 Wi T et al. (2017) Antimicrobial resistance in Neisseria gonorrhoeae: global surveillance and a call for international collaborative action. PLoS Med 14, e1002344.
| Crossref | Google Scholar | PubMed |
2 Machalek DA et al. (2020) Prevalence of mutations associated with resistance to macrolides and fluoroquinolones in Mycoplasma genitalium: a systematic review and meta-analysis. Lancet Infect Dis 20, 1302-1314.
| Crossref | Google Scholar | PubMed |
3 Murray GL et al. (2022) parC variants in Mycoplasma genitalium: trends over time and association with moxifloxacin failure. Antimicrob Agents Chemother 66, e0027822.
| Crossref | Google Scholar | PubMed |
4 Horner PJ, Martin DH (2017) Mycoplasma genitalium infection in men. J Infect Dis 216, S396-S405.
| Crossref | Google Scholar | PubMed |
5 Lis R et al. (2015) Mycoplasma genitalium infection and female reproductive tract disease: a meta-analysis. Clin Infect Dis 61, 418-426.
| Crossref | Google Scholar | PubMed |
6 Htaik K et al. (2024) Systematic review and meta analysis of the association between Mycoplasma genitalium and pelvic inflammatory disease. Clin Infect Dis: ciae295.
| Crossref | Google Scholar | PubMed |
7 Fookes MC et al. (2017) Mycoplasma genitalium: whole genome sequence analysis, recombination and population structure. BMC Genomics 18, 993.
| Crossref | Google Scholar | PubMed |
8 Hamasuna R et al. (2005) Antibiotic susceptibility testing of Mycoplasma genitalium by TaqMan 5’ nuclease real-time PCR. Antimicrob Agents Chemother 49, 4993-4998.
| Crossref | Google Scholar | PubMed |
9 Pitt R et al. (2022) Challenges of in vitro propagation and antimicrobial susceptibility testing of Mycoplasma genitalium. J Antimicrob Chemother 77, 2901-2907.
| Crossref | Google Scholar | PubMed |
10 Bradshaw CS et al. (2017) New horizons in Mycoplasma genitalium treatment. J Infect Dis 216, S412-S419.
| Crossref | Google Scholar | PubMed |
11 Hamasuna R et al. (2009) Antimicrobial susceptibilities of Mycoplasma genitalium strains examined by broth dilution and quantitative PCR. Antimicrob Agents Chemother 53, 4938-4939.
| Crossref | Google Scholar | PubMed |
12 Hamasuna R et al. (2018) Mutations in ParC and GyrA of moxifloxacin-resistant and susceptible Mycoplasma genitalium strains. PLoS ONE 13, e0198355.
| Crossref | Google Scholar | PubMed |
13 Jensen JS et al. (2008) Azithromycin treatment failure in Mycoplasma genitalium-positive patients with nongonococcal urethritis is associated with induced macrolide resistance. Clin Infect Dis 47, 1546-1553.
| Crossref | Google Scholar | PubMed |
14 Hamasuna R et al. (2022) Analysis of fluoroquinolone-resistance using MIC determination and homology modelling of ParC of contemporary Mycoplasma genitalium strains. J Infect Chemother 28, 377-383.
| Crossref | Google Scholar | PubMed |
15 Chua T-P et al. (2024) Evolving patterns of macrolide and fluoroquinolone resistance in Mycoplasma genitalium: an updated global systematic review and metaanalysis. SSRN 2024, 4764790 [Preprint, posted 21 March 2024] .
| Crossref | Google Scholar |
16 Murray GL et al. (2023) gyrA mutations in Mycoplasma genitalium and their contribution to moxifloxacin failure: time for the next generation of resistance-guided therapy. Clin Infect Dis 76, 2187-2195.
| Crossref | Google Scholar | PubMed |
17 Ando N et al. (2023) Effectiveness of sitafloxacin monotherapy for quinolone-resistant rectal and urogenital Mycoplasma genitalium infections: a prospective cohort study. J Antimicrob Chemother 78, 2070-2079.
| Crossref | Google Scholar | PubMed |
18 Clarke EJ et al. (2023) Efficacy of minocycline for the treatment of Mycoplasma genitalium. Open Forum Infect Dis 10, ofad427.
| Crossref | Google Scholar | PubMed |
19 Read TRH et al. (2019) Outcomes of resistance-guided sequential treatment of Mycoplasma genitalium infections: a prospective evaluation. Clin Infect Dis 68, 554-560.
| Crossref | Google Scholar | PubMed |
20 Chua TP et al. (2022) Impact of 16S rRNA single nucleotide polymorphisms on Mycoplasma genitalium organism load with doxycycline treatment. Antimicrob Agents Chemother 66, e0024322.
| Crossref | Google Scholar | PubMed |
21 Vodstrcil LA et al. (2022) Combination therapy for Mycoplasma genitalium, and new insights into the utility of parC mutant detection to improve cure. Clin Infect Dis 75, 813-823.
| Crossref | Google Scholar | PubMed |
23 Unemo M et al. (2021) WHO global antimicrobial resistance surveillance for Neisseria gonorrhoeae 2017–18: a retrospective observational study. Lancet Microbe 2, e627-e636.
| Crossref | Google Scholar | PubMed |
24 Trembizki E et al. (2016) Further evidence to support the individualised treatment of gonorrhoea with ciprofloxacin. Lancet Infect Dis 16, 1005-1006.
| Crossref | Google Scholar | PubMed |
25 Bell SFE et al. (2023) Antimicrobial susceptibility assays for Neisseria gonorrhoeae: a proof-of-principle population-based retrospective analysis. Lancet Microbe 4, e544-e551.
| Crossref | Google Scholar | PubMed |
26 Adawiyah RA et al. (2024) Cost effectiveness of resistance-guided therapy for Mycoplasma genitalium in Australia. Sci Rep 14(1), 12856.
| Crossref | Google Scholar | PubMed |
![]() Gerald Murray is a senior research officer in the department of obstetrics, gynaecology and newborn health at The University of Melbourne, based at the Royal Women’s Hospital. His research interests include investigating mechanisms of antimicrobial resistance, studying the human microbiota in health and disease, and human papillomavirus (HPV). |
![]() Emma Sweeney is a senior research fellow at The University of Queensland Centre for Clinical Research. Her research interests include the development of novel molecular tools to detect and characterise pathogens of global health importance, with a particular focus on the sexually transmitted infection (STI) superbug Mycoplasma genitalium. |
![]() Catriona Bradshaw is a clinician researcher at Melbourne Sexual Health Centre, Monash University and Alfred Hospital. Her research interests include translational research to improve the treatment and control of drug resistant and refractory sexually transmitted infections. |