‘There must be something in the soil our little plants need’: exploring patterns of potential mycorrhizal associations in the flora of the Sunshine Coast heathlands, Queensland, Australia
Hilary Pearl

A
Abstract
The first general overview of mycorrhizal functional groups associated with the flora of the Queensland Sunshine Coast heathlands, a community of low phylogenetic diversity, is provided in this Short Communication. Broad proportions of plant species associated with mycorrhizal and non-mycorrhizal functional groups in the heathlands were compared with those in the surrounding rainforest flora, and across the heath strata. This overview suggests that a greater diversity of mycorrhizal strategies and an increased number of plant genera with non-mycorrhizal associations are found in the heathlands, with proportions varying among the strata. These associations may be facilitating the coexistence of plant species and increasing phylogenetic dispersion.
Keywords: community assembly, diversity, facilitation, heathlands, mycorrhiza, phylogenetic dispersion, phylogenetic diversity, Queensland.
Introduction
Mycorrhizal associations in low-fertility substrates
The extensive loss of heathlands on the Sunshine Coast, Queensland, Australia, at the end of last century, prompted enthusiasts to translocate small heath plants prior to patches being razed for urban structures. These small-scale attempts were invariably unsuccessful, prompting the comment ‘There must be something in the soil our little plants need!’ In light of this comment, and more recent research on mycorrhizal associations in the patterning of heathland vegetation (Lambers et al. 2011, 2018; Silveira et al. 2016), this Short Communication overviews some general patterns we have observed in the Sunshine Coast heathlands, highlighting opportunities for further exploration of the significance of these associations.
Mycorrhizal symbionts, and other root specialisations, have long been recognised as associated with many Australian heathland plants, naturally growing in low-fertility or seasonally waterlogged substrates (Westman 1975; Lamont 1981; Malajczuk and Lamont 1981). Mycorrhizal fungi colonise plant roots, where they benefit from plant carbon resources, facilitate plant access to nutrients and water, and may form extensive below-ground networks between conspecific and heterospecific plants (Soudzilovskaia et al. 2020; Tedersoo et al. 2020; Albornoz et al. 2021). Increasingly, it appears that some mycorrhizal associates can perform protective functions against pathogens (Albornoz et al. 2017, 2021; Lambers et al. 2018). Work in phosphate-impoverished soils in some heaths of Western Australian have found increasing age and soil infertility associated with a higher diversity of plants, and a higher diversity of mycorrhizal strategies (Lambers et al. 2008, 2011; Zemunik et al. 2016). On the most phosphate-impoverished substrates there was also an observed increase in the proportion of non-mycorrhizal plants (NM) with specialised root clusters that effectively ‘mine’ the soil for phosphorus (Lambers et al. 2008; Silveira et al. 2016; Lamont and Keith 2017).
Recent work in our group found the Sunshine Coast heathlands to have a low phylogenetic diversity in comparison with surrounding rainforest, and one of the nine ‘regional ecosystems’ was found to be ‘phylogenetically clustered’, suggestive of environmental filtering by low-fertility substrates and variable moisture regimes (Pearl et al. 2022). We raise here the possibility that mycorrhizal associations may affect community assembly in these heathlands and thus the plant phylogenetic diversity. Although phylogenetic clustering may represent locations where species had been subject to environmental filtering, such as recently expanded communities (Webb et al. 2002; Swenson 2012), it has also been argued that facilitative interactions may play a part in patterns of community assembly (Cadotte et al. 2017; Veron et al. 2019; Munkemuller et al. 2020). Mutualistic exchange among closely related species, facilitated by sharing a common mycorrhizal symbiont, may decrease their vulnerability to environmental change, resulting in a clustered community (Prinzing et al. 2017). In contrast, facilitation between species with different life strategies, such as sharing a less specialised mycorrhizal symbiont, could result in over-dispersed or random community patterns (Gerhold et al. 2018; Munkemuller et al. 2020).
There has been no overview of the proportions of mycorrhizal functional groups associated with the heath flora in the south-eastern Queensland region, nor in the surrounding rainforest communities. Such an overview may instigate research towards understanding whether ‘there is something in the soil our little (heath) plants need’. Recent work on potential facilitation between some Proteaceae and Myrtaceae shrub species (Albornoz et al. 2017) also suggested that an overview of the patterns of associations in the different heath strata could be informative.
The publication of the ‘FungalRoot’ database (Soudzilovskaia et al. 2020) made it possible to explore mycorrhizal patterns in a general sense, by allocating potential mycorrhizal functional groups to each plant genus in the Sunshine Coast heath and rainforest flora list. The four major types of recognised mycorrhizal groups are arbuscular mycorrhiza (AM), ectomycorrhiza (EcM), ericoid mycorrhiza (ErM) and orchid mycorrhiza (OM) (Brundrett and Tedersoo 2018; Soudzilovskaia et al. 2020). In contrast, non-mycorrhizal plants (NM) have specialised root structures such as cluster roots (e.g. Proteaceae) or dauciform roots (e.g. Cyperaceae), which release carboxylates to extract nutrients such as phosphate from the soil, or have other strategies such as carnivory or parasitism (Lambers et al. 2011, 2018; Barrett 2013; Brundrett and Tedersoo 2018). Some species are known to employ a variety of strategies, such as many Cyperaceae, which are NM (dauciform roots) as well as AM, and many Myrtaceae employing EcM and AM strategies (Soudzilovskaia et al. 2020).
