Effect of selected environmental factors on the seed germination of the invasive species Polygala myrtifolia (Polygalaceae) in Australia
Natalie Roberts A , Katrina Moloney A , Kristin Monie A and Singarayer Florentine
A The Future Regions Research Centre, Institute of Innovation, Science and Sustainability, Federation University Australia, Mount Helen, PO Box 663, Ballarat, Vic. 3350, Australia.
B School of Science, RMIT University, Melbourne, Vic. 3001, Australia.
Australian Journal of Botany 71(6) 286-295 https://doi.org/10.1071/BT22094
Submitted: 22 August 2022 Accepted: 27 April 2023 Published: 26 May 2023
© 2023 The Author(s) (or their employer(s)). Published by CSIRO Publishing. This is an open access article distributed under the Creative Commons Attribution-NonCommercial-NoDerivatives 4.0 International License (CC BY-NC-ND)
Abstract
Context: Polygala myrtifolia L. has become a significant environmental weed in Australia, where it has invaded coastal ecosystems in temperate regions and there is a high risk of extensive further spread. Knowledge of seed-germination behaviour is essential to understand the potential future impact of this species.
Aims: We investigated the effects of selected environmental factors and dormancy on P. myrtifolia seed germination and emergence to improve management strategies.
Methods: Seeds were exposed to light, temperature, pH, salinity, osmotic potential and burial depth treatments to assess germination responses, dormancy and viability.
Key results: Non-dormant seeds readily germinated to high percentages (93.0–95.0%) under specific day–night temperatures of 25°C–15°C regardless of light conditions and across all soil pH (75.0–100.0%). Salinities were tolerated up to 100 mM NaCl (70.0% germination) before sharply declining. Germination reduced from 98.3% to 40.0% at osmotic potentials of −0.4 MPa and −0.6 MPa respectively. Emergence was greatest on the soil surface (48.33%) and absent from depths of 8 cm.
Conclusions: Germination was high during autumn, winter and spring conditions and across soils of any pH. The species is unlikely to invade areas of high salinity; however, it is moderately tolerant of low soil moisture during germination.
Implications: There is high risk of P. myrtifolia further invading sensitive ecosystems because of its high germination success. Preventing seed dispersal and ensuring seedlings are controlled on emergence are critical to reducing its impact. We recommend that the cultivation and sale of this species should be prohibited across Australia to prevent further spread.
Keywords: burial depth, coastal, dune ecosystem, salinity, seed germination, soil moisture, temperature, weeds.
Introduction
Invasive plants species have a range of traits that enable establishment and persistence across different habitats, and pose a significant threat to global biodiversity (Kaushik et al. 2022). Reproductive traits relating to seed production, dispersal, dormancy and germination all influence a species’ colonising ability, therefore an understanding of their reproductive ecology can inform effective management and prevent further spread (Nešić et al. 2022).
Polygala myrtifolia L. is an invasive environmental weed of the Polygalaceae family, and, in Australia, is predominantly found in temperate coastal environments (Adair et al. 2012). It is a terrestrial, perennial, evergreen shrub that forms dense thickets, is fast-growing and reaches heights of up to 3 m within 18 months of establishment (Adair et al. 2011; Inkson 2015), by which time it also possesses a substantial tap and lateral root system (Inkson 2015). Reaching sexual maturity in approximately 2 years (Carr et al. 1992), flowering occurs throughout the year and peaks from late winter into spring (Adair et al. 2012). Fruits are flattened, heart-shaped pods that release two seeds when ripe in December and January (Eyre Peninsula Natural Resources Management Board 2018). Seeds have a mean weight of 11.1 mg (Adair et al. 2012) and are dark brown, approximately 4–5 mm long and oblong-shaped, with an elaiosome covered in microscopic white hairs (Agriculture Victoria 2020a) (Fig. 1a, b). Elaiosomes are associated with both ant dispersal and absorption of water into seed during germination in arid environments (Forest et al. 2007) and P. myrtifolia seeds are known to germinate close to parent plants, unless dispersed further by animals, ants or human activity (Agriculture Victoria 2020a). Seeds are reported to remain viable in the soil for more than 10 years (Eyre Peninsula Natural Resources Management Board 2018); however, it is unclear whether this species has any dormancy mechanism. Dormancy is defined as the prevention of a seed from germinating, even when environmental conditions are suitable (Baskin and Baskin 2004). Adair et al. (2012) suggested potential innate dormancy because of slow and poor germination success in their trial, but stated that the optimal temperature for germination is unclear.
In Australia, P. myrtifolia has escaped from gardens and naturalised across Victoria, South Australia, New South Wales, Tasmania and Western Australia (Adair et al. 2011), where it has successfully invaded sensitive ecosystems such as dune systems and coastal bluffs (Carter et al. 1990) (Fig. 2). Dense infestations are rapidly threatening biodiversity values, particularly in South Australia and Victoria (Adair et al. 2012), such as Port Phillip Bay, Western Port Bay and the Bellarine Peninsula (Atlas of Living Australia 2021). As a consequence, P. myrtifolia is now a declared weed under the Natural Resources Management Act 2004 in South Australia (Eyre Peninsula Natural Resources Management Board 2018), and the Victorian, South Australian, Tasmanian and New South Wales State governments have assessed the species as a weed of potential national significance (Inkson 2015). In Victoria, it was nominated as a restricted weed under the Catchment and Land Protection Act 1994 and was added to the Advisory list of environmental weeds in Victoria in 2018 (Agriculture Victoria 2020a; White et al. 2022). This species is ranked in the most urgent risk-rating category in Victoria, and poses a very high risk of extensive further spread into coasts and heathlands, inland plains, mallee and semi-arid regions (White et al. 2022). In Victoria, P. myrtifolia has invaded over 23 vegetation communities, with four communities and 12 species at risk, including the endangered Eucalyptus leucoxylon ssp. bellarinensis (Moxham 2003; Adair et al. 2012). This invasive woody shrub also provides habitat for pest species, such as foxes and rabbits (Eyre Peninsula Natural Resources Management Board 2018). To limit dispersal of the species from parks and gardens in Australia, P. myrtifolia var. grandiflora has been cultivated and planted as a non-invasive alternative for P. myrtifolia (Carter et al. 1990).
