Seed row placement relative to the previous crop stubble row can harness systems benefits on water repellent sands
Therese McBeath
A CSIRO Agriculture and Food, Locked Bag 2, Glen Osmond, SA 5064, Australia.
B Agricultural Machinery Research and Design Centre, University of South Australia, Mawson Lakes, SA 5095, Australia.
Abstract
Innovations to improve crop establishment are critical to unlocking the full potential of early sown winter cereal cropping systems.
The aim of this set of experiments was to evaluate the systems benefits of near-row sowing treatments for surface soil water, crop establishment, crop competition with weeds, root disease infection and cereal crop yield on sandy soils in low-rainfall environments.
Six of the 10 site–years evaluated were on water repellent sands with the remainder on sand and loam. A second set of experiments on a water repellent sand evaluated if furrow applied amendments to increase soil fertility in the crop row might generate additional benefits to the near-row sowing effect.
Significant grain yield benefits from near-row placement (up to 30%) were measured in 2 site years and these were associated with crop establishment gains, improved access to furrow soil water storage and weed seed reductions, which occurred in 3 site years. In a water repellent sand, near-row placement combined with extra nutrition input generated an additional grain yield benefit (+0.4 t/ha), as did a one-off 22 cm deep furrow tilling treatment designed to bring deeper soil moisture into the seed zone (+0.4 t/ha).
Near-row placement of crop seeds can provide systems benefits in water repellent sands. The conversion of these benefits to yield benefits occurred in 30% of site-years tested on repellent sand.
Further innovation is required to ensure that these seeder-based innovations generate predictable responses and scaled up evaluation is necessary to fully understand the systems and landscape level benefits of improved crop establishment in soils that often suffer low and uneven crop establishment numbers.
Keywords: crop establishment, edge row, integrated weed management, nutrition, soilborne diseases, soil fertility, sowing, water repellency.
Introduction
The semi-arid Mallee environment of southeastern Australia covers some 2.4 M ha of farming land. It features low and risky rainfall (<350 mm average annual rainfall), with a Mediterranean to Temperate distribution. The landscape features dune swale systems with soil types that vary from deep sands to clay loams within relatively small distances (Whitbread et al. 2008; Rab et al. 2009). The sandy soils of this system often impose constraints on the productivity of agricultural crops, through mechanisms that include, but are not limited to: water repellency, high soil strength, low microbial activity, poor nutrient supply and low pH (Unkovich et al. 2020). These constraints on productivity are important not just for the crop produced on the sands in the paddock, they also have implications for whole of paddock management. Growers are investing significant resources into the lowest yielding parts of the paddock to minimise the impacts of soil erosion, disease incursion, weed competition, low fertility, trafficability and other factors that add undesirable complexity to paddock management.
Intensive cropping in semi-arid Mallee environments can increase productivity compared to traditional cereal–volunteer pasture-based rotations (Sadras 2002; Sadras and Roget 2004; Whitbread et al. 2015). However, its success is increasingly underpinned by early sown systems, which have shown substantial benefits for crop productivity in a range of southern Australian cropping environments (Hunt et al. 2019). In addition to avoiding yield penalties associated with late sowing, earlier seeding also offers management benefits to the typically large-scale cropping programs (Fletcher et al. 2016). A key impediment to achieving success with the system is the reliability of crop establishment in the optimal window. The shift to early sowing times of major crops has occurred during a period of declining autumn rainfall (Hochman et al. 2017). Even and optimum timing of establishment of a crop population is critical for productivity as it synchronises critical crop development phases with environment, and aids whole-of-paddock management including weed management and maintenance of ground cover to reduce erosion potential. Water repellent sands are a particularly challenging environment in which to establish crops with patchy and staggered patterns of crop establishment, a common occurrence (Roper et al. 2015; Unkovich et al. 2020). Current practice is to wait until water repellent sands are sufficiently wet for crop establishment before sowing, which is often after the optimal time of sowing.
In Australia, inter-row sowing is a widespread no-till practice, whereby the seed row is located mid-way between the previous crop stubble rows, providing a wide range of agronomic benefits, including better managing seeder performance in high residue loads (Scott et al. 2010). This practice relies on satellite guidance providing 2 cm tractor autosteering accuracy. In contrast, near-row sowing (also referred to as edge-row sowing) is the practice of accurately sowing alongside the previous year’s crop stubble row with minimum disturbance of that stubble and placing the seed in an near-row position. Harnessing resources from the previous crop stubble row has been examined in the context of sowing grain crops near to pasture and corn drill rows to utilise the fertility benefits of pasture systems (Kane et al. 2014; Sweeney et al. 2018; Hayes et al. 2020; Roper et al. 2022). In the case of water repellent sands, where access to early moisture for emergent seedlings is critical, remnant root systems provide useful pathways for water infiltration and storage in the surface layer of soil (Blackwell 2000; Roper et al. 2015). On-row sowing, where seeds are placed over the previous crop stubble row (Roper et al. 2022), is sometimes used but is limited by excessive stubble disturbance and clumping, which reduces seeder performance, and the uniformity of crop establishment. Achieving on-row and near-row sowing requires 2 cm accuracy autosteer technology, but in all guided-row sowing cases, the position of the seeder relative to the previous crop stubble rows is particularly challenging on slopes where skewing of the towed seeder can cause significant differences between the intended and actual row placement.
The implementation of near-row sowing requires careful thought, both because of the requirement for additional investment in seeder set-up but also due to the potential for net negative effects in environments that are not water repellent. A recent example from corn grown in China showed a negative outcome for water use efficiency when sown on the previous crop row (Sun and Li 2020) while earlier work showed that sowing close to the previous row is a practice that can exacerbate Fusarium pseudograminearum (crown rot) root disease risk (Verrell et al. 2017) but not Rhizoctonia solani AG8 (rhizoctonia) root rot (Davis et al. 2008). The aim of this set of experiments was to evaluate the no-till systems benefits of near-row sowing treatments for soil water access in the furrow seed zone, crop establishment, crop competition with weeds, root disease infection and cereal crop grain yield in low-rainfall sandy soil environments.
Materials and methods
Site description and management
Two experiments were conducted during 2014–2019 at sites in South Australia (Table 1). The average annual rainfall and growing season rainfall (GSR) for the sites are 338 mm and 237 mm at Karoonda and 262 mm and 172 mm at Loxton (Experiment 1), and 381 mm and 270 mm at Lameroo (Experiment 2), respectively. Growing season rainfall at the sites ranged from Decile 1 (2018) to Decile 9 (2016) (Table 1). The experiments evaluated a range of crop and soil responses to the seeding of the crop seed row relative to the previous crop stubble row.
Experiment no. | 1 | 1 | 1 | 1 | 1 | 2 | 2 | 2 | |
---|---|---|---|---|---|---|---|---|---|
Year | 2014 | 2015 | 2015 | 2016 | 2017 | 2017 | 2018 | 2019 | |
Location | Karoonda | Karoonda | Loxton | Karoonda | Karoonda | Lameroo | Lameroo | Lameroo | |
Soil type | Deep sand Clay loam | Deep sand Clay loam | Deep sand | Deep sand | Deep sand | Deep sand | Deep sand | Deep sand | |
Sow date | 30 April 14 May | 27 April 21 May | 28 April 18 May | 31 May | 8 May | 24 May | 24 May | 30 May | |
Row placement | Near-row Inter-row | Near-row Inter-row | Near-row Inter-row | Near-row Inter-row | Near-row Inter-row | Perpendicular to previous stubble row | Near-row Inter-row | Near-row Inter-row | |
Cultivar and crop | Corack wheat | Mace wheat | Mace wheat | Scepter wheat | Scepter wheat | Trojan wheat | Compass barley | Scope barley | |
Growing season rainfall (mm) | 182 | 172 | 174 | 346 | 236 | 246 | 140 | 205 | |
Average growing season rainfall (mm) | 237 | 237 | 172 | 237 | 237 | 270 | 270 | 270 |
Locally adapted wheat (Triticum aestivum) or barley (Hordeum vulgare) cultivars were sown in experimental plots of 20–25 m length. Each plot had six rows set at 0.28 m apart and sown with a narrow opener seeding system. Seeding rates were adjusted for seed size and germination to achieve a target population of approximately 150 plants/m2. The delivery of inter- and near-row treatments involved sowing the new crop row either half-way between the previous crop rows (inter-row) or as close as practical to the previous crop row (near-row) with minimal residue disturbance. This was approximately 2–3 cm from the centre of the previous crop row. In Experiment 2 at Lameroo, near-row sowing was facilitated by a side-banding sowing attachment. This was offset from the furrow opener towards the stubble row for minimal disturbance of standing residue. Optimal agronomy was used to ensure that leaf fungal infection and insect pests were controlled at all sites and no major incursions were recorded.