This Short Communication provides an overview of patterns of potential mycorrhizal functional groups for the Sunshine Coast heath flora. We emphasise that this is not a study documenting the presence of the mycorrhiza, but of the potential mycorrhizal strategies of the plant communities. We recognise that evidence for the linking of plant community structure with mycorrhizal community structure is limited (Horn et al. 2017). We are aware that FungalRoot is a constantly evolving global database, and our use of this database acknowledges that allocated functional groups to plant genera cannot always be assumed within specific plant genera or in an Australian context (Albornoz et al. 2021).
However, so as to explore ideas and to invite discussion and interest from mycorrhizal and heathland specialists, we used existing species lists and vegetation cover data to compare the following:
The proportions of plants associated with the various mycorrhizal functional groups in the rainforest and heath flora communities from the Sunshine Coast region; are they different from each other?
The differences in coverage of plants associated with the various mycorrhizal functional groups in the groundcover, shrub and tree and emergent strata of the heath community; are the strata different, and what questions does this raise?
Materials and methods
Comparison of rainforest and heath mycorrhizal functional groups in the Sunshine Coast
This dataset of plant taxa used for this study was based on the Sunshine Coast heath species list of 366 species derived from 80 sites, and records from other data sources (Pearl et al. 2022; Supplementary Table S1). This was compared with a species list of 470 species from a rainforest study over 109 sites in the same geographic area (Fig. 1), and from the same research group (Scanlan et al. 2018). Neither of these datasets focussed on a complete orchid inventory. All sites were 0.1 ha in size.
Map of Australia, indicating the Sunshine Coast study area. In the expanded box, the Sunshine Coast area, showing the location of the 80 heathland study sites (red dots), spread over the four local government areas (LGAs) in which the sites are predominantly located. The rainforest species list was derived from 109 sites (not shown here) from the Noosa Shire, and Sunshine Coast Regional Council areas (Scanlan et al. 2018). All sites were 0.1 ha in size.
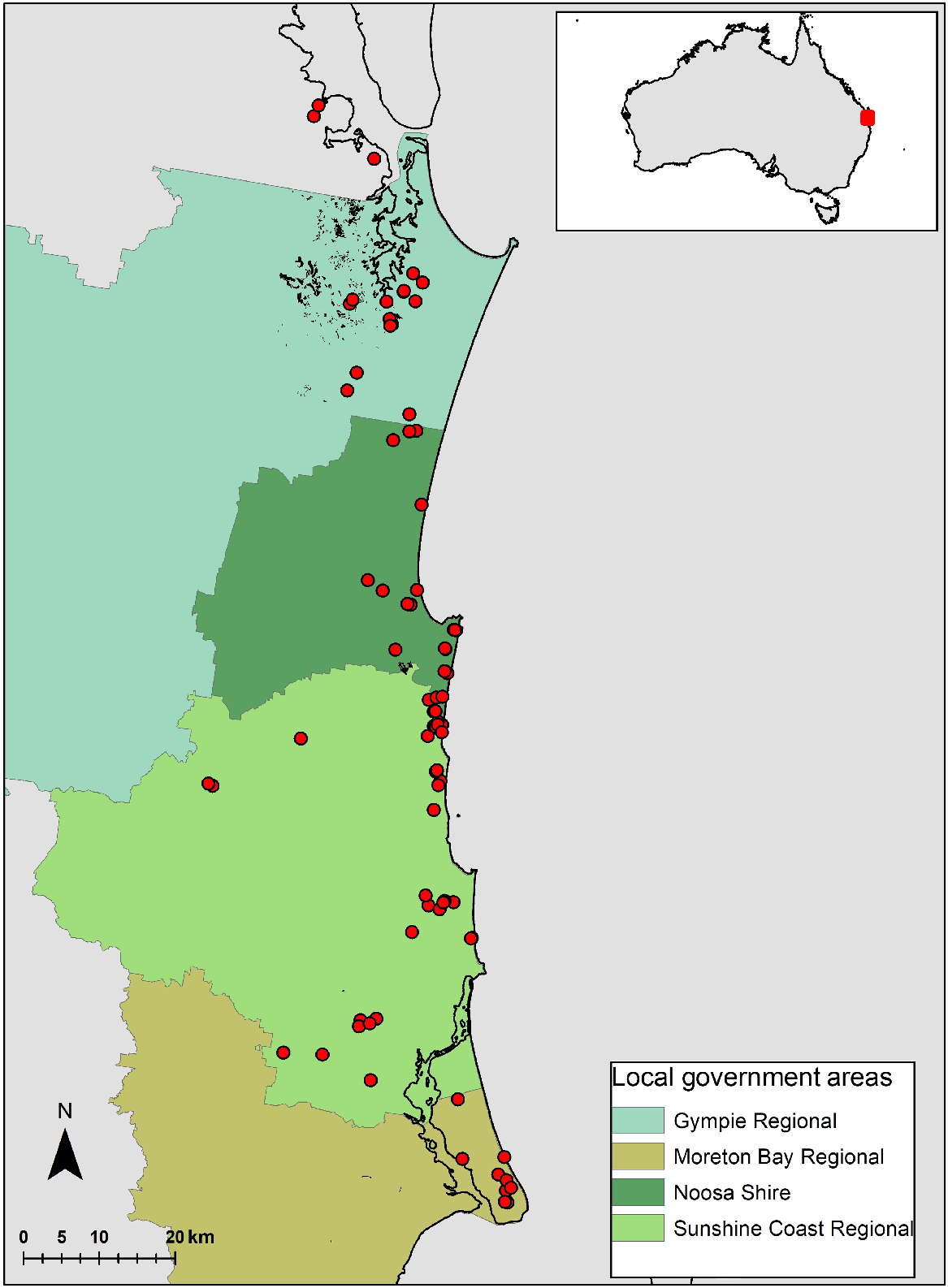
For the Sunshine Coast heath and rainforest communities, each plant species was allocated a mycorrhizal functional group, using the FungalRoot database (Soudzilovskaia et al. 2020). Species were allocated as AM, EcM–AM, ErM, NM, N–AM (Table S1). Ten species with no clear allocation to one of those groups (e.g. Brachyloma spp., Macarthuria spp.) were allocated ‘Other’. The proportion of plant species allocated to each functional group was calculated and graphically displayed using Excel (Microsoft Corporation, https://www.microsoft.com/en-au/microsoft-365/excel).