Global distribution of Polygala myrtifolia. Locations are represented by the dots (GBIF.org 2021).
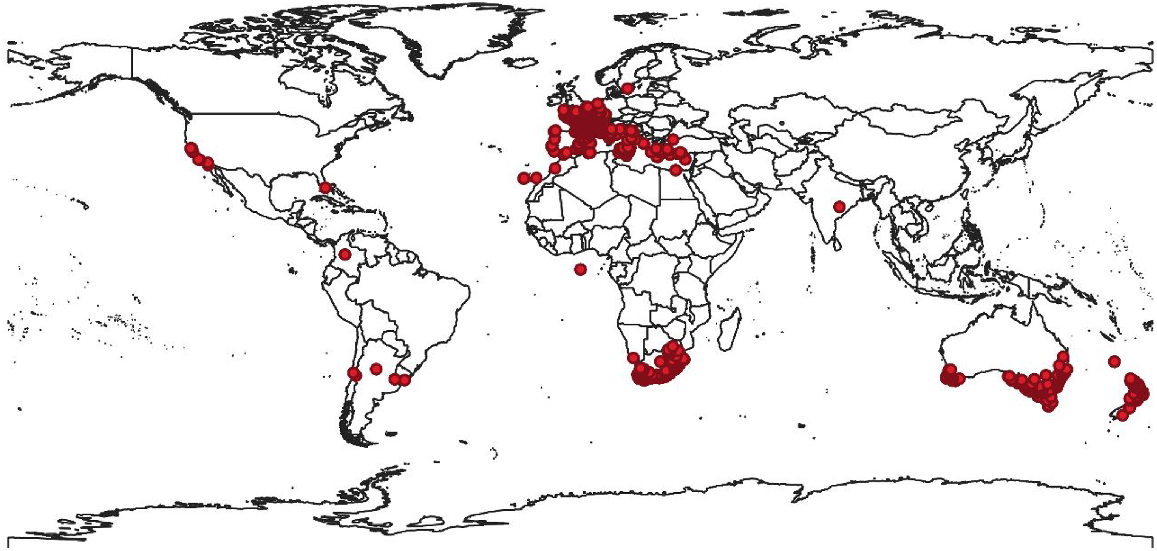
While there is no official documentation stating the cost of controlling P. myrtifolia, it has been estimated that ~A$13.6 billion is spent annually to control invasive species in Australia (Hoffmann and Broadhurst 2016). The highest costs are incurred because of invasive plants, which pose an ongoing challenge to Australian agriculture, ecology, cultural values and the economy (Bradshaw et al. 2021). Management techniques to control P. myrtifolia involve long-term options such as physical removal and the use of a range of herbicides (Carr et al. 1992; Department of Primary Industries and Regions 2021; Government of South Australia 2021). The impact of fire on population dynamics of P. myrtifolia is largely unknown, and although fire is reported to trigger germination of the soil seedbank (Adair et al. 2012), it is not stated whether this is due to heat, smoke or another mechanism. Cool burns are reported to result in high-density seedling recruitment (Government of South Australia 2021), whereas high-intensity fires are required to kill mature plants (Adair et al. 2012). Members of Polygalaceae are described as having non-fire released dormancy, a bet-hedging strategy in unpredictable environments where long-lived seeds can withstand fire and are unaffected by either smoke or heat, germinating after disturbances to the vegetation canopy (Pausas and Lamont 2022).
It is clear that a greater understanding is required of the dormancy and germination responses of P. myrtifolia, so as to develop specific control strategies that maximise effectiveness. While there is some information on control methods suitable for the management of adult plants and seedlings, there is a lack of information surrounding reproduction, which can affect both the timing and geographic regions into which this species may spread. This study therefore aimed to investigate the effect of selected environmental factors on the germination success of P. myrtifolia seeds, to inform management strategies that will effectively control this invasive species. To achieve this aim, we
assess for dormancy and germinability under a range of environmental conditions, including temperature, light, pH, salinity, osmotic stress and burial depth, and to establish risk of invasion into new regions
recommend improvements to control strategies that consider dormancy and germination responses to maximise success.
Materials and methods
Seed collection and interim storage
Polygala myrtifolia seeds were collected from approximately 200 parent plants during January 2021 by the Bellarine Landcare Group, who sourced them from the Queenscliff Natural Features Reserve (38°16′18.1″S, 144°38′13.5″E) on the Bellarine Peninsula, Victoria, Australia. Seeds were transported in a labelled zip-lock bag to the seed-ecology laboratory at Federation University, Mount Helen, Australia, and stored dry in a glass jar in a dark cabinet at room temperature of 21°C, until required.
Seed imbibition and dormancy
To assess for the presence of seed dormancy, imbibition tests were conducted on four replicates of 25 seeds, followed by emergence time for radicle and shoots. After recording the dry weight of each replicate, seeds were placed in Petri dishes on moistened filter paper at room temperature of 21°C. Seeds were then dried carefully with paper towel and reweighed at intervals of 5, 10, 15 and 30 min, followed by 1, 2, 3, 4, 6, 24, 48, 72, 96, 120, 144, 168 and 192+ h, before returning to the Petri dishes to continue imbibition. Increase in mean seed weight (%) owing to imbibition was calculated as follows: Weight increase (%) = [(Wi − Wd)/Wd] × 100, where Wi is the mass of imbibed seeds and Wd is the mass of dry seeds.
