Hidden in plain sight: Tripneustes kermadecensis (Echinodermata: Echinoidea) is a junior synonym of the eastern Australian sea urchin Evechinus australiae described in 1878
Emily McLaren





A
B
C
D
E
F
Abstract
Accurate taxonomy and descriptions of species are key to understanding biodiversity. The echinoid genus Tripneustes is an ecologically and commercially important taxon that includes the tropical Tripneustes gratilla gratilla and the recently described T. kermadecensis from Australia and New Zealand. While examining the Australian Museum collections to clarify the distributions of these two species in eastern Australia we found potential senior type material for T. kermadecensis. These specimens from Sydney Harbour were originally described in 1878 as Evechinus australiae by Tenison-Woods but neither illustrated nor redescribed in any subsequent report. We undertook molecular and morphological analysis of these specimens to determine whether T. kermadecensis and E. australiae represent two distinct taxa or not. This included micro-computed tomography, quantification of test traits and molecular genetic analysis. The COI sequence and morphology of Evechinus australiae matched that of Tripneustes kermadecensis. As such, T. kermadecensis is a junior synonym of Evechinus australiae. The correct designation of this taxon is therefore Tripneustes australiae (Tenison-Woods, 1878).
ZooBank: urn:lsid:zoobank.org:pub:9B9E685C‐9C1C‐4645‐A799‐D97969BAA033
Keywords: Australia, Echinodermata, Echinoidea, integrative taxonomy, molecular taxonomy, morphology, taxonomy, Tripneustes.
Introduction
The echinoid genus Tripneustes is an ecologically and commercially important pan-tropical taxon. Tripneustes species are important herbivores (Lawrence and Agatsuma 2020) and as fast growing species, are of interest to fisheries and aquaculture (Sonnenholzner-Varas et al. 2018; Mos and Dworjanyn 2019). Tripneustes species occur in sea grass, coral and rocky reef ecosystems, and can have boom – bust population dynamics (Valentine and Edgar 2010; Lawrence and Agatsuma 2020). In high densities Tripneustes gratilla gratilla can overgraze sea grass (Alcoverro and Mariani 2002; Moreira‐Saporiti et al. 2023) and in Hawaii, T. g. gratilla are used as a biological control measure to limit the growth and impact of invasive algae (Neilson et al. 2018).
Globally there are five recognised species-group taxa within the Tripneustes genus. Tripneustes ventricosus, the Atlantic species, T. g. gratilla, the Indo-Pacific species, T. depressus, the eastern Pacific species, T. kermadecensis, the western Pacific species and the Red Sea endemic T. gratilla elatensis (Bronstein et al. 2016, 2017; Lawrence and Agatsuma 2020). Within Tripneustes, there is discordance in phylogenetic relationships indicated by data from nuclear and mitochondrial loci, and therefore conflicting information with respect to species delimitation (Lessios et al. 2003; Zigler and Lessios 2003; Bronstein et al. 2016, 2017). In a phylogeographic study of Tripneustes, Lessios et al. (2003) concluded that the eastern Pacific T. depressus and western Pacific T. g. gratilla are not distinct species. This finding was based on data from the mitochondrial locus cytochrome oxidase subunit I (COI) and Zigler and Lessios (2003) came to the same conclusion using the nuclear gene Bindin. However, when an integrative approach is used, combining nuclear and mitochondrial genetic markers with morphological traits, the five taxa are distinct and there is genetic evidence that T. g. gratilla might be a cryptic species complex (Bronstein et al. 2016, 2017). Introgression of the mitochondrial genome is hypothesised to be the driver of the discordance between nuclear and mitochondrial markers (Bronstein et al. 2016, 2017). Nevertheless, T. ventricosus and T. kermadecensis can be discerned from the other members of the genus using COI but nuclear markers are needed to distinguish between T. g. gratilla, T. g. elatensis and T. depressus (Bronstein et al. 2016, 2017).
In the subtropical-to-temperate transition zone of New South Wales (NSW) in eastern Australia, two species of Tripneustes co-occur, the tropical species T. g. gratilla and the subtropical species T. kermadecensis. Until recent taxonomic clarification (Bronstein et al. 2019), the presence of T. kermadecensis was misidentified as a range extension of T. g. gratilla (Castro et al. 2020). This confusion may have been driven by the wide variety of colour morphs that exist within T. g gratilla globally (Bronstein et al. 2016). The misidentification of the Tripneustes species in NSW highlights a knowledge gap in our understanding of the biology and ecology of these species in Australia. Tripneustes kermadecensis was first identified as a distinct species by morphological and molecular genetic analysis of specimens from the Kermadec Islands, New Zealand (Bronstein et al. 2017). Subsequently, T. kermadecensis was confirmed to be the species that also commonly occurs in the subtropical and temperate regions of NSW, locally as the ‘lamington urchin’ (Bronstein et al. 2017, 2019).
Owing to the previous confusion of T. kermadecensis and T. g. gratilla, we used the Australian Museum collection of Tripneustes to clarify the distributions of these species on the east coast of Australia (McLaren et al., in prep.). During our survey of the collection, we discovered juvenile Tripneustes specimens that represent type material of an eastern Australian species originally described by Tenison-Woods (1878) as Evechinus australiae, with the type locality Sydney Harbour (Port Jackson). We combine morphological analyses of a growth series, micro-computed tomography and genetic tools to test if the type material of Evechinus australiae is conspecific with Tripneustes g. gratilla or T. kermadecensis.
Materials and methods
Tripneustes material in the Australian Museum
The Australian Museum collection contains 232 Tripneustes specimens from Australia. Among these specimens we discovered three partly fragmented juvenile specimens (jointly registered under the inventory number AM J. 1099) that were labelled as type material of Evechinus australiae Tenison-Woods, 1878. The lot was subsequently split into three separate inventory numbers, each containing the remains of a single individual (AMJ. 30960, J. 30961 and J. 1099). According to Ramsay (1885), the type material of E. australiae was collected from Port Jackson (Sydney Harbour), New South Wales (NSW) (see below). This material included three dried specimens, one intact (J. 30960) and two fragmented tests (J. 30961 and J. 1099), all of which are juveniles.
Institutional abbreviations
AIM MA, Auckland War Memorial Museum, Auckland, New Zealand.
AM, Australian Museum.
NIWA, National Institute of Water and Atmospheric Research, Wellington, New Zealand.
NHMW-Geo, Naturhistorisches Museum Wien, Geologisch-Paläontologische Abteilung, Vienna, Austria.
NHMW-EV, Naturhistorisches Museum Wien, 3. Zoologische Abteilung, Sammlung Evertebrata Varia, Vienna, Austria.
Morphological analysis
To examine the morphology of specimens AM J. 30960 and J. 30961 the tests were analysed by micro-computed tomography (μCT) at the Australian Centre for Microscopy & Microanalysis, The University of Sydney, Australia. The specimens were scanned using a Bruker SkyScan 2214 system. The J. 30960 specimen scan was performed at 60 kV, 200 µA, with 2179 ms of exposure time, 1 × 1 binning and 3601 projection images, using a 0.25-mm aluminium filter. The J. 30961 scan was performed at 60 kV, 150 µA, with 757 ms of exposure time, 1 × 1 binning and 1801 projection images, using a 0.25-mm aluminium filter. T. kermadecensis paratype specimen NHMW-GEO-2017-0016-0001 was scanned at the Natural History Museum Vienna, Austria, using a YXLON FF35 CT equipped with a YXLON FXT 225.48 micro-focus directional beam tube and a 4343CT CsJ flat panel detector. Scanning was performed at 100 kV, 130 µA, with 1000 ms exposure time, 1 × 1 binning and 2700 projection images using a 0.4-mm copper filter. Isotropic voxel sizes of the reconstructed datasets were 36.5 to 144 µm, depending on the size of the specimen. Reconstructions of the specimens were performed using the program Dragonfly (ver. 2020.2 for Microsoft Windows, Object Research Systems (ORS) Inc, Montreal, QC, Canada, see http://www.theobjects.com/dragonfly).