An existing phylogeny developed for the 366 heath species from this research group (Pearl et al. 2022) was used to visualise the distribution of mycorrhizal functional groups across the Sunshine Coast heath phylogenetic tree, by using the Interactive Tree of Life (Letunic and Bork 2011).
Patterns within the heath strata
We used an existing dataset collected for the Sunshine Coast heathlands over 80 sites, 50 × 20 m in size, selected as representative from nine heath types mapped as Queensland regional ecosystems (Neldner et al. 2019), extending from Cooloola in the north, 150 km south to Bribie Island, and west up to 30 km to Mapleton and the Glasshouse Mountains (Pearl et al. 2022). Over the 80 sites, 280 species were encountered of the 366 species of the heath species of this project, with species identification being confirmed with Queensland Herbarium curators (Pearl et al. 2022).
These data were collected as follows, and it is emphasised that this was collected only for the heath sites, and the same methodology was not employed at the rainforest sites. At each site, the vegetation strata were identified as ‘tree and emergent,’ ‘shrub,’ or ‘groundcover.’ The number of species was collated for each stratum over each 50 × 20 m site. The percentage cover of each species of the ‘tree and emergent’ and ‘shrub’ strata was collected during fieldwork by using the line-intercept method over a 50-m length (Neldner et al. 2017). The cover of groundcover stratum components was measured using the projective foliage-cover method in five 1-m2 quadrats randomly allocated along the transect line (Neldner et al. 2017).
Using this existing dataset, for each of the 80 sites, the relative number of plant species allocated to each functional mycorrhizal group was calculated for each stratum (groundcover, shrub, tree and emergent) by using the ‘decostand’ function in ‘vegan’, in R (ver. 2.6-4; Oksanen et al. 2020; R Core Team 2020). Similarly, the relative cover of the plant species allocated to each functional group was calculated for each of the 80 sites for each stratum.
All 80 sites contained a ground stratum and a shrub stratum, and the average relative number of plant species, and the average relative cover were calculated, along with the standard deviations (n = 80), and displayed using ‘ggplot2’ in R (ver. 3.4.2; Wickham 2016; R Core Team 2020). This was repeated for the emergent stratum for the 55 sites that contained emergent or tree species (n = 55).
Results
Comparison of rainforest and heath flora mycorrhizal function groups in the Sunshine Coast
For this general overview, in the Sunshine Coast rainforest species list, the proportion of plant species associated with each mycorrhizal functional group was 80.4% AM, 0.2% EcM, 3.8% EcM–AM, 0.2% ErM, 2.3% NM, and 8.5% NM–AM, and 4.5% other associations (Fig. 2). We found the distribution of potential mycorrhizal strategies associated with plant species to be different in the Sunshine Coast heathlands, with less than half (38.1%) the percentage of AM genera as compared with the rainforest community (Fig. 2). In the heath flora, ericoid mycorrhizal (EM) strategies were more prevalent (5.4%), with double the proportion of NM–AM genera (17.4%), and four times the proportion of NM genera (10.1%; Fig. 2).
Sunshine Coast rainforest and heath species, showing the percentage of species allocated to each functional type of mycorrhizal strategy: arbuscular (AM), ectomycorrhizal (EcM), ericoid (ErM), non-mycorrhizal (NM), NM–AM variable NM or AM, EcM–AM variable EcM or AM. Rainforest species list of 470 species from Scanlan et al. (2018). Heath species list of 366 species as listed in Table S1. Mycorrhizal allocations for each species on the basis of genera, from the ‘FungalRoot’ database (Soudzilovskaia et al. 2020).