General germination protocol
All germination experiments were conducted from March to June 2021. In all trials, experimental units comprised 20 seeds, with each treatment being replicated three times. Further replication was not conducted because of a lack of seeds. Seeds were initially surface-sterilised by submerging them in 1% (w/v) sodium hypochlorite (NaOCl) for 5 min, then thoroughly rinsed with sterilised reverse-osmosis (RO) water. The surface-sterilised seeds were placed in 9-cm-diameter Petri dishes lined with sterilised Whatman No. 5 filter paper, which was moistened with sterilised RO water or treatment solution. Petri dishes were sealed with Parafilm M (Hach Pacific, Vic., Australia) and were replenished with relevant germination solutions as required to retain moisture levels throughout trials. Petri dishes were wrapped with aluminium foil and inspected only under green light to maintain the required 24 h dark conditions for the experiments. The Petri dishes were incubated in temperature-controlled cabinets (Thermoline Scientific, Model Climatron-520-DL-H, Wetherill Park, NSW, Australia), as specified according to trial conditions, and monitored every 2–3 days for 35 days. They were considered germinated when the emergent radicle was approximately 2 mm long.
After the completion of germination trials, the viability of ungerminated seeds was tested using 1% 2,3,5-triphenyltetrazolium chloride (tetrazolium) solution (Rowe Scientific, Vic., Australia). These seeds were cut in half longitudinally, and a few drops of tetrazolium were added to the exposed seed embryos. Petri dishes were consequently resealed and covered in aluminium foil, then placed at room temperature 21°C overnight. Seeds that evenly stained a dark pink were classified as viable.
Effect of temperature and light on seed germination
Polygala myrtifolia seeds were exposed to two light regimes (12 h light–12 h dark, and 24 h dark) under four temperature regimes in incubators set at alternating day–night temperatures of 35°C–25°C, 30°C–20°C, 25°C–15°C and 17°C–7°C. These light and temperature regimes were selected to reflect the possible climate variations of the region where seeds were collected, to determine the optimal germination conditions for this species. Seeds were exposed to 24 h dark conditions to mimic being completely buried in soil. As a result of this preliminary work, the optimal germination condition was 25°C–15°C under 24 h darkness. Therefore, all subsequent experiments were conducted under this combination of temperature and light regimes.
Effect of pH on seed germination
The effect of pH on seed germination was tested to determine the range of soils that P. myrtifolia has the potential to inhabit. Buffer solutions were prepared following the method of Chachalis and Reddy (2000), as follows: 2 mM of potassium hydrogen phthalate for pH 4, MES (2-(N-morpholino) ethanesulfonic acid) for pH 5 and 6; HEPES (N-2-hydroxyethyl) piperazine-N′-(2-ethanesulfonic acid)) for pH 7 and 8) and Tricine (N-Tris(hydroxymethyl)methylglycine) for pH 9 and 10. Solutions were adjusted with 1 N HCL or NaOH. Sterilised RO water with a pH value of 6.6 was included as a control.
Effect of salinity on seed germination
The effect of salinity on seed germination was explored to understand how vigorously P. myrtifolia may be able to disperse and establish in a range of Australian soils. A range of NaCl solutions was tested, having concentrations of 25 mM, 50 mM, 75 mM, 100 mM, 150 mM, 200 mM, 250 mM, 450 mM NaCl, and using sterilised RO water as a control. Concentrations up to 200 mM NaCl were selected to represent the threshold beyond which usually only salt-tolerant halophytic plants are able to successfully reproduce (Flowers and Colmer 2008), with two higher concentrations being selected to ascertain the salinity tolerance of P. myrtifolia during the vulnerable germination stage.
Effect of osmotic potential on seed germination
The ability to germinate under high soil-moisture stress may enable weed species to occupy a greater number of habitats. To determine the effect of osmotic potential on P. myrtifolia, seeds were placed in polyethylene glycol (PEG) solutions with osmotic potentials of −0.1, −0.2, −0.4, −0.6, −0.8 and −1.0 MPa, with sterilised RO water being used as the control. PEG 8000 (Sigma-Aldrich Co., Saint Louis, MO, USA) was dissolved in sterilised RO water to prepare the range of solutions with different osmotic potentials (Michel 1983).
Effect of burial depth on seedling emergence
To evaluate the effect of burial depth on seedling emergence, plastic seedling punnets were lined with paper towel and three replicates of 20 seeds were buried at depths of 0, 2, 4, 6 and 8 cm in autoclaved river sand. Punnets were placed in large trays within the temperature cabinet set to 25°C–15°C (12 h light–12 h dark), and kept moist by adding RO water to the trays. Seedlings were considered to have emerged when the cotyledon was visible at the soil surface. The burial trial was conducted for 75 days and monitored for emergence every 2–3 days.
Statistical analysis
Statistical tests were conducted using IBM SPSS Statistics for Windows (ver. 27, IBM, Armonk, NY, USA). ANOVAs were performed to determine whether the final germination percentages (GP) and germination speed (measured by time to start germination (TSG) and time to 50% germination (t50)) of P. myrtifolia were significantly different among treatments. All assumptions were investigated by examining the residuals in terms of normality and equal variance. Final germination % data were arcsine-transformed prior to ANOVA. A two-way ANOVA was used to determine significant differences between temperature and light regimes. One-way ANOVAs were used to determine significant differences in salinity, osmotic potential, burial depth and pH treatments. Results with P < 0.05 were identified as significantly different, with a subsequent post hoc Tukey test being used to determine between which treatments the differences occurred. Graphs were created in Microsoft Excel for Micrsoft 365 MSO (Microsoft Corporation, USA).
Results
Seed imbibition and dormancy
Polygala myrtifolia seeds readily imbibed water and immediately increased in weight, reaching 1.0% ± 0.0, 5.1% ± 0.1, and 50.7% ± 0.5 within 0.25, 1 and 72 h respectively. Seed weight reached a maximum increase of 56.2% ± 0.3 after 192 h, with germination commencing at 264 h (11 days), followed shortly by shoot emergence.
Effect of temperature and light on seed germination
Germination occurred across all temperature treatments under both light regimes (Fig. 3). The germination percentage (GP) was significantly higher at temperatures of 25°C–15°C (F3,16 = 202.8, P < 0.001); however, there was no significant effect owing to light (F1, 16 = 1.5, P = 0.238) or the light and temperature treatment interaction (F3,16 = 0.364, P = 0.780).