Traits from these visualisations were compared to those in the type material of T. kermadecensis (Bronstein et al. 2017) and non-type material of T. gratilla gratilla. The analysed traits included test shape, adapical ambulacral primary tuberculation, ambital ambulacral primary tuberculation, occlusion of ambulacral plates from the perradial suture, size of the interambulacral tubercles and peristome size (Table 1).
Trait | T. gratilla gratilla | T. kermadecensis | J. 30960 | J. 30961 | |
---|---|---|---|---|---|
Test shape | High (TH > 63% TD) | Depressed (mean TH 51% TD) | TH 52% TD | TH 44% TD | |
Adapical ambulacral primary tubercles | Every second plate | Every second plate | Every second plate | Every second plate | |
Ambital ambulacral primary tubercles | Every second plate | Every fourth plate | Varies between 3 and 4, see Fig. 5 | Varies between 3 and 4, see Fig. 6 | |
Adoral ambulacral primary tubercles | Every third plate | Every second plate | Every second plate | Every second plate | |
Ambulacral plates occluded from perradial suture | None | Numerous (2 in 3 at ambitus) | Numerous (2 in 3 at ambitus) | Numerous (2 in 3 at ambitus) | |
Size of interambulacral tubercles | Half of plate height | Full plate height | Does not reach full plate height | Does not reach full plate height | |
Mean peristome size | 28.1% TD | 25.2% TD | 38.38% TD | NA | |
Deeply bifurcate compass ends | Yes | No | NA | NA | |
Compass ends flat and wide | No | Yes | NA | NA | |
Dumb-bell shaped ossicles very abundant in distal tube feet | Yes | No | NA | NA |
TD, test diameter.
The tuberculation patterns of Tripneustes change during growth. As the E. australiae material consists of small individuals, to compare this material to other Tripneustes species, we constructed a growth series of T. kermadecensis consisting of cleaned tests obtained from the Sea Urchin Science Centre and Gallery (SUSCG) (SUSCG. 1–8) and specimens collected from the Solitary Islands (J. 31050, J. 31051, J. 31052) (ranging 20.88 to 101.3 mm in test diameter, TD). To understand the variation in ambulacral tuberculation, both within and between individual specimens, we counted the number of tubercles on 10 random ambulacral plates at the ambitus in both columns of each ambulacrum (n = 5) per specimen (n = 11). Based on the description (fig. 4C in Bronstein et al. 2017), T. kermadecensis would be expected to have a primary tubercule on every 4th plate at the ambitus and therefore would have three tubercles per 10 plates. When the counts for each individual are pooled across all five ambulacra, T. kermadecensis is expected to have 25 tubercles per 100 plates with primary tubercles.
Molecular analysis
Total genomic DNA was extracted in an ultraclean room using the Qiagen Investigator kit with the addition of carrier RNA (at step five of the manufacturer’s protocol) to enhance the binding of DNA to the spin column. As there was no visible tissue left on the E. australiae type specimens, the tests were swabbed with swabs dipped in AL lysis buffer (Qiagen) to collect remaining cells and tissue, followed by extraction according to the manufacturer's protocol for extraction from surface and buccal swabs, with minor modifications. Specifically, at the DNA elution step, we applied 25 μL of pre-heated elution buffer (70°C) and allowed an incubation time of 3 min prior to centrifugation. This process was repeated twice for each sample, re-pipetting the eluted DNA from the first round back on the spin-column filter.
As the Evechinus australiae material was highly degraded and poorly preserved for molecular analysis (dried specimens collected >140 years ago) genetic analysis of the mitochondrial marker cytochrome oxidase subunit I (COI) was attempted by a nested PCR design, including an initial amplification of the material with the specific echinoid primers (COI16bf/COIer). We subsequently used the products of the first PCR reaction as a template for a second amplification using the universal metazoan primers of Folmer (Fig. 1) (Folmer et al. 1994).
Visualisation of the gene regions and primer binding sites used to amplify and re-amplify the mitochondrial cytochrome c oxidase subunit 1 gene (COI).
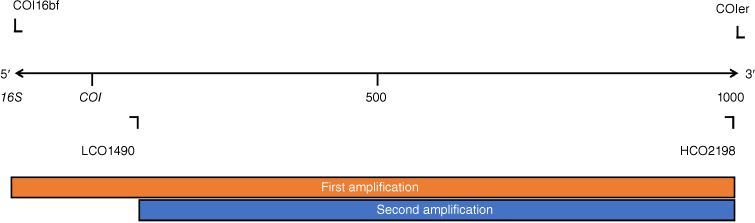
Amplification of the initial COI fragment followed Bronstein et al. (2019) using the forward primer COI16bf 5′-AGCCAGGTCAGTTTCTATCT-3′ (Bronstein et al. 2019) with the reverse primer COIeR5′-GCTCGTGTGTCTACGTCCAT-3′ (Arndt et al. 1996) (flanking a region of ~1000 bp). PCR reactions were done in a total volume of 25 µL and contained 5.0 μL of DNA, 2.5 μL of 10× CoralLoad PCR Buffer, 2.0 μL of 25 mM of MgCl2, 0.5 μL of 10 mM of dNTPs, 0.25 μL of each 10-mM forward and reverse primers, 0.25 μL of CoralLoad DNA polymerase and brought to 25 μL with nuclease free water. PCR conditions were 3 min at 94°C followed by 35 cycles of 94°C for 30 s, 65°C for 30 s and 72°C for 70 s, and a final extension step of 10 min at 72°C.
These PCR products were subsequently PCR re-amplified using the primers LCO1490 5′-GGTCAACAAATCATAAAGATATTGG-3′ and HC02198 5′-TAAACTTCAGGGTGACCAAAAAATCA-3′ (Folmer et al. 1994) to generate a ~700-bp amplicon. PCR reactions and thermocycler conditions were repeated as described above. PCR products were purified using ExoSap. Samples were sequenced in both directions using the nested PCR primers (i.e. the Folmer primers) at the Australian Genome Research Facility (AGRF). Sequences were deposited in GenBank (Accession numbers: OR038167, OR038168, OR038169, OR038170 and OR038171). Sequence assembly was performed in Geneious Prime (ver. 11.0.14.1+1, Domatics, see https://www.geneious.com/). To confirm the identity of the specimens these sequences were subjected to a BLAST search prior to inclusion in a phylogenetic analysis as described below.
