Genetic control of flowering in spotted gum, Corymbia citriodora subsp. variegata and C. maculata
Myralyn Abasolo A E , David J. Lee B , Lyndon Brooks C , Carolyn Raymond D and Mervyn Shepherd A D FA Cooperative Research Centre for Forestry, Southern Cross Plant Science, Southern Cross University, PO Box 157, Lismore, NSW 2480, Australia.
B Faculty of Science, Health, Education and Engineering, University of the Sunshine Coast and Department of Agriculture, Fisheries and Forestry, Locked Bag 4, Maroochydore DC, Qld 4558, Australia.
C Division of Research, Southern Cross University, Lismore, NSW 2480, Australia.
D Southern Cross Plant Science, Southern Cross University, PO Box 157, Lismore, NSW 2480, Australia.
E Present address: Fairbank’s Selected Seed Co. Pty Ltd, 13/53 Gateway Boulevard, Epping, Vic. 3076, Australia.
F Corresponding author. Email: mervyn.shepherd@scu.edu.au
Australian Journal of Botany 62(1) 22-35 https://doi.org/10.1071/BT13223
Submitted: 23 February 2013 Accepted: 16 February 2014 Published: 28 April 2014
Abstract
Genetically controlled asynchrony in anthesis is an effective barrier to gene flow between planted and native forests. We investigated the degree of genetically controlled variation in the timing of key floral developmental stages in a major plantation species in subtropical Australia, Corymbia citriodora subsp. variegata K.D. Hill and L.A.S Johnson, and its relative C. maculata K.D. Hill and L.A.S. Johnson. Flowering observations were made in a common garden planting at Bonalbo in northern New South Wales in spring on 1855 trees from eight regions over three consecutive years, and monthly on a subset of 208 trees for 12 months. Peak anthesis time was stable over years and observations from translocated trees tended to be congruent with the observations in native stands, suggesting strong genetic control of anthesis time. A cluster of early flowering provenances was identified from the north-east of the Great Dividing Range. The recognition of a distinct flowering race from this region accorded well with earlier evidence of adaptive differentiation of populations from this region and geographically-structured genetic groupings in C. citriodora subsp. variegata. The early flowering northern race was more fecund, probably associated with its disease tolerance and greater vigour. Bud abundance fluctuated extensively at the regional level across 3 years suggesting bud abundance was more environmentally labile than timing of anthesis. Overall the level of flowering in the planted stand (age 12 years) was low (8–12% of assessed trees with open flowers), and was far lower than in nearby native stands. Low levels of flowering and asynchrony in peak anthesis between flowering races of C. citriodora subsp. variegata may partially mitigate a high likelihood of gene flow where the northern race is planted in the south of the species range neighbouring native stands.
Additional keywords: anthesis, Eucalyptus, floral development, gene flow, plantation forestry.
Introduction
Natural hybridisation among related species or populations is common in trees (e.g. Thompson et al. 2010; Millar et al. 2012), and in some groups including eucalypts and pines, this is largely attributed to weak intrinsic reproductive barriers (e.g. Ledig 1998; Potts et al. 2003). Speciation, and the emergence of genetic difference at the population level, is therefore thought to largely depend on external factors, geographic distance or population disjunction (i.e. parapatry and allopatry) (Potts and Wiltshire 1997). If these external barriers are disrupted, e.g. where geographic isolation is broken down by translocations, gene flow from exotics may influence the evolutionary trajectories of native populations through changes in fitness and survivorship (Rieseberg et al. 1989). The expansion of hardwood plantations (largely Eucalyptus spp.) in recent decades in Australia has entailed large scale movement of germplasm around some regions of the country (Potts et al. 2003; Gavran and Parsons 2009). This movement resulted in increased exposure of the native populations to risks of locally exotic gene flow (Barbour et al. 2008). Awareness and concern over these risks have resulted in increased research to support sustainable forest management policy and certification practices (Potts et al. 2001; Australian Forestry Standard Limited 2007; Barbour et al. 2008; Laikre et al. 2010; Forest Stewardship Council 2012).
Corymbia citriodora subsp. variegata (CCV) (formerly Eucalyptus citriodora) is the single most important taxa for hardwood plantations in subtropical Australia, with around 20 000 ha established in south-east Queensland (Qld) and northern New South Wales (NSW) over the past decade (Lee 2007). It is one of four taxa of spotted gums that have a wide natural distribution in subtropical and temperate Australia. They occur as a latitudinal replacement series along the eastern coast from around Cairns in Qld to as far south as the Mottle Range in Victoria, but with regions of disjunction and sympatry (Hill and Johnson 1995). Recent studies of genetic structuring in the species complex identified patterns of geographic structuring within CCV, a lack of genetic differentiation from C. henryi (CH) (a broad-leafed form), and that these northern taxa are strongly differentiated from the southern species, C. maculata (CM) (Shepherd et al. 2012). The planting of CCV, mainly in south-east Qld and northern NSW, exposes native forests that contain Corymbia species (mainly spotted gums (Section Maculatae; Parra-O et al. 2009), but also other Corymbia sp. i.e. bloodwoods) to gene flow from locally exotic taxa that are inter-fertile (Barbour et al. 2008). In recent years, commercial plantations of CCV have been derived from one or a few select provenances in the north of its range, particularly Woondum provenance near Gympie, Qld, which is genetically and adaptively differentiated both from the CCV and CH provenances from NSW including its higher tolerance to Quambalaria shoot blight (QSB) (Johnson et al. 2009; Brawner et al. 2011).
Genetic risk decision trees are based on taxonomic, biological and geographic criteria (Byrne et al. 2011). In an assessment of the likelihood and impact of gene flow and hybridisation between planted and native CCV to other spotted gums in northern NSW, Barbour et al. (2008) concluded that overall the likelihood of gene flow was high because taxa are highly inter-fertile and likely share common pollinators, including those able to travel long distances (Southerton et al. 2004; Bacles et al. 2009). The ecological and evolutionary impact of gene flow was more difficult to assess but the impacts are likely to be less profound in such widespread and abundant species, and none are considered threatened or rare. This does not preclude the identification of spotted gum populations that have unique value for conservation, or the possession of adaptations that may be valuable for future survival.
Genetically-determined asynchrony in flowering time is an intrinsic attribute imparting reproductive isolation and is thought to be one factor that can be engaged for management of risk from planted eucalypts (Griffin 1982; Potts et al. 2003). Several studies have shown that differences in flower opening times between taxa, provenances and families of temperate eucalypts are under genetic control (Gore and Potts 1995; Jones et al. 2011). Early and late flowering populations of the temperate Eucalyptus globulus have been identified for example, and the timing of anthesis was shown to be under strong genetic control (Jones et al. 2011).
Differences in anthesis time have also been noted between taxa of subtropical spotted gums (McDonald 2004). However, most surveys have been of native stands (Dale and Hawkins 1983; Pook 1984; Pook et al. 1997; Law et al. 2000); thus, genetic differences are confounded with environmental factors. Observations of floral development in a common garden study are required to separate the degree of genetic and environmental determination in these processes. Comparison of floral development in native stands with that of translocated trees is also useful for assessing the extent of genetic control, because stability in flowering parameters across environments is an indicator of genetic determination.
The present study was undertaken to determine the degree of genetically controlled differences in floral development, and assess the potential of genetic factors in isolating plantings from native spotted gums. We monitored flowering in CCV and CM from different regions across their native range, growing in a common garden trial, to determine the timing of key developmental time points such as bud initiation and peak anthesis, to test for evidence of genetic control and to characterise other factors that influence the capacity of a tree to flower (i.e. year effects and tree size).
Materials and methods
Study site and materials
The study was undertaken in a Forests NSW spotted gum provenance trial located 13 km south-east of Bonalbo (28°52′S, 152°38′E; elevation 164 m asl) in northern NSW. The trial has been described in detail in Johnson et al. (2009). In brief, it contained a wide collection of CCV as well as some CM provenances (Fig. 1). It was established in 1999 on an ex-grazing site with an east-facing aspect and an infertile yellow podzolic soil and receives a mean annual rainfall (MAR) of 1031 mm (Johnson et al. 2009; Australian Bureau of Meteorology 2012). It was laid out using a randomised complete block design (RCBD) with five replicates of 196 open-pollinated families planted in five-tree-line plots; only four replicates were assessed in this study as one replicate had poor survival by 2009. Trees were planted in a 4.0 × 2.5 m spacing and had not been thinned at the time of assessment.
