Rocky landform specialists of the Mount Isa Inlier: camera trapping reveals seasonal occupancy and habitat associations of a unique faunal assemblage in an ancient landscape
Jarrad C. Barnes

A
B
Abstract
Rocky landforms offer a complex and persistent habitat for a diverse range of fauna. The Mount Isa Inlier bioregion of north-west Queensland is dominated by such landforms, but the faunal assemblage inhabiting them has received scant attention. We conducted a 13-month camera trap study at 60 camera stations, totalling 21,965 camera days, aimed at detecting the faunal assemblage present on these landforms. We categorised each identified taxon as either a rocky landform specialist or non-specialist. We identified 15 specialists and implemented a multiseason community occupancy model for 12 of these to assess how they utilised rocky landforms over the course of a year, and according to a suite of environmental characteristics. Rates of use of rocky landforms increased in November and decreased in April. At the community level we found higher rates of use of south and west slopes than north slopes, higher rates of use of rocky hills than jumpups, and higher rates of use of metamorphic and igneous geologies than sedimentary ones. We provide the first evidence of this unique community’s use of the landscape through both space and time and highlight broad site characteristics of candidate areas in which to focus future management and conservation initiatives.
Keywords: camera trap, community ecology, community occupancy model, fauna communities, habitat associations, Mount Isa Inlier, north-west Queensland, rocky landforms, saxicoline fauna.
Introduction
Australia abounds with striking and prominent rocky landforms (Twidale and Campbell 2005; Michael and Lindenmayer 2018). Due to their complex topography, rocky landforms offer habitat for a diverse range of fauna. These include both obligately saxicolous (i.e. rock-loving) taxa, as well as those that may have once persisted in the surrounding landscape matrix but are contemporarily restricted to rocky landforms due to ecological change in the matrix such as altered fire regimes, land clearing, overgrazing, and feral species invasion (Michael et al. 2011; Hohnen et al. 2016a; McDonald et al. 2017; Woolley et al. 2019; Thomas et al. 2021).
Rocky landforms provide habitat in part due to their physical structure (i.e. an abundance of shelter sites) and in part by supporting conditions different from those found in the surrounding landscape (Burke 2003). Because there are typically few barriers to movement between rocky landforms and the surrounding matrix, their importance extends beyond the landform itself into the matrix and to the species that typically inhabit it. Due to complex topography, natural disturbances such as fire and drought often have diminished effects on rocky landforms compared with the surrounding matrix (Braithwaite and Muller 1997; Clarke 2002; Benwell 2007). Complex rocky areas may also affect water availability within the landscape (Freeland et al. 1988; Braithwaite and Muller 1997), as well as support a novel soil profile (Smith and Sage 2006), leading to unique vegetation communities. These factors can lead to the presence of relictual habitats (e.g. mesic rainforest remnants within an arid matrix: Couper and Hoskin 2008), and lead to more stable temperatures compared with the surrounding matrix (García et al. 2020), providing suitable microclimates for fauna species that cannot persist in the broader landscape.
Over a long enough timescale, species that initially retreat to rocky landforms may become trapped if the surrounding matrix changes too drastically (Braithwaite and Muller 1997). These areas can therefore act as evolutionary sources, leading to speciation and the emergence of novel communities. Even common, widespread taxa show typically greater genetic divergence, more evolutionary lineages, and more restricted distributions for each lineage within rock-dwelling species than non-rock-dwelling species (Tapper et al. 2014a; Noble et al. 2017; Oliver et al. 2019). This indicates that rocky landforms, due to their geologically persistent nature, are habitats that provide ecological retreats over geological time (Couper and Hoskin 2008). Rocky landforms are therefore also likely to act as sanctuaries under climate change scenarios, acting as ‘stepping stones’ to support dispersal to more suitable regions and/or providing potential sources of recolonisation to the surrounding landscape following improved climate conditions (Keppel et al. 2012; Tapper et al. 2014b; Speziale and Ezcurra 2015; Oliver et al. 2019).
Rocky landforms consequently play an important role in conserving unique communities, especially in heavily fragmented or disturbed landscapes and through periods of climatic upheaval (Burke 2002; Michael et al. 2010). Unfortunately, despite the importance of rocky landforms, these habitats are not free from human-induced ecological change, both localised (e.g. rock removal for the illegal pet trade, or recreational activities) and landscape-scale (e.g. agriculture, resource extraction, nutrient enrichment, and invasive species proliferation; Fitzsimons and Michael 2017). Although some rocky landforms have been incredibly well-studied (e.g. granite outcrops of Western Australia: Journal of the Royal Society of Western Australia (1997)), others remain vastly under-represented in the scientific literature.
A prominent example of an extensive but understudied rocky landform system is the Mount Isa Inlier Interim Biogeographic Regions of Australia (IBRA) bioregion, which covers 67,782 km2 in north-west Queensland and a small adjacent portion of the Northern Territory (Fig. 1). The bioregion is dominated by a diverse array of ancient, persistent rocky landforms generally >1500 Ma in age (Betts et al. 2006) that account for >65% of the landscape (Fig. 1). Despite their ubiquity in the bioregion, these habitats and their associated fauna are generally poorly represented in the scientific literature, with most available research being conducted in association with mining activities (Griffiths 1998; Baker and Griffiths 2005; Perkins et al. 2009; Burnett et al. 2014, 2015). The bioregion is considered largely intact, but is affected by disturbance mechanisms, especially grazing, weed invasion, and modified fire regimes, that are likely to function antagonistically against ecosystem health in the bioregion under both current and future climate change scenarios (Crowley 2016). The rocky landforms of the bioregion are therefore likely to be of increasing importance to the biodiversity of the region. Further research into the value of these potentially critical habitats and the fauna they support is therefore warranted.
Extent of rocky and non-rocky landforms in the Mount Isa Inlier IBRA bioregion, with a terrain ruggedness overlay. ‘Rock’ refers to any non-regolith outcropping geology; ‘Regolith’ refers to deeply weathered duricrusted surfaces and palaeosols including ferricrete, silcrete and laterite, but may consist in part of deeply weathered sedimentary rocky landforms (‘jumpups’); ‘Sediment’ refers to colluvium, alluvium, sandplains and other unconsolidated deposits; and ‘Anthropogenic’ refers to deposits associated with human activity such as landfill and mining. Rocky landform data are shown only for portions of the Mount Isa Inlier within Queensland (Qld), as data of an equivalent scale are not available for the Northern Territory (NT). Terrain ruggedness is a ruggedness raster developed after Riley et al. (1999), with a colour gradient from transparent to black – the most rugged areas are visible as black polygons and can be seen to align with rocky geologies. The least rugged areas are transparent to allow for visualisation of the rocky landform layer beneath.