Additional (juvenile and adult) specimens (AM J. 1117, J. 4250 and J. 5003) from the Australian Museum from the location where the E. australiae type material was collected, Port Jackson, and newly collected samples from the Solitary Islands (AM J. 31050, J. 31051 and J. 31052) were also included in the genetic analysis. Both wet and dry material was investigated using the mitochondrial marker listed above. Total genomic DNA was extracted from the tube feet and spine muscle or internal tissue that could be extracted from the dry and wet specimens using the Bioline ISOLATE II Genomic DNA kit (Meridian Bioscience) following manufacturer's instructions. For AM J. 1117, J. 4250 and J. 5003, instead of eluting to 100 μL, the DNA was eluted into 50 μL and the final elution buffer was run through the membrane twice and incubated at room temperature for 3 min. PCR conditions for each amplification were performed as outlined above, however 2 μL of DNA template was used in the PCR protocol. For the newly collected samples, AM J. 31050, J. 31051 and J. 31052, the DNA was eluted 100 μL and only one PCR amplification was performed with the COI16bf/COIer primers and PCR conditions as outlined above. All sequences were deposited in GenBank (Accession numbers: OR144098, OR144099, OR144100) (Supplementary Table S1).
Phylogenetic analysis was performed using the COI sequences generated for the Evechinus australiae type material (AM J. 30961), the successfully sequenced T. kermadecensis material from AM (J. 1117, J. 23530, J. 24345, J. 4250 and J. 5003) and the Solitary Islands material (J. 31050, J. 31051 and J. 31052). These sequences were complemented with publicly available data from the original description of T. kermadecensis and T. g. elatensis (Bronstein et al. 2016, 2017, 2019). We subsequently created a COI dataset with these 69 sequences and our nine novel sequences, including the Evechinus australiae material. As sequences varied in length, these were trimmed to align with the shortest sequence generated to maximise overlap (J. 30961, 283 BP). Raw sequences were edited and aligned using Geneious Prime and phylogenetic reconstruction was done using both Maximum Likelihood (ML) and Bayesian Inference (BI) analysis. ML analysis was performed in IQ tree and BI was performed using MrBayes (ver. 3.3.7a, see https://nbisweden.github.io/MrBayes/index.html; Ronquist et al. 2012; Nguyen et al. 2015; Chernomor et al. 2016). MrBayes analysis was run for 10 million generations, sampling every 100th tree and discarding the initial 25% of data as burn-in. Toxopneutes pileolus (GenBank Accession Numbers MK084954.1, MK084955.1) and Lytechinus variegatus (GenBank Accession Numbers MG676469, MG676468) (Bronstein and Kroh 2019; Bronstein et al. 2019) were used as outgroups for all phylogenetic analyses.
Median-joining networks were calculated for the 283 BP alignment of all Tripneustes spp. used for the phylogenetic analysis, including T. kermadecensis from NSW, New Zealand and the Solitary Islands, following morphological and phylogenetic species assignment (see Supplementary Table S1) (See also Bronstein et al. (2016, 2017, 2019), using PopArt with default settings (Leigh and Bryant 2015). The 76 sequences used for the alignment (excluding the outgroups) yielded 25 unique haplotypes across the Tripneustes genus for COI. The 26 NSW, New Zealand and Solitary Islands sequences collapsed into five unique haplotypes and we added biogeographic provinces to the individual sequences to determine unique haplotypes by provinces or genetic connectivity between these provinces. The locations were Port Stephens (32°42′38.1″S, 152°11′11.9″E), the Solitary Islands (29°55′53″S, 153°23′34″E), Norfolk Island (29°02′60″S, 167°59′13″E), Sydney (33°49′13″S, 151°17′12″E), Port Jackson (33°49′S, 151°16′E) and Kermadec Islands (29°4′40″S, 178°09′14″W).
Statistical analysis
To determine the variability of tuberculation within the growth series, a Kolmogorov–Smirnov test was performed to determine if the observed tuberculation differed from a normal distribution with a population mean of 25 tubercules per 100 plates. To test if this trait varied with size, we performed a linear regression between the number of tubercles per 100 plates and test diameter. The model was visually checked for normality and equal variance by inspecting Q–Q plots and the spread of residuals with a residual’s v. fitted plot in R Studio (ver. 4.1.0 (18 May 2021), R Foundation for Statistical Computing, Vienna, Austria). The variability within an individual was analysed by a Chi-Square goodness of fit test with the expected and observed values of tubercles per 100 plates. Each individual was tested for deviance from the expected value of 25 tubercles per 100 plates counted (Supplementary Table S2).
Results
Morphological analysis
The main diagnostic features for the two species are listed in Table 1. Comparing T. kermadecensis individuals across the size range from juveniles to adults (22.8–101.3-mm test diameter) reveals that ambulacral tuberculation varies between and within individuals (Supplementary Table S2, Fig. 2, 3 and 4). The observed tuberculation values of the growth series were significantly different to a normally distributed population with a mean of 25 (D = 0.9, d.f. = 11, P < 0.001). The number of primary tubercles also varied within an individual, some ambulacra having 4 tubercles per 10 plates and others having 3 (Fig. 4, Supplementary Table S2). In total, 6 of the 11 individuals examined deviated significantly from the expected value of 25 tubercles per 100 plates (Supplementary Table S2). These individuals had TD ranging from 91.1 to 22.8 mm (Table 2). There was also no significant relationship between the test diameter of specimens and number of tubercles (F = 0.019, d.f. = 1.9, P = 0.89).
Tests and tuberculation of Tripneustes kermadecensis growth series constructed from specimens from The Sea Urchin Science Centre and Gallery. Numbers (1–8) represent the SUSCG identifier (SUSCG. 1–8). Scale bar: 2 mm.
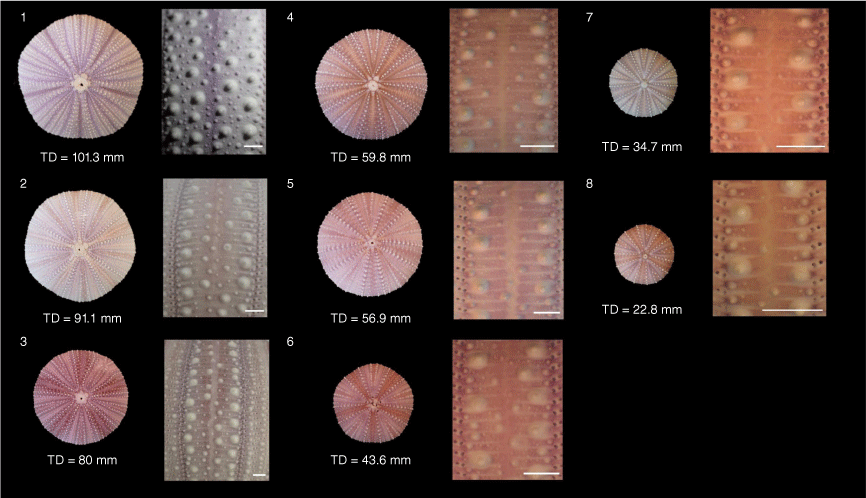
Variation of tuberculation patterns within and between individuals in 11 Tripneustes kermadecensis across a growth series (22.8–101.3-mm test diameter). Letters on the x-axes denote the ambulacra of each individual in accordance with the Lovén plane. Specimen numbers refer to Fig. 2 and 3.