Plant analysis
Crop plant establishment was recorded approximately 4 and 7 weeks after sowing by counting established plants along 2 × 0.5 m row in six locations in every plot. Weeds were measured for experiments in 2014–2017. Brome grass (Bromus rigidus) and annual ryegrass (Lolium rigidum) weed populations were monitored by using fixed sampling points (0.5 m × 0.56 m in four locations for each soil type) with plant counts at 30, 45 and 90 days after sowing, followed by destructive sampling at maturity to count panicle and seed number/m2. At 7 weeks after sowing, 20 crop plants were collected from each plot, roots were washed, and disease assessment made for total roots and seminal and crown roots separately. Disease incidence is expressed as an overall root disease score using a 0–5 rating scale, 0 = no disease and 5 = all the primary roots infected and severely truncated (>80% roots infected) using a modified method of McDonald and Rovira (1985). In addition, the number of total and infected seminal and crown roots and the length of the internode were also recorded. Additionally, in the 2016 deep sand experiment at Loxton, incidence of Take-All disease was estimated based on an integrated measure of patch incidence (number of rows infected) and severity (severity of incidence on a 0–2 scale) made during grain filling stage. For all experiments seed yield and harvest index (HI) were measured in each plot from 6 × 0.75 m length samples cut by hand at ground level. The samples were dried, threshed, and weighed to determine HI, and a subsample of seed was used to measure protein using a FOSS® NIR (near infra-red) analyser. Whole of plot seed yield was also measured using a small plot harvester and reported at 12.5% w/w moisture.
Soil analysis
Prior to the implementation of sowing strategy treatments, surface (0–0.1 m) soils were analysed for disease risk using the DNA-based method for soil borne diseases (Ophel-Keller et al. 2008). Briefly, 20 soil cores (~12 mm diameter corer) were collected from each plot to give a composite sample for DNA extraction and quantification of the abundance of soilborne pathogens i.e., Rhizoctonia solani AG8, Gaeumannomyces graminis var. tritici (Take-All) and Fusarium pseudograminearum. At the same time a soil test was completed for each replicate, comprising of four cores bulked at each sample depth (0–10 cm, 10–20 cm, 20–40 cm, 40–60 cm, 60–80 cm and 80–100 cm). Bulked samples were oven dried at 40°C for 1 week, sieved (<2 mm) and then analysed for a range of properties that regulate soil physical and chemical fertility. Topsoil samples (0–0.1 m depth) had soil pH measured in a 1:5 soil:water suspension, organic carbon (C) measured according to the Walkley–Black method (Walkley and Black 1934; Rayment and Lyons 2011), Colwell-extractable potassium and phosphorus (Colwell 1963; Rayment and Lyons 2011) and extractable sulfur using 0.25 M potassium chloride at 40°C (Blair et al. 1991). Clay content was measured according to Gee and Or (2002) and the water repellency index (Molarity of Ethanol Droplet) according to King (1981).
Soil water was measured for all experiments, using two segmented soil cores to 1 m depth which were taken in each plot or replicate block depending on treatment design before sowing in each year of the experiment. The cores were divided into depths of 0–0.1 m, 0.1–0.2 m, 0.2–0.4 m, 0.4–0.6 m and 0.8–1.0 m and the samples from the two cores were bulked for analysis. For all sites, sub-samples were weighed, then dried for 48 h at 105°C and re-weighed, to calculate pre-sowing gravimetric water content. Gravimetric water content was converted to volumetric water content using the bulk density, which was measured according to Burk and Dalgliesh (2008). Volumetric water content was converted to mm plant available water using the crop lower limit measurement as described by Burk and Dalgliesh (2008). Another set of sub-samples was immediately dried at 40°C for 10 days. Samples were bulked at increments of 0–0.1 m, 0.1–0.6 m and 0.6–1.0 m and then sieved (<2 mm) for nitrate-N and ammonium-N analysis. Soil nitrate-N and ammonium-N were analysed according to Method 7C2b of Rayment and Lyons (2011).
Experiment 1 (2014–2017) – identifying soil types responsive to near-row sowing near Karoonda and Loxton
For all Experiment 1 sites (Table 1), wheat was sown with 10 kg P/ha and 20 kg N/ha banded below the seed as diammonium phosphate and urea. Further, 33 kg/ha potassium sulfate was applied prior to sowing to eliminate the possibility of potassium or sulfur deficiency and trace element foliar sprays containing Zn, Mn and Cu were applied at early tillering (in a formulation containing ZnSO4.7H2O at 1.9 kg/ha, MnSO4.H2O at 3 kg/ha and CuSO4.5H2O at 0.4 kg/ha in 100 L/ha water).
In 2014, Corack wheat was used to compare the effect of two times of sowing (30 April and 14 May) and two row positions relative to the previous crop row (inter-row and near-row) on a deep sand and clay loam at a site near Karoonda. In 2015, the effects of two times of sowing and two row positions (inter-row and near-row) were tested on a deep sand and clay loam near Karoonda and on a deep sand near Loxton. Mace wheat was sown on 27 April and 21 May near Karoonda, and 28 April and 18 May near Loxton. In 2016, Scepter wheat was sown on 31 May, with two row positions (near-row and inter-row) and in 2017, Scepter wheat was sown on May 8 with two row positions (near-row and inter-row) on a deep sand at Karoonda. All sites in Experiment 1 were sown into a new area with established cereal crop rows, therefore the implementation of the near-row treatment was an isolated event. Trifluralin 480 g/L at 1.5 L/ha was applied prior to, and incorporated by, the sowing operation.
Experiment 2 (2017–2019) – exploiting row placement to access soil water at sowing time on a deep sand near Lameroo
In 2017, crop rows of Trojan wheat were established and then harvested at ~10 cm height, with the residue to be used to test near-row and inter-row treatments in 2018. In 2018 Compass barley was sown on 24 May into the standing wheat residue in the inter-row or near-row position. In 2019 the treatments were repeated on the same plots with seed rows placed adjacent to the 2018 crop stubble row and sown to Scope barley on May 30. Plots were always sown in the same direction to ensure consistency in row placement relative to years prior.
Near-row sowing treatments tested a range of inputs designed to increase the potential benefits of near-row sowing, with these treatments first implemented in 2017, and applied annually thereafter. They included inputs of compost at 200 kg/ha (TailorMade™ Organic Compost), clay (Watheroo™ calcium bentonite) at 200 kg/ha and biochar (Cool Terra Engineered Biocarbon®) at 60 kg/ha, supplied at the base of the furrow (10 cm depth), with an additional treatment that supplied the compost and clay combined at 22 cm furrow depth. The deep furrow treatment was designed to raise lower soil layers up into the seed zone, a process referred to as ‘moisture delving’. In the initial year of 2017 there were indications that the combined moisture delving, clay + compost treatment was producing higher yields than other treatments. In 2018, some additional near-row sown plots with moisture delving, and with and without inputs (of clay and compost), were implemented near to Experiment 2 on the same deep sand.