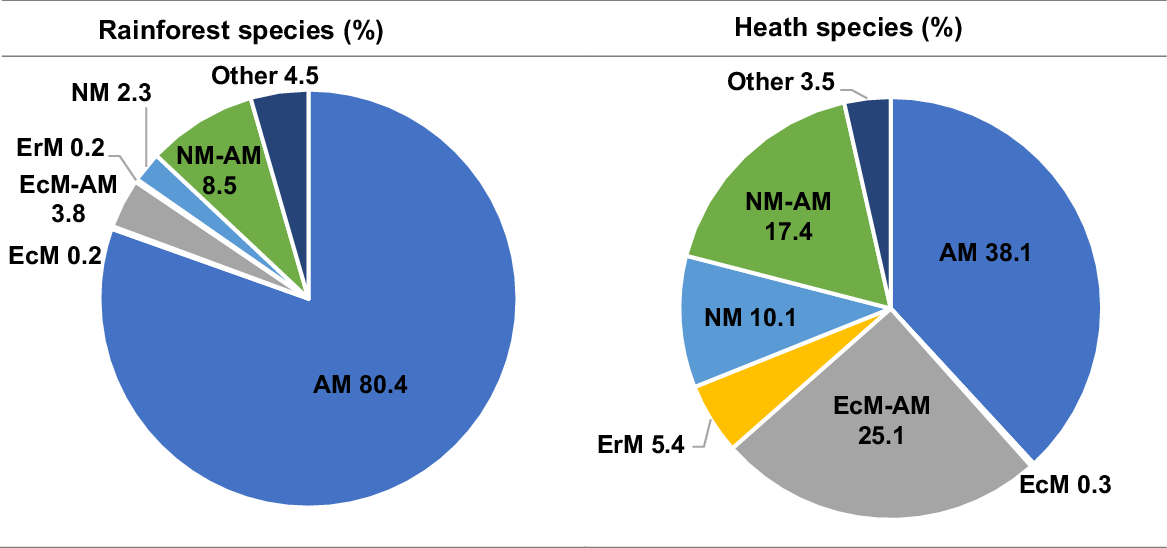
The phylogeny highlights the dominance of the Myrtaceae, and Fabaceae (including Acacia spp.) in the Sunshine Coast heath flora, with the majority of their species potentially forming EcM–AM associations (Fig. 3, and see Table S1). The order Poales contains three major heath families found in this study (Pearl et al. 2022), with the Cyperaceae and Restionaceae potentially forming NM–AM associations, and the Poaceae forming AM associations (Fig. 3). The NM strategies dominate the family of Proteaceae, represented in the Sunshine Coast heaths by genera such as Banksia and Persoonia (Fig. 3). Largely, the family Ericaceae forms ErM associations, and the Rutaceae family (Boronia spp.) forms AM associations (Fig. 3). There are exceptions to these dominant patterns in almost all major families (Fig. 3).
Phylogeny of the 366 Sunshine Coast heath species, showing the allocation of each species to a functional type of mycorrhizal strategy: arbuscular (AM), ectomycorrhizal (EcM), ericoid (ErM), non-mycorrhizal (NM), NM–AM variable NM or AM, EcM–AM variable EcM or AM. The position of major heath families or genera are indicated. Heath species list of 366 species as listed in Table S1. Mycorrhizal allocations for each species are based on genera, from the ‘FungalRoot’ database (Soudzilovskaia et al. 2020). Figure was produced in ITOL (Letunic and Bork 2011).
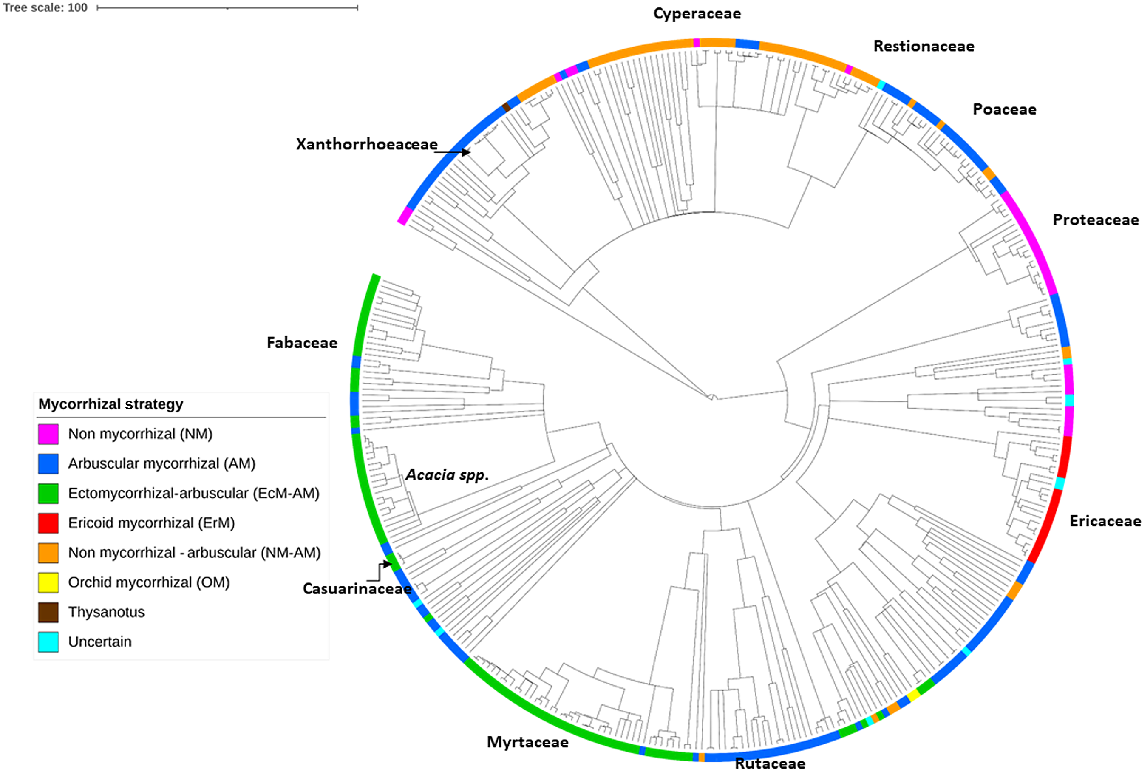
Patterns in the heath strata of plants within their allocated mycorrhizal functional groups
We found that each stratum of the heath showed differing patterns of number and cover of the plants associated with each mycorrhizal functional group. In the groundcover stratum, under a third (a relative average of 0.30, or 30%) of the species in the heath groundcover flora are NM–AM, yet this group dominated the cover ( = 56%) represented by genera such as Empodisma, Ptilothrix, Schoenus, Sporadanthus, Caustis and Baloskion (Fig. 4, Table S2). In contrast, most of the heath groundcover stratum species are AM ( = 48%), but with a much lower cover ( = 34%), and the proportion of AM species declines rapidly in the remaining strata (Fig. 4).