Effect of alternating day–night temperatures (17°C–7°C, 25°C–15°C, 30°C–20°C and 35°C–25°C) on the germination percentage of Polygala myrtifolia seeds under a 12 h light–12 h dark regime and 24 h dark regime. Vertical error bars represent the standard error of the means.
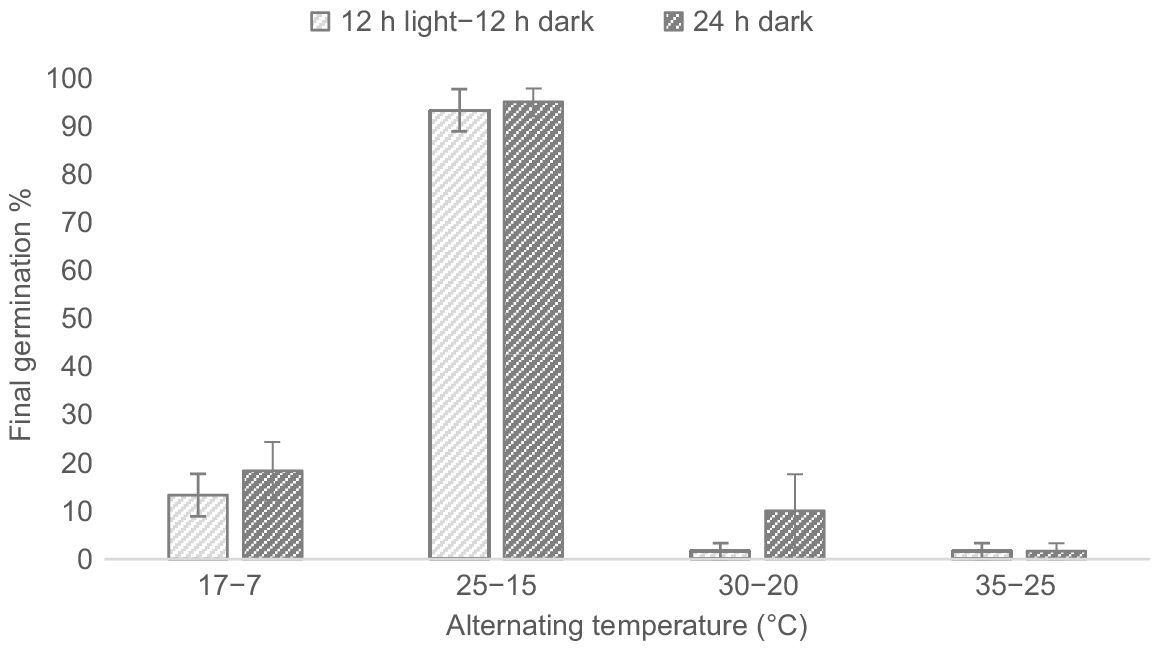
The highest GP was observed under the 25°C–15°C temperature regime for both 12 h light–12 h dark and 24 h dark (93.3% ± 4.4 and 95.0% ± 2.9 respectively). Mean viability of seeds ungerminated at the end of the trial was high, ranging from 75.0% to 100.0% (mean 93.8%) for 12 h light–12 h dark and 65% to 100% (mean 93.8%) for 24 h dark.
Subsequent trials were conducted under the optimal germination temperature of 25°C–15°C, with a 24 h continuous dark regime chosen due to the slightly higher overall germination rate achieved.
Effect of pH on seed germination
The germination of P. myrtifolia seeds was not significantly different among the pH treatments (F7,16 = 1.1, P = 0.386). The germination response was strong, ranging between 75.0% and 100.0% across all pH treatments, except for one replicate (Fig. 4). The highest mean germination percentage occurred at pH 9 (96.7% ± 1.67) and the lowest germination percentage occurred at pH 4 (71.6% ± 15.9) (Fig. 4). Across all treatments in this experiment, seed viability on completion of germination was >99.0% on the basis of post-germination tetrazolium assessment.
Effect of pH, NaCl, osmotic potential and burial depth on the germination and emergence percentage of Polygala myrtifolia seeds incubated in temperature cabinets under optimal temperature and light conditions (25°C–15°C under 24 h of darkness) for 35 days. Capped vertical bars represent ± 1 standard error of the mean. Same lowercase letters indicate means are not statistically different when tested with Tukey’s HSD test.
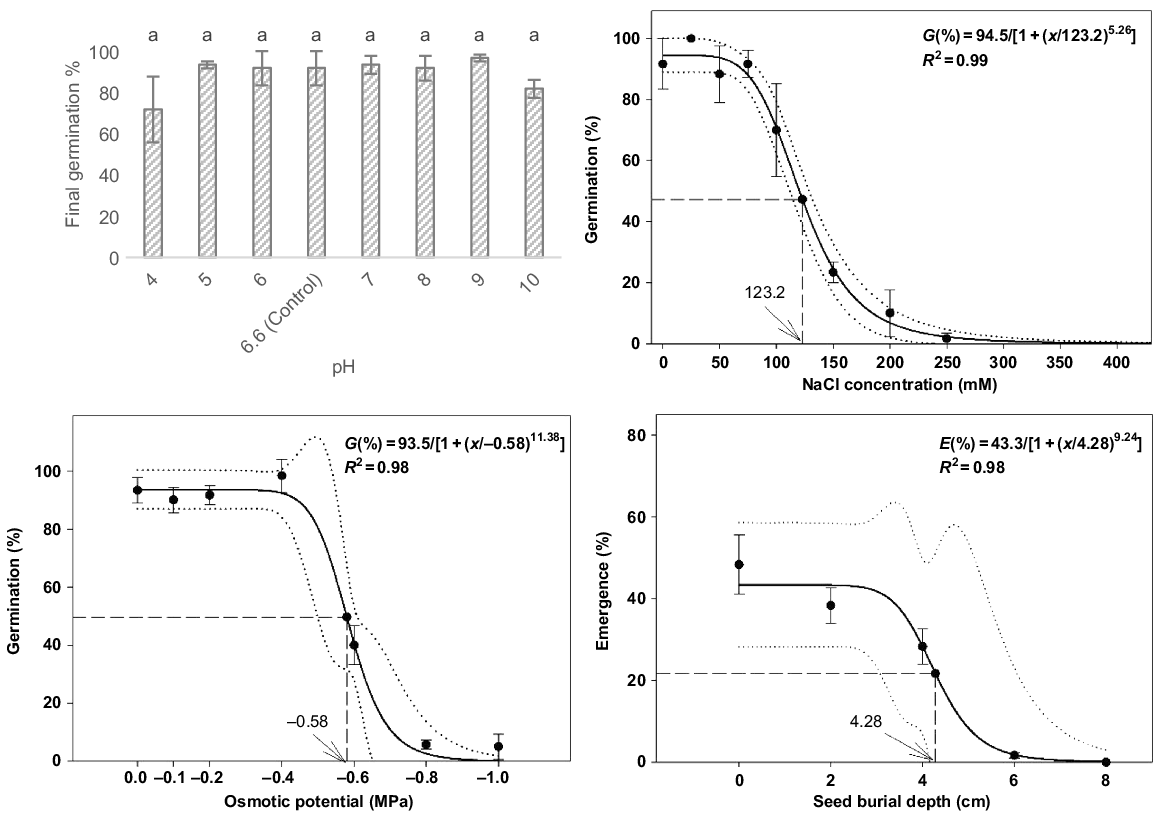
Germination speed was not significantly affected by pH for either time to start germination (TSG) (F7, 16 = 1.4, P = 0.279) or time to 50.0% of maximum germination (t50) (F7, 16 = 1.3, P = 0.317). Germination started between Day 12 and Day 16 for all treatments, and reached 50.0% of maximum germination in 18.8–23.1 days (Fig. 5).