Annual fertiliser inputs across all treatments for Experiment 2 included 19 kg/ha N, 10 kg/ha P and 6 kg/ha S applied as double superphosphate, and a urea/ammonium sulfate blend. Trace elements were applied as a seed-zone-banded liquid formulation, which supplied 3 kg Mn/ha, 4 kg Zn/ha, 1 kg Cu/ha and 4.5 kg S/ha and contained 4 L/ha of SE14® soil wetter and moisture retainer (SACOA). The high nutrition treatments received an additional 20 and 26 kg N/ha (2018 and 2019, respectively) and 0–6 kg S/ha (2018 and 2019) at sowing. The high nutrition treatment placement was modified in 2019, to allow greater seed:fertiliser vertical separation (approximately 2–3 cm), avoiding the potential for fertiliser toxicity. Trifluralin 480 g/L at 1.5 L/ha was applied prior to sowing and incorporated by sowing.
Statistical analysis
Although treatments varied year to year, all experiments were sown in a randomised complete block design with four replicates per treatment. Analysis of variance was used for all experiments. Assumptions of normality of data distribution and additivity of treatment and replicate effects were tested for each analysis. Where required, some of the data for pathogen levels and disease incidence measurements were log-transformed prior to ANOVA analysis. For significant effects (P ≤ 0.05), Fisher’s protected least significant difference (l.s.d.) was used for multiple comparisons between treatments. Where the interactions were not significant and the factors of row placement only (near- and inter-row) were compared, a paired t-test was utilised. All data was statistically analysed with Genstat V20® Software (VSN International, Hemel Hempstead, UK).
Results
Experimental site key soil properties
Although there was variation in the four site × soil combinations examined (e.g. pH 5.6–8.10, organic carbon 0.30–1.31 (% w/w) and water repellency (very low–severe), all soils had low surface soil clay content (<8% w/w) (Table 2). With respect to nutrition, soils appeared adequate for phosphorus and potassium but some had marginal sulfur concentrations (Table 2) according to a critical value of <2.8 mg/kg as described in Anderson et al. (2013).
Soil properties | Karoonda deep sand | Karoonda clay loam | Loxton deep sand | Lameroo deep sand | |
---|---|---|---|---|---|
pH (1:5 CaCl2) | 6.09 | 6.73 | 8.10 | 5.66 | |
Water repellency (WDPT, secs) | >260 | 6.50 | <5 | 95 | |
Water repellency rating | Severe | Very low | Very low | Moderate–severe | |
Organic carbon (% w/w) | 0.42 | 1.31 | 0.30 | 0.46 | |
Clay content (% w/w) | 3 | 8 | 2 | 4 | |
Colwell Extractable P (mg/kg) | 23 | 37 | 17 | 25 | |
Colwell Extractable K (mg/kg) | 100 | 465 | 179 | 100 | |
KCl Extractable S (mg/kg) | 3.0 | 8.5 | 1.9 | 4.5 |
Values are the average of samples taken from on-row and inter-row positions.
WDPT, water drop penetration test (King 1981).
Experiment 1. Crop benefits from row position
In four of the 10 site–year comparisons near-row sowing resulted in a significant increase in crop establishment (Table 3). In four of the 6 site–year comparisons where near-row sowing did not result in a crop establishment benefit, the plant populations were close to or exceeded 100 plants/m2 (range 96–155 plants/m2) in the control treatments. In no case did near-row sowing have a negative effect on crop establishment (Table 3). Two site–year comparisons showed a significant benefit of near-row sowing for anthesis biomass and grain yield, with a 0.3 t/ha yield increase (cf. 0.7 t/ha control) in 2015 and 0.5 t/ha yield increase (cf. 1.3 t/ha control) in 2018 (note at P = 0.06) (Table 3). Near-row sowing did not significantly reduce yield in any site years.
Establishment (plants/m2) | GS65 biomass (t/ha) | Grain yield (t/ha) | ||||||||
---|---|---|---|---|---|---|---|---|---|---|
Row placement | Inter-row | Near-row | P-value | Inter-row | Near-row | P-value | Inter-row | Near-row | P-value | |
Experiment 1A | ||||||||||
2014 deep sand Karoonda | 59 ± 5 | 70 ± 6 | 0.20 | 3.4 ± 0.26 | 3.7 ± 0.4 | 0.56 | 1.4 ± 0.1 | 1.3 ± 0.1 | 0.57 | |
2014 clay loam Karoonda | 96 ± 5 | 97 ± 6 | 0.91 | 6.8 ± 0.31 | 7.0 ± 0.4 | 0.79 | 2.1 ± 0.3 | 2.1 ± 0.1 | 0.59 | |
2015 deep sand Karoonda | 17b | 69a | <0.001 | 1.0b | 2.7a | <0.001 | 0.7b | 1.0a | 0.04 | |
2015 clay loam Karoonda | 121 ± 15 | 137 ± 3 | 0.08 | 5.7 ± 0.2 | 5.0 ± 0.5 | 0.33 | 2.1 ± 0.2 | 2.2 ± 0.1 | 0.23 | |
2015 deep sand Loxton | 155 ± 3 | 144 ± 8 | 0.14 | 2.9 ± 0.1 | 2.9 ± 0.2 | 0.84 | 1.3 ± 0.1 | 1.3 ± 0.1 | 0.87 | |
2016 deep sand Karoonda | 60b | 94a | 0.004 | 3.4 ± 0.6 | 3.1 ± 0.6 | 0.39 | 1.9 ± 0.3 | 1.9 ± 0.3 | 0.70 | |
2016 deep sand Loxton | 112 ± 4 | 116 ± 6 | 0.37 | 2.5 ± 0.1 | 2.1 ± 0.3 | 0.13 | 1.3 ± 0.1 | 1.2 ± 0.1 | 0.35 | |
2017 deep sand Karoonda | 53b | 80a | <0.001 | 4.3b | 5.4a | 0.04 | 1.9 ± 0.1 | 2.0 ± 0.2 | 0.17 | |
Experiment 2B | ||||||||||
2018 deep sand Lameroo | 50 ± 1.3 | 71 ± 11.0 | 0.14 | 2.1 ± 0.7 | 3.3 ± 0.1 | 0.15 | 1.4 ± 0.12 | 1.8 ± 0.16 | 0.06 | |
2019 deep sand Lameroo | 131a | 114b | 0.007 | 3.6 ± 0.3 | 4.3 ± 0.5 | 0.29 | 1.8 ± 0.1 | 1.8 ± 0.1 | 0.90 |
Where there was a significant difference between treatments, the row placement mean is annotated by a different letter using the l.s.d. calculated at P = 0.05. Where the treatment means did not differ, the standard error is presented.
AExperiment 1 results are averaged across April and May sowing and tested for the effect of row position using a t-test as the row position × time of sowing interactions were not significant.
BExperiment 2 is a comparison of inter-row and near-row treatments only, with further examination of the full treatment list given in Table 6.
Experiment 1. Systems benefits from row position
One of the most consistent observations across the experimental sites was that of a small but significant amount of extra surface (0–10 cm) soil water stored at the near-row position in seven of 10 site–years (0.6–5.4 mm), including those where a yield benefit from near-row sowing was measured (Table 4). There was no advantage for pre-sowing surface soil mineral N according to the row position, except for the inter-row at Loxton in 2015 which had 7.5 kg/ha more mineral N (Table 4). Pre-sowing disease inoculum for Rhizoctonia (RsAG8), the most prevalent root disease at the experimental sites, only differed between crop row positions in 2016 at Loxton and 2017 at Karoonda, where higher inoculum levels were seen in the near-row position (Table 4). However, soils from the near-row position showed higher inoculum levels for Take-All and Fusarium crown rot diseases in most experiments (Table 4).