![]() |
A subset of trees from 31 CCV and CM provenances was selected for assessment in the Bonalbo trial. The term provenance here is used in the sense of White et al. (2007), i.e. the geographical location of the native population where the plant material originated. Some provenances were excluded if they were represented by few families and were proximal to provenances with more families. Provenances that were included in the subset were selected to cover a wide geographical range and include the main sources of CCV used in the current breeding programs (Lee 2007). The selected provenances were categorised into eight eco-geographic regions on the basis of the following four environmental variables: latitude, elevation, mean annual rainfall and temperature (Fig. 1). However, the most northerly occurring CM provenances may be intergrades with CCV, because they originate from a recognised intergrade region (Hill and Johnson 1995; Shepherd et al. 2012).
Flowering assessments
Flowering observations were recorded for a total of 1855 trees from 128 families from 26 CCV and five CM provenances (Table 1). Each CCV or CM provenance was represented by 1–13 families, each family having nominally 20 trees.
![]() |
For each tree, the canopy was surveyed for reproductive structures from the ground with 10 × 40 binoculars. Five stages of flower development were distinguishable; initials, buds, open flowers, green capsules and older capsules. Pre-anthesis flowers were classified as initials when they were visible and up to ~3 mm in width, or buds, if they were larger. Flowers were classified as open when opercula had been shed and anthers were evident. Green capsules are post-anthesis structures (i.e. reproductive structures for the current season in which anthers and styles were no longer evident) and are distinguishable from older capsules in their appearance and position on the branch. Green capsules (current season) appear plump and mostly located towards the tip of the branches, whereas older capsules appear shrivelled and concentrated on branches near the trunk of the tree.
Annual flowering assessments
The full complement of selected trees (1855) at the Bonalbo trial was assessed annually in November of 2009–2011 (trees aged 10, 11 and 12 years from planting), so as to assess flowering among provenances of spotted gum. The November census time was chosen to commence monitoring because this was before the previously recorded anthesis period for spotted gums in northern NSW (Law et al. 2000; Table 2).
Monthly flowering assessments
Monthly assessments were also conducted on the Bonalbo trial over a period of 12 months to observe progression and rate of floral development in the different provenances of spotted gum. Monthly assessments were undertaken on a subset of 208 trees from 22 provenances in two replicates (Table 1). The trees chosen for the monthly assessments were those found to be reproductively active during the initial November 2009 assessment and had the largest diameter at breast height (DBH) within each plot. Monthly assessments were undertaken from September 2010 to November 2011; however, no assessment was undertaken during December 2010, January 2011 and March 2011, because of heavy rainfall that precluded site access. Trees were assessed as for annual assessments and, in addition, period of bud initiation and peak anthesis time were noted (Table 2).
Statistical analysis
Flowering intensity and synchrony
The monthly flowering intensity and degree of synchrony at the provenance level were determined using the monthly observations of 208 trees from the Bonalbo trial. An intensity value of 0–5 was determined for each provenance derived as a function of the quantity of flowers and the proportion of trees in flower from that provenance (modified from Keatley et al. (2004) and Keatley and Hudson (2007)). The quantity score ranged from 0 to 3 and was assigned as follows: 0 = no flowering; 0.5 = <100 flowers; 1 = 101–1000 flowers; 2 = 1001–5000 flowers and 3 = >5000 flowers. The score for the proportion of trees in flower in a provenance was assigned as follows: 0.5 = 0.1–10%; 1 = 11–40%; 1.5 = 41–60%; 2 = 61–100%. Modification of the original scoring system was necessary in our case to adjust for the lower flower abundance in the trials than the more abundant flowering detected in the native stands studied by Keatley et al. (2004). We report intensity scores on a monthly basis as appropriate for our analysis but they are usually given as an annual average in long-term flowering studies (e.g. Keatley et al. 2004; Keatley and Hudson 2007). An intensity score of zero indicated that no tree from the provenance flowered, whereas a score of five indicated flowering was heavy in that month. Peak flowering was defined as the month or months with the highest flowering intensity (Keatley et al. 2004; Keatley and Hudson 2007).
Assessing the variation in the potential to flower
We tested for variation in flowering among regions (owing to genetics), years and interaction effects based on the November assessment over 3 years (2009–2011). The presence of buds was used as an indicator of the ‘potential to flower’ because of the very few trees with flowers at the census time-point. The use of buds as surrogate to flowers is justified as buds and flowers have strong, positive correlation with each other (O’Brien et al. 2007).
Although more trees had buds than flowers, the number of trees with buds was still too few (i.e. in 2011, only 8% of trees had buds) to use standard parametric statistics (see Results). Thus, the potential to flower for material from a particular region, or year, in our zero-inflated dataset, was assessed using odds, calculated as the number of trees with buds divided by the number of trees without buds for each year in each replicate. Odds were used because simple quantity counts were not appropriate because of unequal sample sizes and the binary nature of the data (i.e. trees with buds, trees without buds) at any group level (i.e. family in a replicate).
A mixed, multilevel binary logistic regression model was used to test the significance of region, year and region by year interaction effects. A logit function was necessary to link the non-linear response variable to components of a linear model. It was also necessary to remove families from the analysis that had no trees with buds in any replicate in any year because the logarithm of zero is undefined. This was not thought to bias effect estimates unduly because there was no regional basis for the families to be excluded. Of the 128 families in the original set assessed, 105 families had at least one tree with buds in each replicate in each year and were included in the analysis.
We explored the power of alternative models to identify a model that best explained the variation in the odds of a tree having buds, as a measure of the potential to flower, by adding factors into the model and comparing their deviance information criterion (DIC) values. A more optimal model that explains more of the variation will have a lower DIC, and the drop in DIC with the addition of a term is an indication of the importance of the term to the model (Spiegelhalter et al. 2002). A series of models were tested with the sequential addition of year, region and interaction terms, and their DIC values compared. The model of best fit included all terms, with the interaction term providing the largest improvement in fit, and the model was defined as
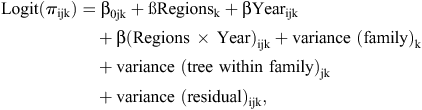
where Logit (πijk) is the natural logarithm of the odds of trees having flower buds in Observation i in Replicate j from Family k; β0jk is the intercept in the model (Region 1 in Year 1); βRegionsk is a set of dummy variables representing the eight regions; βYearijk is a set of dummy variables representing the 3 years of observations; β(Regions × Year)ijk is a set of dummy variables representing the interaction of regions and years, variance (family)k, variance (replicate)jk and variance (residual)ijk are the residual variances at the family, replicate and observation levels. The models were estimated by the Markov chain Monte Carlo (MCMC) method in MLwiN software v2.24 (Rasbash and Browne 2000). Each model was run with a 500-cycle burn-in and a monitoring chain length of 175 000 iterations.
For descriptive purposes, the logit-scale estimates from the models were back-transformed to estimates of the odds of trees with buds for each region in each year, and odds ratios for comparing each region between years and comparing regions in each year. For example, the odds ratio comparing the odds of trees with buds in Region 1 in Year 1 to the odds of trees with buds in Region 2 in Year 1 equals the number of trees with buds from Region 1 in Year 1 on the number of trees without buds from Region 1 in Year 1, divided by the number of trees with buds from Region 2 in Year 1 on the number of trees without buds from Region 2 in Year 1. Odds ratios for multiple pairwise comparisons were tested using single degree of freedom Wald chi-square tests (χ2 = (estimate ÷ s.e.)2). No adjustment was made for the number of comparisons because the power for the tests was low (data not shown) as a result of the small proportions of trees with buds.