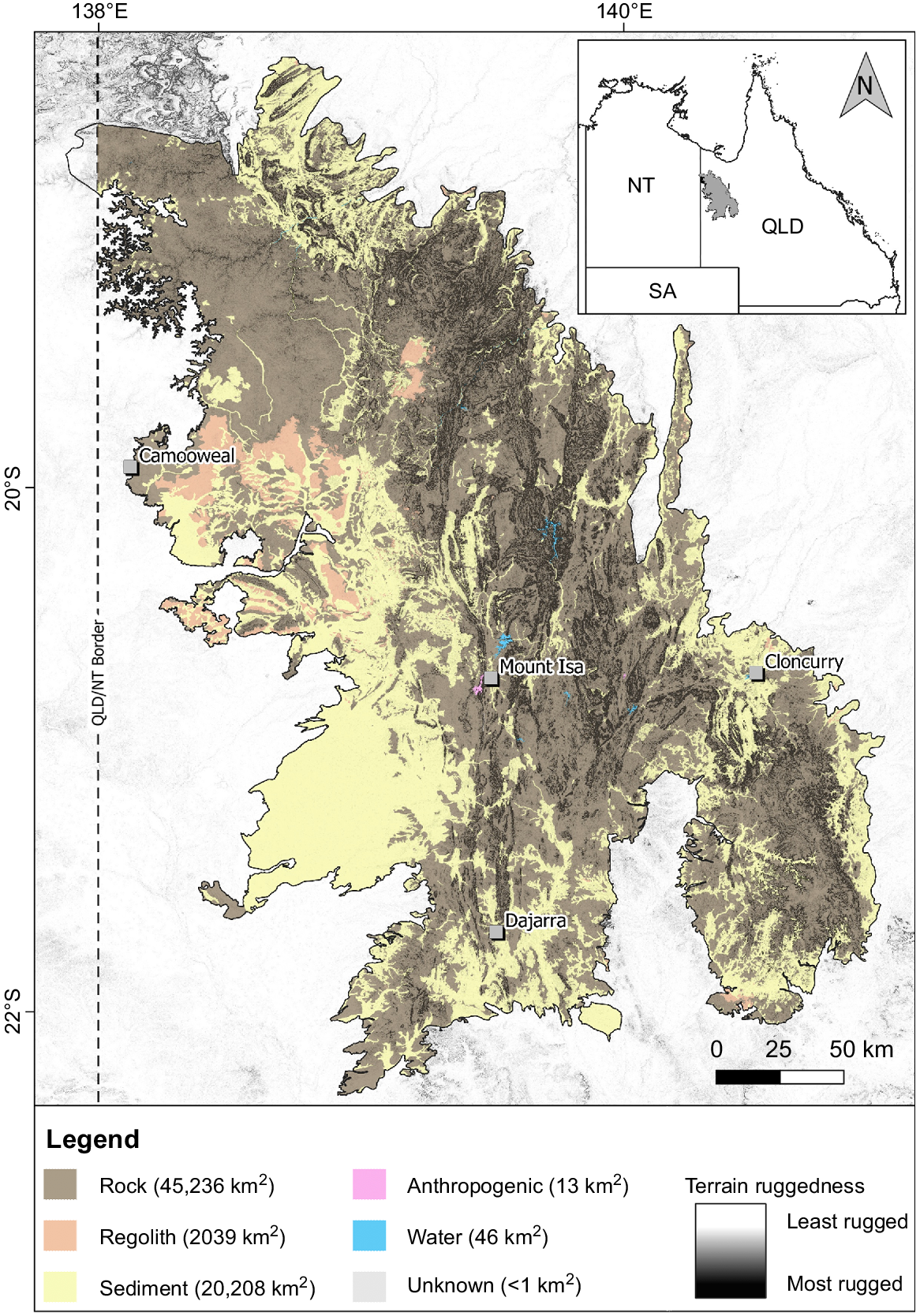
We conducted a 13-month study focused on the faunal assemblage inhabiting the rocky landforms of the Mount Isa Inlier. Because rocky landforms provide stable, persistent habitats over large timescales, we expected to find that these areas support a unique vertebrate fauna, i.e. a community of rocky landform specialists. For the purposes of this study, ‘community’ simply refers to a collection of fauna species utilising a shared habitat and is not intended to imply any level of trophic interaction or coevolution. Using a combination of camera trap surveys and occupancy analysis, we investigated (a) the fauna assemblage that utilises the rocky landforms of the bioregion and whether this indeed constitutes a specialist community, (b) how rates of use of rocky landforms by the community and by individual species change throughout the year, and (c) how rates of use of rocky landforms by the community and by individual species are affected by a suite of environmental characteristics.
Methods
Study area
The study area (Fig. 2) lies within Queensland’s Mount Isa Inlier bioregion and is characterised by hot, monsoonal summers, and dry winters with warm days and cool nights. Mean annual temperatures in the region are between 17.3 and 32°C (range = 8.7–37.3°C) (Bureau of Meteorology 2024). Rainfall is summer-dominant with an annual mean of 459.8 mm (Bureau of Meteorology 2024). Total survey area by minimum convex polygon was 11,523 km2.
The study area showing camera station locations, major access routes, and local terrain ruggedness (Riley et al. 1999) to show the extent of rocky landforms within the study area.