R. solani AG8A | Take-all (Ggt) | Fusarium pseudograminearum | Plant available water (mm/10 cm) | Mineral nitrogen (kg/ha) | ||||||||||||
---|---|---|---|---|---|---|---|---|---|---|---|---|---|---|---|---|
Season/site | Inter-row | Near-row | P-value | Inter-row | Near-row | P-value | Inter-row | Near-row | P-value | Inter-row | Near-row | P-value | Inter-row | Near-row | P-value | |
2014 deep sand Karoonda | 78 ± 17 | 43 ± 13 | 0.16 | 71.2 ± 4.4 | 67 ± 1.1 | 0.441 | 58.9 ± 2.3 | 66.3 ± 2.5 | 0.87 | 1.7b | 4.1a | 0.02 | 7.8 ± 1.6 | 10.9 ± 1.8 | 0.35 | |
2014 clay loam Karoonda | 376 ± 96 | 195 ± 59 | 0.15 | 59.5 ± 3.0 | 68.4 ± 3.9 | 0.43 | 59.0 ± 4.0 | 52.9 ± 2.8 | 0.98 | 18.2 ± 2.2 | 17.5 ± 2.3 | 0.52 | 27.0 ± 6.8 | 32.5 ± 5.1 | 0.43 | |
2015 deep sand Karoonda | 31 ± 8 | 38 ± 8 | 0.53 | 53.8b | 28.0a | 0.017 | 31.2 ± 4.5 | 23.4 ± 4.5 | 0.29 | 0.5b | 2.4a | <0.001 | 11.3 ± 2.3 | 15.3 ± 2.8 | 0.56 | |
2015 clay loam Karoonda | 46 ± 15 | 56 ± 13 | 0.63 | 35.2 ± 4.6 | 32.5 ± 4.6 | 0.67 | 33.1 ± 4.5 | 33.2 ± 4.5 | 0.99 | 2.3b | 7.5a | <0.001 | 27.1 ± 6.5 | 30.0 ± 6.0 | 0.11 | |
2015 deep sand Loxton | 40 ± 11 | 56 ± 16 | 28.8 ± 8.8 | 18.8 ± 3.5 | 0.24 | 13.0 ± 5.7 | 18 ± 4.6 | 0.47 | 3.8b | 4.4a | 0.03 | 11.3a | 3.8b | 0.03 | ||
2016 deep sand Karoonda | 19 ± 6 | 13 ± 5 | 0.38 | 26.6 ± 6.0 | 27.6 ± 5.1 | 0.88 | 15.9 ± 3.0 | 18.6 ± 3.3 | 0.47 | 2.1 ± 0.6 | 3.8 ± 0.6 | 0.64 | 6.3 ± 3.5 | 5.4 ± 2.1 | 0.70 | |
2016 deep sand Loxton | 207a | 360b | 0.01 | – | – | – | – | – | – | 3.4 ± 1.2 | 4.6 ± 1.6 | 0.55 | 12.8 ± 1.6 | 9.1 ± 2.6 | 0.30 | |
2017 deep sand Karoonda | 8 ± 2 | 151 ± 96 | 7.3b | 32.6a | 0.028 | 14.1b | 40.6a | 0.028 | 1.3b | 2.7a | <0.001 | 21.2 ± 2.9 | 24.2 ± 3.6 | 0.54 | ||
2018 deep sand Lameroo | 125 ± 39 | 100 ± 19 | 0.70 | 1.4b | 4.6a | <0.001 | 12.0 ± 2.4 | 11.2 ± 2.0 | 0.81 | |||||||
2019 deep sand Lameroo | 49 ± 0.4 | 39 ± 0.4 | 0.60 | 2.7b | 8.1a | <0.001 | 12.7 ± 1.9 | 9.2 ± 1.2 | 0.17 |
Where there was a significant difference between treatments, the row placement mean is annotated by a different letter using the l.s.d. calculated at P = 0.05. Where the treatment means did not differ, the standard error is presented.
For 2014–2015 sites, results are averaged across April and May sowing and tested for the effect of row position using a t-test as the row position × time of sowing interactions were not significant.
Ggt, Gaeumannomyces graminis var. tritici.
AAbundance of soilborne pathogens measured using a pathogen specific RT-PCR method (reverse transcription polymerase chain reaction) and expressed as pg of DNA per gram soil.
Within-season measurements indicated some important crop competition benefits for the near-row sown treatments. Where brome grass was the prevalent grass weed (e.g. deep sand at Karoonda), near-row sowing consistently resulted in a lower brome grass seed set, except in 2016, despite near-row sowing generating a higher crop density (Tables 3, 5). Results from the in-season assessment of root disease incidence indicated that rhizoctonia root rot was the most common disease observed in all experiments, but increased disease incidence was not commonly observed when crops were sown near to the previous cereal crop row (Table 5). Exceptions were 2015 and 2017 Experiment 1 crops at Karoonda, where near-row plants showed significantly higher disease incidence (Table 5). Despite significantly higher establishment and G65 biomass at Karoonda in 2017, there was no benefit in grain yield between the two crop row placement treatments. This could be partly attributed to the significantly higher root disease incidence both for the seminal and crown roots. In the 2016 deep sand experiment at Loxton, the incidence of Take-All disease measured as patch score was significantly higher in the near-row treatment compared to under inter-row sowing strategy (Table 5).
Disease scoreA | % Crown roots infected | % Seminal roots infected | Brome seeds (seeds/m2) | ||||||||||
---|---|---|---|---|---|---|---|---|---|---|---|---|---|
Inter-row | Near-row | P-value | Inter-row | Near-row | P-value | Inter-row | Near-row | P-value | Inter-row | Near-row | P-value | ||
2014 deep sand Karoonda | 3.06 ± 0.09 | 3.15 ± 0.02 | 0.85 | 71.2 ± 4.4 | 67 ± 1.1 | 0.441 | 58.9 ± 2.3 | 66.3 ± 2.5 | 0.87 | 1859a | 389b | 0.05 | |
2014 clay loam Karoonda | 2.70 ± 0.15 | 2.65 ± 0.15 | 0.69 | 59.5 ± 3.0 | 68.4 ± 3.9 | 0.43 | 59.0 ± 4.0 | 52.9 ± 2.8 | 0.98 | NAB | NA | ||
2015 deep sand Karoonda | 2.24b | 1.65a | 0.03 | 53.8b | 28a | 0.017 | 31.2 ± 4.5 | 23.4 ± 4.5 | 0.29 | 8844a | 3271b | 0.02 | |
2015 clay loam Karoonda | 1.97 ± 0.12 | 1.91 ± 0.12 | 0.77 | 35.2 ± 4.6 | 32.5 ± 4.6 | 0.67 | 33.1 ± 4.5 | 33.2 ± 4.5 | 0.99 | NA | NA | ||
2015 deep sand Loxton | 1.71 ± 0.44 | 1.30 ± 0.17 | 0.97 | 28.8 ± 8.8 | 18.8 ± 3.5 | 0.24 | 13.0 ± 5.7 | 18 ± 4.6 | 0.47 | NA | NA | ||
2016 deep sand KaroondaB | 1.20 ± 0.22 | 1.23 ± 0.14 | 0.91 | 26.6 ± 6.0 | 27.6 ± 5.1 | 0.88 | 15.9 ± 3.0 | 18.6 ± 3.3 | 0.47 | 2932 ± 275 | 2312 ± 137 | 0.60 | |
2016 deep sand LoxtonC | 1.5b | 3.5a | 0.05 | – | – | – | – | – | – | NA | NA | ||
2017 deep sand Karoonda | 0.6b | 1.4a | 0.034 | 7.3b | 32.6a | 0.028 | 14.1b | 40.6a | 0.028 | 4650a | 1469b | 0.05 |
Where there was a significant difference between treatments, the row placement mean is annotated by a different letter using the l.s.d. calculated at P = 0.05. Where the treatment means did not differ, the standard error is presented.