Effect of pH, NaCl, osmotic potential and burial depth on the germination and emergence speed of Polygala myrtifolia seeds incubated in temperature cabinets under optimal temperature and light conditions (25°C–15°C under 24 h of darkness) for 35 days. Capped vertical bars represent the standard error of the mean.
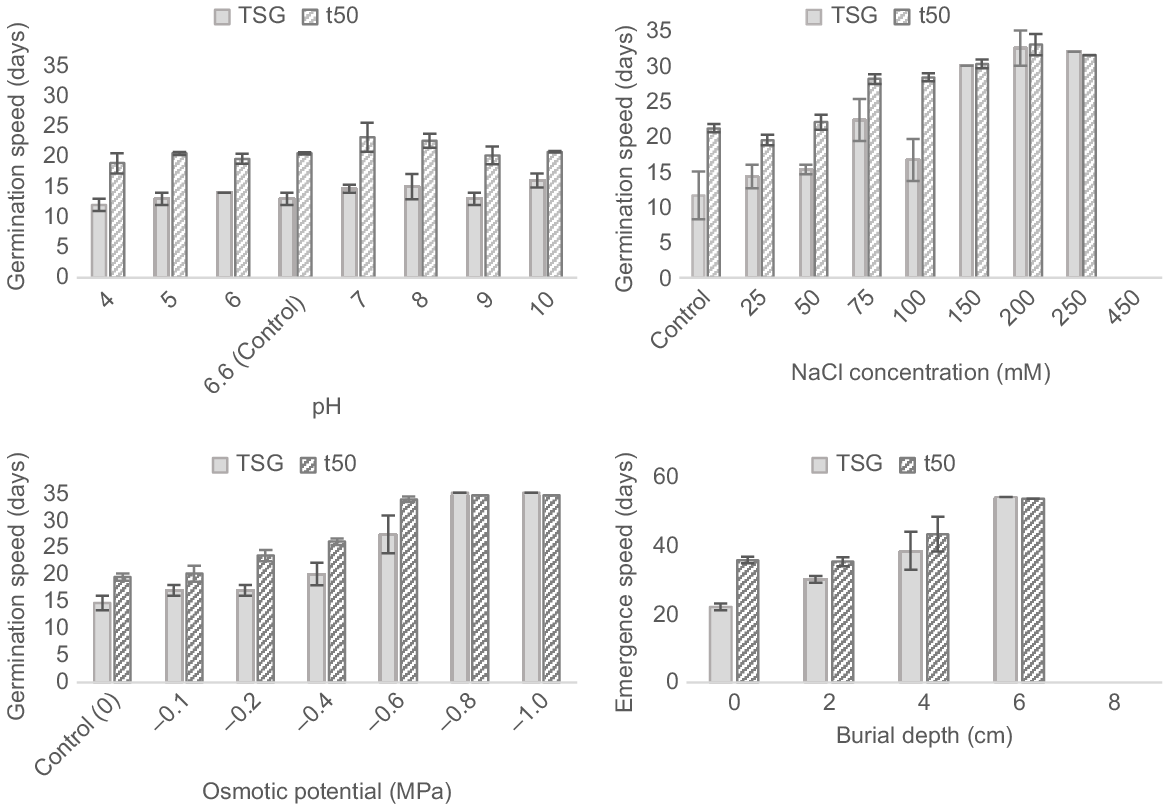
Effect of salinity on seed germination
Salinity had a significant influence on the germination of P. myrtifolia (F8,18 = 34.9, P < 0.001). Maximum germination was observed at 25 mM NaCl (100.0% ± 0.0) and sharply declined with increasing NaCl concentrations higher than 100 mM (70.0% ± 2.8). Estimated from the fitted model, germination was inhibited to 50.0% of the maximum at 123.2 mM NaCl (Fig. 4). Germination was completely inhibited at 450 mM of NaCl. Viability of ungerminated seeds on completion was 100.0% across all treatments, excluding one replicate of 200 mM (95.0%).
Higher-salinity treatments of 150 and 200 mM NaCl took a significantly longer time (>30 days) to start germination than did low-salinity treatments (11.6–15.3 days; F6, 13 = 10.856, P < 0.001). The time to 50.0% of the maximum germination was also significant (F6,13 = 39.066, P < 0.001), occurring within 19.5–22.0 days for the lowest three treatments and delayed until between 30.3 and 33.0 days for the highest three treatments (Fig. 5).