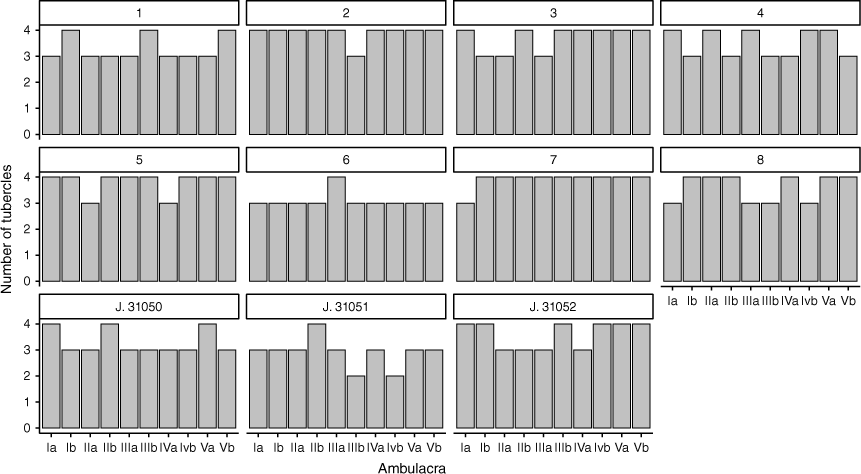
Individual | Test diameter (mm) | Height (mm) | Height (% TD) | Peristome (mm) | Peristome (% TD) | |
---|---|---|---|---|---|---|
SUSCG. 1 | 101.3 | 57.8 | 57.06 | 22.3 | 22.01 | |
SUSCG. 2 | 91.1 | 54.7 | 60.043 | 20.2 | 22.17 | |
SUSCG. 3 | 80 | 46.1 | 57.63 | 18.6 | 23.25 | |
SUSCG. 4 | 59.8 | 33.5 | 56.02 | 15.8 | 26.42* | |
SUSCG. 5 | 56.9 | 33.5 | 58.88 | 15.8 | 27.77* | |
SUSCG. 6 | 43.6 | 23.5 | 53.9 | 13.6 | 31.19* | |
SUSCG. 7 | 34.7 | 19.3 | 55.62 | 11 | 31.7* | |
SUSCG. 8 | 22.8 | 11.9 | 52.19 | 8.4 | 36.84* | |
J. 31050 | 63.1 | 33.1 | 52.46 | 17.2 | 27.26 | |
J. 31051 | 59 | 30.8 | 52.2 | 16.7 | 28.31 | |
J. 31052 | 72.3 | 33.3 | 46.06 | 20.8 | 28.77 | |
J. 30960 | 27.1 | 14.1 | 52.03 | 10.4 | 38.38* | |
J. 30961 | 35.9 | 16 | 44.57 | NA | NA |
Data that are significant are denoted by an asterisk (*).
When comparing the type material of T. kermadecensis (Bronstein et al. 2017) with that of E. australiae, the specimens are similar with respect to most of the diagnostic traits described by Bronstein et al. (2017). However, as the E. australiae material consists of juveniles, some of the traits were not evident (Table 1). The E. australiae specimens share the depressed test characteristic of T. kermadecensis, a similar pattern of adapical and adoral ambulacral plating, and occlusion of plates from the perradial suture. However, the size of the interambulacral tubercles did not reach the full plate height and the ambitally ambulacral plating varied between every third and fourth plate (Fig. 5 and 6). The peristome size also seems to not align with the description in Bronstein et al. (2017), showing a proportion of 38.38% of the TD, as opposed to the 25.2% TD noted in the original description of T. kermadecensis that was based on specimens larger than those of E. australiae (Table 2). Our examination of test tuberculation in an ontogenetic series of T. kermadecensis revealed that the ambulacral tuberculation varied both between and within individuals. This trait may not be as useful a diagnostic trait as previously considered and ontogenetic stage and test sizes have to be considered. Notably, Bronstein et al. (2017) remains the key diagnostic resource for identification of this species and differentiation from T. gratilla gratilla.
Micro computer tomography reconstruction of Evechinus australiae lectotype AM J. 30960. (a) Lateral view focusing on an ambulacrum. (b) Lateral view focusing on an interambulacrum. (c) Detail of the ambital region in another ambulacrum.
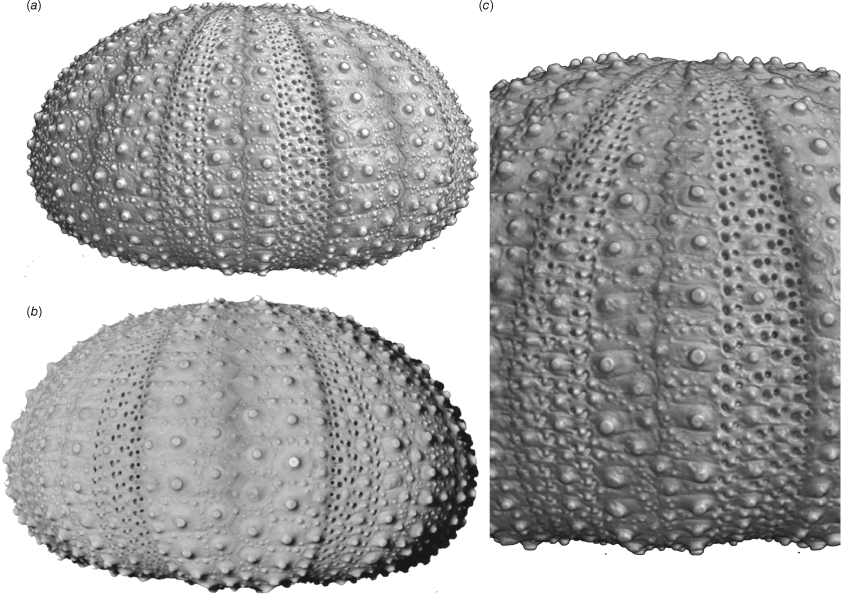
Micro computer tomography reconstruction of Evechinus australiae paralectotype AM J. 30961. (a) Lateral view of two fragments. (b) Lateral view focusing on an ambulacrum of the largest fragment. (c) Lateral view focusing on an interambulacrum of the largest fragment. (d) Detail of the ambital region in another ambulacrum.
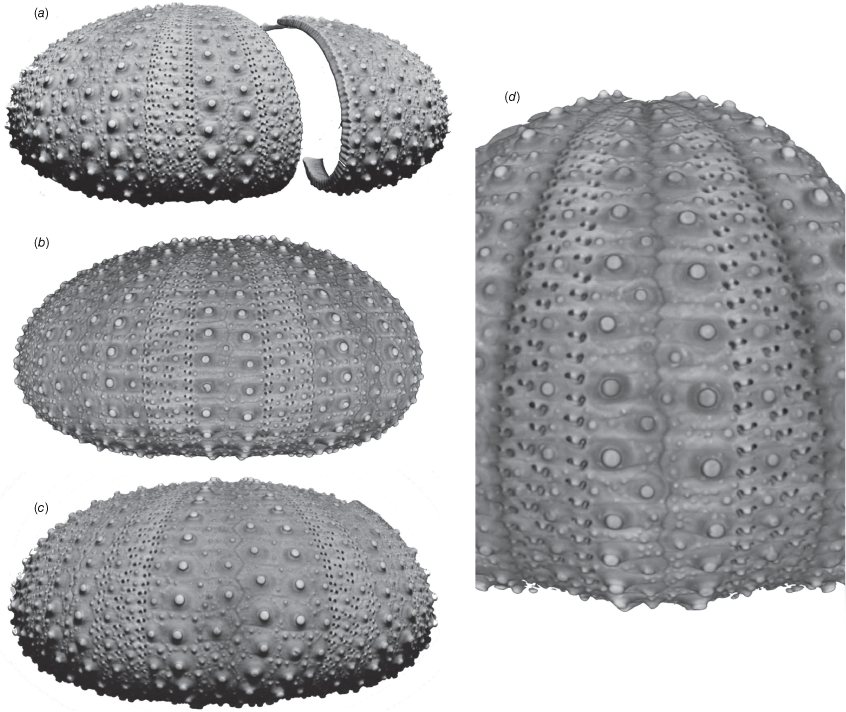
We recommend using the following morphological traits to diagnose Tripneustes australiae and assist in the identification of this species in the field.