The average relative number of plant species (left), and average relative coverage of plants (right), with standard deviations indicated by the bars, from each stratum of the Sunshine Coast heathlands, of plants allocated to each functional group of mycorrhizal strategy: arbuscular (AM), ectomycorrhizal (EcM), ericoid (ErM), non-mycorrhizal (NM), NM–AM variable NM or AM, EcM–AM variable EcM or AM. The data are based on the 280 heath species encountered over 80 sites of 0.1 ha, over the Sunshine Coast region (Pearl et al. 2022). Mycorrhizal allocations for each species are based on genera, from the ‘FungalRoot’ database (Soudzilovskaia et al. 2020).
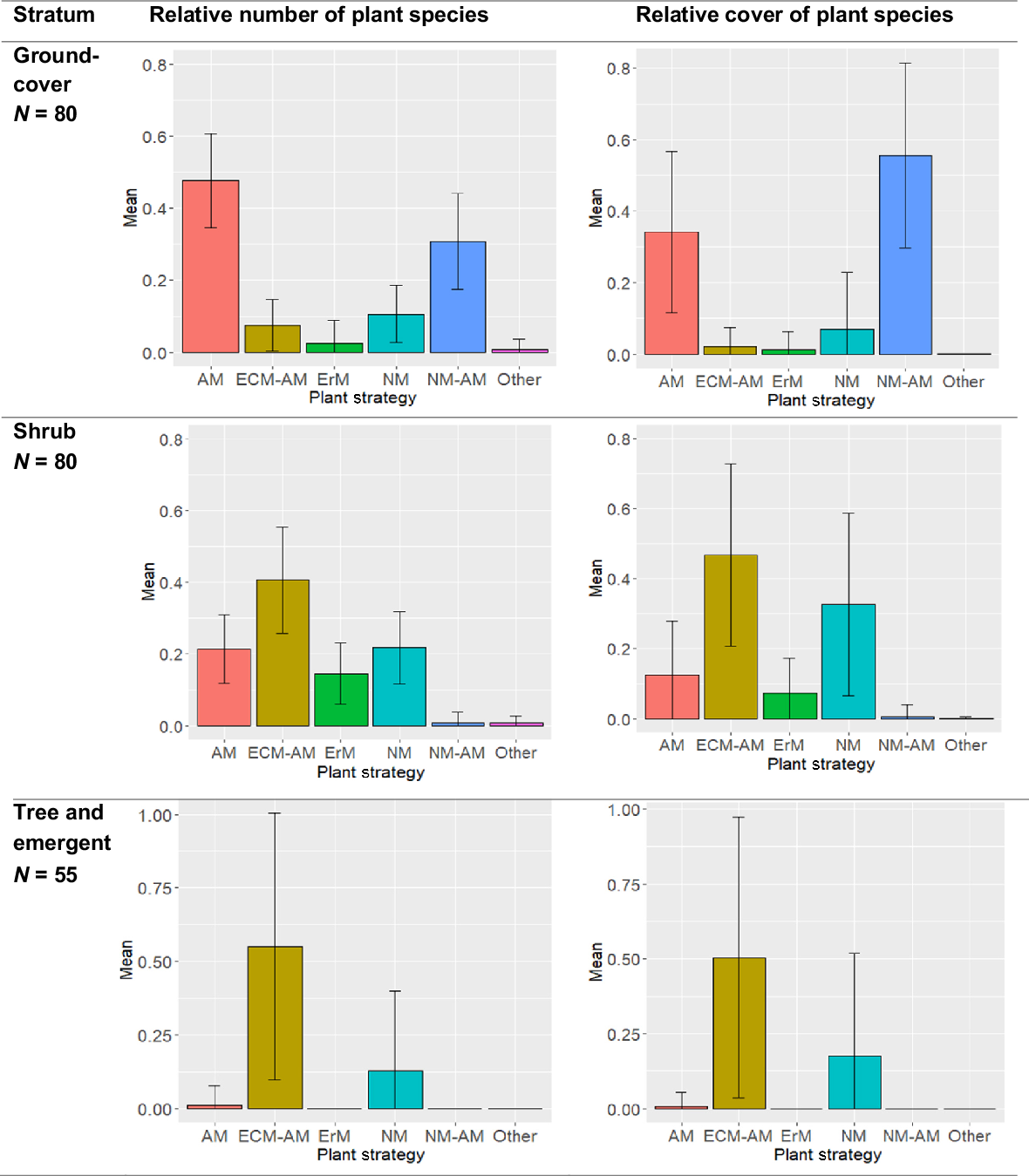
The heath shrub stratum is dominated in number ( = 41%) and cover ( = 47%) by EcM–AM genera including Leptospermum, Melaleuca and Baeckea (Table S2). The NM genera, represented by Banksia and Hakea, represent just over a fifth of the average number of species ( = 21%), but a third ( = 33%) of the cover (Fig. 4). It is in the shrub stratum that ErM species, represented by genera such as Epacris and Leucopogon, appear most strongly, although the proportion of species and amount of cover is lower than for other mycorrhizal strategies (Fig. 4).
