Understanding Rhododendron intraspecific compatibility in botanic garden collections for species conservation
Ling Hu
A
B
C
Abstract
Controlled pollination is an important technique for maintaining intraspecific diversity in integrated plant conservation practices, particularly in genera such as Rhododendron, where open pollination usually produces hybrids with unknown paternal lineages.
This study investigated the capacity for viable seed set from self- and intraspecific cross-pollination for Rhododendron taxa in different categories of the International Union for Conservation of Nature (IUCN) Red List, to guide conservation management of threatened species in botanic garden collections.
The following five taxa of subsection Maddenia were studied: R. dalhousiae var. dalhousiae (Least Concern), R. dalhousiae var. rhabdotum (Vulnerable), R. lindleyi (Least Concern), R. nuttallii (Near Threatened), and R. excellens (Vulnerable). Controlled pollination was performed on selected garden accessions, and seed germination was tested at an alternating temperature regime of 15/25°C, 8 h photoperiod, and ~6 μmol m−2 s−1 photosynthetic photon flux density (PPFD).
Intraspecific compatibilities varied among different taxa and between self- and outcross treatments. X-ray images for Rhododendron seeds showed low capacity to predict seed germination. Neither X-ray scan nor fungicide (Ridomil) treatment showed any adverse impact on seed germination, which has positive implications for seed-banking and subsequent raising of Rhododendron seedlings.
Controlled intraspecific pollination can be used to maintain diversity of ex situ accessions for selected Rhododendron species. However, the zero or low compatibility demonstrated in some species, such as R. excellens, suggests that these species may require a different approach.
Intraspecific pollination should be evaluated for each Rhododendron species before a propagation program is initiated in ex situ conservation.
Keywords: biodiversity conservation, botanic garden, controlled pollination, ex situ collection, germplasm, intraspecific compatibility, Rhododendron, seed germination, subsection Maddenia.
Introduction
As part of an ‘integrated conservation strategy’ for safeguarding plant biodiversity, ex situ conservation focuses on holding plant collections outside their natural habitats in facilities such as seed banks or botanic gardens, which provides easy access for utilisation and re-introduction of species under threat (Oldfield and Newton 2012; Gratzfeld 2017; Heywood 2017; Smith and Pence 2017). Maintaining genetic diversity in collections is essential for ex situ conservation, which may require strategic breeding to generate new plants through sexual (seed) reproduction (Gratzfeld 2017; Smith and Pence 2017). Management of ex situ seed reproduction is particularly necessary for genera such as Rhododendron (Ericaceae), which hybridise easily (Zha et al. 2008; Ma et al. 2010; Jamieson 2021), and where open-pollinated seeds are likely to be hybrids. Indeed, collections of taxa and populations that are geographically isolated in nature and brought together in a botanic garden can generate an extreme form of artificial sympatry (Maunder et al. 2004b), which is undesirable in species conservation. Careful management is needed to facilitate the safe or ‘pure’ propagation of threatened taxa for conservation (Volis 2017; Forgiarini et al. 2023). Controlled pollination increases genetic exchange between representative (ideally wild) accessions, which helps maintain the germplasm of prioritised species (Maunder et al. 2004a; Volis 2017).
Rhododendron is a ‘big genus’ (Frodin 2004) of over 1000 species, in which some 349 (25%) taxa (species, subspecies, varieties) are under threat (Gibbs et al. 2011; MacKay et al. 2018). Some species such as R. coxianum and R. fleuryi (both Critically Endangered) have small wild populations and are also scarce in cultivation (Hu et al. 2024), whereas R. kanehirae (Extinct in the Wild) presently relies on botanic garden cultivation for its continued existence. For many Rhododendron species, there is a limited number of accessions held in ex situ collections (Hu et al. 2024) and use of controlled pollination to increase the number of individuals in cultivation, while maintaining genetic diversity, may be a useful ex situ conservation approach.
Understanding the reproductive biology and breeding system of threatened species is important for the production of viable seeds and offspring in conservation horticulture (Schoen and Brown 1991; Hollingsworth et al. 2006; van der Walt et al. 2022). Williams et al. (1990) summarised the detailed reproductive biology of Rhododendron, including a description of the morphological characteristics of reproductive structures, fertilisation process, and seed development. Rhododendron species are mostly pollinated by bees, butterflies and birds, with pollen tetrads connected by viscin threads that can be easily attached to the pollinator (Bowers 1930; Williams et al. 1990; Basnett and Ganesan 2022). Reproductive biology of Rhododendron species has been generally studied to develop an understanding of the process of fertilisation (Palser 1986; Palser et al. 1989a; Williams et al. 1990). Breeding systems have been studied for several taxonomic groups (Williams et al. 1990; Rouse et al. 1993) or species (Mejías et al. 2002; Okamoto and Suto 2004; Okamoto and Ureshino 2015; Ma et al. 2016; Yan et al. 2019), but these usually focused on interspecific crosses and the generation of horticultural hybrids. Only a few have investigated the reproductive biology of Rhododendron species in relation to conservation of species (Li et al. 2018a, 2018b; Cai et al. 2022).
Intraspecific pollination, including selfing and outcrossing, is important for conservation of genetic diversity of species in ex situ collections (van der Walt et al. 2022; Forgiarini et al. 2023). However, not all species are able to produce seeds through selfing. Although Williams et al. (1990) concluded that most Rhododendron species were fully self-compatible, some Rhododendron species (e.g. R. catawbiense) have been reported as self-incompatible (Bowers 1930; Zhuang 2019). Indeed, Williams et al. (1984) noted that post-zygotic abortion may inhibit production of seeds; or even if seeds are formed, they may have problems such as emptiness and failure to germinate, or produce non-viable seedlings (Rouse et al. 1993). Seed yields varied from species to species following self-pollination (Rouse et al. 1993). Takahashi and Itino (2021) reported inbreeding depression of R. kaempferi, with the fruit set from self-pollination being significantly lower than for outcrossing. Whether a species is self- and/or cross-compatible and the extent of compatibility need to be investigated for each individual species, prior to making a conservation decision as to the most appropriate ex situ conservation method to apply (e.g. seed banking, living collections).
Investigation of self- and cross-compatibilities starts with assessing the production of fruit and seed from controlled pollinations. The quality of any seeds produced (such as the proportion filled and their viability) can then be evaluated to ensure healthy offspring to be established in a collection (Martyn Yenson et al. 2021). Percentage of seeds germinated is a key criterion determining seed quality (ISTA 2023). Radiography of seeds (X-ray scan) is a quick and non-destructive approach to determine seed fill, which can predict germination potential before sowing the seeds (Medeiros et al. 2018; Musaev et al. 2022). However, there is no published information on the use of X-ray scans to test Rhododendron seed quality. Also, there is limited information on intraspecific pollination and seed germination for species in subsection Maddenia (subgenus Rhododendron, section Rhododendron) (Palser et al. 1989b; Rouse et al. 1993; Tiwari and Chauhan 2007; Tian 2011).
This case study investigated the feasibility of controlled pollination for viable seeds to conserve intraspecific germplasm of ex situ collections of Rhododendron species in subsection Maddenia. It complements prior studies on ex situ conservation, including examining the ex situ conservation status (Hu et al. 2024), cytogenetics (Hu et al. 2023) and molecular phylogeny of Maddenia species (L Hu, JA Tate, SE Gardiner, MB MacKay, unpubl. data), studied as a means of urgently prioritising distinct species for conservation action.
The following issues were examined: (1) can the selected species produce viable seeds through controlled pollination, and if yes, (2) is there a difference in seed production and quality (such as germination percentage) between self- and outcross pollinations; (3) can X-ray images of Rhododendron seeds indicate seed quality and, hence, predict seed germination; (4) learning from this case study, what issues should botanic gardens consider when conserving Rhododendron through controlled pollination for ex situ collections?
Materials and methods
Species selection and sample accession
In total, 13 accessions of four Rhododendron species (five taxa) in different IUCN Red List categories were used for controlled pollination, with taxa including R. dalhousiae var. dalhousiae (Least Concern), R. dalhousiae var. rhabdotum (Vulnerable), R. excellens (Vulnerable), R. lindleyi (Least Concern), R. nuttallii (Near Threatened) (Table 1, Fig. 1).