Vigour assessments
To investigate whether tree diameter was correlated with capacity to flower, the DBH over bark was recorded for the 1855 trees monitored for flowering in the Bonalbo Trial in November 2009. A one-tailed independent sample t-test was used to test whether there was a significant difference in the mean DBH between the class of trees that were reproductively active compared with the class of trees that were not in flower.
We also compared ‘flowering effort’ and DBH at the regional level. Flowering effort was a measure of fecundity and was defined as the total quantity of unique reproductive materials (i.e. such as initials, buds, flowers) observed on a tree at any time during the 3 years of study. To avoid double counting, only initials were added to the total amount of materials evident in the first year of assessment, 2009; hence, the formula to estimate flowering effort was

A two-way ANOVA and l.s.d. were used to determine significant differences in the mean flowering effort among regions, using DBH as a covariate in the analysis. All analyses were performed using SPSS v19 (SPSS 2010).
Results
Few trees flowered and overall flowering intensity was low
The annual assessment of flowering in November at the Bonalbo trial showed that, overall, the level of reproductive activity (i.e. percentage of trees with buds, flowers or green capsules) was low, with the percentage of reproductively active trees 10.45%, 7.33% and 18.98% in 2009, 2010 and 2011, respectively. The most abundant reproductive structures in November was buds, which were present on ~1/2 of the reproductively active trees in 2009, 2010 and 2011, 7.44%, 3.07% and 8.30% in each year, respectively. That few trees flowered within the year was also reflected in the low flowering-intensity scores in each month calculated on a subsample of trees (n = 208) for the time course of observations between September 2010 and November 2011. Provenance values for flowering intensity ranged between 1 and 2, of a maximum possible score of 5 (Fig. 2).
![]() |
A similar low level of flowering activity was evident at a nearby trial during the same years (2010 and 2011; Emu Creek, 15 km west of the Bonalbo trial; data not shown) and in other plantings in northern NSW (Barbour et al. 2008), suggesting that this low level of flowering activity at the Bonalbo trial was typical for similar-aged plantings of spotted gum in the region.
Regional differences in anthesis
In terms of anthesis, the monthly observations at the Bonalbo trial between September 2010 and November 2011 indicated that CCV flowered from winter (June) to early summer (November) (Fig. 2). Trees from some regions may have also flowered in December and January of those years (no observations were made in December 2010 and January 2011) and the flowering season appeared to be finished by March 2011, with no tree being in flower at this time. Our assessment was based on 10 of the 22 provenances in our study because four provenances (Dalmorton, Ewingar, Lower Bucca and Kangaroo River) had only a single tree that flowered during the assessment period, and no tree from the other eight provenances flowered. Hence, the provenances with only one tree that flowered, and those that did not flower were excluded from the following analysis.
One of the most striking patterns was the accord among higher-latitudinal provenances (>27°S) to forgo anthesis during the 2011 flowering season, despite many trees carrying initials or buds in that year (Fig. 2). A minor exception to this was the observation of one tree from the Richmond Range with flowers during February 2011.
Among the group of northern provenances flowering in 2011 (the two Toolara sites, Woondum, Brooyar and Wondai provenances), there tended to be a delay in the onset of anthesis among more inland provenances (Brooyar and Wondai) (Fig. 2). The coastal provenances of Toolara and Woondum commenced flowering in June or July, the more inland, although still east of the GDR provenance of Brooyar, commenced in September, whereas Wondai, the only provenance west of the GDR, commenced in October (Fig. 2).
Also striking was the year-to-year variation in anthesis. There was a 3-month window (September–November) of observations in both years that allowed an across-year comparative analysis. Whereas mainly higher-latitudinal provenances flowered during this period in 2010, only lower-latitudinal provenances flowered in the September–November window in 2011.
Genetic control of flowering in spotted gum
Regional differences in the potential to flower
Our analysis of the potential for flowering using buds as a surrogate for open flowers, and assessing the likelihood of flowering in odds in a multi-year analysis, revealed significant differences among regions. In all, five models with different sets of terms were evaluated (Table 3). The full model (Model 5) provided the best fit (i.e. lowest DIC value) of any model tested and was used in all further analyses (Table 3; Model 5, DIC = 2215). Examining the impact of the sequential fitting of terms to the model indicated that the inclusion of the Region × Year interaction term had the largest effect overall (indicated by the largest reduction in the DIC value, i.e. between Models 4 and 5, a delta DIC = –105), followed by year, then region (Table 3).
Single-year analyses were undertaken to simplify the interpretation of region effects (ANOVA not shown) because of the importance of interaction. Significant differences were found among regions within each year (Fig. 3a–c). As an example, we consider regional effects in 2011, because the largest differences among regions were evident in this year (Fig. 3c). Together, C. maculata (MAC) and coastal NSW (CNSW) regions recorded the highest odds for the potential to flower for any region at any time during the 3 years, 0.31 and 0.25, respectively. The MAC region had significantly higher odds of trees with buds than did any other region except CNSW (P < 0.05; Fig. 3c). The odds ratios between MAC and three other southern regions, high-elevation NSW (HENSW), high-rainfall NSW (HRNSW) and Richmond Range (RICH) were 9.97, 7.68 and 4.63, respectively (P < 0.001; Fig. 3c). Thus, the MAC region was almost 176 times more likely to have trees with buds than was coastal Qld (CQld) (odds ratio = 175.91; P < 0.001), and more than four times more likely than were inland Qld (IQld) (odds ratio = 4.46; P < 0.05) and subcoastal Qld (SCQld) (odds ratio = 4.45; P < 0.05). The CNSW region was also 128 times more likely to have had trees with buds than was CQld, its coastal counterpart in the north (odds ratio = 127.88; P < 0.001). These large changes in the odds for the MAC and CNSW regions in 2011, relative to earlier years, appeared to drive the significance of the Region × Year interaction effect.
![]() |
Correspondence in floral development between translocated and native stands
We compared the timing of bud initiation and anthesis in the translocated materials in the present study with those recorded for representative populations from native stands, for further evidence of genetic control at the population level. Because of limited sampling, three broad latitudinal zones were defined to allow comparative analysis of flowering between translocated and native populations within a zone, i.e. a northern subtropic, southern subtropic and temperate zones (Table 2). At this scale within latitudinal zones, patterns of flowering observed in the translocated populations tended to reflect those recorded in the native stands (Table 2). For example, CQld, which is encompassed within the northern subtropics zone, initiated buds in September to November in our study, which was similar to the bud initiation time recorded for native CCV in the same zone (September to October; Table 2). The duration of bud development was also similar for translocated trees we observed from CQld, which took 9–13 months for initials to progress to anthesis, with native populations from coastal (Gympie region) and more inland (Barakula) sites being recorded taking 10–11 months to undergo bud development (Dale and Hawkins 1983).
The comparison of translocated and native materials from the southern subtropical zone also suggested congruence in the timing and duration of floral development. Our observations of translocated trees representing this region in the Bonalbo trial indicated that they took 20–21 months to develop, which was notably longer than the translocated CCV from the northern subtropical zone, but appeared to be in accord with the duration between initiation and anthesis for more southerly native populations of CCV (i.e. 15–24 months; Law et al. 2000). Similar congruence in the key floral-development phases was evident for translocated and native CM (Table 2). Because translocated material from this latitudinal zone was not included in the subsample for our monthly observations at the Bonalbo trial, we referred to observations conducted at Grafton Agricultural Research Advisory Station (GARAS) to benchmark floral development of translocated CM. Bud initiation occurred in October in translocated CM at GARAS, close to the September date recorded for CM in southern NSW (Table 2). We could not ascertain the exact bud-development time of the translocated CM because the buds aborted before anthesis; however, bud development was more than 12 months, which was congruent with the recorded bud development of native CM (Table 2). This correspondence in the flowering behaviour at the population level across sites suggested genetic determination in some aspects of the flowering processes of spotted gum.