Depressed test: test height between ~45 and 60% of the test diameter (TD).
Large peristome or mouth: between 22 and 27% of the TD.
Black interambulacral or pedicellariae.
White spines.
Dark grey, red–orange or white tube feet.
Absence of banded or greenish tube feet (as seen in T. g. gratilla).
We encourage those identifying these two species in the field to primarily rely on the shape of the test and tuberculation pattern for identification, as there can be large variation in colour within T. gratilla gratilla and T. australiae. In particular, there are two common colour morphs of T. australiae on the east coast of Australia, the lamington morph with black interambulacra or pedicellaria and white or dark tube feet and another morph with orange–red tube feet that was noted to occur on Norfolk Island (Bronstein et al. 2019) but has also been collected in Port Stephens and Sydney on the NSW mid coast (E. McLaren, pers. obs.).
Molecular diagnosis of the type material of Evechinus australiae
DNA extraction and PCR amplification were successful in one of the three syntypes of Evechinus australiae. COI sequences of specimen J. 30961, despite the highly degraded DNA, matched those of T. kermadecensis. The sequence produced was 283 BP and a BLAST search identified the sequence as T. kermadecensis (query cover 100%; E value = 0; Accession number MK084948.1).
Molecular analysis
Both ML and BI analyses produced similar phylogenies for all clades of Tripneustes (Fig. 7). As expected with mitochondrial markers, the three major clades recovered were T. kermadecensis, T. ventricosus and a mixed clade consisting of T. g gratilla, T. g. elatensis and T. depressus samples. All of the Australian Museum material was identified as T. kermadecensis from the type region (Supplementary Table S3) from which DNA could be extracted (Table 3), and the Evechinus australiae type material also belonged to the T. kermadecensis clade. Specimens from the Solitary Islands (AM: J. 31050, J. 31051 and J. 31052, GenBank: OR144098, OR144099 and OR144100) (Fig. 7) also fell within the T. kermadecensis–australiae clade.
Phylogenetic tree of Tripneustes based on 80 partial sequences (283 bp) of the mitochondrial COI gene. Toxopneustes pileolus and Lytechinus variegatus were used as outgroups. Bootstrap support values and posterior probabilities from both maximum likelihood and Bayesian inference analyses are reported above the respective nodes, before and after the slash respectively. Evechinus australiae type specimen AM J. 30961 (OR038171) falls into the T. kermadecensis clade. All sequences generated for this study are in bold and the type specimen is marked with an asterisk (*) (AM J. 30961 (OR038171)).
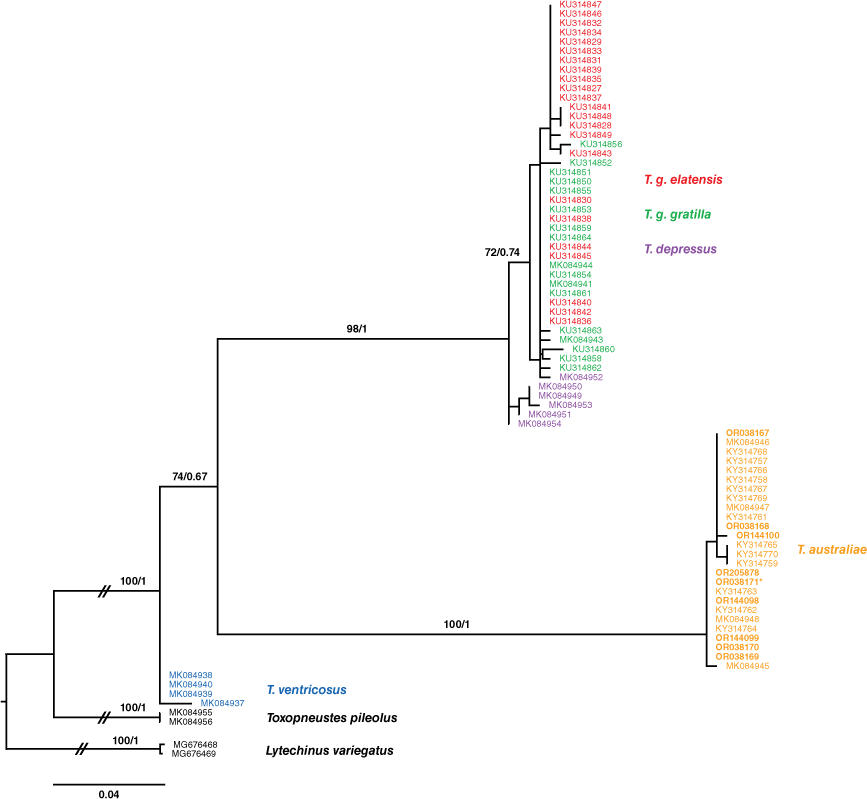
Registration number | Collection location | Year collected | Preservation method | |
---|---|---|---|---|
J. 30961 | Port Jackson | 1878 | Dry | |
J. 1117 | Port Jackson | NA | Dry | |
J. 4250 | Port Jackson | 1923 | Ethanol | |
J. 23530 | Port Stephens | 1998 | Ethanol | |
J. 5003 | Port Jackson | 1926 | Dry |
Our haplotype-joining network for COI for Tripneustes showed similar groupings to the phylogenetic trees. Tripneustes australiae and T. ventricosus clustered separately and had unique haplotypes, while T. g. gratilla, T. g. elatensis and T. depressus grouped together and shared haplotypes (Fig. 8a). Regarding T. australiae, the two most frequent COI haplotypes occurred from the Solitary Islands to Sydney, suggesting that the populations of T. australiae on the east coast of Australia and those on offshore islands are genetically similar. However, there is one haplotype that occurs exclusively in the Kermadec Islands, one that occurs exclusively in the Solitary Islands and one that only occurs in Sydney (Sydney) (Fig. 8b).
Median-joining haplotype networks for COI sequences of Tripneustes (a) and T. australiae (b). The Tripneustes and T. australiae network consists of 76 and 26 sequences respectively that are 283 bp long. The bars represent the number of substitutions between the nodes and haplotype frequency is represented by the size of the nodes. Our geographical categories were Port Stephens (PS), the Solitary Islands (SI), Norfolk Island (NI), Sydney (SYD) and Port Jackson (PJ). Details on the sequences used are given in Supplementary Table S1. The position of the type specimen J. 30961 is highlighted and marked with an asterisk (*) in (a) and (b).