Species | Red List | Experimental accessions | Pollination | Number | Thousand-seed weight (g) | Approx. seed number/capsule | ||
---|---|---|---|---|---|---|---|---|
Stigmas | Capsules | |||||||
R. dalhousiae var. dalhousiae | LC | OM32, CH15 – both cultivated source; both flowering in mid-November 2021 | OM32 | 5 | 5 | 0.0940 ± 0.0022 | 4580 | |
OM32 × CH15 | 4 | 1 | 0.1007 ± 0.0013 | 3512 | ||||
R. dalhousiae var. rhabdotum | VU | OM26, OM29 – both cultivated source; both flowering in early January 2022 | OM29 | 9 | 6 | 0.1139 ± 0.0015 | 4150 | |
OM29 × OM26 | 5 | 4 | 0.1065 ± 0.0039 | 3553 | ||||
OM26 × OM29 | 3 | 3 | 0.0895 ± 0.0008 | 4081 | ||||
R. excellens | VU | HP01, PK01, PK10 – all wild origin but source unknown; all flowering in early January 2022 | HP01 | 6 | 1 (empty) | – | – | |
HP01 × PK01 | 7 | 1 (empty) | – | – | ||||
HP01 × PK10 | 11 | 0 | – | – | ||||
R. lindleyi | LC | OM52, PK39 – all cultivated source; both flowering in early November 2021 | OM52 | 7 | 7 | 0.0803 ± 0.0080 | 2423 | |
OM52 × PK39 | 10 | 10 | 0.0821 ± 0.0205 | 2377 | ||||
R. nuttallii | NT | CH16 – cultivated source, flowering in late November 2021; OM50 – wild source from northern Viet Nam, flowering in late November 2021; OM51 – wild source from China, flowering in early November 2021 | OM50 | 11 | 9 | 0.0704 ± 0.0069 | 6825 | |
OM51 | 11 | 11 | 0.0727 ± 0.0029 | 5428 | ||||
OM50 × OM51 | 13 | 13 | 0.0780 ± 0.0044 | 6512 | ||||
OM50 × CH16 | 11 | 11 | 0.0804 ± 0.0041 | 7522 |
In the ‘Red List’ column, categories follow Gibbs et al. (2011) and the updated Red List category in Hu et al. (unpubl. data). In the ‘Pollination’ column, self is represented by ‘’, whereas outcross is ‘(maternal parent) × (paternal parent)’. In the ‘Experimental accessions’ column, flowering time was recorded in the North Island of New Zealand. Initials in accession ID refer to Omahuri (OM; private garden in Manawatū region), Cross Hills Gardens (CH; private garden in Manawatū region), Heritage Park (HP; public garden in Manawatū region) and Pukeiti Gardens (PK; public garden in Taranaki region). Stigmas were pollinated by being dipped either in stored pollen (for outcross) or directly with fresh pollen (for selfing).
LC, Least Concern; NT, Near Threatened; VU, Vulnerable.
Taxa of Rhododendron subsection Maddenia used for controlled pollination in this study. (a) R. dalhousiae var. dalhousiae (OM32); (b) R. dalhousiae var. rhabdotum (OM26); (c) R. excellens (HP01); (d) R. lindleyi (OM52); and (e) R. nuttallii (OM50).
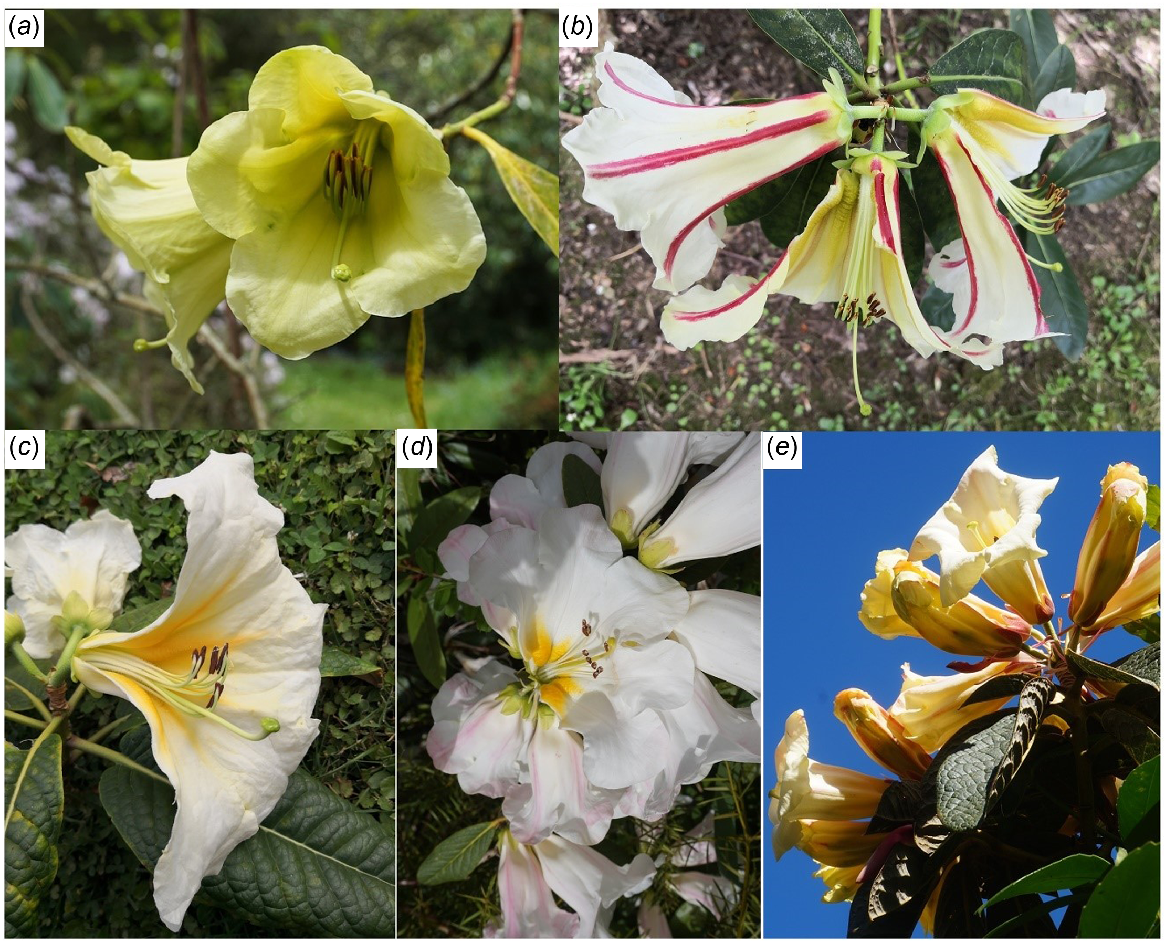
Accessions were selected to provide a range of different threatened levels, depending on their availability and accessibility in nearby gardens, blooming period, as well as lack of information on pollination compatibility of the species. Pollen was collected from, and pollinations were performed on, plants cultivated in the following four gardens in New Zealand: Omahuri (OM; private garden in Manawatū region), Cross Hills Gardens (CH; private garden in Manawatū region), Heritage Park (HP; public garden in Manawatū region) and Pukeiti Gardens (PK; public garden in Taranaki region).
Controlled pollination
Flowers were first emasculated by removing anthers immediately before anthesis, or at anthesis. Surgical tape (micropore tape) was used to create a cover around the stigma to prevent contamination from self-pollen or insect-borne foreign pollen. Both selfing and intraspecific crosses were made 5–7 days after anthesis when the stigma presented a visible exudate indicating receptivity, on at least three flowers from each plant (Table 1). Fresh pollen was used for self-pollination. For intraspecific crosses, mature and fresh anthers were collected before dehiscence, dried and preserved in silica gel at 4°C until pollination (Fig. 2a), because of non-overlapping flowering time of accessions or accessions being located in different gardens. Pollinations were performed between 10:00 am and 12:00 pm on sunny days in November 2021 (spring) or January 2022 (summer), in New Zealand, depending on the blooming period of different accessions. After the micropore tape was carefully removed from around the stigma, the receptive stigma was pollinated by being dipped either in a medical capsule of pollen grains released from the dehisced anthers (for outcross) or directly with dehiscing anthers (for selfing) (Fig. 2a). Pollinated stigmas were covered with surgical tape to stop pollen contamination from other sources while the stigmas were still fertile. Each pollinated flower was tagged with the parental combination (or selfing) and pollination date.