ARoot disease score was made using a 0–5 rating scale (McDonald and Rovira 1985).
BTake-All – %incidence – 24 ± 6.5 and 35 ± −4.4 for inter-row and near-row samples, respectively.
CTake-All patch score measured during grain filling stage.
Experiment 2. Seeder system innovations to better harness potential benefits of the near-row system
A 3-year evaluation (with a set-up phase in year 1) of combining row placement treatments with extra amendments and tillage generated some interesting benefits over and above that of row placement alone. The row placement alone generated a significant yield benefit in one of the two seasons (2018) (Table 6). Despite a significant difference in anthesis biomass and similar HI in 2019 (average 0.53 ± s.e. 0.02), there were similar yields, suggesting differences in crop growth and stress during grain fill. Unlike Experiment 1, this experiment had the near-row treatments implemented in two consecutive seasons.
Treatment | Establishment (plants/m2) | GS65 Biomass (t/ha) | Grain Yield (t/ha) | |
---|---|---|---|---|
2018 | ||||
Inter-row | 50ab | 2.1 ± 0.7 | 1.4d | |
Near-row | 71ab | 3.3 ± 0.2 | 1.8c | |
Inter-row with high nutrition | 49b | 1.6 ± 0.2 | 1.4d | |
Near-row with high nutrition | 49ab | 3.6 ± 0.7 | 1.8c | |
Near-row with clay | 69ab | 2.8 ± 0.3 | 1.8c | |
Near-row with compost | 74a | 3.0 ± 0.8 | 1.9bc | |
Near-row with high nutrition and biochar | 73ab | 3.4 ± 0.7 | 2.2ab | |
Near-row with clay and compost | 63ab | 3.1 ± 0.6 | 1.8c | |
Near-row with moisture delving, clay and compost | 71ab | 3.0 ± 0.5 | 2.2a | |
P-value | 0.003 | 0.31 | <0.001 | |
2019 | ||||
Inter-row | 132a | 3.6b | 1.8 ± 0.1 | |
Near-row | 114abc | 4.3ab | 1.8 ± 0.1 | |
Inter-row with high nutrition | 126ab | 4.9ab | 2.1 ± 0.1 | |
Near-row with high nutrition | 108bc | 5.3a | 2.1 ± 0.2 | |
Near-row with clay | 122abc | 4.5ab | 1.9 ± 0.2 | |
Near-row with compost | 121abc | 4.2ab | 2.1 ± 0.1 | |
Near-row with high nutrition and biochar | 102c | 5.0ab | 2.3 ± 0.2 | |
Near-row with clay and compost | 118abc | 4.3ab | 2.0 ± 0.2 | |
Near-row with moisture delving, clay and compost | 101c | 3.8ab | 2.0 ± 0.2 | |
P-value | <0.001 | 0.05 | 0.15 |
Where there was a significant difference between treatments, the row placement mean is annotated by a different letter using the l.s.d. calculated at P = 0.05. Where the treatment means did not differ, the standard error is presented.
Measuring the benefits of higher nutrition alone combined with row placement may have been impacted by fertiliser toxicity in 2018, as indicated by lower plant counts in the high nutrition treatments (Table 6). In the subsequent year, when there was greater seed:fertiliser separation, the near-row treatment combined with high nutrition was the only treatment to generate a yield higher than the inter-row placement alone. Given the 1.7 t/ha average biomass difference between these treatments in 2019, but lack of a statistically significant treatment difference, the biomass production across replicates was clearly highly variable (Table 6).
In the first year, treatments that generated benefit over and above row placement were the near-row placement combined with high nutrition and biochar, and a tillage (moisture delving) treatment that was combined with clay and compost input. The components of the near-row sowing with moisture delving, clay and compost treatments were evaluated separately in 2018. It was clear that the yield benefit was derived from the moisture delving, deep furrow tilling pass (+0.3 t/ha) and not from the clay and compost amendment inputs into the furrow (Table 7). Given the observation of the potential for fertiliser toxicity with the high nutrition treatment in 2018, it is possible that the use of biochar with high nutrition mediated the toxicity and allowed for a nutrition benefit (Table 6).
Treatment | Establishment (plants/m2) | GS65 Biomass (t/ha) | Grain Yield (t/ha) | |
---|---|---|---|---|
Near-row | 91 ± 3 | 2.5 ± 0.1 | 1.6b | |
Near-row with clay and compost | 86 ± 2 | 2.5 ± 0.3 | 1.5b | |
Near-row with moisture delving | 87 ± 3 | 3.1 ± 0.1 | 1.9a | |
Near-row with moisture delving, clay and compost | 85 ± 2 | 3.1 ± 0.3 | 1.9a | |
P-value | 0.4 | 0.17 | <0.001 |
Where there was a significant difference between treatments, the row placement mean is annotated by a different letter using the l.s.d. calculated at P = 0.05. Where the treatment means did not differ, the standard error is presented.
Discussion
Large areas of the Southern Australian cropping zone are experiencing the dual challenges of a later median break of season (Flohr et al. 2021) and difficult to wet sandy soils (Unkovich et al. 2020). Innovation at seeding time to increase the reliability of crop establishment is required to capture the benefits of an early sown cropping system (Hunt et al. 2019). Currently growers are waiting to sow later in the season to allow sufficient time for repellent soil to wet up, risking a loss in grain yield potential. Near-row sowing is one such innovation, where the aim is to utilise small amounts of extra water that infiltrate and store in the vicinity of the root zone of the previous crop stubble row to support more reliable and rapid establishment (Roper et al. 2015, 2022; Blackwell 2020).
Despite the challenge of variable plant numbers that is inherent for the soil types tested, there were crop establishment benefits in 30% of cases. Critically, the seeding-based treatments generated viable plant populations that were in the range of 59–80 plants/m2, compared with the inter-row sown baseline control of just 17–50 plants/m2, a population of <50 plants/m2 can create both significant management and yield disadvantage (Table 3) (Fischer et al. 2019). These benefits for crop establishment align with those measured in Western Australia by Roper et al. (2022). From a practical perspective, the value of increased and more even crop establishment in the most challenging part of the paddock landscape offers several benefits for whole of paddock management including erosion management, reduced pest and weed incursions, increased simplicity in nutrient management and increased amenity to controlled or reduced traffic.
We measured cereal yield benefits with near-row sowing in 20% of our site–years measured (Table 3), and it is clear that these benefits are more likely on water repellent sands as was the case in work in Western Australia (Roper et al. 2022). Grain yield responses to plant density are variable (Fischer et al. 2019). Early sown crops are often more responsive to winter growing conditions and have more time to compensate, recover and tiller to negate any significant changes in plant density compared to later emerged crops. One explanation that has been given for a lack of yield response to plant density is that reducing plant density can reduce early biomass accumulation and improve HI in early established cultivars of winter wheats (Kirkegaard et al. 2014). However, the experiments presented here achieved consistent HI across a range of seasons and sites (data not shown), with no responses resembling ‘haying off’ to suggest high plant density and or early N led to excessive vegetative growth and water use (van Herwaarden et al. 1998). Porker et al. (2020) found little evidence of yield penalty at low plant densities (e.g. 30–50 plants/m2) of early established winter wheat due to the capacity of wheats to tiller and negate any significant changes in plant density. These thresholds differ compared to the shorter vegetative period of fast developing spring wheats used in our study, where there was a significant yield benefit to near-row sowing in situations where the control had less than 50 plants/m2. However, with the limited scenarios in which this benefit was measured, the focus on early vigour and the interaction with other systems factors remains the priority for this study.