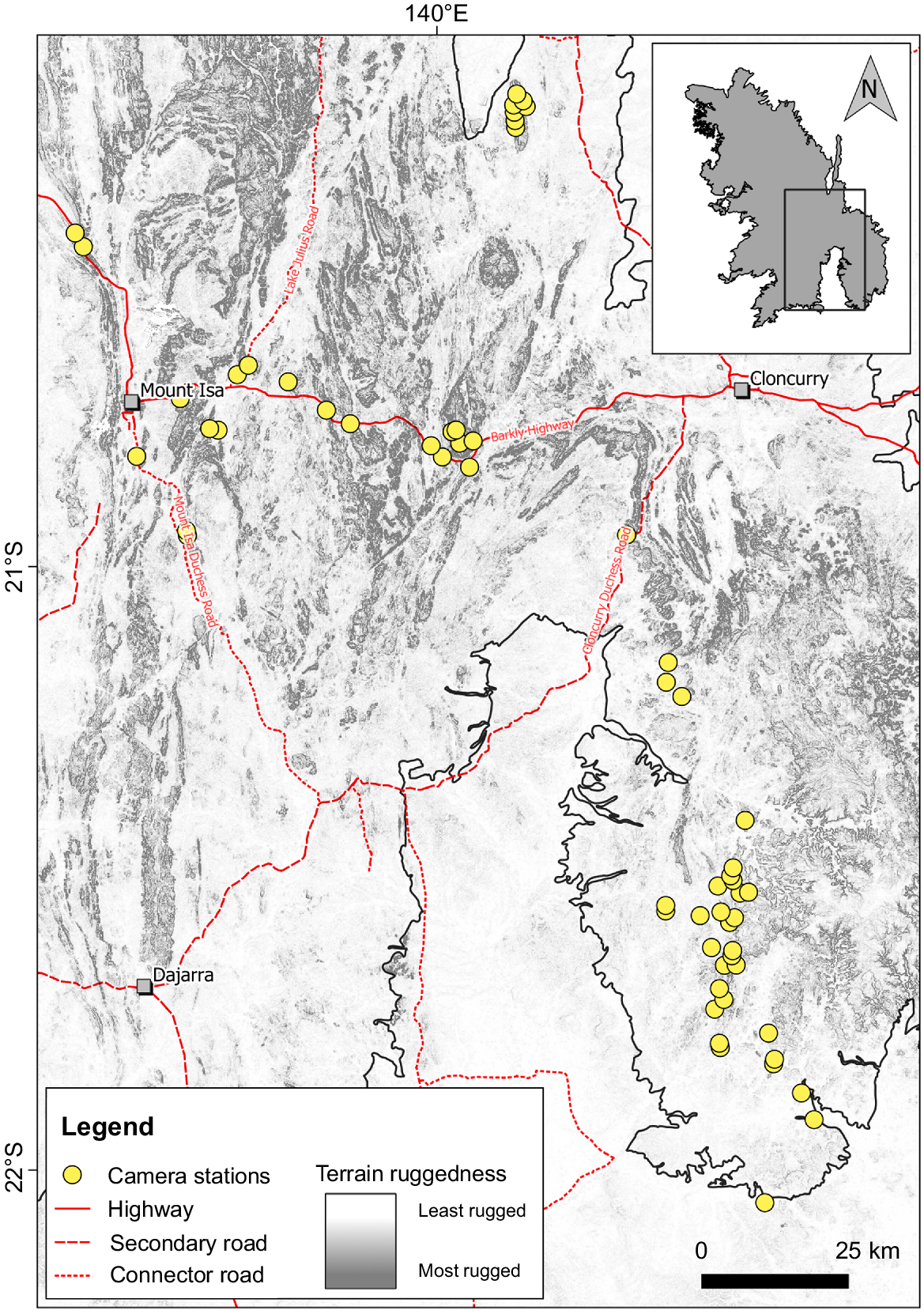
Survey conditions
Although temperatures across the study area are relatively stable from one location to another, rainfall is often patchily distributed and totals at one location may not reflect totals at another. Weather data was therefore obtained from three Bureau of Meteorology weather stations within the survey area (Mount Isa Airport station ID 029127, Cloncurry Airport station ID 029141, and Trepell Airport station ID 037036), and averages calculated across all three stations. The study commenced following a below-average wet season, with an average total rainfall of ~390 mm between October 2017 and April 2018 (Supplementary data Fig. S1). Average daily temperature during the survey fluctuated similarly to long-term means. Total rainfall exceeded the long-term mean with ~599 mm recorded (Fig. S1). Almost 60% of rain fell in February 2019 alone, and >90% from January to March 2019.
Field surveys
Field surveys investigated the presence of vertebrate fauna on rocky landforms over a 13-month period by repeated targeted surveys using ScoutGuard/BolyGuard SG562-C white-flash PIR camera traps. Cameras were set to 24 h operation, ‘high’ sensitivity, three captures per trigger and a 0 s delay between photographs within each trigger. This camera has a trigger speed of 1.2 s and an in-built delay period of 15 s between trigger events; however, field testing showed that, with the chosen settings, the delay period between triggers was ~30 s. Several cameras were inadvertently set to video mode for at least one sampling period during the survey. These data were omitted from analysis.
We undertook a total of nine sampling periods at 60 camera stations (Fig. 2), all on rocky landforms and each consisting of a single camera. Intercamera station distance was between 772 and 24,462 m (mean ± s.d. = 3265 ± 4026 m). To assess whether camera stations were spatially independent we collated home range data for all identified specialists (or their analogues if no data were available: Supplementary data Table S1). Home range is generally more readily available than ranging distance or dispersal data and still provides some context to the likely maximum area covered by an individual. Home range size cannot account for occasional long-range movements of some taxa (e.g. Dickman et al. 1995; Higgins and Davies 1996; Puckey et al. 2004; Sharp 2009; Burnett et al. 2023), but such movements are typically stochastic (e.g. in relation to rainfall) and difficult to control for. Home range size also provides no information on home range shape, which can take many forms and is difficult to standardise. Noting these limitations, we calculated a hypothetical circular home range with a 386 m radius (equating to a minimum 772 m between camera stations), giving a minimum non-overlapping area of 46.8 ha between adjacent cameras. This calculation suggests that all camera stations could be considered spatially independent for all rocky landform specialists except the euro (Osphranter robustus erubescens) and potentially the purple-necked rock-wallaby (Petrogale purpureicollis). On average, however, camera stations were much further apart than 772 m, with a mean of >3 km, suggesting sufficient spacing to satisfy an assumption of spatial independence for all specialists.