Effect of osmotic potential on seed germination
Osmotic potential significantly affected germination of P. myrtifolia (F6,14 = 50.5, P < 0.001), declining after −0.4 MPa (Fig. 4). The −0.8 and −1.0 MPa treatments had significant lower germination percentages than did the other osmotic potential treatments. Further, the −0.6 MPa treatments had significant lower germination percentages than did the lower osmotic potential treatments, reducing from 98.3% (at −0.4 MPa) to 40.0% (at −0.6 MPa). Germination was estimated to reduce to 50% of the maximum at the osmotic potential of −0.58 MPa, on the basis of the fitted model. Seed viability in the trial ranged from 85.0% to 100.0%, with an overall mean across all treatments of 97.6%.
Germination speed was significantly affected by osmotic potential treatments for both TSG (F5,11 = 13.3, P < 0.001) and t50 (F5,11 = 48.6, P < 0.001; Fig. 5). Germination commenced within 14.7 and 20.0 days for 0 to −0.4 MPa treatments, reaching 50% of the maximum germination within 19.4 to 26.0 days. In contrast, lower osmotic potential treatments between −0.6 and −1.0 MPa recorded germination between Days 27.3 and 35, reaching 50% by Days 33.8 to 34.5.
Effect of burial depth on seed germination
Seed-burial depth significantly influenced the seedling emergence of P. myrtifolia (F4,10 = 25.0, P < 0.001). Emergence decreased with burial depth (Fig. 4), with mean emergence being greatest at the soil surface (48.3% ± 7.3), decreasing significantly at 6 cm (1.7% ± 1.7) and no emergence observed at 8 cm. Reduction of germination to 50.0% of the maximum was estimated from the fitted model at 4.28 cm. Viability of ungerminated seeds decreased with an increased burial depth, from 98.3% at 0 cm to 43.3% at 8 cm.
Emergence speed was significantly delayed with burial depth for TSG (F2,6 = 6.1, P = 0.036); however, t50 was not significant (F3,6 = 4.0, P = 0.07) (Fig. 5). Emergence was first recorded after 22 days at the soil surface, reaching 50% of the maximum after 35.6 days, whereas at 6 cm, the single record of emergence occurred after 54 days.
Discussion
Seed imbibition and dormancy
The rapidity of imbibition and germination of untreated P. myrtifolia seeds under suitable light and temperature regimes, followed shortly by shoot emergence, indicated that the species is non-dormant, with no physical dormancy, as per Baskin and Baskin (2004). Seeds are dispersed in December to January in Victoria and it is likely that most seeds germinate as soon as conditions become suitable. Because there are reports of seeds remaining viable in the soil for up to 10 years (Eyre Peninsula Natural Resources Management Board 2018), it is recommended that soil seedbank studies are undertaken to determine the persistence of P. myrtifolia seeds, both in situ and through artificial ageing studies in the laboratory (Newton et al. 2009).
Effect of temperature and light on seed germination
High germination under one temperature regime (25°C–15°C) demonstrated that P. myrtifolia has specific conditions under which most germination will occur. The Bureau of Meteorology (2022) reports that in Queenscliff, where the seeds were sourced for this experiment, mean temperatures range from 14°C to 22°C in summer and from 6°C to 12°C in July. As the optimal germination temperature is similar to the climate of Queenscliff, P. myrtifolia poses a threat to further invade coastal environments of the Bellarine Peninsula, as well as regions with similar climate regimes. Its tolerance to both light and dark conditions indicates light is not a germination trigger for this species, and seeds would germinate both on and beneath the soil surface, and also under dense plant canopies. Adair et al. (2012) also found no significant difference as a result of light, with slightly lower germination in darkness. However, because the authors reported that seedling establishment and growth is supressed in shaded environments, and re-establishing the overstorey of natives assists management, further trials that investigate the influence of light during seedling establishment are recommended.
Effect of pH on seed germination
Germination of P. myrtifolia readily occurred under either acidic or alkaline conditions, indicating that soil pH is not a limiting factor for the germination of this species, nor did it delay germination. Currently, this species occurs primarily on sandy, alkaline, and calcareous soils in the Bellarine Peninsula (Adair et al. 2011). The ability of P. myrtifolia to germinate in such a wide range of soil pH values suggests that P. myrtifolia germination may occur over the pH range that encompasses most, if not all, soils in Australia (Fenner and Thompson 2009). Around the Bellarine Peninsula and Queenscliff district, the pH of the topsoil is reported to range from 4.5 to 8.5 (Rees 2002; Rees et al. 2002), therefore providing optimal soil conditions for the germination of this species.
Effect of salinity on seed germination
Saline soils provide unfavourable conditions for the germination of P. myrtifolia; however, germination still occurred in low numbers in higher salinity conditions, although germination speed was significantly delayed. Seeds were able to survive exposure to high salt concentrations during the trial, with almost all ungerminated seeds being viable after 35 days. Because of these inhibitory factors, the timing of germination might coincide with periods of higher rainfall, diluting the salts dissolved in the soil water (Fenner and Thompson 2009). Currently, this species is widely distributed in coastal environments of Australia, including the Bellarine Peninsula, primarily on secondary and tertiary dunes where soils are sandy and well drained, with high salt leaching (Agriculture Victoria 2020b). This species is known to be tolerant of salt-spray, but little is known about its soil-salinity tolerance (Agriculture Victoria 2020b). Soil salinity in Drysdale and the Queenscliff district has been recorded as EC (1:5) of 0.09 at 0–10 cm (non-saline) and EC of 3.8 at 55–110 cm (slightly saline), which may affect deeper-rooted plants (Rees et al. 2002). This topsoil would be equivalent to 0.9 mM NaCl, therefore similar to our control. Regions of highly saline soils are unlikely to support populations of this weed, because germination is both slow and minimal.