Whole of paddock yields are dependent on the establishment of an even and adequate population of seedlings across the landscape (Finch-Savage and Bassel 2016). Seedling vigour, and therefore crop establishment, is often considered a genetic and seed quality problem. Although there are exciting innovations to increase seedling vigour (e.g. long coleoptile wheats, screening for larger seed size) in dry and challenging environments (Zhao et al. 2022), environment x management systems that facilitate the best possible implementation of these genetic options will ensure that these benefits have the broadest possible impact. The more compelling arguments for the implementation of near-row sowing perhaps relate to improved crop competition, while maintaining the integrity of standing stubble residue, and whole-of-paddock management benefits, especially for higher risk crops like canola.
Crop competition is a desirable attribute for improved weed management and a critical element of any integrated weed management program (Jha et al. 2017). Previous research has shown that doubling plant density is effective against ryegrass, particularly in low-rainfall systems where herbicide options are limited by cost and suitability for sandy soils (Lemerle et al. 2004). Most research in this area has focused on the use of row spacing, sowing rate, row orientation and cultivar selection as tools to increase the crop density (Jha et al. 2017), with little previous attention paid to the role of row placement relative to previous rows for the establishment of a more competitive and even crop stand. Brome grass seed set with near-row sowing was found to be 20–37% of that produced with inter-row sowing (Table 5). In addition to crop competition, soil throw by the furrow opener may influence brome seed set in the different systems. The soil throw can have the effect of displacing and burying surface weed seeds onto a ‘moist’ stubble row zone (under inter-row sowing), or alternatively onto a dry inter-row zone (under near-row sowing). Weeds like brome grass present significant challenges in low-rainfall, water repellent systems with stubble retention, due to difficulty in controlling their numbers in low-rainfall, low-input cost systems (Kleemann and Gill 2006; Malik et al. 2015). The combination of soil, climate and brome grass weed attributes means that herbicide efficacy is constantly challenged (Boutsalis et al. 2012; Owen et al. 2015) and often the most efficacious herbicide options are very expensive (Preston et al. 2019). While ryegrass was the other grass weed present at the sites, the population was very low (<5 plants/m2, data not shown), so the effect of row placement and crop competition on ryegrass was not tested here. Clearly, further testing of row placement for crop competition benefits is warranted.
A major concern when implementing near-row sowing in cereal-on-cereal rotations is the potential for increased root disease burden. This has been observed to be a particular issue for crown rot in other studies (Verrell et al. 2017), with a lesser effect in the presence of Rhizoctonia (Davis et al. 2008). The sites of this study had Rhizoctonia, Take-All and crown rot inoculum but root disease scoring indicated that the major disease pressure was derived from Rhizoctonia root rot with significant incidence of other diseases such as Take-All only observed in specific experiments (e.g. Loxton, 2016). Overall, this presented as a limited negative effect of near-row sowing on disease infection. Rhizoctonia root rot is known to be an opportunistic disease with greater impact in soils where background soil microbial activity is low and in situations of crop stress (e.g. moisture and temperature stress) (Cook 1973; Gill et al. 2001). The benefits of increased crop establishment with small amounts of extra crop row water are likely to have improved seedling vigour, allowing the plant population to overcome the Rhizoctonia inoculum load and have a lower infection rate.
The exception to the general trend of lack of significant biotic stress is seen in the 2017 deep sand experiment at Karoonda. In this crop, near-row soils contained higher pathogen inoculum levels for all three diseases and showed significantly higher disease incidence compared to that in the inter-row treatment, which would have contributed to the lack of benefits in grain yield despite higher crop establishment and shoot biomass. Soilborne root diseases observed in this study, such as Take-All and Fusarium crown rot, are known to restrict water and nutrient uptake later in the season thereby affecting grain filling and contributing to the lack of grain yield benefits in this crop (Hornby and Bateman 1998; Alahmad et al. 2018).
Fertility and the adequate supply of nutrition, in particular nitrogen, to cereal crops remains a significant challenge in water repellent sands (McBeath et al. 2019; Unkovich et al. 2020). In Experiment 2 nutrient and biochar inputs were combined to boost seed row fertility. This treatment was intended to increase the fertility benefits over and above the near-row fertility benefits observed by Kane et al. (2014) and Hayes et al. (2020), similar to the approach of Sweeney et al. (2018). Treatments with extra nitrogen (biochar plus high nutrition, 2018 and high nutrition, 2019) generated yield benefit in Year 1 but only a biomass effect in Year 2. Fertiliser toxicity is a risk in sandy soil types, even at relatively low fertiliser rates (Dockerill 2019). The response to the biochar plus high nutrition may relate to the biochar overcoming the effects of fertiliser toxicity, as biochar is known to slow the release of nutrient from fertilisers (Wang et al. 2022). It is unclear why the high nutrition alone generated significantly more biomass, whereas the high nutrition plus biochar treatment did not generate a significant benefit in the second year (Table 6). However, the biomass production across plots was highly variable with an l.s.d. of 1.0 t/ha. It is difficult to determine whether the lesser response in the second year also relates to a seasonal effect where water repellence did not express as much as indicated by all plant densities exceeding 100 plants/m2, despite rainfall being similar but perhaps slightly higher through June in 2019 (Fig. 1). Alternatively, there may have been implementation issues or diminishing returns that arise because it was the third consecutive season of the near-row system (with the first year a set-up season).
Monthly rainfall for each of the site–year combinations at (a) Karoonda, (b) Loxton and (c) Lameroo.
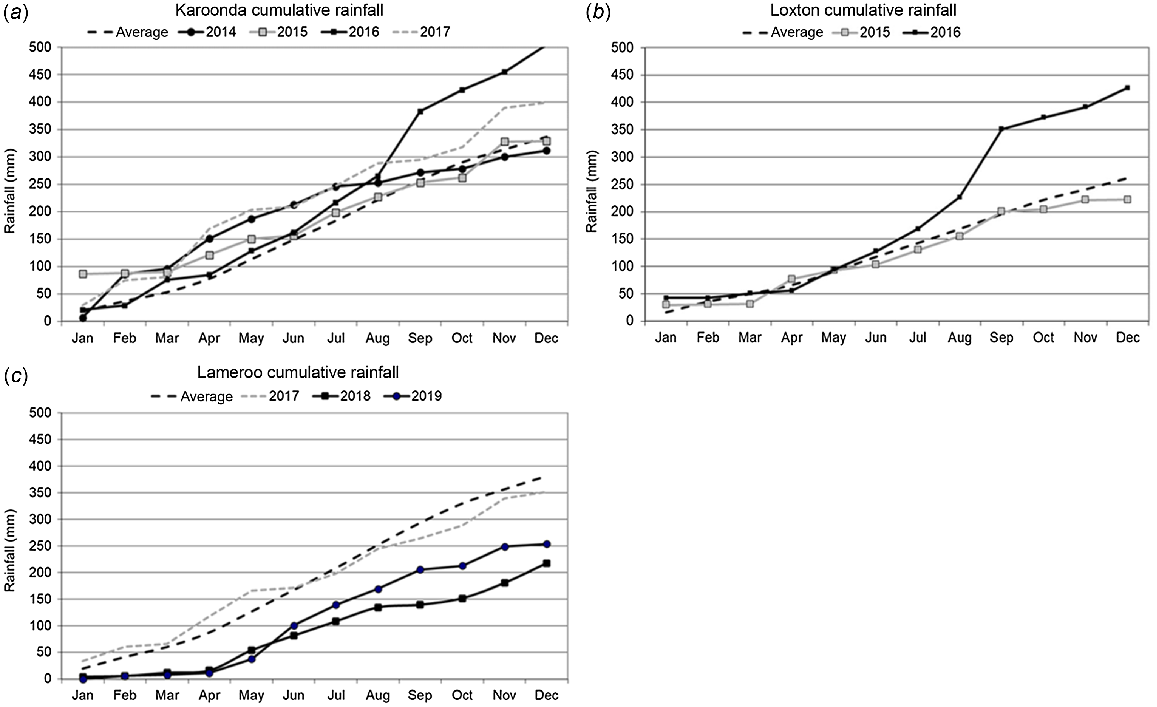
An alternative to seeder-based innovations to improve productivity on water repellent sands is the use of soil amelioration techniques largely based on targeted tillage operations which either: shatter the sandy soil profile (e.g. deep ripping/tillage), translocate surface layers to depth (e.g. inclusion ripping), invert the profile (e.g. mouldboard ploughing), lift deeper layers to the surface (delving) or mix (e.g. rotary spading) the soil profile. Amelioration of sandy soils to depths of 0.4–0.6 m has gained rapid adoption throughout the Western and Southern cropping regions of Australia in the last decade with yield benefits in the order of 20–100% (Davies et al. 2019; Hall et al. 2020; McBeath et al. 2022; Unkovich et al. 2023). However, a persistent issue with many of these amelioration-based techniques is the high erosion risk and resultant crop establishment difficulties, sometimes failures, in the season following the amelioration pass (Unkovich et al. 2020). Moisture delving using the seeding pass was implemented as an intermediate deep furrow tilling treatment that may help to overcome some of the early water supply and crop establishment constraints of a water repellent and potentially compacted sand without the erosion risk and cost of an amelioration pass. This moisture delving plus clay and compost treatment generated a 0.4 t/ha benefit over and above the near-row treatment and 0.8 t/ha more than the inter-row baseline control (Table 6). An adjacent experiment to split out the treatment factors revealed that the tillage-based moisture delving alone generated the 0.4 t/ha benefit above the near-row treatment and the clay and compost did not generate additional benefit (Table 7). The lack of benefit in the second growing season (2019) reveals work is still to be done to understand the environment by management scenarios where innovations in seeding management will offer consistent benefits.