Cameras were initially deployed and baited between 17 and 26 May 2018 and were operated continuously until 10 June 2019 (Supplementary data Table S2). During this period, cameras were serviced (batteries and memory cards changed) and rebaited on eight occasions with a service interval ranging from 27 to 68 days (mean ± s.d. = 50.88 ± 11.72 days). Bait consisted of a simple peanut butter lure (approximately 125 g) smeared directly onto the rock surface in the centre of the camera’s field of view. In each sampling period, between 56 and 60 camera stations were active simultaneously, over a total of 21,965 camera days (Table S2). Cameras were mounted horizontally on either a Joby GorillaPod 3 K tripod (Vitec Imaging Solutions, Italy; n = 46), star picket (n = 7) or tree (n = 7) depending on station-specific mounting opportunities. Distance to bait varied between 90 and 159 cm from bare rock (mean ± s.d. = 115.46 ± 13.56 cm). Regardless of the mounting method, cameras were deployed with the lens ~20 cm above the ground surface. Where necessary, the area within a camera’s field of view was cleared of vegetation to reduce the incidence of false triggers from vegetation movement. In August 2018, cameras were fitted with heat shields made from air-cell insulation (Kingspan Insulation, Australia) to protect them from extreme temperatures; however, these were later removed after interference from corvids (Corvus spp.) which resulted in cameras becoming dislodged.
Environmental covariates
Seven environmental covariates were recorded for each camera station (Table 1). Lithology, landform and plant species richness were recorded in the field. In some instances, landform could not be determined from visual assessment in the field and was later confirmed by assessment of aerial imagery. Elevation, aspect and ruggedness were recorded for each camera station in a geographic information system (GIS) environment (QGIS Development Team 2020).
Variable | Levels (N) | Description | |
---|---|---|---|
Lithology | Sedimentary (11), Metamorphic (38), Igneous (11) | Basic lithological composition of the landform on which each camera station was located | |
Landform | Outcrop (3), Boulders (8), Jumpup (7), Hill (15), Ravine (2), Ridge (21), Scarp (4) |
| |
Elevation | N/A | Height above sea level in metres extracted for each camera station from 1 s (~30 m) digital elevation model (DEM; Gallant et al. 2011) | |
Aspect | North (12), East (12), South (14), West (22) | Compass direction of the raster cell in which a camera station was located, extracted from an aspect raster derived from the DEM. The raw raster provides aspect as a bearing (between 0 and 359°) and aspect levels were determined in 90° intervals: North 315–45°, East 45–135°, South 135–225°, West 225–315° | |
Ruggedness | N/A | A terrain ruggedness value (Riley et al. 1999) extracted for each camera station from a ruggedness raster derived from the DEM. For any given raster cell, the greater the difference in elevation between that cell and its eight neighbouring cells, the more rugged that cell is deemed to be | |
Plant species richness | N/A | Total number of plant species within a 25 m radius of each camera station that subjectively represented at least 10% of the cover for each stratum (ground, shrub, tree) |
Rocky landform specialist species selection
Not all species observed on rocky landforms are necessarily rocky landform specialists (i.e. saxicolous species). Additionally, the likelihood of recording non-target species on camera during this study was increased by baiting cameras with peanut butter, which is likely to attract a range of species not confined to rocky landforms. All taxa identified in camera trap images were therefore identified as rocky landform specialists or non-specialists. We adhere to the definition used by Freeland et al. (1988) in defining a rocky landform specialist as a species with a consistent association with rocky habitats across its entire distribution, and we support our classification of each rocky landform specialist through available evidence in the scientific literature (Table 2). Non-specialists were all species recorded for which this consistent association with rocky habitats was either not reported or not supported by the scientific literature. Further analysis was conducted only on taxa classified as rocky landform specialists. We also determined the dietary niche of rocky landform specialists, either from direct reports of a species’ diet, or inferred from the reported diet of congeners (Table 2).
Group | Species | Total detections | Diet | Reference(s) | ||||
---|---|---|---|---|---|---|---|---|
Herbivore | Granivore | Insectivore | Carnivore | |||||
Birds | Amytornis ballarae* Kalkadoon grasswren | 194 | ∘ | • | Noske (1992)B, Brooker (1998)B, Harrington et al. (2017)A | |||
Geophaps plumifera leucogaster Spinifex pigeon | 13 | ∘ | • | Frith and Barker (1975)B, Johnstone (1981)A | ||||
Mammals | Osphranter robustus erubescens Euro | 269 | • | Telfer and Bowman (2006)B, Telfer et al. (2008)A, Creese et al. (2019)B | ||||
Petrogale purpureicollis Purple-necked rock-wallaby | 2309 | • | Eldridge and Johnson (2023)A, Papp (2023)B | |||||
Pseudantechinus mimulus Carpentarian pseudantechinus | 2493 | • | ∘ | Burnett et al. (2014)A, Burnett et al. (2023)B | ||||
Zyzomys argurus Common rock rat | 19,195 | • | • | Watts (1977)B, Begg and Dunlop (1985)B, Woinarski et al. (1992)A | ||||
Reptiles | Ctenophorus slateri Slater’s ring-tailed dragon | 24 | • | Storr (1967)A, James (1991)B, Twigg et al. (1996)B, Goodyear and Pianka (2011)B, Pianka (2014)A | ||||
Ctenotus lateralis* Gravelly-soil Ctenotus | 172 | • | Storr (1978)A, Pianka (2014)B | |||||
Egernia hosmeri Hosmer’s spiny-tailed skink | 736 | • | Shea (1995)B, Cogger (2018)A | |||||
Gehyra robusta* Robust dtella | 444 | • | Henle (1990)B, Cogger (2018)A, Nordberg et al. (2018)B | |||||
Liasis olivaceus olivaceus Olive python | 4 | • | Shine and Slip (1990)B, Cogger (2018)A | |||||
Nephrurus eromanga* Eromanga Basin knob-tailed gecko | 0 | • | How et al. (1990)B, Oliver et al. (2021)A | |||||
Pseudechis pailsei* Eastern dwarf mulga snake | 4 | • | Shine (1977)B, Cogger (2018)A | |||||
Varanus acanthurus Ridge-tailed monitor | 282 | • | ∘ | King (2008)B, Cogger (2018)A | ||||
Varanus glebopalma Black-palmed rock monitor | 51 | ∘ | • | James et al. (1992)B, Sweet (1999)A |
‘Total detections’ is the number of independent detections of each species within the 21-day sampling window based on a 15-min independence interval. References with superscript ‘A’ support a species’ classification as a rocky landform specialist. References with superscript ‘B’ are dietary studies. Asterisks (*) denote species for which no dietary studies are available; their diet was inferred from available studies on congeners. Filled circles (•) indicate predominant dietary habit. Open circles (∘) indicate supplementary dietary habit.