Controlled pollination. (a) Pollen grains preserved in silica gel; (b) stigma taped and labelled after hand-pollination; and (c) harvested seed capsules.
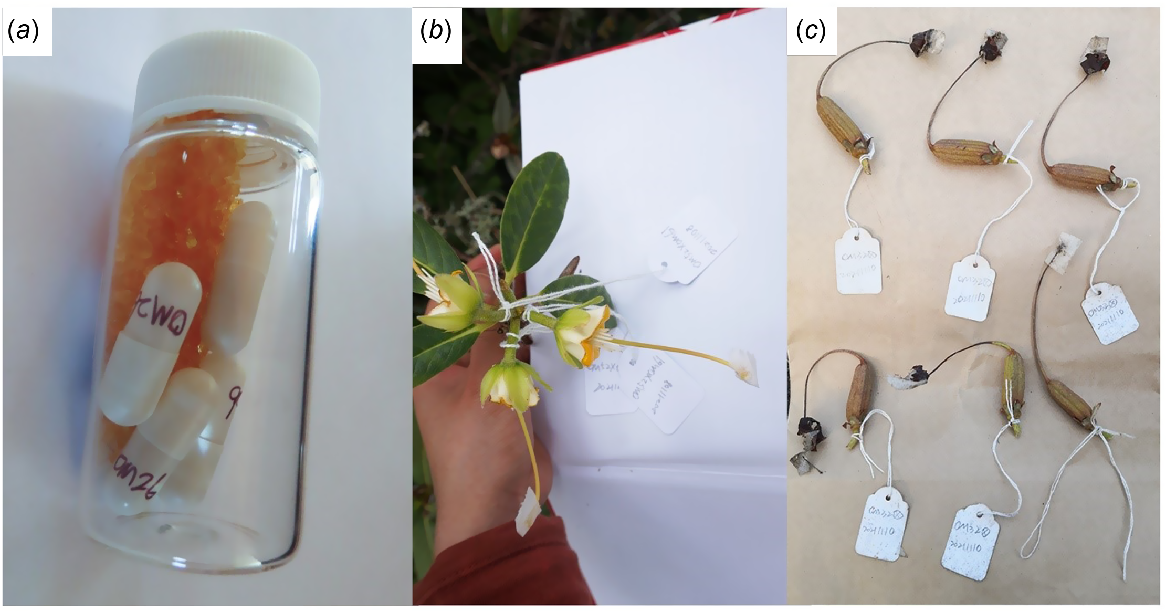
Pollinations were replicated on at least three flowers per plant and on two plants for each selfing or intraspecific cross (Fig. 2, Table 1). Because of different flowering times of plants, the limited availability of pollen and geographical distance between different accessions, crosses were made only one-way rather than reciprocally, or only intraspecific crosses were made, without selfing for some accessions. Details of plant accessions, pollination sets and the number of pollinated flowers per plant are shown in Table 1.
Harvest and storage of seed capsules
Seed capsules were harvested before dehiscing when they were turning brown (ripe), ~4 months post-pollination. The seed set was recorded (Table 1). The seed capsules, harvested separately in paper bags, were stored at room temperature (~20°C) for ~6 months. After they ripened (i.e. most had dehisced), the capsules were shifted to a cold room (~4°C) until seed germination experiments were conducted (within 1 year).
Seed viability
Seed capsules were cracked by hand and all seeds shaken off, then filtered with a sieve (355 μm) to remove debris. Seed weight was measured for each of three random capsules per pollination. For each capsule, five lots of 50 seeds were weighed; the mean weight was used to calculate thousand-seed weight and to determine the number of seeds per capsule (Fig. 3).
Seeds from each pollination were germinated to examine their viability. The harvested seeds of each representative capsule were divided into three subsets and sown for the germination test. First, two subsets of seeds were tested to identify the possible impact of X-ray scanning on the germination of Rhododendron seeds. For the ‘X-ray’ group, seeds were X-rayed to confirm seed fill before sowing, for ~7 s with a dosage of 31.0 kV, 0.35 mA (UltraFocus 60; Faxitron Bioptics LLC, Tuscon, AZ, USA).
Second, seeds in both the ‘X-ray’ and ‘Untreated’ groups were germinated. For each group, three replicates (capsules), each with 50 randomly selected seeds, were sown on wet blotting paper in a sealed transparent box. All seeds were incubated in a plant growth chamber (Conviron Adaptis CMP6010; Manitoba, Canada) to promote germination. The thermoperiod was set to 15/25°C and 8 h photoperiod at ~6 μmol m−2 s−1 photosynthetic photon flux density (PPFD) provided by cool fluorescent tubes, following the optimal germination conditions identified for other Rhododendron species (Tiwari and Chauhan 2007; Lin and Wang 2017). Seeds were watered and seed germination was recorded weekly for 7 weeks, after which no further seeds germinated. Seeds showing radicle emergence were recorded as germinated. The percentage of germinated seeds from the total number of seeds sown was calculated.
Following mould development during seed germination in the ‘Untreated’ and ‘X-ray’ groups as described above, another subset of seeds from the corresponding capsules tested were treated with 1 g L−1 Ridomil fungicide (Gold® MZ WG; Syngenta Crop Protection Ltd, Auckland, New Zealand) during sowing. Seeds in this ‘Ridomil’ group were also sprayed with water weekly, and germinated under the same condition as the other two groups.
Data were analysed using SAS® 9.4 TS1M8 (SAS Institute Inc. 2023) with a rejection level set at P = 0.05. Two separate significance analyses were performed to compare the seed germination results, namely, one comparing among treatments (i.e. ‘Untreated’, ‘X-ray’, ‘Ridomil’) for each pollination, the other comparing between pollinations (i.e. self, outcross) for each taxon under the same seed germination treatment. To perform these analyses, a generalised linear model (GLIMMIX procedure) was used with a binomial distribution, followed by a Logit function. A Tukey–Kramer test was applied for multiple comparisons. The number of seeds and number of germinated seeds were ‘x + 1’ transformed for valid significance analysis, because some data were zero for germination.
To examine whether seed fill shown by radioscopy could predict germination, a validation test was performed to investigate the correlation between seed fill (determined by X-ray scanning) and germination percentage. Six lots of 25 seeds each from different pollinations were scanned under X-ray, then moved directly onto blotting paper in the corresponding position. Seeds were sprayed with Ridomil prior to sowing to limit fungal infection. X-ray settings and germination conditions were the same as described in the ‘Seed germination’ section. As a comparison, seeds of each pollination (self/outcross) were also observed under a stereo microscope (Olympus) to assess maturity and fullness.
Results
Seed set and seed weight
Most pollinations, both self and outcross, produced capsule(s) with seeds in the five experimental taxa (Table 1). The one exception was R. excellens, which produced no seed; although the self and outcross manipulations had one capsule set each, there was no seed in the capsule. In other taxa, most pollinations produced seeds successfully, with all pollinated flowers setting capsules (e.g. R. lindleyi).
Thousand-seed weight of the four taxa (except for R. excellens that did not produce seeds) ranged from 0.0704 to 0.1139 g (Table 1). The thousand-seed weight of R. dalhousiae (including the two subspecies) was higher than that of R. lindleyi and R. nuttallii. For R. dalhousiae var. dalhousiae, R.lindleyi and R. nuttallii, the thousand-seed weight arising from self-pollination was lower than that of the outcross. In contrast, for R. dalhousiae var. rhabdotum, the thousand-seed weight of the outcross was lower.
The approximate seed number in each capsule showed different ranges in the four taxa measured (Table 1). Whereas seed numbers in the capsules of the two R. dalhousiae subspecies were similar (3512–4580), seed capsules of R. lindleyi had fewer (2377–2400) seeds, and those of R. nuttallii had as many as 5428–7522 seeds.