Conclusions
Using near-row sowing meant that crop densities above 50 plants/m2 were achieved in 100% of site–season years. The sub-optimal establishment of inter-row sown crops that was experienced in 30% of site-years was avoided using near-row sowing. Whereas near-row sowing is likely to reduce the risk of very poor establishment on these soils and thereby reduce risk of erosion and yield loss due to poor establishment, there was no evidence that it reduced yield in any year. However, monitoring of potential for greater disease risks is needed. Near-row sowing has additional management challenges and requires specialist equipment compared to inter-row seeding. For growers, it becomes a question of whether reducing the risk and costs of very poor establishment in a minority of years outweighs the overall costs of implementation, including the majority of years where inter-row seeding will provide adequate establishment and no yield advantages are experienced.
Acknowledgements
The authors thank the landholders who hosted the paddock trials and to Willie Shoobridge, Stasia Kroker, Dean Thiele and Marcus Hicks for technical delivery of experiments. Special thanks to Murray Unkovich and Kenton Porker for their comments on an earlier version of this manuscript.
References
Alahmad S, Simpfendorfer S, Bentley AR, Hickey LT (2018) Crown rot of wheat in Australia: Fusarium pseudograminearum taxonomy, population biology and disease management. Australasian Plant Pathology 47, 285-299.
| Crossref | Google Scholar |
Anderson GC, Peverill KI, Brennan RF (2013) Soil sulfur – crop response calibration relationships and criteria for field crops grown in Australia. Crop & Pasture Science 64, 523-530.
| Crossref | Google Scholar |
Blackwell PS (2000) Management of water repellency in Australia, and risks associated with preferential flow, pesticide concentration and leaching. Journal of Hydrology 231–232, 384-395.
| Crossref | Google Scholar |
Blair GJ, Chinoim N, Lefroy RDB, Anderson GC, Crocker GJ (1991) A soil sulfur test for pastures and crops. Australian Journal of Soil Research 29, 619-626.
| Crossref | Google Scholar |
Boutsalis P, Preston C, Gill G (2012) Herbicide cross resistance in Bromus diandrus and B. rigidus populations across southeastern Australia. In ‘Developing solutions to evolving weed problems, Proceedings 18th Australasian Weeds Conference, 8–11 October 2012, Melbourne, Vic.’. (Ed. V Eldershaw) pp. 224–228. (Weed Science Society of Victoria Inc.)
Colwell JD (1963) The estimation of the phosphorus fertilizer requirements of wheat in southern New South Wales by soil analysis. Australian Journal of Experimental Agriculture 3, 190-197.
| Crossref | Google Scholar |
Cook RJ (1973) Influence of low plant and soil water potentials on diseases caused by soilborne fungi. Phytopathology 63, 451-457.
| Google Scholar |
Davis RA, Huggins D, Cook RJ, Paulitz TC (2008) Can placement of seed away from relic stubble limit Rhizoctonia root rot in direct-seeded wheat? Soil and Tillage Research 101, 37-43.
| Crossref | Google Scholar |
Finch-Savage WE, Bassel GW (2016) Seed vigour and crop establishment: extending performance beyond adaptation. Journal of Experimental Botany 67, 567-591.
| Crossref | Google Scholar |
Fischer RA, Moreno Ramos OH, Ortiz Monasterio I, Sayre KD (2019) Yield response to plant density, row spacing and raised beds in low latitude spring wheat with ample soil resources: an update. Field Crops Research 232, 95-105.
| Crossref | Google Scholar |
Fletcher A, Lawes R, Weeks C (2016) Crop area increases drive earlier and dry sowing in Western Australia: implications for farming systems. Crop & Pasture Science 67, 1268-1280.
| Crossref | Google Scholar |
Flohr BM, Ouzman J, McBeath TM, Rebetzke GJ, Kirkegaard JA, Llewellyn RS (2021) Redefining the link between rainfall and crop establishment in dryland cropping systems. Agricultural Systems 190, 103105.
| Crossref | Google Scholar |
Gill JS, Sivasithamparam K, Smettem KRJ (2001) Soil moisture affects disease severity and colonisation of wheat roots by Rhizoctonia solani AG-8. Soil Biology and Biochemistry 33, 1363-1370.
| Crossref | Google Scholar |
Hall DJM, Davies SL, Bell RW, Edwards TJ (2020) Soil management systems to overcome multiple constraints for dryland crops on deep sands in a water limited environment on the south coast of Western Australia. Agronomy 10, 1881.
| Crossref | Google Scholar |
Hayes RC, Li GD, Rawnsley RP, Pembleton KG, Corkrey R, Peoples MB (2020) The legacy of pasture drill rows on soil chemical characteristics and subsequent wheat production. Plant and Soil 455, 319-337.
| Crossref | Google Scholar |
Hochman Z, Gobbett DL, Horan H (2017) Climate trends account for stalled wheat yields in Australia since 1990. Global Change Biology 23, 2071-2081.
| Crossref | Google Scholar | PubMed |
Hunt JR, Lilley JM, Trevaskis B, Flohr BM, Peake A, Fletcher A, Zwart AB, Gobbett D, Kirkegaard JA (2019) Early sowing systems can boost Australian wheat yields despite recent climate change. Nature Climate Change 9, 244-247.
| Crossref | Google Scholar |
Jha P, Kumar V, Godara RK, Chauhan BS (2017) Weed management using crop competition in the United States: a review. Crop Protection 95, 31-37.
| Crossref | Google Scholar |
Kane DA, Snapp SS, Davis AS (2014) Ridge tillage concentrates potentially mineralizable soil nitrogen, facilitating maize nitrogen uptake. Soil Science Society of America Journal 79, 81-88.