Other factors influencing flowering in spotted gum
Year-to-year differences in the potential to flower
Although region was significant in determining the potential to flower (see above), overall, region explained less variation than the Year or the interaction of Region and Year terms. The significance of the interaction term could largely be attributed to the reactivity of a few regions, namely MAC and CNSW (Fig. 3c). The high likelihood of the presence of buds on trees from the MAC and CNSW regions in 2011 was in contrast to their low odds in 2010 (odds = 0.013 and 0.026, respectively; Fig. 3a–c). The MAC region was almost seven times more likely to have trees with buds in 2011 than in 2009 (odds ratio = 6.93; P < 0.001) and 48 times more likely than in 2010 (odds ratio = 48.61; P < 0.001; Fig. 3a–c). The CNSW region, however, was three times more likely to have trees with buds in 2009 than in 2010 (odds ratio = 3.04, P < 0.001), and nine times more likely to have trees with buds in 2011 than in 2010 (odds ratio = 9.03; P < 0.001; Fig. 3a–c). The odds of a tree having buds in the other regions were relatively stable across the 3 years compared with CNSW and MAC (Fig. 3a–c).
Tree vigour and flowering
Among the 1855 trees with DBH measurements, only 421 trees (22.69%) had reproductive structures from at least one developmental stage present, i.e. initials, bud, flowers or capsules, and thus, they were reproductively active at least once during the 3 years of the study. The mean DBH of trees that were reproductively active (mean ± s.e.; 16.15 ± 0.21 cm, n = 421) was significantly higher than the mean DBH of trees that were not active during the observation period (mean ± s.e.; 11.85 ± 0.12 cm, n = 1434; t-test, P < 0.001).
To test whether there was a correspondence in tree vigour (as assessed by DBH in 2009) and reproductive activity, a correlation analysis was conducted on the mean DBH of each region. A variable ‘flowering effort’ was generated to represent the amount of tree resources spent on flowering during the 3 years of observations. This assumes that DBH in 2009 was correlated with DBH in 2010 and 2011. This correlation is reasonable, given the many studies that have shown high inter-correlation among DBH values over years (e.g. Stackpole et al. 2010). The correlation analysis indicated that there was a weak but highly significant positive correlation between DBH and flowering effort (r2 = 0.26; P < 0.001) when assessed at the regional level (Fig. 4).
![]() |
Discussion
The objective of our study was to describe patterns of flowering in spotted gum planted at Bonalbo, NSW, and determine the degree to which floral development is under genetic control. Our results provided some evidence for regionally based differences in the timing of anthesis and other floral development stages, and the stability in the timing of these processes despite translocations.
Low levels of flowering – impacts on gene flow and the ability to detect variation in flowering patterns
A key finding of this study was that there was a very low flowering level in young (≤12 years old) planted spotted gums. There are two lines of evidence for the low levels of flowering. First, the FIS value (measure of the number of flowers and the number of trees flowering) of each provenance was very low (i.e. ranged from 0 to 2 of a maximum of 5, see Fig. 2). Second, the proportion of trees with current reproductive materials (initials, buds, flowers or green capsules) at the trial was also low in the 3 years of study, being 10.45%, 7.33% and 18.98% in 2009, 2010 and 2011, respectively. The low levels of flowering were typical across a range of plantations and trials in northern NSW that were observed at the same time as the Bonalbo trial (Abasolo 2013). Observations on other planted spotted gums indicated that flowering intensity was similar to that in the Bonalbo trial (FIS ≤ 2.5) and that the natives had higher FIS values (maximum of 5) than did the planted spotted gums.
The low levels of flowering, and, thus, low pollen production, may moderate gene flow from young plantations (i.e. <12 years old; see section on Implications for risk assessment of gene flow from plantings of CCV). Pollen output from plantations should be revisited at rotation age because flowering is expected to increase with age. However, flowering may also depend on many other factors influencing growth rates, including thinning regimes and stocking rates, which are important if the plantations are grown for a further 20–30 years.
The observation of low flowering levels in this study severely constrained the ability to use standard statistical methods used to test for genetic control and in exploring variation in flowering patterns. Our data were ‘zero-inflated’, meaning that at some levels (i.e. family and provenance), there were no observations or variance. The zero-inflated dataset precluded the use of traditional quantitative methods to partition variance and estimate heritability. Approaches that model the presence or absence of reproductive structures are valid in such cases (Faddy 1998; Kuhnert et al. 2005). One approach is the use of a binary logistic regression model, which takes into consideration the presence or absence of a unit (Welsh et al. 1996; Fletcher et al. 2005). For our analysis, we had to pool observations at higher levels (i.e. region rather than provenance) and used variables such as number of buds rather than number of open flowers to obtain sufficient data for comparison. In situations where the dataset is zero-inflated, generalised regression models (GLM) provide limited improvement in fit because GLM is based on a data with a normal distribution. Hall (2000) illustrated the use of alternative models (zero-inflated Poisson (ZIP) and zero-inflated binomial (ZIB)) to generalised regression models, and their application in agriculture.
Two flowering groups align with geographic and genetic groupings
In the present study, we identified the following two flowering groups: a cluster of early flowering northern coastal provenances, and a late-flowering group consisting of inland northern, and southern provenances. The northern provenances also tended to have earlier bud initiation time than did the southern provenances (Abasolo 2013). The geographic origins of flowering groups in CCV broadly accorded with the genetic structuring previously identified using molecular markers (Shepherd et al. 2008, 2012) and differentiation at other adaptive traits in spotted gums (see below). Trees from south-eastern Queensland localities (northern flowering group) were distinguished by allele-frequency differences at microsatellite marker loci when compared with trees from northern NSW (southern flowering group). Such an alignment in genetic structure, revealed by neutral molecular markers and patterns in adaptive variation, may arise where there has been historical isolation among populations that facilitates differentiation at neutral loci via genetic drift, and adaptive loci via selection (e.g. Li and Adams 1989; Butcher et al. 2009).
Although there was alignment between genetic and flowering groups from the CQld region and various NSW regions, trees from IQld (Wondai) and SCQld (Esk and Lockyer) may not fit this overall pattern. These provenances were not investigated in the genetic structure study, so their genetic affinities are unknown. But it is recognised that there is complexity and exceptions to latitudinal patterns concerning inland Qld, and populations neighbouring the Border Ranges, because of the long and narrow distribution, isolation by distance effects and disjunctions in the habitat of CCV (Shepherd et al. 2008). This complexity may be reflected in mis-alignment in genetic and flowering grouping from these regions. Further investigation of the genetic structure in CCV, including the populations studied here (Wondai, Esk and Lockyer), will be necessary to see whether the congruency in genetic and flowering groups holds, or whether they are exceptions, perhaps explained by differential adaption within genetic groups.
Notwithstanding the potential complexities of alignment of flowering and genetic groupings in the north, common geographic origin for a genetically and adaptively distinct cluster of provenances suggests that distinct geographic races in the sense of White et al. (2007) should be recognised in CCV i.e. ‘a subdivision of a species consisting of genetically similar individuals occupying a particular territory in which they have become adapted through natural selection’. It is proposed that because of its distinctness at the genetic level, anthesis and disease-resistance attributes, the Coastal provenances around Gympie (Qld), including Toolara and Woondum, but also tentatively Brooyar, also still east of the GDR, are recognised as a geographic race within CCV.
We hypothesised that the geographic patterns in flowering time in CCV observed may be linked to local adaptation to climate. Environmental factors such as temperature, duration of growth season and daylength often vary in a predictable and continuous manner with latitude and plant populations frequently exhibit clines in growth and phenological traits as a result of adaptive responses to these variables (Colautti et al. 2009). Studies of introduced annuals in the northern hemisphere revealed a common pattern in flowering times that emerged in response to climatic gradients during historical times across a wide range of plant species (Kollmann and Banuelos 2004; Bastlová et al. 2006; Montague et al. 2008). Unlike annuals, however, where the need to reproduce within one growing season, and photoperiod, appears to be the main driver of adaption (Riihimaki and Savolainen 2004; Hancock et al. 2011), there is increasing evidence that photoperiod is not a main driver for flowering time in perennials (Keller et al. 2012) where temperature, and more particularly heat sum, better predicts floral development and anthesis (Reader 1983; Yeh et al. 1999; Trudgill et al. 2005).