Seed fill and germination
Fig. 4 shows the seed fill observed under stereo microscope and X-ray, the germination test and a developing seedling. Seeds reached peak germination mostly within 6 weeks after sowing. Few seeds germinated in the seventh week, whereas some seeds that germinated earlier had started to die. Treatment with fungicide (‘Ridomil’ group) prevented the fungal growth that occurred in the ‘Untreated’ and ‘X-ray’ groups.
Example of the result from experiments on seed fill and germination (Rhododendron dalhousiae var. dalhousiae OM32 × CH15). (a) Seed morphology under stereo microscope (scale bar: 1 mm); (b) X-ray scan showing the bright seed and faint seed appendage including the ‘wing’ structure and ‘tails’ (micropylar and chalazal ends); (c) germination test (example of the ‘Untreated’ group); and (d) seedlings with emerged cotyledons. Full triangles in (a) and (b) point to examples of full seeds, whereas empty triangles point to partially full seeds; empty seeds were invisible in the X-ray images.
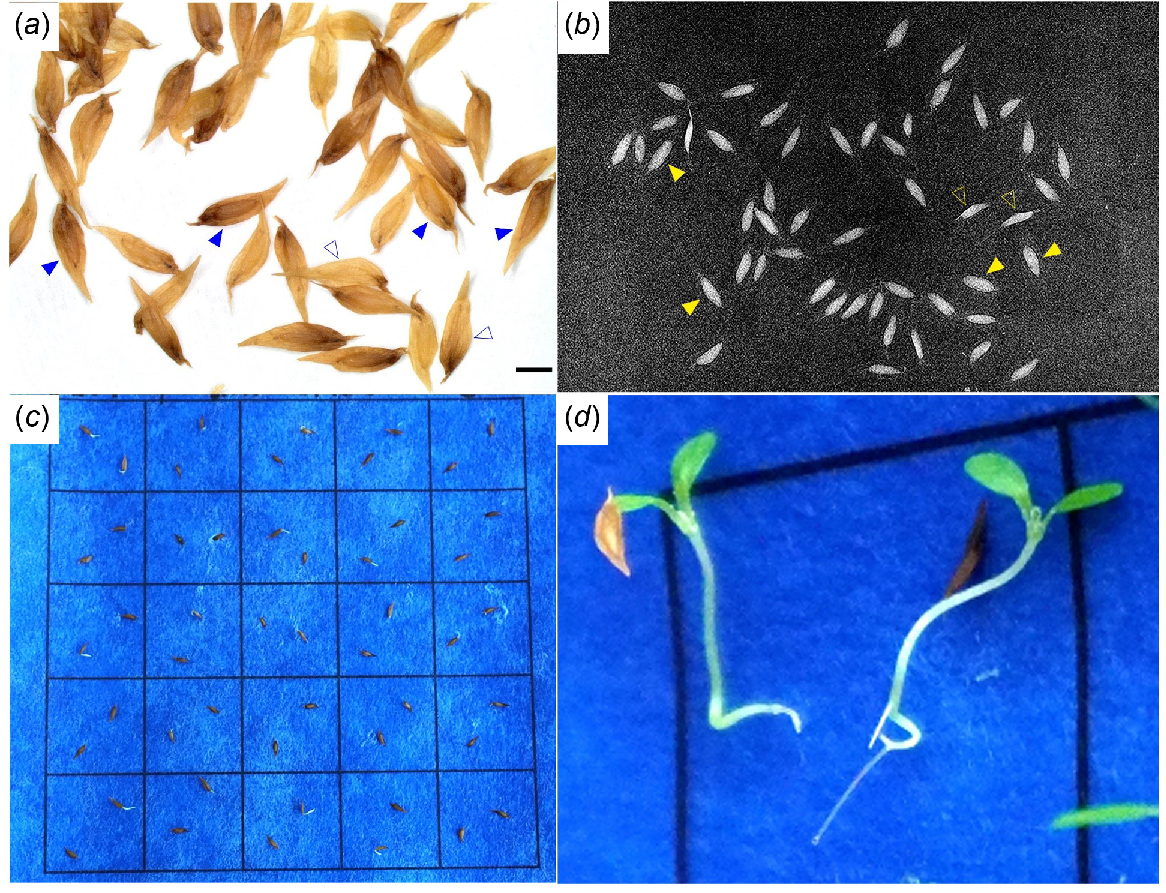
The final germination percentages differed among taxa and in some cases differed significantly among pollination treatments (Table 2). For R. dalhousiae var. dalhousiae, seeds from the outcrosses had a significantly higher germination percentage than those from the self-pollination treatments (P = 0.0018, 0.0014, 0.0067 for the ‘Untreated’, ‘Ridomil’, ‘X-ray’ groups respectively). However, for R. dalhousiae var. rhabdotum, the selfed seeds had a significantly higher germination percentage than did outcrossed seeds (P = 0.0136, 0.0315, 0.0210). In R. lindleyi, seeds from selfed and outcrossed showed similar germination percentages (P = 0.0809, 0.2411), except for the seeds treated with Ridomil (P = 0.0370). In R. nuttallii, seeds from OM51 selfed exhibited a significantly higher germination percentage than those from OM50 selfed or outcrossed (P < 0.0001 for all three treatments).
Taxon | Pollination | Treatment | F(d.f.) | P | |||
---|---|---|---|---|---|---|---|
Untreated | Ridomil | X-ray | |||||
R. dalhousiae var. dalhousiae | OM32 | 47.7 ± 6.4bα | 38.7 ± 13.3bα | 52.7 ± 21.7bα | 2.95(2,6) | 0.1283 | |
OM32 × CH15 | 92.5 ± 2.5aα | 86.7 ± 1.8aαβ | 81.8 ± 1.2aβ | 3.64(2,6) | 0.0921 | ||
F(d.f.) | 54.20(1,4) | 62.70(1,4) | 26.69(1,4) | ||||
P | 0.0018 | 0.0014 | 0.0067 | ||||
R. dalhousiae var. rhabdotum | OM29 | 71.3 ± 9.7aα | 59.7 ± 5.4aα | 65.6 ± 2.0aα | 2.19(2,6) | 0.1933 | |
OM29 × OM26 | 72.7 ± 5.7aα | 38.7 ± 8.5bβ | 48.0 ± 14.2bαβ | 17.43(2,6) | 0.0032 | ||
OM26 × OM29 | 50.7 ± 2.4bα | 47.5 ± 3.4abα | 43.3 ± 8.7bα | 0.80(2,6) | 0.4928 | ||
F(d.f.) | 9.58(2,6) | 6.50(2,6) | 7.88(2,6) | ||||
P | 0.0136 | 0.0315 | 0.0210 | ||||
R. lindleyi | OM52 | 36.7 ± 20.3aα | 34.0 ± 18.1aα | 32.0 ± 16.4aα | 0.32(2,6) | 0.7381 | |
OM52 × PK39 | 24.0 ± 15.9aα | 17.8 ± 9.1bα | 25.1 ± 17.7aα | 1.20(2,6) | 0.3632 | ||
F(d.f.) | 5.40(1,4) | 9.48(1,4) | 1.89(1,4) | ||||
P | 0.0809 | 0.0370 | 0.2411 | ||||
R. nuttallii | OM50 | 5.3 ± 5.3bα | 1.3 ± 1.3bα | 6.7 ± 6.7bα | 1.80(2,6) | 0.2447 | |
OM51 | 64.7 ± 3.5aα | 65.3 ± 6.8aα | 52.3 ± 7.9aα | 3.28(2,6) | 0.1091 | ||
OM50 × OM51 | 2.1 ± 2.1bα | 0bα | 0.7 ± 0.7bcα | 0.56(2,6) | 0.5976 | ||
OM50 × CH16 | 0bα | 0bα | 0cα | 0(2,6) | 1.0000 | ||
F(d.f.) | 54.22(3,8) | 51.51(3,8) | 40.53(3,8) | ||||
P | <0.0001 | <0.0001 | <0.0001 |
In the ‘Pollination’ column, self is represented by ‘’ and outcross is ‘(maternal parent) × (paternal parent)’. Within each taxon, values (means ± s.e.) followed by the same letter in each column (a, b, c) comparing pollinations or the same Greek letter (α, β) in each row comparing treatments are not significantly different (P > 0.05), using a Tukey–Kramer test. The F value represents statistical significance of the test. The (d.f.) values represent numbers of degrees of freedom, in the model and associated with the model errors, respectively. Stigmas were pollinated by being dipped either in stored pollen (for outcross) or directly with fresh pollen (for selfing).