This study found the tree and emergent stratum to be dominated in the number of species ( = 80%) and cover ( = 73%) by EcM–AM genera such as Eucalyptus, Melaleuca, Corymbia, Acacia, and Allocasuarina (Fig. 4, Table S2). However, although there are only three NM tree and emergent species ( = 18% of species of that stratum) in the Sunshine Coast heath from the genera Banksia (two species) and Persoonia, they represent an average relative cover of 0.26 (Fig. 4).
Discussion
What causes the patterning of mycorrhizal strategies in Sunshine Coast rainforest and heath?
Arbuscular mycorrhiza were the earliest evolving association in land plants, and are globally found in 80% of vascular plants (Brundrett and Tedersoo 2018). The proportion of plant species in each mycorrhizal functional group across the globe is estimated at 72% AM, 7% NM–AM, 2.0% EcM, 1.5% ErM, 10% OM, and 8% NM (Brundrett and Tedersoo 2018). Arbuscular mycorrhiza (AM) are ubiquitous, and this overview supports this in both the Sunshine Coast heath and rainforest plant communities. However, although all types of mycorrhizal strategies are potentially associated with the heath and rainforest communities, there appears to be a lower proportion of plant species with possible AM associations in the heath community. This is consistent with general patterns observed in other low-fertility heath environments in Australia and Brazil (Lambers et al. 2011; Silveira et al. 2016; Lamont and Keith 2017).
Globally, ectomycorrhizal (EcM) associations are found mostly in trees in poor soil, ErM in shrubs in highly acidic soils, and NM in extremely disturbed or phosphorus-starved soils (Soudzilovskaia et al. 2020; Tedersoo et al. 2020). Non-mycorrhizal plants with dauciform roots (e.g. Cyperaceae) and proteoid roots (e.g. Proteaceae) have been found elsewhere to represent a significant proportion of species on the poorest soils (Lambers et al. 2011; Hayes et al. 2021). Thus, the possible higher proportion of NM and EcM mycorrhizal functional groups in the heath flora, and the possible greater diversity of mycorrhizal strategies, is consistent with this, and further targeted research would confirm these patterns. The NM strategies, which are the most efficient for phosphorus ‘mining’, could be related to possible phosphorus limitations of the Sunshine Coast heathland (Coaldrake and Haydock 1958; Chen et al. 2015), as has been suggested in Western Australian kwongan heaths (Lambers et al. 2011; Zemunik et al. 2016).
What causes the patterning in the heath strata of plants associated with each mycorrhizal functional group?
Broad global patterns show that most ErM-associated species are found in the shrub stratum, most EcM species in trees, and EcM–AM (e.g. Myrtaceae) species in shrubs and trees (Soudzilovskaia et al. 2020). Non-mycorrhizal plants and facultative AM species are more often found as herbs or graminoids (Tedersoo et al. 2020). This study found similar patterns, with almost all ErM species (e.g. Ericaceae) found in the shrub stratum, and EcM–AM associations dominating the proportion of species in the tree and emergent stratum. The high proportion (0.30) of species associated with NM–AM strategies present in the groundcover stratum is consistent with the presence of restiads and sedges in this stratum. This is in contrast to grasses, which are mostly AM-associated species (Tedersoo et al. 2020).
It is noted that within the three strata of heath, the cover of plants associated with different mycorrhizal strategies was not aligned with the proportion of species. Species with AM associations in the groundcover stratum represented a lower proportion of the plant cover than their species numbers would suggest. In contrast, fewer species were associated with NM–AM (restiads and sedges), yet they dominated the groundcover. Clearly, in the groundcover stratum of the Sunshine Coast heath, there is dominance by just a few NM–AM species, such as Empodisma minus, consistent with this species being proposed as an ‘ecosystem engineer’ in peat formation in the fens and wet heaths of this region (Fairfax and Lindsay 2019).
This overview found a high proportion of ECM–AM plants both in species numbers and cover, in the shrub stratum and the tree and emergent stratum. These plants include the Myrtaceae, represented by the genera Leptospermum, Melaleuca, and Eucalyptus, and the broad family of Fabaceae (including Acacia spp.; Table S2). It has been suggested plants with EcM associations can dominate some ecosystems, owing to the protective hyphal sheath around the plant roots (Tedersoo et al. 2020). Further, in this overview, the tree and emergent stratum contained almost exclusively EcM–AM-associated species alongside NM species (Proteaceae). The coexistence of these plant mycorrhizal strategies may be related to defence against pathogens, competitive interactions, and facilitation (Albornoz et al. 2017, 2021; Lambers et al. 2018). The competitive advantage of the NM strategies of the cluster-rooted Proteaceae, which mobilise phosphate, may also facilitate phosphate acquisition by neighbouring EcM species (Albornoz et al. 2017). The almost exclusive coexistence of NM and EcM–AM species in the tree and emergent stratum of Sunshine Coast heathlands raises the possibility that facilitative strategies may be occurring, and invites the development of projects to investigate this further. Facilitation and competitive interactions among different plant genera may be contributing to a greater phylogenetic dispersion in the community structure than may otherwise be the case (Gerhold et al. 2018; Munkemuller et al. 2020).