Effect of osmotic potential on seed germination
High moisture stress owing to osmotic potential can inhibit or delay seed germination for many invasive species (Florentine et al. 2018; Welgama et al. 2019). P. myrtifolia germinated readily until moisture stress was increased to −0.6, −0.8 and −1.0 MPa, where seeds were unable to obtain moisture levels required for imbibition because of the limited availability of water. This indicates that germination is most likely to occur during the cooler months when rainfall increases soil moisture sufficiently. Many species in arid zones germinate rapidly; however, there are examples of species taking over 62 and 82 days to reach 50.0% germination (Dalziell et al. 2022). P. myrtifolia showed similar capacity to germinate under water stress (t50 = −0.58 MPa) as do some shrub species in the South West (−0.62 MPa) and Goldfields (−0.56 MPa) in arid Western Australia (Dalziell et al. 2022). Hence, although the species is not as tolerant in water-limited situations as are many arid-zone species, and is more likely to become invasive in soils with more moisture, P. myrtifolia has the capacity to establish in lower-rainfall regions. Further studies into the effect of low soil moisture on establishing seedlings is recommended.
Effect of burial depth on seedling emergence
Seed size is a strong driver of the relationship between seed-burial depth and seedling emergence (Bond et al. 1999). Larger seeds have greater carbohydrate reserves providing more energy for seedling emergence from increased burial depths (Hoyle et al. 2013), and seedlings survive better during early establishment (Moles and Westoby 2004). Bond et al. (1999) argued their model is a useful predictor of maximum emergence depth according to seed weight. This model fit our species well, predicting that seedlings would emerge from depths up to 6.1 cm. Seedlings emerged from depths up to 6 cm within 54 days, and although there was no seedling emergence observed at 8 cm, some seeds had germinated at this depth but had not yet emerged. Burial-depth studies indicated that with increasing depth, resources are allocated more to root development than shoot development, so as to access water and nutrients deeper in the soil to enable shoot growth (Müller et al. 2019). Because many ungerminated P. myrtifolia seeds were viable after 75 days of burial, further studies are recommended to assess whether P. myrtifolia seedlings emerge from deeper depths with additional time, or whether seed reserves become depleted. Light is not likely to have a strong influence on seed germination and burial depth. Hoyle et al. (2013) reported that seeds buried at depths greater than 0.2 cm below the soil surface typically receive less than 1% of incidental light. As demonstrated in this study, P. myrtifolia is not dependant on light for germination, therefore any differences can most likely be attributed to resource allocation. Further investigation into differences in emergence and seedling establishment under different light regimes to reflect open and closed canopies would assist management strategies.
Management strategies
Our findings may help inform management strategies for this highly invasive species, which has the potential for extensive further spread into vulnerable environments. P. myrtifolia germinates optimally under autumn, winter and spring temperatures, regardless of light conditions, in soils of any pH, with low to moderate salinity, and low to moderate soil-moisture stress. The highest risk of invasion will occur in moist, low-salinity soils in cool to warm temperatures, and once seeds disperse, seedlings will readily emerge from on and beneath the soil surface, and under plant canopies. Soils with higher salinity and moisture stress are less likely to support populations of P. myrtifolia, although seeds may persist for longer in these soils until conditions are suitable for germination, and there is still capacity for the species to establish in lower-rainfall areas. Seed germination requirements can help inform predictive modelling to determine environments most threatened by the distribution and potential spread of P. myrtifolia.
This seed germination knowledge will assist land managers on the timing and suitability of various control strategies. It is essential to focus both on removal of mature plants, ideally prior to seedset, to prevent further dispersal and contribution to the long-lived soil seedbank, as well as the ongoing control of seedlings, which are likely to germinate mostly in optimal conditions during autumn, winter and spring. The size of the infestation and the impact of control mechanisms on native vegetation should determine the most appropriate method. Light-reducing techniques such as mulching, although ineffective at preventing germination, may suppress seedling emergence by burying seeds to a depth of 8 cm. This method is suitable only in heavily infested areas where the ground layer does not contain sensitive native flora, preferably in conjunction with restoration of appropriate species. In more sensitive areas, careful manual removal or herbicide application of seedlings is required to prevent further damage and prevent population spread, potentially in addition to extra native overstorey. Because seeds are naturally dispersed close to the parent plant, care should be taken to reduce the risk of longer-distance dispersal via machinery. The use of fire has been suggested as an effective tool for large or inaccessible populations, because it removes mature plants and triggers mass seed germination (Adair et al. 2012). This actively depletes the soil seedbank, but it is critical to implement follow-up control strategies to prevent rapid reinfestation. Any burns should be conducted at times that take into account both the fire responses of local native vegetation and their ability to re-establish, and also the likely germination response of P. myrtifolia to subsequent soil moisture. Further investigations should explore the impact of radiant heat on seed survival, the longevity and persistence of seeds in the soil seedbank, and the impact of moisture stress and closed versus open canopies on the establishment of seedlings. These findings provide a foundation for further studies into the germination requirements and potential spread of P. myrtifolia in Australia to help facilitate the development of effective control strategies.
Acknowledgements
The authors especially thank Lachlan Forbes, a representative of the Bellarine Landcare Group, for providing the seeds for this study. Thanks also go to Mansoor Jarvid for assistance developing figures, and Michael McBain for developing Fig. 2.
References
Adair RJ, Neser S, Stajsic V (2011) Phytophagous organisms associated with the woody shrub Polygala myrtifolia (Polygalaceae) and their potential for classical biological control in Australia. Plant Protection Quarterly 26, 72-80.
| Google Scholar |
Adair RJ, Shackleton A, Stajsic V, Gajaweera R (2012) The biology of Australian Weeds 61. Polygala myrtifolia L. Plant Protection Quarterly 27, 119-130.
| Google Scholar |
Agriculture Victoria (2020a) Impact assessment – myrtle-leaf milkwort (Polygala myrtifolia) in Victoria. (Agriculture Victoria: Vic., Australia). Available at http://vro.agriculture.vic.gov.au/dpi/vro/vrosite.nsf/pages/impact_myrtle_leaf_milkwort
Agriculture Victoria (2020b) Salt-spray tolerant plants – coastal region. (Agriculture Victoria: Vic., Australia). Available at https://vro.agriculture.vic.gov.au/dpi/vro/vrosite.nsf/pages/sip_coastal_salt_spray
Atlas of Living Australia (2021) Polygala myrtifolia L. Available at https://spatial.ala.org.au/?q=lsid:https:%2F%2Fid.biodiversity.org.au%2Fnode%2Fapni%2F2 895392
Baskin JM, Baskin CC (2004) A classification system for seed dormancy. Seed Science Research 14(1), 1-16.