The germination percentage was also compared among different treatments (‘Untreated’, ‘Ridomil’, ‘X-ray’) for each pollination. In general, germination of seeds scanned under X-ray or treated with Ridomil were not significantly different from the ‘Untreated’ group. However, for two intraspecific outcrosses, R. dalhousiae var. dalhousiae OM32 × CH15 (P = 0.0921) and R. dalhousiae var. rhabdotum OM29 × OM26 (P = 0.0032, significant), seed germination of the ‘Ridomil’ and ‘X-ray’ groups was lower than for the ‘Untreated’ group (Table 2).
X-ray seed quality for seed germination
A set of seeds was sown in the same position as under X-ray, to investigate the correlation between seed fullness shown by radiography and seed germination. Seeds that were identified as empty in the X-ray image (invisible) did not germinate (e.g. Fig. 5a, right: A3). When the X-ray image indicated that a seed was partially full (embryo faintly visible or deformed), the seed in some cases did not germinate; this was the case especially for R. nuttallii OM50 × OM51 (Fig. 5f). Other pollinations with partially full seeds exhibited poor growth (unable to develop cotyledons and/or exhibiting browning) (e.g. Fig. 5e, right: C3). Even if germination occurred, some of the resultant seedlings were not normal (e.g. Fig. 5c, right: B4). Additionally, not all the seeds that were identified ‘full’ under X-ray germinated (e.g. Fig. 5d, right: A4).
Validation test of Rhododendron seeds comparing fullness under the stereo microscope (left) and by X-ray imaging (middle) with seed germination (right). Position of seeds in the X-ray image corresponds to that in the germination in the same row. Every row represents seeds from the same pollination: (a) R. dalhousiae var. dalhousiae OM32; (b) R. dalhousiae var. rhabdotum OM29; (c) R. lindleyi OM52; (d) R. lindleyi OM52 × PK39; (e) R. nuttallii OM51; and (f) R. nuttallii OM50 × OM51. Self is represented by ‘’ and outcross is ‘(maternal parent) × (paternal parent)’. Scale bar: 1 mm.
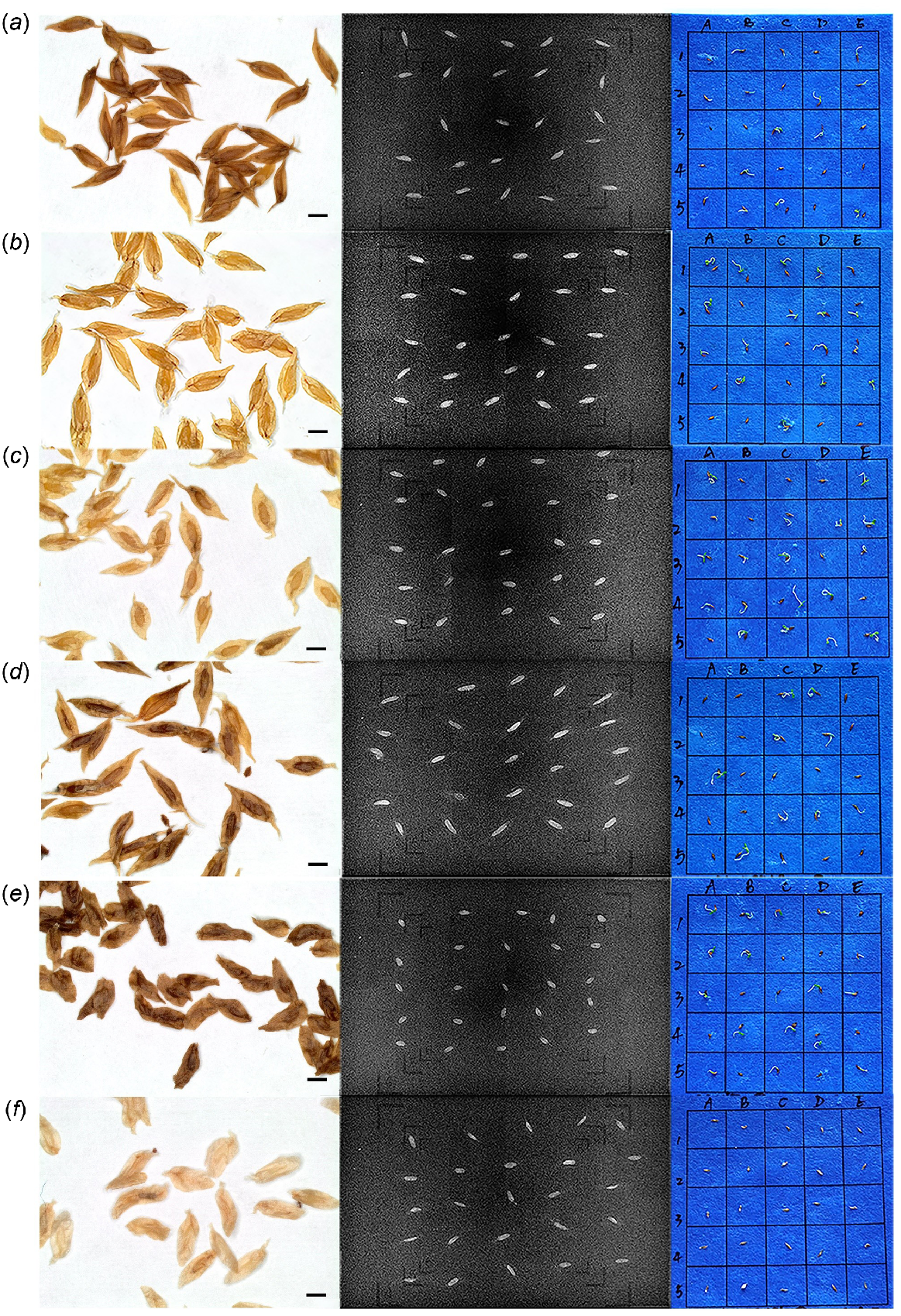
The stereo microscopy image was also used as a reference for seed maturity and fullness, because the structure of seeds, including the embryo and the appendage (wing structure) could be easily observed. Seeds of R. nuttallii (Fig. 5e, f) had a smaller embryo and a greater area of appendage than did those of R. dalhousiae and R. lindleyi. The seeds of R. nuttallii OM50 × OM51 (Fig. 5f) showed a lower maturity (as indicated by the pale colour) and an embryo that was not so well developed, compared with OM51 selfed (Fig. 5e).
Discussion
Intraspecific compatibility of the examined species
This study examined the intraspecific compatibility of five Rhododendron taxa in subsection Maddenia, by using both fruit set and seed germination as measures of compatibility. The taxa studied displayed differing levels of intraspecific compatibility, and the compatibility between self- and cross-pollinations varied.
R. dalhousiae var. dalhousiae (Least Concern) in this study showed a lower self-compatibility than did the intraspecific cross, whereas R. dalhousiae var. rhabdotum (Vulnerable) was overall the opposite. Rouse et al. (1993) identified the partial self-compatibility of R. dalhousiae, which is consistent with our results. The two varieties show distinction on various aspects. R. dalhousiae var. dalhousiae flowers earlier (December in New Zealand) than does R. dalhousiae var. rhabdotum (January). They also show distinct separation in molecular phylogeny (L Hu, JA Tate, SE Gardiner, MB MacKay, unpubl. data), and their natural distributions show a certain degree of difference, although greatly overlapping in Bhutan and China (southern Xizang). R. dalhousiae var. rhabdotum has a narrower distribution and spreads farther east to Arunachal Pradesh in India, whereas R. dalhousiae var. dalhousiae spreads wider and farther west to north-eastern India (Sikkim and West Bengal) and eastern Nepal (Hu et al. 2024). Because R. dalhousiae var. rhabdotum is both morphologically recognisable and phylogenetically distinct from R. dalhousiae var. dalhousiae (L Hu, JA Tate, SE Gardiner, MB MacKay, unpubl. data), collections of these two varieties should always be differentiated and intraspecific compatibility evaluated separately for their conservation. This differentiation may be neglected in botanic garden collections, because accessions from both varieties could have been recorded simply as R. dalhousiae.