Multispecies multiseason occupancy model
Camera trap data for each sampling period were truncated to the first 21 days to match the duration of the shortest sampling period (May 2019). Total effort of this truncated dataset was 10,966 camera days. We produced a detection database of rocky landform specialists in the R package camtrapR (Niedballa et al. 2016) using an independence interval of 15 min and retained all species with ≥10 detections across the entire study for further analysis. An independence interval of 15 min was chosen based on analysis of a histogram of detections of all analysed species at increasing time from the previous detection, which showed a clear peak for all species at <5 min (Supplementary data Fig. S2). We assumed that any detection interval beyond 5 min was therefore a suitable independence level and selected 15 min as a conservative interval. Species detection data were used to produce detection histories with an occasion length of 7 days to reduce computing time. Detection histories were subsequently used to develop a multispecies community occupancy model using the communityModel function in camtrapR (Niedballa et al. 2016). This function is generally utilised for developing single-season occupancy models and does not allow for dynamic multiseason models; however, by partitioning detection data into discrete sampling periods we were able to produce a multispecies multiseason occupancy model by including survey as a random effect (J. Niedballa pers. comm., Leibniz Institute for Zoo and Wildlife Research, April 2024). We also incorporated site-level covariates of aspect, basic lithology, landform, ruggedness and plant species richness. We omitted elevation from our analysis as it showed a significant moderate correlation with ruggedness (Kendall’s tau, τb = 0.21, P < 0.0001), and we deemed ruggedness to be more ecologically relevant. All covariates were included as random effects in the model to calculate species-specific occupancy estimates for each covariate alongside community occupancy estimates. Random effects were included for occupancy and detection probability intercepts to give species-specific responses for these parameters. The final model was implemented in the JAGS framework within camtrapR (Niedballa et al. 2016) using 15,000 iterations with a burn-in of 3000 across three chains. The model was tested for convergence with the Gelman–Rubin statistic (values <1.1 indicate convergence: Gelman et al. 2004; Supplementary data Table S3) and visual assessment of trace plots.
Significance of occupancy modelling was determined by analysis of 95% credible intervals of effect sizes (95% CIs not including zero are considered significant). Because baited camera traps are effectively a point survey with an indeterminate area of influence, occupancy estimates derived from studies utilising them should more correctly be considered estimates of site utilisation unless the methodology explicitly considers home range size, population density and dispersal ability of the target species (Mackenzie 2005; Efford and Dawson 2012; Rovero et al. 2013). Therefore, we consider effect sizes (±95% CIs) in this study as determining whether a given covariate significantly affects changes in the proportion of the study area utilised (hereafter ‘rates of use’), rather than affecting true occupancy rates.