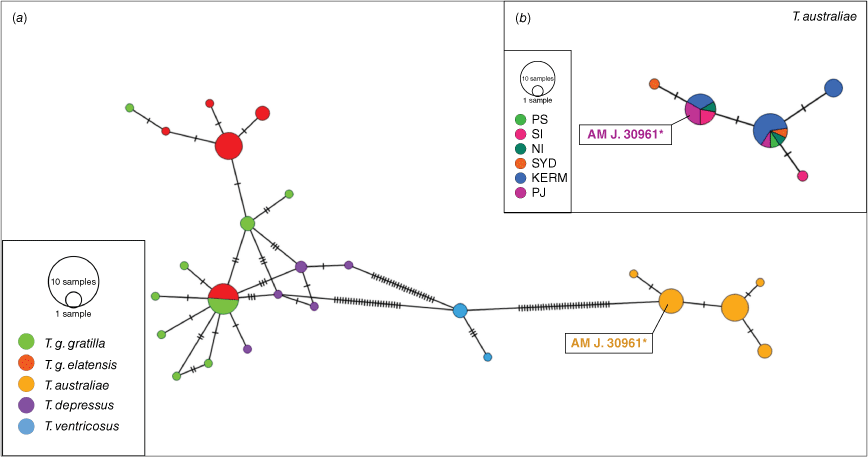
Systematics
Classification follows Kroh (2020). Stokes and Kroh (2022) describe the changed attribution of Echinoidea to Schumacher (1817). As the morphological description of Evechinus australiae is based on juvenile specimens, and due to the fact that many echinoids show allometric growth (e.g. Mooi et al. 2014) and ontogenetic increase of tubercle number, additional T. kermadecensis from NSW (J. 31050, J. 31051 and J. 31052) and T. gratilla gratilla from various Indo-West Pacific localities were examined in a size series to facilitate comparison of similarly sized specimens.
Tripneustes australiae (Tenison-Woods, 1878) comb. nov.
Evechinus australiæ Tenison-Woods, 1878, p. 167.
Evechinus australiæ – Ramsay (1885), p. 23 [type locality information provided].
Evechinus australiæ Ten.-Woods – Whitelegge (1889), p. 204. [citing Tenison-Woods].
Euechinus [sic!] australiae Ten. Woods – Meissner (1891), p. 166. [summary of Whitelegge’s report].
E. australe – Farquhar (1894), p. 195 [misspelling].
Evechinus australiæ – Mortensen (1943), p. 298. [mentioned as synonym of Tripneustes gratilla].
Tripneustes kermadecensis Bronstein et al. (2017), pp. 7–13; fig. 2A–M, 3A–E, 4C, 10A, B, 11A–C, 12A, D, E, H.
Lectotype, designated herein. Australian Museum (AM) J. 30960, 1 intact specimen (Fig. 2) (https://sydney.pedestal3d.com/r/5UJwvpuVld).
Paralectotypes. Two fragmentary specimens: AM J. 30961, AM J. 1099 (Fig. 3).
Holotype. Auckland War Memorial Museum, Auckland, New Zealand (AIM MA) specimen AIM MA73563 (isolate nr. Ker907), a cleaned corona, spines and lantern, collected by J. David Aguirre and Libby Liggins on 31 October 2015 at a depth of 0 to 10 m.
Paratypes. Two cleaned coronas (AIM MA73564 and NHMW-Geo 2017/0016/0001; isolate Ker903 and Ker910 respectively) and five intact, formalin-fixed specimens preserved in ethanol (AIM MA73565, AIM MA73566, NIWA 116558, NHMW-EV 20452 and NHMW-EV 20453 [=isolates Ker902, Ker904, Ker911, Ker901 and Ker906 respectively]); collection details as for holotype.
‘Small sea beach about three miles [~4.8 km] north of Port Jackson heads’ (Ramsay 1885, footnote on p. 23); Sydney Harbour, NSW, Australia; no depth recorded. As described by Ramsey, a small sea beach 3 miles [~4.8 km] north of the Port Jackson Heads could refer to Collins Beach (33°48′31.0″S, 151°17′28.6″E), Store Beach (33°48′42.1″S, 151°17′22.9″E) or Little Manly (33°48′24.6″S, 151°17′14.1″E). To provide as close a location as possible we use the coordinates of Port Jackson (33°49′S, 151°16′E) as the type locality.
West of Meyer Islands (29°14′39.06″S, 177°52′46.56″W), near Raoul Island, Kermadec Islands, New Zealand; 0–10-m depth.
Additional genetically confirmed material of T. australiae has been deposited in the Australian Museum (AM J. 31050, J. 31051 and J. 31052). These were collected from North Solitary Island (29°55′40.8″S, 153°23′31.7″E) on 1 September 2022; GenBank Accession numbers: OR144098, OR144099 and OR144100.
The subtropical and temperate east coast of Australia and off-shore Islands in the Pacific (Lord Howe Island, Norfolk Island and the Kermadec Islands), to the west coast of New Zealand (McLaren et al., in prep.).
A species of Tripneustes with ambulacral primary tubercles typically occurring ambitally on every third to fourth plate (see Fig. 2; very rarely there are cases in which four subsequent ambulacral plates without primary tubercles occur at the ambitus, e.g. Fig. 2, number 6, left column of ambulacrum); flattened test ranging from ~45 to 60% height of the test diameter; large peristomal opening (~25% of TD) without sunken margin; presence of one to two plates for every four ambulacral plates occluded from perradial suture, giving the perradial suture a unique irregular zigzag (except in specimens <80 mm TD); primary series of interambulacral tubercles continuous from peristome to apex; tubercles large; secondary interambulacral tuberculation reduced above ambitus.
Micro computed tomography (μCT) imagery of the lectotype was used to generate a 3-D render of the specimen (Fig. 5) (see https://sydney.pedestal3d.com/r/5UJwvpuVld). The lectotype (J. 30960) is 27.1 mm TD, with a depressed test. The test height is 52% of the TD (Fig. 5). The apical disc and genital plates are not preserved. The ambulacral plating is trigeminate and the pores form three vertical columns separated by columns of tubercles. The inner- and outermost of these are almost straight, while the middle part is less linear and wiggles from the aboral to oral side. Close to the peristome and apical disc, primary tubercles occur on each or every second ambulacral plate and tubercles occur ambitally on every third plate. Some plates are occluded from the perradial suture but not as numerously as seen in adult individuals. This feature of juveniles was also noted by Bronstein et al. (2017). Along the ambulacra, some plates are larger and more asymmetrical than others, and as such the perradial suture is irregular and zigzagged, a typical trait of this species (Bronstein et al. 2017). From the adoral to adapical side the interambulacral plates have one column of primary tubercles running through the middle of the plates, and one column of secondary tubercles on either side of these primary tubercles. Towards the adoral side these plates can have up to four tubercles per single plate (Fig. 5). Unlike the holotype assigned by Bronstein et al. (2017), in J. 30960, the areoles of the tubercles do not take up most of the height of the interambulacral plates. However, this may be a juvenile feature. In other small genetically confirmed specimens, similar tubercule style occurs (see below) (Fig. 3). The peristome (10.4 mm diameter) is 38% of TD that is larger than reported in Bronstein et al. (2017) and typical for the differences observed between juveniles and adults in camarodont echinoids. Specimen colour of the 140+-year-old specimen has faded but the test still has the typical light pink/violet hue. See Bronstein et al. (2017) for a description of recently collected specimens.
Specimen AM J. 30961 has the characteristic depressed test (Fig. 6, Table 2). The test is 35 mm in diameter, with a test height of 16 mm (44% TD). This specimen shares the same traits of ambulacral and interambulacral tuberculation as found in AM J. 30960, however, fewer ambulacral plates are asymmetrical and occluded from the perradial suture, meaning the perradial suture is more symmetrical but still has the distinct zigzag. Specimen AM J. 1099 is a fragmented specimen of between 20 and 30 mm TD. The morphology agrees with that of the other specimens in the type series of T. australiae but offers no additional detail.