In our study, R. excellens (Vulnerable) was unable to produce seeds from selfing or intraspecific outcrossing, even though capsules formed. This is consistent with the low self-compatibility reported in previous studies where the numbers of seeds produced were significantly lower than from intraspecific outcrosses, and a varying seed set was observed in the intraspecific outcross pollinations, including a capsule with no seeds (Tian 2011; Cai et al. 2022). The observed low compatibility may be the grounds for rating of R. excellens as ‘Vulnerable’ in the wild (Gibbs et al. 2011), because the wild populations are small and would face difficulties in producing offspring. The lack of seed produced by outcrossing may result from the individuals crossed being too closely related to set seed; confirmation of this requires analysis to determine the genetic distance between the accessions used. The lack of seed set for the two crosses might also result from low pollen viability because pollen was not used fresh, although early investigation showed that dehydrated Rhododendron pollen remained viable for successful crosses after being stored in the fridge for 1–3 years (Kehr 1977). The possible inbreeding depression of R. excellens requires particular attention for conservation of this species both in situ and ex situ. Furthermore, because R. excellens has a close relationship with R. liliiflorum (Least Concern) and R. levinei (Near Threatened) (L Hu, JA Tate, SE Gardiner, MB MacKay, unpubl. data), morphological identification should be rigorous during field sampling.
Rhododendron lindleyi (Least Concern) showed partial self- and cross-compatibilities, but a smaller seed number per capsule than for other species in the present study. However, this species did not produce seeds following self-pollination of multiple plants in an earlier study (Rouse et al. 1993). Further investigation is needed to elucidate the breeding system of R. lindleyi, as well as to verify its possible paraphyly observed in Hu et al. (unpubl. data), in the context of species conservation.
For R. nuttallii (Near Threatened), self-compatibility was observed to be higher than for the intraspecific cross, although the low seed germination observed may also have resulted from seed immaturity according to the microscope images (Fig. 5e, f). Although this study showed that seed from the capsules collected when not fully mature could germinate as well as those from the mature capsules, we do suggest that capsules should be harvested with frequent (e.g. weekly) observation until they turn as brown as possible prior to dehiscence (i.e. fully mature). The partial compatibility of R. nuttallii is consistent with the degree of self-compatibility observed in prior work (Rouse et al. 1993). The difference in seed production (Table 2) through self-pollination between the two wild accessions of R. nuttallii (OM50 from northern Viet Nam and OM51 from China, Table 1) may also indicate different levels of compatibility in wild populations from different geographic ranges (Roda and Hopkins 2019). This suggests that the diversity conservation of R. nuttallii in China should prioritise more wild sampling for ex situ collections because of its low compatibility, whereas wild collections in Viet Nam might be used to produce seeds that could be returned to the wild to boost diversity. Although the two accessions are located adjacent to each other in the local ex situ garden, they flower at different times (OM51 flowered approximately 2 weeks earlier, as observed, in November 2021 in New Zealand). In addition, the two samples (OM50, OM51) occupy different subclades of a molecular phylogeny, reflecting their genetic difference (L Hu, JA Tate, SE Gardiner, MB MacKay, unpubl. data). These variations in pollination compatibility, flowering time, geographic origin and molecular phylogeny in different accessions of the same species/taxon, all characterise intraspecific diversity.
In summary, to conserve threatened species, our results suggest that selfing and outcrossing could be beneficial for maintaining genetic diversity of the germplasm of R. dalhousiae var. rhabdotum (Vulnerable) and R. nuttallii (Near Threatened). In contrast, for the self-incompatible R. excellens (Vulnerable) (consistent with its varied outcross compatibility (possibly zero seed set) reported in previous studies; Tian 2011; Cai et al. 2022), more wild sampling rather than strategic breeding, should be scheduled to increase the genetic diversity of this species conserved in ex situ collections.
Testing seed viability for Rhododendron species
For Rhododendron species, limited information on seed weight and germination is available from the Seed Information Database (https://ser-sid.org/). Data from the present study have filled this knowledge gap for the four examined taxa, namely, R. dalhousiae var. dalhousiae, R. dalhousiae var. rhabdotum, R. lindleyi and R. nuttallii, and provided information for future research.
Different studies have reported that Rhododendron seeds can germinate when mature, without any pre-treatment (Williams et al. 1990; Glenn et al. 1999; Tiwari and Chauhan 2007; Lin and Wang 2017). Rhododendron seeds retain viability for 1 year or often longer without special storage conditions (apart from chilling and dryness), and appropriate temperature, water, humidity and light regimes are necessary for germination (Williams et al. 1990). In the present study, where the incubation conditions were set at an optimal thermoperiod (15/25°C) and photoperiod (16/8 h) according to previous studies (Glenn et al. 1999; Tiwari and Chauhan 2007; Tian 2011; Lin and Wang 2017), the visibly viable seeds that did not germinate were likely to be affected by other factors, such as seed maturity or shallow seed dormancy (Zhou et al. 2013). This was observed for seeds from R. nuttallii OM50 × OM51, for which the seeds showed pale brown colour (suggesting immaturity) in the microscopy images, and none of them germinated, although they were observed to be viable in the X-ray image (Fig. 5). Our experiment demonstrated the limitation of X-ray, in that it helps identify whether seeds are filled, but not whether they are truly viable or have any degree of dormancy.
A seed staining method such as the common tetrozolium test, used as a back-up method in seed banking (ISTA 2023), could be performed to confirm the viability of these seeds that did not germinate but appeared visible in the X-ray image. However, applicability of the tetrazolium test for Rhododendron seeds awaits investigation to provide reference data, because there is very little information available except for a single study (Kumar and Sharma 2020). In addition, fullness tested from seed staining has shown low reliability for prediction of seed germination in several studies in the Orchidaceae (Lemay et al. 2015; Pradhan et al. 2022). In future Rhododendron seed germination studies, these two issues should be considered when using the seed-staining test.
The present study showed that the X-ray image can assist in identifying ‘dead’ Rhododendron seeds (invisible under X-ray) that will not germinate, but it has a low accuracy for predicting viability through differential seed fullness (Figs 4, 5). The difficulty in identifying the internal structure (i.e. embryo and endosperm) of Rhododendron seeds made it unrealistic to count the number of filled seeds; we were therefore unable to collect more reliable data for viable seeds and analyse its correlation with germination percentage. The small size (~1.5 mm long by ~0.5 mm wide, Fig. 5) of the Rhododendron seeds is likely to be a main cause, although future tests could be optimised by scanning fewer seeds at a time (25 seeds used in this study). The accuracy of X-ray imaging has been reported to depend on seed size and structure (Martyn Yenson et al. 2021), and has been successful for species in various groups, including Brassicaceae species, Leucaena (Fabaceae) or Pinus (Pinaceae) (Tay and Hu 2005; Medeiros et al. 2018; Karamysheva et al. 2020; Musaev et al. 2022). Other factors such as water content may also affect the image quality (Gagliardi and Marcos-Filho 2011). There remains the knowledge gap regarding adjustment of X-ray scanning in achieving higher image quality for plant seeds in general.
Williams et al. (1990) mentioned the high risk of fungal diseases of Rhododendron seedlings during the emergence of the cotyledons. In this study, the application of fungicide when sowing seeds effectively prevented infection, with generally little impact on the germination percentage; it is unlikely that seedlings would have survived beyond a few weeks without this treatment. For some orchid seeds, fungicides were reported to inhibit the growth in vitro of shoot-tip explants, but not seed germination (Brown et al. 1982). Because Ridomil did inhibit seed germination from the cross R. dalhousiae var. rhabdotum OM29 × OM26, the inhibition by fungicide treatment on the growth of seedlings, owing to a decrease of beneficial endophytes associated with root systems (Zhang et al. 2009; Ayesha et al. 2021), could be further investigated on other Rhododendron species. Nevertheless, as this study has identified, fungicide is effective for protecting Rhododendron seeds/seedlings from infection. Therefore, the application of fungicide is a necessary precaution in the raising of healthy offspring from intraspecific pollinations in botanic garden practice.