Conclusions
This study has provided the first general overview of plant species grouped by potential mycorrhizal associations in the Sunshine Coast heathlands, and is unique in being able to compare those proportions with those in the surrounding rainforest flora. Our results suggest that there may be a greater diversity of mycorrhizal strategies in the heath plant community, and a higher proportion of plant species with mycorrhizal strategies consistent with low-nutrient substrates (Lambers et al. 2011; Zemunik et al. 2016). This overview found a dominance of plants associated with AM and NM–AM in the groundcover stratum. In contrast, the shrub and tree and emergent strata contained plants associated with EcM–AM and NM strategies, being consistent with the possibility of facilitation of competitive interactions among these plants species.
The Sunshine Coast heath community is phylogenetically clustered when compared with the surrounding rainforest community (Pearl et al. 2022). General patterns from this overview suggest that facilitation and competitive interactions related to the differing mycorrhizal and non-mycorrhizal strategies of the heath plant species may be enabling the intermingling of plant species from the dominant heath families (Myrtaceae, Fabaceae, Proteaceae, Cyperaceae, Restionaceae, and Ericaceae) in the Sunshine Coast heathlands. This could be contributing to a higher phylogenetic dispersion in the heath than would otherwise be the case, and invites the development of projects to explore these interactions.
Data availability
The heath species list and allocated mycorrhizal strategies are available in the Table S1. The GenBank and BOLD accession numbers for the heath species sequences are listed by Pearl et al. (2022). Further data that support this study will be shared upon reasonable request to the corresponding author.
Declaration of funding
This work formed part of a PhD project supported by an Australian Government Research Training Program Scholarship, the University of the Sunshine Coast, the Holsworth Wildlife Research Endowment – Equity Trustees Charitable Foundation and the Ecological Society of Australia. Funding was also received from the Friends of the Maroochy Bushland Botanic Gardens, Tanawha, and the Somerville House Old Girl’s Association.
Acknowledgements
Rainforest sequences data and species lists were developed by Alison Shapcott and Marion Howard, in collaboration with W. John Kress and David Erickson of the National Museum of Natural History at the Smithsonian Institution, Washington, DC, funded by a Queensland Smithsonian Fellowship and the Genecology Research Centre at the University of the Sunshine Coast. This work was undertaken in collaboration with Mr Tim Ryan, Science Leader, Queensland Herbarium, Queensland Department of Environment and Science, who assisted with field-work training. We also thank Ann Moran, Allan Ward, Alex Pearl and Erin Cameron for assistance with field work, and the University of the Sunshine Coast fieldwork staff. This work was supported by the Centre for Bioinnovation and the School of Science, Technology and Engineering at the University of the Sunshine Coast. The Queensland Department of Environment and Heritage Protection provided permits for fieldwork in protected estates: permit numbers WITK 17429716 and WITK 18628117.
References
Albornoz FE, Burgess TI, Lambers H, Etchells H, Laliberte E (2017) Native soilborne pathogens equalize differences in competitive ability between plants of contrasting nutrient-acquisition strategies. Journal of Ecology 105, 549-557.
| Crossref | Google Scholar |
Albornoz FE, Dixon KW, Lambers H (2021) Revisiting mycorrhizal dogmas: are mycorrhizas really functioning as they are widely believed to do? Soil Ecology Letters 3, 73-82.
| Crossref | Google Scholar |
Barrett RL (2013) Ecological importance of sedges: a survey of the Australasian Cyperaceae genus Lepidosperma. Annals of Botany 111, 499-529.
| Crossref | Google Scholar | PubMed |
Brundrett MC, Tedersoo L (2018) Evolutionary history of mycorrhizal symbioses and global host plant diversity. New Phytologist 220, 1108-1115.
| Crossref | Google Scholar | PubMed |
Cadotte MW, Davies TJ, Peres-Neto PR (2017) Why phylogenies do not always predict ecological differences. Ecological Monographs 87, 535-551.
| Crossref | Google Scholar |
Chen CR, Hou EQ, Condron LM, Bacon G, Esfandbod M, Olley J, Turner BL (2015) Soil phosphorus fractionation and nutrient dynamics along the Cooloola coastal dune chronosequence, southern Queensland, Australia. Geoderma 257–258, 4-13.
| Crossref | Google Scholar |
Coaldrake JE, Haydock KP (1958) Soil phosphate and vegetal pattern in some natural communities of south-eastern Queensland, Australia. Ecology 39, 1-5.
| Crossref | Google Scholar |
Fairfax R, Lindsay R (2019) An overview of the patterned fens of Great Sandy Region, far eastern Australia. Mires and Peat 24, 1-18.
| Crossref | Google Scholar |
Gerhold P, Carlucci MB, Proches S, Prinzing A (2018) The deep past controls the phylogenetic structure of present, local communities. Annual Review of Ecology, Evolution, and Systematics 49, 477-497.
| Crossref | Google Scholar |
Hayes PE, Nge FJ, Cramer MD, Finnegan PM, Fu P, Hopper SD, Oliveira RS, Turner BL, Zemunik G, Zhong H, Lambers H (2021) Traits related to efficient acquisition and use of phosphorus promote diversification in Proteaceae in phosphorus-impoverished landscapes. Plant and Soil 462, 67-88.
| Crossref | Google Scholar |
Horn S, Hempel S, Verbruggen E, Rillig MC, Caruso T (2017) Linking the community structure of arbuscular mycorrhizal fungi and plants: a story of interdependence? The ISME Journal 11, 1400-1411.