Although the trait of one primary tubercule on every fourth ambulacral plate around the ambitus is suggested to be diagnostic (Bronstein et al. 2017), comparison of a larger size range including specimens as small as 22.8 mm revealed that this trait is variable (see above).
Conclusions
Considering the weight of evidence; locality of the specimen, the depressed test morphology and irregular zigzag of the perradial suture, we conclude that the type material of Evechinus australiae Tenison-Woods, 1878 is conspecific with that of Tripneustes kermadecensis described by Bronstein et al. (2017). The apparent differences between the two nominal taxa are solely caused by the fact that the former is based on juvenile specimens. Both our novel morphological and genetic data support the synonymy of T. kermadecensis and Evechinus australiae, wherefore australiae is transferred to the genus Tripneustes and T. kermadecensis placed into the synonymy of T. australiae.
Genetic evidence based on the mitochondrial gene COI supports the synonymy of T. kermadecensis and T. australiae that share haplotypes (Fig. 8) that do not occur in and are very distinct from those of other Tripneustes species. Mitogenome introgression that drives discordance between nuclear and mitochondrial markers in this genus (Lessios et al. 2003; Zigler and Lessios 2003; Bronstein et al. 2016, 2017) was thus far only observed between T. gratilla gratilla, T. gratilla elatensis and T. depressus. As such, because this study solely employed mitochondrial loci (due to the age and condition of the specimens), recognising that nuclear molecular evidence would be required to completely delineate species of Tripneustes is important. However, as previously established by Bronstein et al. (2017), T. kermadecensis (now T. australiae) can be unambiguously discerned from T. g. gratilla using COI.
With clarification of the taxonomy of the Australian subtropical and temperate T. australiae the Australian Museum collections indicated that the current distribution of this species extends from Byron Bay (28°37′S) in the north to Narooma (36°15′S) in the south in NSW, over 8° of latitude and 900 km. Tripneustes australiae has a trans-Tasman distribution of 17° and over 1800 km to Norfolk Island and Lord Howe Island in Australia, and in New Zealand to the Kermadec Islands and the west coast of the North Island.
Our haplotype network indicates genetic similarity between the populations of T. australiae on the east coast of Australia and the offshore islands in the Tasman Sea and New Zealand. The larval supply of T. australiae from the east coast of Australia to the Kermadec Islands and other off-shore Islands in the Tasman Sea such as Lord Howe and Norfolk Island is likely facilitated by transport in the Tasman Front. This is a major current that crosses from the mid north coast of NSW to northern New Zealand (Ridgway and Godfrey 1997). Interestingly, there are also haplotypes unique to the east coast of Australia that are not shared with the offshore islands and there is also a unique haplotype in the Kermadec Islands, although the latter may be an artefact of a low sample size (n = 25) of individual sequences. Future studies are needed to expand the collection effort of T. australiae in locations across the distribution range to understand how well these populations are connected through population genetics analyses. Sea urchins sympatric with T. australiae in eastern Australia, the diadematoid Centrostephanus rodgersii and the echinometrid Heliocidaris tuberculata have similar cross-Tasman distributions. Population genetic analyses for both of these species indicate that populations on the east coast of Australia colonised across the Tasman Sea, facilitated by the Tasman Front (Gall 2016; Thomas et al. 2021).
Highlighting that the type locality of T. australiae is Sydney Harbour (Port Jackson) and that this species has been present at this location at least since description by Tenison-Woods in 1878 is important. Tripneustes australiae is an ecologically important species in Australia and should be considered in regional conservation and management plans. T. australiae has a relatively limited latitudinal distribution along the coast of Australia for a species with a planktotrophic larva, despite the potential for a greater distribution facilitated by the southward-flowing East Australia Current (EAC). In this regard T. australiae is similar to H. tuberculata that has a similar limited poleward distribution that is considered to be due to the cold intolerance of the larvae (Byrne et al. 2022).
The sea scape of the south-east coast of Australia is dynamic and undergoing tropical/sub-topicalisation, as the poleward flow and temperature of the EAC increase with ocean warming (Suthers et al. 2011; Vergés et al. 2014). This results in poleward range extension of tropical and subtropical species with dispersive larvae. Tropical species are expanding while the distribution range of temperate species is contracting (Gervais et al. 2021). The fate of species such as T. australiae that appear limited to the subtropical and warm temperate regions of Australia and New Zealand is uncertain in the face of changing climate.
Data availability
CT scanning data generated for this study have been deposited in the NHMW research data repository (doi:10.57756/a1x6kf, doi:10.57756/5zzydy and doi:10.57756/7pkzpx) and the genetic data have been uploaded to GenBank (Supplementary Table S1). Data related to the phylogenetic analysis and haplotype networks have been uploaded to FigShare (https://figshare.com/s/e434c29cf729268b6384).
Declaration of funding
This research was financially supported by the Australian Government Research Training Program (ATP) PhD scholarship and the Holsworth Wildlife Research Endowment – Equity Trustees Charitable Foundation and the Ecological Society of Australia to E. McLaren and an Australian Research Council Discovery Early Career Research Award (DE230100141) to B. Sommer.
Acknowledgements
We gratefully acknowledge the Australian Museum and the Australian Centre for Wildlife Genomics for providing samples used in this study and access to laboratory space. We thank staff at the Australian Centre for Microscopy and Microanalysis for assistance in CT scanning, particularly Mathew Foley.
References
Alcoverro T, Mariani S (2002) Effects of sea urchin grazing on seagrass (Thalassodendron ciliatum) beds of a Kenyan lagoon. Marine Ecology Progress Series 226, 255-263.
| Crossref | Google Scholar |
Arndt A, Marquez C, Lambert P, Smith MJ (1996) Molecular phylogeny of Eastern Pacific sea cucumbers (Echinodermata: Holothuroidea) based on mitochondrial DNA sequence. Molecular Phylogenetics and Evolution 6, 425-437.
| Crossref | Google Scholar | PubMed |
Bronstein O, Kroh A (2019) The first mitochondrial genome of the model echinoid Lytechinus variegatus and insights into Odontophoran phylogenetics. Genomics 111(4), 710-718.
| Crossref | Google Scholar | PubMed |
Bronstein O, Kroh A, Haring E (2016) Do genes lie? Mitochondrial capture masks the Red Sea collector urchin’s true identity (Echinodermata: Echinoidea: Tripneustes). Molecular Phylogenetics and Evolution 104, 1-13.
| Crossref | Google Scholar | PubMed |
Bronstein O, Kroh A, Tautscher B, Liggins L, Haring E (2017) Cryptic speciation in pan-tropical sea urchins: a case study of an edge-of-range population of Tripneustes from the Kermadec Islands. Scientific Reports 7, 5948.
| Crossref | Google Scholar | PubMed |
Bronstein O, Kroh A, Miskelly AD, Smith SDA, Dworjanyn SA, Mos B, Byrne M (2019) Implications of range overlap in the commercially important pan-tropical sea urchin genus Tripneustes (Echinoidea: Toxopneustidae). Marine Biology 166, 34.
| Crossref | Google Scholar |
Byrne M, Gall ML, Campbell H, Lamare MD, Holmes SP (2022) Staying in place and moving in space: contrasting larval thermal sensitivity explains distributional changes of sympatric sea urchin species to habitat warming. Global Change Biology 28, 3040-3053.