Geographic patterns in flowering time have been recorded in several eucalypts. In Jarrah (E. marginata), like CCV, trees from more northerly populations were found to flower earlier than those from southern populations when grown in a common garden trial (O’Brien et al. 2007). Indeed, this pattern of earlier flowering in populations from the north of a species range may be common in eucalypts because analysis of long-term flowering records from apiculturists for 28 eucalypt species in Victoria show a general north–south trend (Birtchnell and Gibson 2006). Although these observations in native stands do not allow us to conclude that these differences are necessarily adaptive (they could be plastic responses to differences in the environment), the correspondence in rankings of populations based on flowering time between translocated and native populations, as evident in our study as well as other studies of eucalypts (E. marginata and E. globulus) (O’Brien et al. 2007; Jones et al. 2011), suggests genetic control and the potential for adaptive significance in flowering-time differences in some cases. In the case of E. globulus, in contrast to CCV and E. marginata, more northerly populations from Victoria flowered later than eastern coast Tasmanian populations when grown together in a seed orchard at Hobart (Jones et al. 2011). This showed that control of flowering time is complex and latitudinal differences are not always a reliable predictor.
Less directly, climatic factors may influence anthesis through pollinator availability. One possible driver of adaptation is the avoidance of anthesis during high-rainfall months to maximise the number of pollinators (Elzinga et al. 2007). The northern coastal provenances receive 50% of the 1066 mm mean annual rainfall during the summer months from December to March. This high rainfall may significantly reduce the number of pollinators during summer. Thus, flowering earlier in the season might be a strategy for northern coastal provenances to attract more pollinators; however, more study will be required to test this hypothesis.
The northern flowering race may be more fecund because of greater vigour owing to disease resistance
A trend of higher fecundity and greater vigour of trees from the northern flowering race than the southern race may be due to its higher tolerance to QSB (Brawner et al. 2011; Pegg et al. 2011). It has been shown that populations from the higher-rainfall region around Gympie (Qld), including the provenances assessed in the present study belonging to CQld, have a higher tolerance to QSB than do the NSW provenances, and this leads to higher early plantation productivity of trees from the CQld region (Brawner et al. 2011). Shoot blight damage caused by QSB has a major effect on the crowns of planted CCV because the lesions and distortion of new shoots, including stems and expanding leaves, can cause a massive reduction in foliage, or loss of apical dominance in severe cases (Pegg et al. 2011). Northern populations were found to be more tolerant to damage caused by QSB in the Bonalbo trial (Johnson et al. 2009) and this may account for the larger size of trees from these populations in the current study. However, the productivity of CM is usually greater when it is grown in temperate regions where QSB is unlikely to be significant, due to a drier climate (Arnold et al. 2005).
Although there was a significant correlation between the flowering effort and tree size at the regional level (Fig. 4), the relationship was relatively weak (r2 = 0.2). Several studies have shown that reproductively active plants tend to be larger and put more effort in to reproduction than do smaller members of these species (Klinkhamer et al. 1991; Hanzawa and Kalisz 1993). From these observations, it has been concluded that plants must reach a threshold size before reproducing and the degree of resources committed to reproduction is balanced with vegetative growth (Bonser and Aarssen 2009). A tendency for larger trees from better growing regions that have greater tolerance to QSB to flower, as observed here, may be a function of both these factors, because stressed, less vigorous trees may not have reached a threshold size or do not have the resources to undertake flowering. The IQld region, like the lack of accord in patterns between geographic location and anthesis timing, did not fit the overall pattern between tree size and fecundity well. This region largely contributed to the weakness of the correlation because it had the highest mean flowering-effort score (0.688; P < 0.05) and relatively poor growth.
Potential to flower is plastic and appears to be affected by tree vigour and yearly climatic variation
Region by year interaction was the single most important term in explaining potential to flower. Year-to-year variation was most pronounced in trees from the MAC region, which had low potential to flower in both 2009 and 2010 but was very prolific in 2011 (Fig. 4a–c). Such high plasticity in year-to-year variation has often been related to climatic variation. Climatic conditions, particularly warm temperature, trigger key development steps in bud initiation and bud growth (Moncur et al. 1994). Continued high temperature during bud growth accelerates the pace of development by increasing the availability of photosynthates. For example, photosynthates necessary for bud development can be allocated in the shoots only at a temperature higher than 18°C among subtropical species (Specht and Brouwer 1975). Exposure to warm temperatures can be expressed as heat sum, which is the linear accumulation of temperature above a base temperature measured in degree-days (Trudgill et al. 2005). Heat sum has been found to determine the timing of bud break and subsequent reproductive development in temperate eucalypts (Moncur et al. 1994; Jones et al. 2011), and heat-sum models have been used to predict anthesis time in other trees, e.g. Norway spruce (Nikkanen 2001) and Quercus (García-Mozo et al. 2006).
Implications for risk assessment of gene flow from plantings of CCV
The recognition of two geographic races (exhibiting both adaptive differences in anthesis time and disease tolerance) within CCV presents a challenge for forest managers where trees from the northern race are planted in the south of the natural range of CCV. Our study revealed two factors that may mitigate gene flow between these races, namely, partial asynchrony in anthesis time and the overall low levels of flowering in planted stands of CCV. The likelihood may be further moderated by the observation of low levels of pollen production in plantations of young age (i.e. less than 14 years old) relative to nearby native stands, so that the source to sink ratio for pollen may be low.
These factors may reduce the overall risk profile of such planting that otherwise may be high owing to biological factors such as high cross-compatibility because of a lack of many pre- (e.g. structural incompatibilities) and post-zygotic (genetic) reproductive barriers (Barbour et al. 2008).
On the other hand, increasing recognition of distinct geographic races with adaptive differences and evidence of genome-wide isolation among natural populations of CCV from neutral genetic markers may indicate that the potential impacts on the evolutionary trajectories of native stands, and their ecologies, may be higher than previously thought (Barbour et al. 2008). Evidence is mounting that populations of CCV differ in potentially important adaptive traits such as disease tolerance (Johnson et al. 2009; Pegg et al. 2011), frost tolerance (Larmour et al. 2000), terpene chemistry (Asante et al. 2001) and peak flowering time (this study), reflecting natural selection among populations for particular genes and gene combinations. Gene flow between populations may contribute to either mal-adaption (e.g. reduced reproductive fitness), or range expansion (e.g. perhaps because of higher tolerance to abiotic or biotic stress or enhanced dispersal) or both, in hybrids, as well as having wider ecological impacts on dependant fauna (perhaps owing to altered timing in the availability of nectar or pollen food source for pollinators, for example). Spotted gums are a food source for birds as well as flying mammals such as the grey headed flying fox (Southerton et al. 2004). These impacts may be increased where the ratio of pollen in the source (plantation) and the sink (native stand) is greater (Potts et al. 2003), and in some cases, the viability of small remnants may be threatened because of pollen swamping (Petit et al. 2004) or dilution of the reproductive capacity of native stands (i.e. by soaking up otherwise viable female gametes with mal-adapted pollen; Keim et al. 1989). Mal-adaption may manifest itself early in the life of the first-generation hybrid, perhaps mitigating further introgression, or it may be delayed beyond the onset of reproduction (such a temporal delay might be a consequence of climate change, for example), having longer-term implications for the recipient population.
Crossing and evaluation of fitness is also needed to assess whether outbreeding depression (OBD) will manifest in advanced generations of hybrids between genetic groups of spotted gum. A lowered fitness in the first-, and particularly later-generation hybrids of plants relative to the parental taxa (OBD) may also arise as a result of intrinsic (i.e. breakdown in co-adapted gene complexes) or extrinsic (i.e. hybrid less fit in particular environments) factors (Frankham et al. 2007). The breakdown in favourable epistatic interactions in co-adapted gene complexes, for example, is viewed as a likely cause of reduced vigour in other later-generation eucalypt hybrids (Costa e Silva et al. 2012). The degree of OBD in hybrids may relate to the genetic distance between the parents (e.g. because of distance, habitat or topographical barriers; Waser and Price 1989) and can be assessed by genetic markers and the Fst metric (Beaumont 2005). Three geographically based genetic groups have been identified within CCV and its relative CH, that align with habitat barriers are thought to be indicative of long-term restrictions to gene flow in the these taxa (Shepherd et al. 2008). It is unclear yet whether OBD will be evident in inter-racial crosses of CCV; however, if so, this may limit introgression between planted and native CCV in some circumstances.