| Crossref | Google Scholar |
King PM (1981) Comparison of methods for measuring severity of water repellence of sandy soils and assessment of some factors that affect its measurement. Australian Journal of Soil Research 19, 275-285.
| Crossref | Google Scholar |
Kirkegaard JA, Hunt JR, McBeath TM, Lilley JM, Moore A, Verburg K, Robertson M, Oliver Y, Ward PR, Milroy S, Whitbread AM (2014) Improving water productivity in the Australian grains industry – a nationally coordinated approach. Crop & Pasture Science 65, 583-601.
| Crossref | Google Scholar |
Kleemann SGL, Gill GS (2006) Differences in the distribution and seed germination behaviour of populations of Bromus rigidus and Bromus diandrus in South Australia: adaptations to habitat and implications for weed management. Australian Journal of Agricultural Research 57, 213-219.
| Google Scholar |
Lemerle D, Cousens RD, Gill GS, Peltzer SJ, Moerkerk M, Murphy CE, Collins D, Cullis BR (2004) Reliability of higher seeding rates of wheat for increased competitiveness with weeds in low rainfall environments. Journal of Agricultural Science 142, 395-409.
| Google Scholar |
Malik RS, Seymour M, French RJ, Kirkegaard JA, Lawes RA, Liebig MA (2015) Dynamic crop sequencing in Western Australian cropping systems. Crop & Pasture Science 66, 594-609.
| Crossref | Google Scholar |
McBeath TM, Gupta VVSR, Llewellyn RS, Mason SD, Davoren CW, Correll RL, Jones B, Whitbread AM (2019) Combined application of nitrogen and phosphorus to enhance nitrogen use efficiency and close the wheat yield gap on varying soils in semi-arid conditions. Journal of Agronomy and Crop Science 205, 635-646.
| Crossref | Google Scholar |
McBeath T, Unkovich M, Wilhelm N, Fraser M, Moodie M, Trengove S, Desbiolles J, Suanders C, Whitworth R, da Silva RC, Llewellyn R, Macdonald L (2022) Targeted amelioration of constraints in deep sands to maximise crop water use. In ‘Solutions for complex problems. Proceedings of the 20th Australian Society of Agronomy Conference. Toowoomba, Qld.’ (Ed. LW Bell). (Australian Society of Agronomy)
McDonald HJ, Rovira AD (1985) Development of inoculation technique for Rhizoctonia solani and its application to screening cereal cultivars for resistance. In ‘Ecology and management of soil-borne plant pathogens.’ (Eds CA Parker, AD Rovira, KJ Moore, PTW Wong, JF Kollmorgen) pp. 174–176. (American Phytopathological Society: St Paul, MN, USA)
Ophel-Keller K, McKay A, Hartley D, Herdina , Curran J (2008) Development of a routine DNA-based testing service for soilborne diseases in Australia. Australasian Plant Pathology 37, 243-253.
| Crossref | Google Scholar |
Owen MJ, Martinez NJ, Powles SB (2015) Herbicide resistance in Bromus and Hordeum spp. in the Western Australian grain belt. Crop & Pasture Science 66, 466-473.
| Crossref | Google Scholar |
Porker K, Straight M, Hunt JR (2020) Evaluation of G × E × M interactions to increase harvest index and yield of early sown wheat. Frontiers in Plant Science 11, 994.
| Crossref | Google Scholar | PubMed |
Preston C, Boutsalis P, Adu-Yeboah P, Gill G (2019) Sustaining our herbicides into the future. Grains Research and Development Corporation (GRDC). Available at https://grdc.com.au/resources-and-publications/grdc-update-papers/tab-content/grdc-update-papers/2019/07/sustaining-our-herbicides-into-the-future
Rab MA, Fisher PD, Armstrong RD, Abuzar M, Robinson NJ, Chandra S (2009) Advances in precision agriculture in south-eastern Australia. IV. Spatial variability in plant-available water capacity of soil and its relationship with yield in site-specific management zones. Crop & Pasture Science 60, 885-900.
| Crossref | Google Scholar |
Roper MM, Davies SL, Blackwell PS, Hall DJM, Bakker DM, Jongepier R, Ward PR (2015) Management options for water-repellent soils in Australian dryland agriculture. Soil Research 53, 786-806.
| Crossref | Google Scholar |
Roper MM, Ward PR, Betti G, Davies SL, Wilhelm N, Kerr R, Micin SF, Blacker T (2022) Seeding next to previous year’s crop row (near-row sowing) can increase grain yields on water repellent soils. Soil Research 60, 360-372.
| Crossref | Google Scholar |
Sadras V (2002) Interaction between rainfall and nitrogen fertilisation of wheat in environments prone to terminal drought: economic and environmental risk analysis. Field Crops Research 77, 201-215.
| Crossref | Google Scholar |
Sadras VO, Roget DK (2004) Production and environmental aspects of cropping intensification in a semiarid environment of Southeastern Australia. Agronomy Journal 96, 236-246.
| Crossref | Google Scholar |
Sun T, Li Z (2020) Row placement affects yield and water use efficiency of continuous corn. Agronomy Journal 112, 2624-2635.
| Crossref | Google Scholar |
Sweeney DW, Ruiz-Diaz D, Jardine DJ (2018) Nitrogen management and uptake by corn on no-till and ridge-till claypan soil. Agrosystems, Geosciences & Environment 1, 1-6.
| Crossref | Google Scholar |
Unkovich M, McBeath T, Llewellyn R, Hall J, Gupta VVSR, Macdonald LM (2020) Challenges and opportunities for grain farming on sandy soils of semi-arid south and south-eastern Australia. Soil Research 58, 323-334.
| Crossref | Google Scholar |
Unkovich M, McBeath T, Moodie M, Macdonald LM (2023) High soil strength and cereal crop responses to deeper tillage on sandy soils in a semi-arid environment. Field Crops Research 291, 108792.
| Crossref | Google Scholar |
van Herwaarden AFv, Farguhar GD, Angus JF, Richards RA, Howe GN (1998) ‘Haying-off’, the negative grain yield response of dryland wheat to nitrogen fertiliser I. Biomass, grain yield and water use. Australian Journal of Agricultural Research 49, 1067-1081.
| Crossref | Google Scholar |
Verrell AG, Simpfendorfer S, Moore KJ (2017) Effect of row placement, stubble management and ground engaging tool on crown rot and grain yield in a no-till continuous wheat sequence. Soil and Tillage Research 165, 16-22.
| Crossref | Google Scholar |
Walkley A, Black IA (1934) An examination of the Degtjareff method for determining soil organic matter, and a proposed modification of the chromic acid titration method. Soil Science 37, 29-38.
| Crossref | Google Scholar |
Wang C, Luo D, Zhang X, Huang R, Cao Y, Liu G, Zhang Y, Wang H (2022) Biochar-based slow-release of fertilizers for sustainable agriculture: a mini review. Environmental Science & Technology 10, 100167.
| Crossref | Google Scholar |
Whitbread A, Llewellyn R, Gobbett DL, Davoren B (2008) EM38 and crop-soil simulation modelling can identify differences in potential crop performance on typical soil zones in the Mallee. In ‘Proceedings of the Australian Agronomy Conference, Adelaide’. (Ed. M Unkovich). (Australian Society of Agronomy)
Whitbread AM, Davoren CW, Gupta VVSR, Llewellyn R, Roget DK (2015) Long-term cropping system studies support intensive and responsive cropping systems in the low-rainfall Australian Mallee. Crop & Pasture Science 66, 553-565.
| Crossref | Google Scholar |
Zhao Z, Wang E, Kirkegaard JA, Rebetzk GJ (2022) Novel wheat varieties facilitate deep sowing to beat the heat of changing climates. Nature Climate Change 12, 291-296.
| Crossref | Google Scholar |