Results
Rocky landform specialists
Camera trap surveys recorded 34,861 independent detections of 70 taxa that could be identified to at least genus level (Fig. 3; Supplementary data Table S4; Table S5). We identified 15 taxa as rocky landform specialists and recorded 31,672 detections of these taxa across the entire survey (~91% of all detections). Over the complete survey period, rocky landform specialists had a detection rate (number of camera days detected per 100 camera days) of 3.06 (±6.26) compared to 0.18 (±0.89) for non-specialists (Table S4).
Number of detections (square root-transformed) of rocky landform specialists and non-specialists recorded on camera traps during the complete study period. Only binomial nomenclature is provided in the interests of space. Common names for all taxa are given in Tables S4 and S5. Values at the end of each bar are actual total detections based on a 15 min independence interval. (*) Based on field observations, Corvus sp. are almost certainly C. coronoides, but C. orru and C. bennetti also occur in the bioregion and were considered too difficult to differentiate in camera trap imagery. (^) Cryptoblepharus sp. includes the rocky landform specialist C. zoticus, which occurs syntopically with the non-specialists C. australis and C. pannosus and was too difficult to differentiate in camera trap imagery in this study. (#) Denotes invasive species.
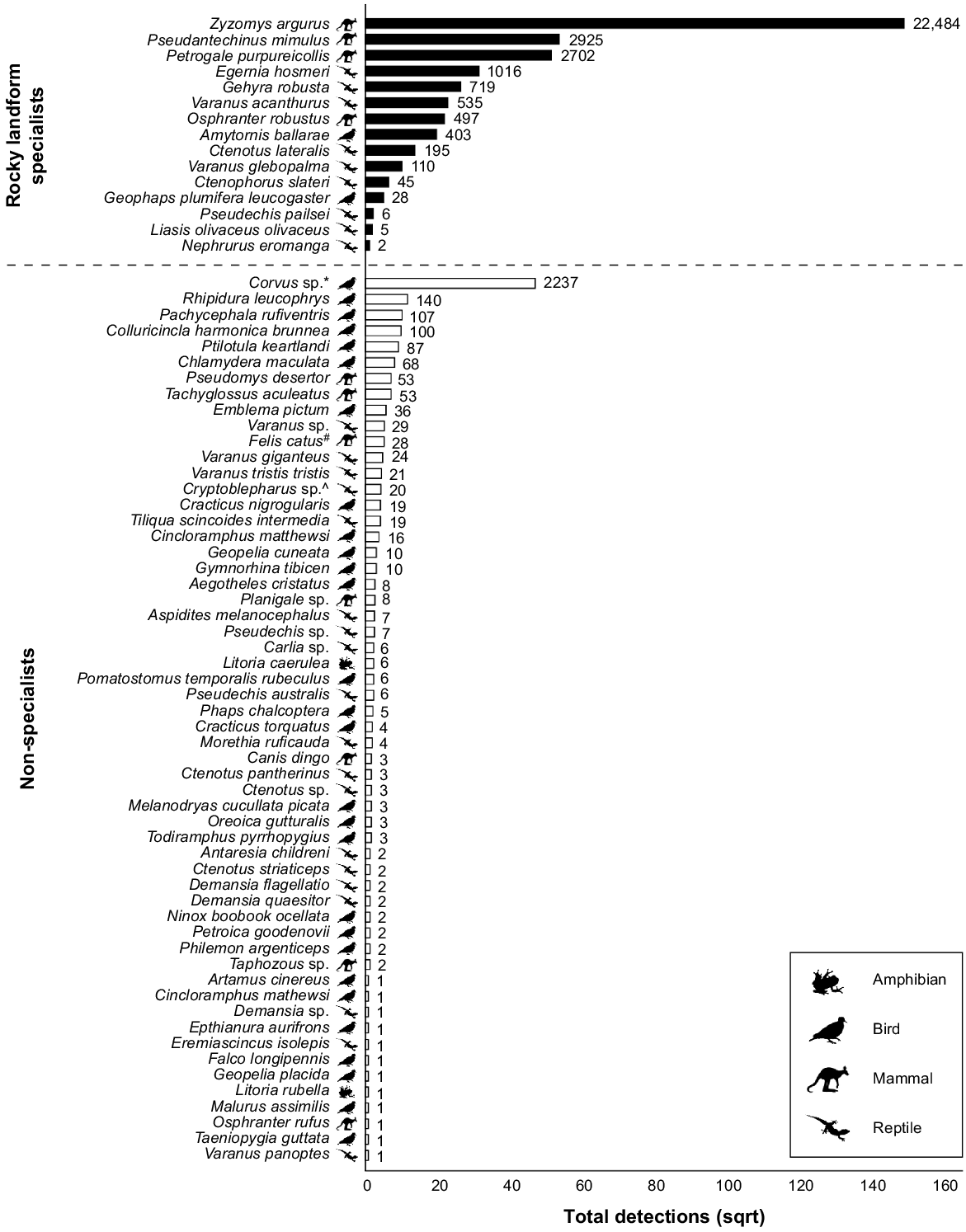
We recorded 28,546 detections of 59 taxa within the 21-day sampling window, of which 26,190 were of 14 rocky landform specialists (~92% of all detections within this window). Of the 14 specialists, 12 had a sufficient number of detections (i.e. ≥10) to support further investigation (Table 2). The final rocky landform specialist community analysed consisted of two birds, four mammals, and six reptiles (Table 2).
Sampling period effects
There was a significant effect of sampling period on community rates of use (indicated by effect size 95% CIs), with a positive effect of the November 2018 sampling period and a negative effect of the April 2019 sampling period compared to the initial sampling period in May 2018 (Fig. 4). For individual rocky landform specialists, sampling period had a significant effect on rates of use for nine out of 12 species (indicated by effect size 95% CIs) (Fig. 5).
Effect size (correlation coefficient) of sampling period and environmental covariates on estimates of utilisation rates (±95% credible interval) by the community. Credible intervals that do not include zero are depicted by filled circles and indicate a significant effect of a covariate (for continuous variables) or a significant effect of a covariate level compared to the reference covariate level (for categorical variables) on estimates of utilisation rates by the community.
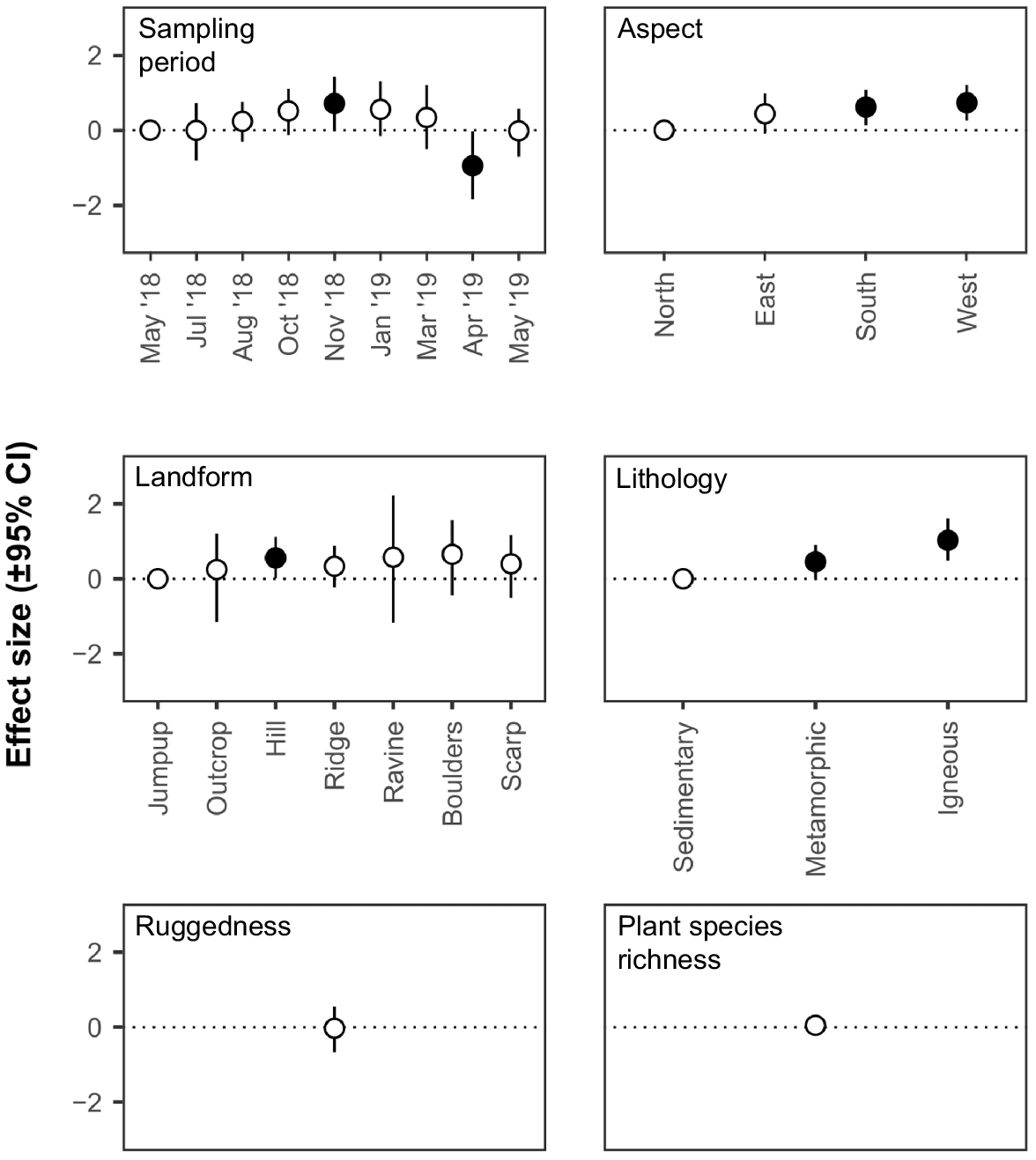
Effect size (correlation coefficient) of sampling period and environmental covariates on estimates of utilisation rates (±95% credible interval) by individual rocky landform specialists. Credible intervals that do not include zero are depicted by filled circles and indicate a significant effect of a covariate (for continuous variables) or a significant effect of a covariate level compared with the reference covariate level (for categorical variables) on estimates of each species’ utilisation rates.