| Crossref | Google Scholar | PubMed |
Lambers H, Raven JA, Shaver GR, Smith SE (2008) Plant nutrient-acquisition strategies change with soil age. Trends in Ecology & Evolution 23, 95-103.
| Crossref | Google Scholar | PubMed |
Lambers H, Brundrett MC, Raven JA, Hopper SD (2011) Plant mineral nutrition in ancient landscapes: high plant species diversity on infertile soils is linked to functional diversity for nutritional strategies. Plant and Soil 348, 7-27.
| Crossref | Google Scholar |
Lambers H, Albornoz F, Kotula L, Laliberte E, Ranathunge K, Teste FP, Zemunik G (2018) How belowground interactions contribute to the coexistence of mycorrhizal and non-mycorrhizal species in severely phosphorus-impoverished hyperdiverse ecosystems. Plant and Soil 424, 11-33.
| Crossref | Google Scholar |
Letunic I, Bork P (2011) Interactive Tree Of Life v2: online annotation and display of phylogenetic trees made easy. Nucleic Acids Research 39, W475-W478.
| Crossref | Google Scholar | PubMed |
Munkemuller T, Gallien L, Pollock LJ, Barros C, Carboni M, Chalmandrier L, Mazel F, Mokany K, Roquet C, Smycka J, Talluto MV, Thuiller W (2020) Dos and don’ts when inferring assembly rules from diversity patterns. Global Ecology and Biogeography 29, 1212-1229.
| Crossref | Google Scholar |
Neldner VJ, Butler DW, Guymer GP (2019) Queensland’s regional ecosystems: building and maintaining a biodiversity inventory, planning framework and information system for Queensland Version 2.0. (Queensland Herbarium, Queensland Department of Environment and Science: Brisbane, Qld, Australia) Available at https://www.publications.qld.gov.au/dataset/redd/resource/42657ca4-848f-4d0e-91ab-1b475faa1e7d
Oksanen J, Blanchet FG, Friendly M, Kindt R, Legendre P, McGlinn D, Minchin R, O’Hara B, Simpson G, Solymos P, Stevens H, Szoecs E, Wagner H (2020) vegan: community ecology package. Available at https://CRAN.R-project.org/package=vegan
Pearl H, Ryan T, Howard M, Shimizu Y, Shapcott A (2022) DNA barcoding to enhance conservation of sunshine coast heathlands. Diversity 14, 436.
| Crossref | Google Scholar |
Prinzing A, Ozinga WA, Brandle M, Courty P-E, Hennion F, Labandeira C, Parisod C, Pihain M, Bartish IV (2017) Benefits from living together? Clades whose species use similar habitats may persist as a result of eco-evolutionary feedbacks. New Phytologist 213, 66-82.
| Crossref | Google Scholar | PubMed |
R Core Team (2020) R project for statistical computing. Available at https://www.r-project.org/
Scanlan L, McDonald WJF, Shapcott A (2018) Phylogenetic diversity and conservation of rainforests in the Sunshine Coast region, Queensland, Australia. Australian Journal of Botany 66, 518-530.
| Crossref | Google Scholar |
Silveira FAO, Negreiros D, Barbosa NPU, Buisson E, Carmo FF, Carstensen DW, Conceicao AA, Cornelissen TG, Echternacht L, Fernandes GW, Garcia QS, Guerra TJ, Jacobi CM, Lemos J-P, Le Stradic S, Morellato LPC, Neves FS, Oliveira RS, Schaefer CE, Viana PL, Lambers H (2016) Ecology and evolution of plant diversity in the endangered campo rupestre: a neglected conservation priority. Plant and Soil 403, 129-152.
| Crossref | Google Scholar |
Soudzilovskaia NA, Vaessen S, Barcelo M, He J, Rahimlou S, Abarenkov K, Brundrett MC, Gomes SIF, Merckx V, Tedersoo L (2020) FungalRoot: global online database of plant mycorrhizal associations. New Phytologist 227, 955-966.
| Crossref | Google Scholar | PubMed |
Swenson NG (2012) Phylogenetic analyses of ecological communities using DNA barcode data. In ‘DNA barcodes: methods and protocols’. (Eds WA Kress, DL Erikson) pp. 409–419. (Springer: New York, NY, USA) doi:10.1007/978-1-61779-591-6_20
Tedersoo L, Bahram M, Zobel M (2020) How mycorrhizal associations drive plant population and community biology. Science 367, eaba1223.
| Crossref | Google Scholar |
Veron S, Saito V, Padilla-Garcia N, Forest F, Bertheau Y (2019) The use of phylogenetic diversity in conservation biology and community ecology: a common base but different approaches. The Quarterly Review of Biology 94, 123-148.
| Crossref | Google Scholar |
Webb CO, Ackerly DD, McPeek MA, Donoghue MJ (2002) Phylogenies and community ecology. Annual Review of Ecology and Systematics 33, 475-505.
| Crossref | Google Scholar |
Westman WE (1975) Pattern and diversity in swamp and dune vegetation, North Stradbroke Island. Australian Journal of Botany 23, 339-354.
| Crossref | Google Scholar |
Zemunik G, Turner BL, Lambers H, Laliberte E (2016) Increasing plant species diversity and extreme species turnover accompany declining soil fertility along a long-term chronosequence in a biodiversity hotspot. Journal of Ecology 104, 792-805.
| Crossref | Google Scholar |