Maintaining germplasm in botanic garden collections of Rhododendron
In germplasm conservation, controlled pollination is an important tool used to maintain diversity of ex situ accessions, particularly in genera where open pollination cannot be used because of the potential for cross-hybridisation (Diaz-Martin et al. 2023). For Rhododendron species in particular, existing botanic garden collections can be valuable for maintaining conserved germplasm, because the wild populations of many species are either very limited, or under considerable threat (Gibbs et al. 2011). For these species, ex situ collections provide back-up material for threatened species, and collection management should include maintenance of diversity through sexual reproduction via strategic breeding.
Coordinated breeding, desirably across multiple botanic gardens, helps maximise the genetic diversity of species conserved in ex situ collections. Under the concept of ‘metacollection’, this strategy has been suggested by studies of other plants (Foster et al. 2022; Diaz-Martin et al. 2023). There are three main issues to consider in controlled intraspecific pollination for ex situ collections of Rhododendron. First, coordinated breeding is recommended to be conducted on the basis of knowledge of wild origin and assessment of genetic diversity in the conserved collections to optimise breeding pairs. However, current ex situ collections of Rhododendron require better data management and wild representation of the collections remains greatly unknown (Hu et al. 2024). Second, pollen collection and storage are required to enable intraspecific pollination across ex situ collections. This addresses more concern about coordinated pollen sampling through the conservation network, to collect and store pollen from various sites for diversity studies, considering dehydrated Rhododendron pollen could remain viable for years when chilled (Kehr 1977; Williams et al. 1990). Third, flowering synchrony of different accessions of the same species is an important factor when selecting the parental plants, with pollen storage being required if there is nil or limited synchrony (Rouse 1984). Otherwise, the plant flowering later can be used only for pollen collection in that season, i.e. as a paternal parent. Its use as a maternal parent must be in the next blooming season, receiving pollen collected in the prior season.
The varied compatibility among taxa and among different accessions of one taxon, together with variation reflected from other studied aspects for Rhododendron such as wild distribution, morphology, ploidy level and molecular phylogeny (Hu et al. 2023; Hu et al. 2024; L Hu, JA Tate, SE Gardiner, MB MacKay, unpubl. data), all represent intraspecific diversity. These factors are essential for developing effective strategies, while prioritising the threatened species to support biodiversity conservation both in situ and ex situ.
Conclusions
This study investigated the intraspecific compatibility of five taxa in Rhododendron subsection Maddenia that have been classified in different IUCN Red List categories. Seed set and germination percentages differed significantly among taxa and, in some cases, between selfed and outcrossed treatments within taxa. These results suggest that conservation actions should be considered on a taxon-by-taxon basis. Both radiography and microscopy were found to be useful in identifying seed fill, but seed fill was not a useful predictor of germination percentage. Future work should focus on evaluating more accessions (preferably of wild origin) to confirm the intraspecific compatibility of related taxa. In this study, X-ray radiography was inconsistent in predicting viability of Rhododendron seeds. It may be possible to improve application of this technology and further investigation is warranted for seed banking. Information on intraspecific compatibility and seed characterisation for the species examined in this study helps inform conservation strategies. It demonstrates how various aspects of knowledge about a species (conservation status, diversity captured in ex situ collections, taxonomic distinction and pollination compatibility) can be incorporated to choose the optimised strategy for species conservation in botanic garden practice.
Data availability
All data in the present study are included in this paper or available from the corresponding author upon request.
Declaration of funding
This work is part of a PhD project supported by the Sir Victor Davies Fund through the Pukeiti Rhododendron Trust, New Zealand. Ling Hu was funded through the China Scholarship Council – Massey University PhD Scholars Program.
Acknowledgements
The authors thank Andrew Brooker for helping with pollen collection at Pukeiti Gardens (Taranaki region), and Heritage Park and Cross Hills Gardens (Manawatū region) for access to the plant accessions in New Zealand. Ling Hu thanks Gina Aubia and Craig McGill (New Zealand Indigenous Flora Seed Bank) for instructions on X-ray scanning and seed-germination experiment, and Dr Xiong-zhao He for consultation about the statistical analysis (Massey University).
References
Ayesha MS, Suryanarayanan TS, Nataraja KN, Prasad SR, Shaanker RU (2021) Seed treatment with systemic fungicides: time for review. Frontiers in Plant Science 12, 654512.
| Crossref | Google Scholar |
Basnett S, Ganesan R (2022) A comprehensive review on the taxonomy, ecology, reproductive biology, economic importance and conservation status of Indian Himalayan Rhododendrons. The Botanical Review 88, 505-544.
| Crossref | Google Scholar |
Bowers CG (1930) The development of pollen and viscin strands in Rhododendron catawbiense. Bulletin of the Torrey Botanical Club 57, 285-313.
| Crossref | Google Scholar |
Brown DM, Groom CL, Cvitanik M, Brown M, Cooper JL, Arditti J (1982) Effects of fungicides and bactericides on orchid seed germination and shoot tip cultures in vitro. Plant Cell, Tissue and Organ Culture 1, 165-180.
| Crossref | Google Scholar |
Cai B, Liu CQ, Huang SX (2022) Exploring wild plant conservation from the perspective of reproductive biology: a case study of Rhododendron excellens. In ‘Proceedings of the 4th International Conference on Biomedical Engineering and Bioinformatics (ICBEB 2022)’. pp. 160–165. (SCITEPRESS – Science and Technology Publications)
Diaz-Martin Z, Fant J, Havens K, Cinea W, Tucker Lima JM, Griffith MP (2023) Current management practices do not adequately safeguard endangered plant species in conservation collections. Biological Conservation 280, 109955.
| Crossref | Google Scholar |
Forgiarini C, Parzefall F, Reisch C (2023) The impact of ex situ cultivation on the genetic variation of endangered plant species – Implications for restoration. Biological Conservation 284, 110221.
| Crossref | Google Scholar |
Foster JA, Walsh SK, Havens K, Kramer AT, Fant JB (2022) Supporting long-term sustainability of ex situ collections using a pedigree-based population management approach. Applications in Plant Sciences 10, e11491.
| Crossref | Google Scholar | PubMed |
Frodin DG (2004) History and concepts of big plant genera. Taxon 53, 753-776.
| Crossref | Google Scholar |
Gagliardi B, Marcos-Filho J (2011) Relationship between germination and bell pepper seed structure assessed by the X-ray test. Scientia Agricola 68, 411-416.
| Crossref | Google Scholar |
Glenn CT, Blazich FA, Warren SL (1999) Secondary seed dormancy of Rhododendron catawbiense and Rhododendron maximum. Journal of Environmental Horticulture 17, 1-4.
| Crossref | Google Scholar |
Gratzfeld J (2017) What is conservation horticulture? BGjournal 14, 14-17.
| Google Scholar |
Heywood VH (2017) The future of plant conservation and the role of botanic gardens. Plant Diversity 39, 309-313.
| Crossref | Google Scholar | PubMed |
Hollingsworth PM, Squirrell J, Hollingsworth ML, Richards AJ, Bateman RM (2006) Taxonomic complexity, conservation and recurrent origins of self-pollination in Epipactis (Orchidaceae). In ‘Current taxonomic research on the British and European flora’. (Eds JP Bailey, RG Ellis) pp. 27–44. (Botanical Society of the British Isles: London, UK)
Hu L, Tate JA, Gardiner SE, MacKay M (2023) Ploidy variation in Rhododendron subsection Maddenia and its implications for conservation. AoB Plants 15, plad016.
| Google Scholar |
Hu L, MacKay M, Gardiner SE, Tate JA (2024) Rhododendron diversity conservation in global botanic gardens: a case study of Maddenia species. Oryx, in press. https://doi.org/10.1017/S0030605324000759
Jamieson G (2021) Subsections Maddenia, Boothia and Edgeworthia in section Rhododendron subgenus Rhododendron. Rhododendrons International 6, 32-53.
| Google Scholar |
Karamysheva A, Trofimuk L, Priyatkin N, Arkhipov M, Gusakova L, Sshukina P, Staroverov N, Potrakhov N (2020) Comparative study of the fullness of dwarf Siberian pine seeds Pinus pumila (Pall.) Regel from places of natural growth and collected from plants introduced in northwestern Russia by microfocus X-ray radiography to predict their sowing qualities. Biological Communications 65, 297-306.