| Crossref | Google Scholar | PubMed |
Castro LC, Cetina‐Heredia P, Roughan M, Dworjanyn S, Thibaut L, Chamberlain MA, Feng M, Vergés A (2020) Combined mechanistic modelling predicts changes in species distribution and increased co‐occurrence of a tropical urchin herbivore and a habitat‐forming temperate kelp. Diversity and Distributions 26, 1211-1226.
| Crossref | Google Scholar |
Chernomor O, von Haeseler A, Minh BQ (2016) Terrace aware data structure for phylogenomic inference from supermatrices. Systematic Biology 65, 997-1008.
| Crossref | Google Scholar | PubMed |
Farquhar H (1894) Notes on New Zealand echinoderms. Transactions of the New Zealand Institute 27, 194-208 Available at https://www.biodiversitylibrary.org/page/3300653.
| Google Scholar |
Folmer O, Black M, Hoeh W, Lutz R, Vrijenhoek R (1994) DNA primers for amplification of mitochondrial cytochrome c oxidase subunit I from diverse metazoan invertebrates. Molecular Marine Biology and Biotechnology 3(5), 294-299.
| Google Scholar | PubMed |
Gervais CR, Champion C, Pecl GT (2021) Species on the move around the Australian coastline: A continental‐scale review of climate‐driven species redistribution in marine systems. Global Change Biology 27, 3200-3217.
| Crossref | Google Scholar | PubMed |
Kroh A (2020) Chapter 1. Phylogeny and classification of echinoids. In ‘Sea Urchins: Biology and Ecology’, 4th edn. (Ed. JM Lawrence) pp. 1–17. (Elsevier) 10.1016/B978-0-12-819570-3.00001-9
Lawrence JM, Agatsuma Y (2020) Chapter 37 – Tripneustes. In ‘Developments in Aquaculture and Fisheries Science’. (Ed. JM Lawrence) Vol. 43. pp. 681–703. (Elsevier) 10.1016/B978-0-12-819570-3.00037-8
Leigh JW, Bryant D (2015) POPART: full-feature software for haplotype network construction. Methods in Ecology and Evolution 6, 1110-1116.
| Crossref | Google Scholar |
Lessios HA, Kane J, Robertson DR (2003) Phylogeography of the pantropical sea urchin Tripneustes: contrasting patterns of population structure between oceans. Evolution 57, 2026-2036.
| Crossref | Google Scholar | PubMed |
Meissner M (1891) Bericht über die wissenschaftlichen Leistungen in der Naturgeschichte der Echinodermen während des Jahres 1890. Archiv für Naturgeschichte 57(2), 155-170 Available at https://www.biodiversitylibrary.org/page/6377920 [In German].
| Google Scholar |
Mooi R, Kroh A, Srivastava DK (2014) Phylogenetic re-evaluation of fossil and extant micro-echinoids with revision of Tridium, Cyamidia, and Lenicyamidia (Echinoidea: Clypeasteroida). Zootaxa 3857(4), 501-526.
| Crossref | Google Scholar | PubMed |
Moreira‐Saporiti A, Hoeijmakers D, Reuter H, Msuya FE, Gese K, Teichberg M (2023) Bottom‐up and top‐down control of seagrass overgrazing by the sea urchin Tripneustes gratilla. Marine Ecology 44, e12734.
| Crossref | Google Scholar |
Mos B, Dworjanyn SA (2019) Ready to harvest? Spine colour predicts gonad index and gonad colour rating of a commercially important sea urchin. Aquaculture 505, 510-516.
| Crossref | Google Scholar |
Neilson BJ, Wall CB, Mancini FT, Gewecke CA (2018) Herbivore biocontrol and manual removal successfully reduce invasive macroalgae on coral reefs. PeerJ 6, e5332.
| Crossref | Google Scholar | PubMed |
Nguyen LT, Schmidt HA, von Haeseler A, Minh BQ (2015) IQ-TREE: a fast and effective stochastic algorithm for estimating maximum likelihood phylogenies. Molecular Biology and Evolution 32, 268-274.
| Crossref | Google Scholar | PubMed |
Ridgway KR, Godfrey JS (1997) Seasonal cycle of the East Australian Current. Journal of Geophysical Research: Oceans 102, 22921-22936.
| Crossref | Google Scholar |
Ronquist F, Teslenko M, van der Mark P, Ayres DL, Darling A, Höhna S, Larget B, Liu L, Suchard MA, Huelsenbeck JP (2012) MRBAYES 3.2: efficient Bayesian phylogenetic inference and model choice across a large model space. Systematic Biology 61, 539-542.
| Crossref | Google Scholar | PubMed |
Schumacher CF (1817) ‘Essai d’un nouveau système des habitations des vers testacés.’ (Schultz: Copenghagen, Denmark) Available at http://www.biodiversitylibrary.org/item/81329 [In French and Latin]
Sonnenholzner-Varas JI, Touron N, Orrala MMP (2018) Breeding, larval development, and growth of juveniles of the edible sea urchin Tripneustes depressus: a new target species for aquaculture in Ecuador. Aquaculture 496, 134-145.
| Crossref | Google Scholar |
Stokes RB, Kroh A (2022) Attribution of the taxon name Echinoidea to Schumacher, 1817. Zootaxa 5182(2), 165-174.
| Crossref | Google Scholar | PubMed |
Suthers IM, Young JW, Baird ME, Roughan M, Everett JD, Brassington GB, Byrne M, Condie SA, Hartog JR, Hassler CS, Hobday AJ, Holbrook NJ, Malcolm HA, Oke PR, Thompson PA, Ridgway K (2011) The strengthening East Australian Current, its eddies and biological effects — an introduction and overview. Deep Sea Research Part II: Topical Studies in Oceanography 58, 538-546.
| Crossref | Google Scholar |
Tenison-Woods JE (1878) The echini of Australia. Proceedings of the Linnean Society of New South Wales 2, 145-176 Available at https://www.biodiversitylibrary.org/page/3341652.
| Google Scholar |
Thomas LJ, Liggins L, Banks SC, Beheregaray LB, Liddy M, McCulloch GA, Waters JM, Carter L, Byrne M, Cumming RA, Lamare MD (2021) The population genetic structure of the urchin Centrostephanus rodgersii in New Zealand with links to Australia. Marine Biology 168, 138.
| Crossref | Google Scholar |
Valentine JP, Edgar GJ (2010) Impacts of a population outbreak of the urchin Tripneustes gratilla amongst Lord Howe Island coral communities. Coral Reefs 29, 399-410.
| Crossref | Google Scholar |
Vergés A, Steinberg PD, Hay ME, Poore AGB, Campbell AH, Ballesteros E, Heck KL, Booth DJ, Coleman MA, Feary DA, Figueira W, Langlois T, Marzinelli EM, Mizerek T, Mumby PJ, Nakamura Y, Roughan M, van Sebille E, Gupta AS, Smale DA, Tomas F, Wernberg T, Wilson SK (2014) The tropicalization of temperate marine ecosystems: climate-mediated changes in herbivory and community phase shifts. Proceedings of the Royal Society B: Biological Sciences 281, 20140846.
| Crossref | Google Scholar | PubMed |
Whitelegge T (1889) List of the marine and fresh-water invertebrate fauna of Port Jackson and the neighbourhood. Journal and Proceedings of the Royal Society of New South Wales 23(2), 163-323.
| Crossref | Google Scholar |
Zigler KS, Lessios HA (2003) Evolution of bindin in the pantropical sea urchin Tripneustes: comparisons to bindin of other genera. Molecular Biology and Evolution 20, 220-231.
| Crossref | Google Scholar | PubMed |