Acknowledgements
This study was part of M. Abasolo’s PhD study, ‘Assessing and managing gene flow from locally exotic spotted gums’, supported by scholarships from the CRC Forestry and Southern Cross University. The authors thank the Forest NSW for access to the trial sites, G. Ablett for assistance in the field, and G. Luker for preparing Fig. 1.
References
Abasolo M (2013) Assessing and managing pollen mediated gene flow from locally exotic Corymbia plantations. PhD Thesis, Southern Cross University, Lismore, NSW.Arnold R, Bush D, Stackpole D (2005) Genetic variation and tree improvement. In ‘New forests: wood production and environmental services’. (Eds S Nambiar, I Ferguson) pp. 25–49. (CSIRO: Melbourne)
Asante KS, Brophy J, Doran J, Goldsack R, Hibbert D, Larmour J (2001) A comparative study of the seedling leaf oils of the spotted gums: species of the Corymbia (Myrtaceae), section Politaria. Australian Journal of Botany 49, 55–56.
| A comparative study of the seedling leaf oils of the spotted gums: species of the Corymbia (Myrtaceae), section Politaria.Crossref | GoogleScholarGoogle Scholar |
Australian Bureau of Meteorology (2012) ‘Climate and past weather information.’ Available at http://www.bom.gov.au/climate/data/. [Accessed July 2012]
Australian Forestry Standard Limited (2007) Australian forestry standard AS 4708-2007. Available at http://www.forestrystandard.org.au/. [Accessed June 2007]
Bacles CFE, Brooks J, Lee DJ, Schenk PM, Lowe AJ, Kremer A (2009) Reproductive biology of Corymbia citriodora subsp. variegata and effective pollination across its native range in Queensland, Australia. Southern Forests 71, 125–132.
Barbour RC, Crawford AC, Henson M, Lee DJ, Shepherd M, Potts BM (2008) The risk of pollen-mediated gene flow from exotic Corymbia plantations into native Corymbia populations in Australia. Forest Ecology and Management 256, 1–19.
| The risk of pollen-mediated gene flow from exotic Corymbia plantations into native Corymbia populations in Australia.Crossref | GoogleScholarGoogle Scholar |
Bastlová D, Bastl M, Čížková H, Květ J (2006) Plasticity of Lythrum salicaria and Phragmites australis growth characteristics across a European geographical gradient. Hydrobiologia 570, 237–242.
| Plasticity of Lythrum salicaria and Phragmites australis growth characteristics across a European geographical gradient.Crossref | GoogleScholarGoogle Scholar |
Beaumont M (2005) Adaptation and speciation: what can F st tell us? Trends in Ecology & Evolution 20, 435–440.
| Adaptation and speciation: what can F st tell us?Crossref | GoogleScholarGoogle Scholar |
Birtchnell MJ, Gibson M (2006) Long-term flowering patterns of melliferous Eucalyptus (Myrtaceae). Australian Journal of Botany 54, 745–754.
| Long-term flowering patterns of melliferous Eucalyptus (Myrtaceae).Crossref | GoogleScholarGoogle Scholar |
Bonser SP, Aarssen LW (2009) Interpreting reproductive allometry: individual strategies of allocation explain size-dependent reproduction in plant populations. Perspectives in Plant Ecology, Evolution and Systematics 11, 31–40.
| Interpreting reproductive allometry: individual strategies of allocation explain size-dependent reproduction in plant populations.Crossref | GoogleScholarGoogle Scholar |
Brawner J, Lee DJ, Hardner C, Dieter MJJ (2011) Relationships between early growth and Quambalaria shoot blight tolerance in Corymbia citriodora progeny trials established in Queensland Australia. Tree Genetics & Genomes 7, 759–772.
| Relationships between early growth and Quambalaria shoot blight tolerance in Corymbia citriodora progeny trials established in Queensland Australia.Crossref | GoogleScholarGoogle Scholar |
Butcher PA, McDonald MW, Bell JC (2009) Congruence between environmental parameters, morphology and genetic structure in Australia’s most widely distributed eucalypt, Eucalyptus camaldulensis. Tree Genetics & Genomes 5, 189–210.
| Congruence between environmental parameters, morphology and genetic structure in Australia’s most widely distributed eucalypt, Eucalyptus camaldulensis.Crossref | GoogleScholarGoogle Scholar |
Byrne M, Stone L, Millar MA (2011) Assessing genetic risk in revegetation. Journal of Applied Ecology 48, 1365–1373.
| Assessing genetic risk in revegetation.Crossref | GoogleScholarGoogle Scholar |
Colautti RI, Maron JL, Barrett SCH (2009) Common garden comparisons of native and introduced plant populations: latitudinal clines can obscure evolutionary inferences. Evolutionary Applications 2, 187–199.
| Common garden comparisons of native and introduced plant populations: latitudinal clines can obscure evolutionary inferences.Crossref | GoogleScholarGoogle Scholar |
Costa e Silva J, Potts BM, Tilyard P (2012) Epistasis causes outbreeding depression in eucalypt hybrids. Tree Genetics & Genomes 8, 249–265.
| Epistasis causes outbreeding depression in eucalypt hybrids.Crossref | GoogleScholarGoogle Scholar |
Dale JA, Hawkins PJ (1983) Phenological studies of spotted gum in southern inland Queensland. Report no. 35. Department of Forestry Queensland, Government Printer Queensland.
Elzinga JA, Atlan A, Biere A, Gigord L, Weis AE, Bernasconi G (2007) Time after time: flowering phenology and biotic interactions. Trends in Ecology & Evolution 22, 432–439.
| Time after time: flowering phenology and biotic interactions.Crossref | GoogleScholarGoogle Scholar |
Faddy M (1998) Stochastic models for analysis of species abundance data. In ‘Statistics in ecology and environmental monitoring 2: decision making and risk assessment in biology’. (Eds DJ Fletcher, L Kavalieris, BFJ Manly) pp. 33–40. (University of Otago Press: Dunedin, New Zealand)
Fletcher D, Mackenzie D, Villouta E (2005) Modelling skewed data with many zeros: a single approach combining ordinary and logistic regression. Environmental and Ecological Statistics 12, 45–54.
| Modelling skewed data with many zeros: a single approach combining ordinary and logistic regression.Crossref | GoogleScholarGoogle Scholar |
Forest Stewardship Council (2012) FSC international standard FSC principles and criteria for forest stewardship. Forest Stewardship Council. Report no. FSC-STD-01-001 (version 4–0) EN, Forest Stewards Council, A.C. Bonn, Germany.
Frankham R, Ballou JD, Briscoe DA (2007) ‘Introduction to conservation genetics.’ 1st edn. (Cambridge University Press: Cambridge, UK)
García-Mozo H, Galán C, Jato V, Belmonte J, de la Guardia CD, Fernández D, Gutiérrez M, Aira MJ, Roure JM, Ruiz L (2006) Quercus pollen season dynamics in the Iberian Peninsula: response to meteorological parameters and possible consequences of climate change. Annals of Agricultural and Environmental Medicine 13, 209–224.