| Crossref | Google Scholar |
Kehr A (1977) Storage of pollen and seed. Journal American Rhododendron Society 31(2), Available at https://scholar.lib.vt.edu/ejournals/JARS/v31n2/v31n2-storage.html.
| Google Scholar |
Kumar V, Sharma R (2020) Seed germiability, viability and longevity of Rhododendron arboreum var. arboreum Sm. International Journal of Chemical Studies 8, 2505-2517.
| Google Scholar |
Lemay M-A, De Vriendt L, Pellerin S, Poulin M (2015) Ex situ germination as a method for seed viability assessment in a peatland orchid, Platanthera blephariglottis. American Journal of Botany 102, 390-395.
| Crossref | Google Scholar | PubMed |
Li S, Sun W, Ma Y (2018a) Current conservation status and reproductive biology of the giant tree Rhododendron in China. Nordic Journal of Botany 36, 1-9.
| Crossref | Google Scholar |
Li T, Liu X, Li Z, Ma H, Wan Y, Liu X, Fu L (2018b) Study on reproductive biology of Rhododendron longipedicellatum: a newly discovered and special threatened plant surviving in limestone habitat in Southeast Yunnan, China. Frontiers in Plant Science 9, 33.
| Crossref | Google Scholar |
Lin L-C, Wang C-S (2017) Influence of light intensity and photoperiod on the seed germination of four Rhododendron species in Taiwan. Pakistan Journal of Biological Sciences 20, 253-259.
| Crossref | Google Scholar | PubMed |
Ma Y-P, Milne RI, Zhang C-Q, Yang J-B (2010) Unusual patterns of hybridization involving a narrow endemic Rhododendron species (Ericaceae) in Yunnan, China. American Journal of Botany 97, 1749-1757.
| Crossref | Google Scholar | PubMed |
Ma Y-P, Xie W-J, Sun W-B, Marczewski T (2016) Strong reproductive isolation despite occasional hybridization between a widely distributed and a narrow endemic Rhododendron species. Scientific Reports 6, 19146.
| Crossref | Google Scholar | PubMed |
Maunder M, Guerrant E, Havens K, Dixon KW (2004a) Realizing the full potential of ex situ contributions to global plant conservation. In ‘Ex situ plant conservation: supporting species survival in the wild’. (Eds EO Guerrant, K Havens-Young, M Maunder) pp. 389–418. (Island Press: Washington, DC, USA)
Maunder M, Hughes C, Hawkins JA, Culham A (2004b) Hybridization in ex situ plant collections: conservation concerns, liabilities, and opportunities. In ‘Ex situ plant conservation: supporting species survival in the wild’. (Eds EO Guerrant, K Havens-Young, M Maunder) pp. 325–364. (Island Press: Washington, DC, USA)
Medeiros AD, Araújo JO, León MJZ, Silva LJ, Dias DCFS (2018) Parameters based on X-ray images to assess the physical and physiological quality of Leucaena leucocephala seeds. Ciência e Agrotecnologia 42, 643-652.
| Crossref | Google Scholar |
Mejías JA, Arroyo J, Ojeda F (2002) Reproductive ecology of Rhododendron ponticum (Ericaceae) in relict Mediterranean populations. Botanical Journal of the Linnean Society 140, 297-311.
| Crossref | Google Scholar |
Musaev F, Priyatkin N, Potrakhov N, Beletskiy S, Chesnokov Y (2022) Assessment of Brassicaceae seeds quality by X-ray analysis. Horticulturae 8, 29.
| Crossref | Google Scholar |
Okamoto A, Suto K (2004) Cross incompatibility between Rhododendron sect. Tsutsusi species and Rhododendron japonicum (A. Gray) JV Suringar f. flavum Nakai. Journal of the Japanese Society for Horticultural Science 73, 453-459.
| Crossref | Google Scholar |
Okamoto A, Ureshino K (2015) Pre- and Post-fertilization barriers in interspecific hybridization between evergreen azalea species and Rhododendron uwaense H. Hara & T. Yamanaka. The Horticulture Journal 84, 355-364.
| Crossref | Google Scholar |
Palser BF (1986) Rhododendron: an intimate glimpse into the flower. Journal American Rhododendron Society 40(1), Available at https://scholar.lib.vt.edu/ejournals/JARS/v40n1/v40n1-palser.html.
| Google Scholar |
Palser BF, Philipson WR, Philipson MN (1989a) Development of ovule, megagametophyte and early endosperm in representative species of Rhododendron L. (Ericaceae). Botanical Journal of the Linnean Society 101, 363-393.
| Crossref | Google Scholar |
Palser BF, Rouse JL, Williams EG (1989b) Coordinated timetables for megagametophyte development and pollen tube growth in Rhododendron nuttallii from anthesis to early postfertilization. American Journal of Botany 76, 1167-1202.
| Crossref | Google Scholar |
Pradhan N, Fan X, Martini F, Chen H, Liu H, Gao J, Goodale UM (2022) Seed viability testing for research and conservation of epiphytic and terrestrial orchids. Botanical Studies 63, 3.
| Crossref | Google Scholar | PubMed |
Roda F, Hopkins R (2019) Correlated evolution of self and interspecific incompatibility across the range of a Texas wildflower. New Phytologist 221, 553-564.
| Crossref | Google Scholar | PubMed |
Rouse JL, Knox RB, Williams EG (1993) Inter-and intraspecific pollinations involving Rhododendron species. Journal American Rhododendron Society 47(1), Available at https://scholar.lib.vt.edu/ejournals/JARS/v47n1/v47n1-rouse.htm.
| Google Scholar |
Schoen DJ, Brown AH (1991) Intraspecific variation in population gene diversity and effective population size correlates with the mating system in plants. Proceedings of the National Academy of Sciences 88, 4494-4497.
| Crossref | Google Scholar |
Takahashi K, Itino T (2021) Measurement of inbreeding depression in Rhododendron kaempferi: seed production, germination, juvenile survival, and growth. Botany 100, 359-365.
| Crossref | Google Scholar |
Tay D, Hu X (2005) (423) Effect of seed radiography on seed quality. HortScience 40, 1022B-1022.
| Crossref | Google Scholar |
Tiwari ON, Chauhan U (2007) Seed germination studies in Rhododendron maddenii Hook.f. and Rhododendron niveum Hook.f. Indian Journal of Plant Physiology 12, 50-56.
| Google Scholar |
van der Walt K, Alderton-Moss J, Lehnebach CA (2022) Cross-pollination and pollen storage to assist conservation of Metrosideros bartlettii (Myrtaceae), a critically endangered tree from Aotearoa New Zealand. Pacific Conservation Biology 29, 141-152.
| Crossref | Google Scholar |
Volis S (2017) Conservation utility of botanic garden living collections: setting a strategy and appropriate methodology. Plant Diversity 39, 365-372.
| Crossref | Google Scholar | PubMed |
Williams EG, Kaul V, Rouse JL, Knox RB (1984) Apparent self-incompatibility in Rhododendron ellipticum, R. championae and R. amamiense: a post-zygotic mechanism. Plant Cell Incompatibility Newsletter 16, 10-11.
| Google Scholar |
Williams EG, Rouse JL, Palser BF, Knox RB (1990) Reproductive biology of Rhododendron. Horticultural Reviews 12, 1-67.
| Google Scholar |
Yan LJ, Burgess KS, Zheng W, Tao ZB, Li DZ, Gao LM (2019) Incomplete reproductive isolation between Rhododendron taxa enables hybrid formation and persistence. Journal of Integrative Plant Biology 61, 433-448.
| Crossref | Google Scholar | PubMed |
Zha H-G, Milne R, Sun H (2008) Morphological and molecular evidence of natural hybridization between two distantly related Rhododendron species from the Sino-Himalaya. Botanical Journal of the Linnean Society 156, 119-129.
| Crossref | Google Scholar |
Zhang C, Yin L, Dai S (2009) Diversity of root-associated fungal endophytes in Rhododendron fortunei in subtropical forests of China. Mycorrhiza 19, 417-423.
| Crossref | Google Scholar | PubMed |
Zhuang P (2019) Progress on the fertility of Rhododendron. Biodiversity Science 27, 327-338.
| Crossref | Google Scholar |