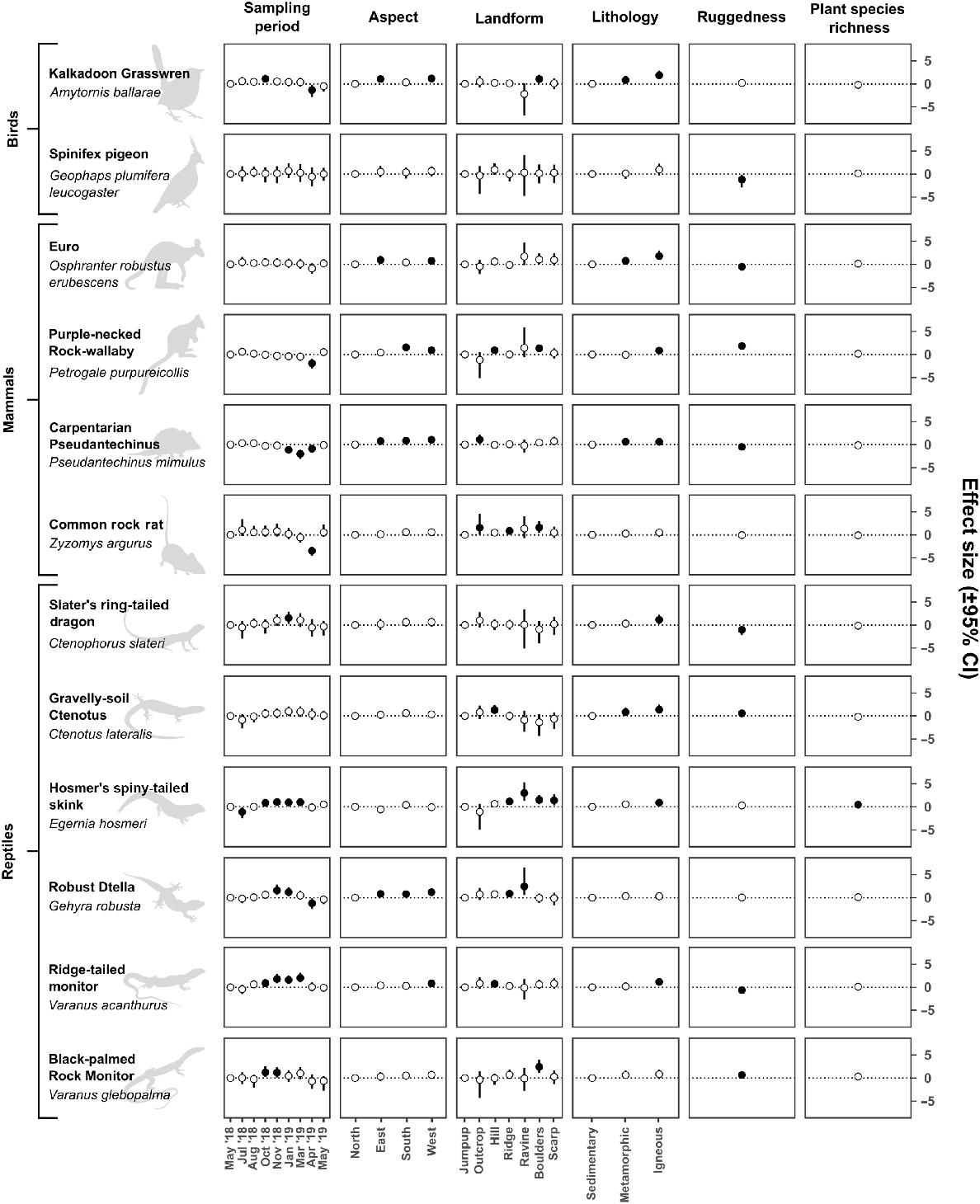
Environmental covariate effects
There was a significant effect (indicated by effect size 95% CIs) of aspect on estimates of community rates of use (Fig. 4) and for six individual species (Fig. 5). At the community level, north-facing slopes were utilised less frequently than south- and west-facing slopes. At the species level, if aspect had a significant effect on a species, there was always at least a significant positive effect of west-facing slopes compared with south-facing slopes. If there was additionally a significant effect of south- and/or east-facing slopes the effect was always positive compared with north-facing slopes.
There was a significant effect (indicated by effect size 95% CIs) of lithology on estimates of community rates of use (Fig. 4) and for eight individual species (Fig. 5). At the community level there was a significant positive effect of metamorphic and igneous geologies compared with sedimentary geologies. At the species level, if lithology had a significant effect on a species, there was always at least a positive effect of igneous lithologies compared with sedimentary ones. If there was additionally a significant effect of metamorphic lithologies the effect was always positive compared with sedimentary ones.
There was a significant positive effect (indicated by effect size 95% CIs) on estimates of community rates of use on hills compared with jumpups (Fig. 4). Landform had a significant effect on nine individual species (Fig. 5), with each showing a significant positive response to one or more landform types compared with jumpups. No landform type appeared to be consistently important between species.
Ruggedness had no effect (indicated by effect size 95% CIs) on estimates of community rates of use (Fig. 4) but had a significant positive effect (i.e. rates of use increased with increasing ruggedness values) for three species and a significant negative effect (i.e. rates of use increased with decreasing ruggedness values) for five species (Fig. 5).
Plant species richness had no effect (indicated by effect size 95% CIs) on estimates of community rates of use (Fig. 4) but had a significant positive effect (indicated by effect size 95% CIs) on one species (Fig. 5).