| Crossref | Google Scholar |
Bond WJ, Honig M, Maze KE (1999) Seed size and seedling emergence: an allometric relationship and some ecological implications. Oecologia 120, 132-136.
| Crossref | Google Scholar |
Bradshaw CJA, Hoskins AJ, Haubrock PJ, Cuthbert RN, Diagne C, Leroy B, Andrews L, Page B, Cassey P, Sheppard AW, Courchamp F (2021) Detailed assessment of the reported economic costs of invasive species in Australia. NeoBiota 67, 511-550.
| Crossref | Google Scholar |
Bureau of Meteorology (2022) Climate statistics for Australian locations: summary statistics Queenscliff. (Australian Government). Available at http://www.bom.gov.au/climate/averages/tables/cw_087054.shtml
Chachalis D, Reddy KN (2000) Factors affecting Campsis radicans seed germination and seedling emergence. Weed Science 48, 212-216.
| Crossref | Google Scholar |
Dalziell EL, Lewandrowski W, Commander LE, Elliott CP, Erickson TE, Tudor EP, Turner SR, Merritt DJ (2022) Seed traits inform the germination niche for biodiverse ecological restoration. Seed Science and Technology 50, 103-124.
| Crossref | Google Scholar |
Department of Primary Industries and Regions (2021) Controlling weeds – Polygala myrtifolia. (Government of South Australia). Available at https://pir.sa.gov.au/biosecurity/weeds/controlling-weeds/polygala
Fenner M, Thompson K (2009) ‘The ecology of seeds.’ (Cambridge University Press: New York, USA). doi:10.1017/cbo9780511614101
Florentine S, Weller S, King A, Florentine A, Dowling K, Westbrooke M, Chauhan BS (2018) Seed germination response of a noxious agricultural weed Echium plantagineum to temperature, light, pH, drought stress, salinity, heat and smoke. Crop & Pasture Science 69(3), 326-333.
| Crossref | Google Scholar |
Flowers TJ, Colmer TD (2008) Salinity tolerance in halophytes. New Phytologist 179(4), 945-963.
| Crossref | Google Scholar |
Forest F, Chase MW, Persson C, Crane PR, Hawkins JA (2007) The role of biotic and abiotic factors in evolution of ant dispersal in the milkwort family (Polygalaceae). Evolution 61(7), 1675-1694.
| Crossref | Google Scholar |
GBIF.org (2021) GBIF occurrence download (Polygala myrtifolia). Available at https://doi.org/10.15468/dl.j7jwqm
Government of South Australia (2021) Declare plant policy: Polygala myrtifolia. Available at https://pir.sa.gov.au/__data/assets/pdf_file/0012/223212/polygala_policy.pdf
Hoffmann BD, Broadhurst LM (2016) The economic cost of managing invasive species in Australia. NeoBiota 31, 1-18.
| Crossref | Google Scholar |
Hoyle JA, McElroy JS, Guertal EA (2013) Soil texture and planting depth affect large crabgrass (Digitaria sanguinalis), Virginia buttonweed (Diodia virginiana), and Cock’s-comb Kyllinga (Kyllinga squamulata) emergence. HortScience 48, 633-636.
| Crossref | Google Scholar |
Kaushik P, Pati PK, Khan ML, Khare PK (2022) Plant functional traits best explain invasive species’ performance within a dynamic ecosystem – a review. Trees, Forests and People 8, 100260.
| Crossref | Google Scholar |
Michel BE (1983) Evaluation of the water potentials of solutions of polyethylene glycol 8000 both in the absence and presence of other solutes. Plant Physiology 72, 66-70.
| Crossref | Google Scholar |
Moles AT, Westoby M (2004) Seedling survival and seed size: a synthesis of the literature. Journal of Ecology 92, 372-383.
| Crossref | Google Scholar |
Müller FL, Raitt LM, Cyster LF, Cupido CF, Samuels MI, Chimphango SBM, Boatwright JS (2019) The effects of temperature, water availability and seed burial depth on seed germination and seedling establishment of Calobota sericea (Fabaceae). South African Journal of Botany 121, 224-229.
| Crossref | Google Scholar |
Nešić M, Obratov-Petković D, Skočajić D, Bjedov I, Čule N (2022) Factors affecting seed germination of the invasive species Symphyotrichum lanceolatum and their implication for invasion success. Plants (Basel) 11(7), 969.
| Crossref | Google Scholar |
Newton R, Hay FR, Probert RJ (2009) Protocol for comparative seed longevity testing. (Millennial Seed Bank Project, Kew: London, UK) p. 2. Available at www.kew.org/msbp. [Accessed 10 January 2020]
Pausas JG, Lamont BB (2022) Fire-released seed dormancy – a global synthesis. Biological Reviews 97(4), 1612-1639.
| Crossref | Google Scholar |
Rees D (2002) Sorrento mapsheet – soil pit sites: CLRA07. (Agriculture Victoria: Vic., Australia). Available at https://vro.agriculture.vic.gov.au/dpi/vro/coranregn.nsf/pages/soil_landform_soil_pits_clra7
Rees D, Crawford D, Robinson N, Reynard K, Boyle G (2002) CLRA44. Sorrento map sheet – soil pit sites. (Agriculture Victoria: Vic., Australia). Available at http://vro.agriculture.vic.gov.au/dpi/vro/coranregn.nsf/pages/soil_landform_soil_pits_clra44
Welgama A, Florentine S, Marchante H, Javaid MM, Turville C (2019) The germination success of Acacia longifolia subsp. longifolia (Fabaceae): a comparison between its native and exotic ranges. Australian Journal of Botany 67(5), 414-424.
| Crossref | Google Scholar |