Gavran M, Parsons M (2009) ‘Australia’s plantations, 2009 inventory update. National forest inventory.’ (Bureau of Rural Sciences: Canberra)
Gore PL, Potts BM (1995) The genetic control of flowering time in Eucalyptus globulus, E. nitens and their F1 hybrids. In ‘Eucalypt plantations: improving fibre yield and quality. Proceedings CRCTHF–IUFRO conference, 19–24 February 1995, Hobart, Tasmania’. (Eds BM Potts, NMG Borralho, JB Reid, RN Cromer, WN Tibbits, CA Raymond), pp. 241–242. (CRC for Temperate Hardwood Forestry: Hobart)
Griffin AR 1982. Pollination ecology of eucalypts: a framework for study. In ‘Pollination ‘82. Proceedings of the symposium held at Melbourne University, 1982’. (Eds EG Williams, RB Knox, JH Gilbert, P Bernhardt) pp. 42–56. (University of Melbourne)
Hall DB (2000) Zero-inflated poisson and binomial regression with random effects: a case study. Biometrics 56, 1030–1039.
| Zero-inflated poisson and binomial regression with random effects: a case study.Crossref | GoogleScholarGoogle Scholar | 1:STN:280:DC%2BD3M7it1Cnsg%3D%3D&md5=4f0d2345d39314364d62f38b7351169aCAS | 11129458PubMed |
Hancock AM, Brachi B, Faure N, Horton MW, Jarymowycz LB, Sperone FG, Toomajian C, Roux F, Bergelson J (2011) Adaptation to climate across the Arabidopsis thaliana genome. Science 334, 83–86.
| Adaptation to climate across the Arabidopsis thaliana genome.Crossref | GoogleScholarGoogle Scholar | 1:CAS:528:DC%2BC3MXht1Gms7%2FI&md5=657a1cc6a4f048a66d00b73405b19a05CAS | 21980108PubMed |
Hanzawa FM, Kalisz S (1993) The relationship between age, size, and reproduction in Trillium grandiflorum (Liliaceae). American Journal of Botany 80, 405–410.
| The relationship between age, size, and reproduction in Trillium grandiflorum (Liliaceae).Crossref | GoogleScholarGoogle Scholar |
Hill KD, Johnson LAS (1995) Systematic studies in the eucalypts 7. A revision of the bloodwoods, genus Corymbia (Myrtaceae). Telopea 6, 185–504.
Johnson IG, Carnegie A, Henson M (2009) Growth, form and Quambalaria shoot blight tolerance of spotted gum in north-eastern New South Wales. Silvae Genetica 58, 180–191.
Jones R, Vaillancourt RE, Gore P, Potts BM (2011) Genetic control of flowering time in Eucalyptus globulus ssp. globlulus. Tree Genetics & Genomes 7, 1209–1218.
| Genetic control of flowering time in Eucalyptus globulus ssp. globlulus.Crossref | GoogleScholarGoogle Scholar |
Keatley MR, Hudson IL (2007) A comparison of long-term flowering patterns of box-ironbark species in Havelock and Rushworth forests. Environmental Modeling and Assessment 12, 279–292.
| A comparison of long-term flowering patterns of box-ironbark species in Havelock and Rushworth forests.Crossref | GoogleScholarGoogle Scholar |
Keatley MR, Hudson IL, Fletcher TD (2004) Long-term flowering synchrony of box-ironbark eucalypts. Australian Journal of Botany 52, 47–54.
| Long-term flowering synchrony of box-ironbark eucalypts.Crossref | GoogleScholarGoogle Scholar |
Keim P, Paige KN, Whitham TG, Lark KG (1989) Genetic analysis of an interspecific hybrid swarm of Populus: occurrence of unidirectional introgression. Genetics 123, 557–565.
Keller SR, Levsen N, Olson MS, Tiffin P (2012) Local adaptation in the flowering-time gene network of balsam poplar, Populus balsamifera L. Molecular Biology and Evolution 29, 3143–3152.
| Local adaptation in the flowering-time gene network of balsam poplar, Populus balsamifera L.Crossref | GoogleScholarGoogle Scholar | 1:CAS:528:DC%2BC38XhsVehtbvN&md5=ef15afaf2188393f0e058ea39f37269dCAS | 22513286PubMed |
Klinkhamer PGL, De Jong TJ, Meelis E (1991) The control of flowering in the monocarpic perennial Carlina vulgaris. Oikos 61, 88–95.
| The control of flowering in the monocarpic perennial Carlina vulgaris.Crossref | GoogleScholarGoogle Scholar |
Kollmann J, Banuelos MJ (2004) Latitudinal trends in growth and phenology of the invasive alien plant Impatiens glandulifera (Balsaminaceae). Diversity & Distributions 10, 377–385.
| Latitudinal trends in growth and phenology of the invasive alien plant Impatiens glandulifera (Balsaminaceae).Crossref | GoogleScholarGoogle Scholar |
Kuhnert PM, Martin TG, Mengersen K, Possingham HP (2005) Assessing the impact of grazing levels on bird density in woodland habitat: a Bayesian approach using expert opinion. Environmetrics 16, 717–747.
| Assessing the impact of grazing levels on bird density in woodland habitat: a Bayesian approach using expert opinion.Crossref | GoogleScholarGoogle Scholar |
Laikre L, Schwartz MK, Waples RS, Ryman N, Group TGW (2010) Compromising genetic diversity in the wild: unmonitored large-scale release of plants and animals. Trends in Ecology and Evolution 25, 520–529.
| Compromising genetic diversity in the wild: unmonitored large-scale release of plants and animals.Crossref | GoogleScholarGoogle Scholar | 20688414PubMed |
Larmour JS, Whitfeld SJ, Harwood CE, Owen JV (2000) Variation in frost tolerance and seedling morphology of the spotted gums Corymbia maculata, C. variegata, C. henryi and C. citriodora. Australian Journal of Botany 48, 445–453.
| Variation in frost tolerance and seedling morphology of the spotted gums Corymbia maculata, C. variegata, C. henryi and C. citriodora.Crossref | GoogleScholarGoogle Scholar |
Law B, Mackowski C, Schoer L, Tweedie T (2000) Flowering phenology of myrtaceous trees and their relation to climatic, environmental and disturbance variables in northern New South Wales. Austral Ecology 25, 160–178.
| Flowering phenology of myrtaceous trees and their relation to climatic, environmental and disturbance variables in northern New South Wales.Crossref | GoogleScholarGoogle Scholar |
Ledig FT (1998) Genetic variation in Pinus. In ‘Ecology and biogeography of Pinus’. 1 edn. (Ed. DM Richardson) pp. 251–273. (Cambridge University Press: Cambridge, UK).
Lee DJ (2007) Achievements in forest tree genetic improvement in Australia and New Zealand 2: development of Corymbia species and hybrids for plantations in eastern Australia. Australian Forestry 70, 11–16.
| Achievements in forest tree genetic improvement in Australia and New Zealand 2: development of Corymbia species and hybrids for plantations in eastern Australia.Crossref | GoogleScholarGoogle Scholar |
Li P, Adams WT (1989) Range-wide patterns of allozyme variation in Douglas-fir (Pseudotsuga menziesii). Canadian Journal of Forest Research 19, 149–161.
| Range-wide patterns of allozyme variation in Douglas-fir (Pseudotsuga menziesii).Crossref | GoogleScholarGoogle Scholar |
McDonald M (2004) Outcrossing rates in a seedling seed orchard of Corymbia maculata (spotted gum). Report no. 1401. CSIRO Forestry and Forest Products, Canberra.
Millar MA, Byrne M, Nuberg I, Sedgley M (2012) High levels of genetic contamination in remnant populations of Acacia saligna from a genetically divergent planted stand. Restoration Ecology 20, 260–267.
| High levels of genetic contamination in remnant populations of Acacia saligna from a genetically divergent planted stand.Crossref | GoogleScholarGoogle Scholar |
Moncur MW, Hand FC, Ramsden NG (1994) Environmental and cultural effects on flowering and seed production of plantation grown Eucalyptus nitens. Report for the Tasmanian Forest Research Council. Division of Forestry, CSIRO, Canberra.
Montague JL, Barrett SCH, Eckert CG (2008) Re-establishment of clinal variation in flowering time among introduced populations of purple loosestrife (Lythrum salicaria, Lythraceae). Journal of Evolutionary Biology 21, 234–245.
Nikkanen T (2001) Reproductive phenology in a Norway spruce seed orchard. Silva Fennica 35, 39–53.
O’Brien EK, Mazanec RA, Krauss SL (2007) Provenance variation of ecologically important traits of forest trees: implications for restoration. Journal of Applied Ecology 44, 583–593.
| Provenance variation of ecologically important traits of forest trees: implications for restoration.Crossref | GoogleScholarGoogle Scholar |
Parra-O C, Bayly MJ, Drinnan A, Udovicic F, Ladiges P (2009) Phylogeny, major clades and infrageneric classification of Corymbia (Myrtaceae), based on nuclear ribosomal DNA and morphology. Australian Systematic Botany 22, 384–399.
| Phylogeny, major clades and infrageneric classification of Corymbia (Myrtaceae), based on nuclear ribosomal DNA and morphology.Crossref | GoogleScholarGoogle Scholar |
Pegg GS, Carnegie AJ, Wingfield MJ, Drenth A (2011) Variable resistance to Quambalaria pitereka in spotted gum reveal opportunities for disease screening. Australasian Plant Pathology 40, 76–86.
| Variable resistance to Quambalaria pitereka in spotted gum reveal opportunities for disease screening.Crossref | GoogleScholarGoogle Scholar |
Petit RJ, Bodenes C, Ducousso A, Roussel G, Kremer A (2004) Hybridization as a mechanism of invasion in oaks. New Phytologist 161, 151–164.
| Hybridization as a mechanism of invasion in oaks.Crossref | GoogleScholarGoogle Scholar | 1:CAS:528:DC%2BD2cXmsVWltw%3D%3D&md5=da498bae50b8a391b12d185be1dd691bCAS |
Pook EW (1984) Canopy dynamics of Eucalyptus maculata Hook. I distribution and dynamics of leaf populations. Australian Journal of Botany 32, 387–403.
| Canopy dynamics of Eucalyptus maculata Hook. I distribution and dynamics of leaf populations.Crossref | GoogleScholarGoogle Scholar |
Pook EW, Gill AM, Moore P (1997) Long-term variation of litter fall canopy leaf area and flowering in a Eucalyptus maculata forest on the south coast of New South Wales. Australian Journal of Botany 45, 737–755.
| Long-term variation of litter fall canopy leaf area and flowering in a Eucalyptus maculata forest on the south coast of New South Wales.Crossref | GoogleScholarGoogle Scholar |
Potts BM, Wiltshire RJE (1997) Eucalypt genetics and genecology. In ‘Eucalypt ecology: individuals to ecosystems. Vol. 1’. 1st edn. (Eds J Williams, J Woinarski) pp. 56–91. (Cambridge University Press: Cambridge, UK)
Potts BM, Barbour RC, Hingston AB (2001) Genetic pollution from farm forestry. Report no. 01/114. Rural Industries Research and Development Corporation, Hobart.
Potts BM, Barbour RC, Hingston AB, Vaillancourt RE (2003) Genetic pollution of native eucalypt gene pools – identifying the risks. Australian Journal of Botany 51, 1–25.
| Genetic pollution of native eucalypt gene pools – identifying the risks.Crossref | GoogleScholarGoogle Scholar |
Rasbash J, Browne W (2000) ‘A users guide to MLWin, version 2.4, multilevel models.’ (Institute of Education, University of London: London)
Reader RJ (1983) Using heatsum models to account for geographic variation in the floral phenology of two ericaceous shrubs. Journal of Biogeography 10, 47–64.
| Using heatsum models to account for geographic variation in the floral phenology of two ericaceous shrubs.Crossref | GoogleScholarGoogle Scholar |
Rieseberg LH, Zona S, Aberdom L, Martin TD (1989) Hybridisation in the island endemic, Catalina mahogany. Conservation Biology 3, 52–58.
| Hybridisation in the island endemic, Catalina mahogany.Crossref | GoogleScholarGoogle Scholar |
Riihimaki M, Savolainen O (2004) Environmental and genetic effects on flowering differences between northern and southern populations of Arabidopsis lyrata (Brassicaceae). American Journal of Botany 91, 1036–1045.
| Environmental and genetic effects on flowering differences between northern and southern populations of Arabidopsis lyrata (Brassicaceae).Crossref | GoogleScholarGoogle Scholar | 21653459PubMed |
SPSS (2010) ‘IBM SPSS for Windows (version 19).’ (SPSS: Chicago, IL)
Shepherd M, Kasem S, Ablett G, Ochieng JW, Crawford A (2008) Genetic structuring of the spotted gum complex (genus Corymbia, section Politaria). Australian Systematic Botany 21, 15–25.
| Genetic structuring of the spotted gum complex (genus Corymbia, section Politaria).Crossref | GoogleScholarGoogle Scholar | 1:CAS:528:DC%2BD1cXktFyit7w%3D&md5=36ef951cfd8f11af6086820ed2c46908CAS |
Shepherd M, Henson M, Lee DJ (2012) Revisiting genetic structuring in spotted gums (genus Corymbia section Politaria) focusing on C. maculata, an early diverged, insular lineage. Tree Genetics & Genomes 8, 137–147.
| Revisiting genetic structuring in spotted gums (genus Corymbia section Politaria) focusing on C. maculata, an early diverged, insular lineage.Crossref | GoogleScholarGoogle Scholar |
Southerton S, Birt P, Porter J, Ford HA (2004) Review of gene movement by bats and birds and its potential significance for eucalypt plantation forestry. Australian Forestry 67, 44–53.
| Review of gene movement by bats and birds and its potential significance for eucalypt plantation forestry.Crossref | GoogleScholarGoogle Scholar |
Specht RL, Brouwer Y (1975) Seasonal shoot growth of Eucalyptus spp. in the Brisbane area of Queensland (with notes on shoot growth and litter fall in other areas of Australia). Australian Journal of Botany 23, 459–474.
| Seasonal shoot growth of Eucalyptus spp. in the Brisbane area of Queensland (with notes on shoot growth and litter fall in other areas of Australia).Crossref | GoogleScholarGoogle Scholar |
Spiegelhalter DJ, Best NG, Carlin BP, Van Der Linde A (2002) Bayesian measures of model complexity and fit. Journal of the Royal Statistical Society. Series B, Statistical Methodology 64, 583–639.
| Bayesian measures of model complexity and fit.Crossref | GoogleScholarGoogle Scholar |
Stackpole DJ, Vaillancourt RE, de Aguigar M, Potts BM (2010) Age trends in genetic parameters for growth and wood density in Eucalyptus globulus. Tree Genetics & Genomes 6, 179–193.
| Age trends in genetic parameters for growth and wood density in Eucalyptus globulus.Crossref | GoogleScholarGoogle Scholar |
Thompson SL, Lamothe M, Meirmans PG, Perinet P, Isabel N (2010) Repeated unidirectional introgression towards Populus balsamifera in contact zones of exotic and native poplars. Molecular Ecology 19, 132–145.
| Repeated unidirectional introgression towards Populus balsamifera in contact zones of exotic and native poplars.Crossref | GoogleScholarGoogle Scholar | 1:CAS:528:DC%2BC3cXitVSrsLg%3D&md5=2c43cbea5e683e5be63043a891bed91aCAS | 20002578PubMed |
Trudgill DL, Honek A, Li D, Straalen NM (2005) Thermal time-concepts and utility. Annals of Applied Biology 146, 1–14.
| Thermal time-concepts and utility.Crossref | GoogleScholarGoogle Scholar |
Waser NM, Price MV (1989) Optimal outcrossing in Ipomopsis aggregata: seed set and offspring fitness. Evolution 43, 1097–1109.
| Optimal outcrossing in Ipomopsis aggregata: seed set and offspring fitness.Crossref | GoogleScholarGoogle Scholar |
Welsh AH, Cunningham RB, Donelly CF, Lindenmayer DB (1996) Modelling the abundance of rare species: statistical models for counts with extra zeros. Ecological Modelling 88, 297–308.
| Modelling the abundance of rare species: statistical models for counts with extra zeros.Crossref | GoogleScholarGoogle Scholar |
White TL, Adams WT, Neale DB (2007) ‘Forest genetics.’ 2nd edn. (CABI: Wallingford, UK)
Yeh DM, Atherton JG, Craigon J (1999) A thermal time model of post-initiation flower development in the shade plant, cineraria. Annals of Applied Biology 134, 335–340.
| A thermal time model of post-initiation flower development in the shade plant, cineraria.Crossref | GoogleScholarGoogle Scholar |