Discussion
This study presents the first known investigation into the rock-dwelling fauna community of the extensive and ancient rocky landforms of the Mount Isa Inlier and describes the first large-scale effort to determine how and when this diverse community of vertebrate fauna utilise these habitats. We expected to find a unique community utilising these landforms, and our data suggest that this is certainly the case. Significant effects at the community level point to factors, both temporal and spatial, that are important for the majority of species within the community. Namely, our study highlights the peaks and troughs in rates of use of rocky landforms throughout the year, and the importance of a range of environmental variables in either increasing or depressing rates of use of rocky landforms at both the community and species level. These factors are discussed in further detail below, and highlight potential considerations for monitoring programs, land management and conservation planning for fauna biodiversity across the bioregion.
Although rocky landforms were utilised by specialists and non-specialists alike, mean detections and detection rates of specialists were an order of magnitude greater than those of non-specialists. Notably, this was driven primarily by the common rock rat (Zyzomys argurus); however, even when omitting this species, mean detections of specialists were still three times greater than those of non-specialists and detection rates were eight times higher. Additionally, corvids accounted for the majority (~70%) of detections of non-specialists. Corvids are intelligent, opportunistic scavengers, and we regularly recorded their presence at cameras within only a few hours of rebaiting, suggesting that they may have learned to use our cameras as an easy food source. Given the predominance of rocky landforms throughout the Mount Isa Inlier, it is not unexpected to record non-specialists utilising these areas (especially avian or highly mobile species) but, after accounting for the presence of corvids, our data suggest that this usage is occasional at best and shows a clear difference in rates of use of rocky landforms between specialists and non-specialists.
Rocky landform specialists were recorded at all times of year, but rates of use varied by sampling period at both the community and species level. Non-significant effect sizes in most sampling periods suggest that the community utilises rocky landforms at similar rates throughout the year, with a significant increase in November and a significant decrease in April. As a whole, the community utilised nearly every measured facet of rocky habitat in our study area at similar rates, as indicated by non-significant effect sizes for most variables, and this was driven by varying importance of environmental characteristics at the species level.
From the reference sampling period in May 2018 the community experienced a significant increase in rates of use during the November 2018 sampling period, and a significant decrease during the April 2019 sampling period. The effect size of the initial May 2019 sampling period compared to the final May 2018 sampling period was effectively zero, indicating that rates of use of rocky landforms across the study area were equivalent between both sampling periods, and may subsequently point to an annual pattern. Unfortunately, we cannot draw such long-term inferences from a single year-long study, and it is highly likely that environmental conditions (e.g. rainfall) play a role in annual population dynamics (e.g. Dickman et al. 1999). Additional survey effort over multiple years and environmental conditions would provide clearer evidence of trends.
The observed increase in rates of use in November was driven primarily by significant positive effect sizes for most reptile species (the most speciose group we recorded) during this sampling period, whereas the observed decrease in April was driven by a significant negative effect on species across multiple classes, but especially mammals. In the seasonally dry tropics, within which our study area was located, November corresponds to the ‘late dry season’ and April corresponds to the ‘late wet season’. None of the specialists we assessed are migratory or nomadic, so it is likely that significant effect sizes at these times are due to changes in population dynamics in response to local environmental conditions and resource availability. November is around the onset of the reproductive season for a range of reptile taxa (James and Shine 1985), and reptiles are therefore more likely to be mobile while searching for mates. The November sampling period also had the hottest conditions recorded during our study, with a daily average maximum of 46°C (Bureau of Meteorology 2024). Because high temperatures increase the likelihood of recording reptiles (Read and Moseby 2001; Spence-Bailey et al. 2010), this will also lead to increased estimates for utilisation rates. The detectability and trappability of other taxa, especially mammals, also increases after prolonged dry periods, possibly due to decreased food abundance in the landscape, making baited traps more appealing (Geyle et al. 2019; Si et al. 2023), or an influx of less experienced and less cautious juveniles into the population (Geyle et al. 2019). Home range sizes may also vary in size between the wet and dry seasons. For example, allied rock-wallabies (Petrogale assimilis) have larger home ranges in the dry season, increasing rates of use of the landscape, but often shelter in rock piles during rain events, decreasing rates of use (Horsup 1994). This pattern is also likely to be the case for purple-necked rock-wallabies (P. purpureicollis) within the study area. The negative effect of the April 2019 sampling period was particularly dramatic on Z. argurus, which showed a four-fold negative effect size compared with May 2018, consistent with earlier population studies on the species (Begg 1981; Bradley et al. 1988). We cannot comment directly on the mechanism behind this observed decline; however, similar observed declines have been attributed to mortality (Bradley et al. 1988), and to changes in social behaviour, with individuals moving less during the reproductive period (Begg 1981). Similar trends may exist for other members of the community, but such data are currently unavailable.
Environmental covariate effects
Slope aspect affects abiotic factors such as light, heat, moisture and soil composition (Bale et al. 1998; Burnett et al. 2008; Nyman et al. 2015), and the underutilisation of northerly slopes may therefore reflect suboptimal conditions for the community. In the Mount Isa Inlier, the sun tracks north over most of the year, resulting in relatively higher levels of solar radiation hitting northern slopes. Although rocky landforms typically provide sheltering opportunities such as cracks and crevices that may help maintain stable thermal regimes (Shi et al. 2015; Millar and Hickman 2021), shelter sites that are too deep, shallow, thick or thin can be unsuitable as daily refuges and can even lead to mortality (Huey et al. 1989). The reduction in rates of use of northern slopes may therefore be because shelter sites on these slopes have less suitable morphology and are unable to effectively buffer incoming solar radiation. Further research on shelter sites of rocky landform specialists and the thermal regimes of these shelter sites are necessary to shed any further light on this possibility. Other studies from the southern hemisphere have shown that south-facing slopes are cooler than north-facing slopes in spring and summer (Speziale and Ezcurra 2015), and steep landforms with a higher concentration of south-facing slopes have stronger features of ecological refugia (i.e. to which a species can retreat, persist in and expand from: Buschke et al. 2020). In the Yarra Ranges of Victoria, northern slopes are significantly hotter than southern ones, with western and eastern slopes in between (Nyman et al. 2015). A similar pattern likely occurs in north-west Qld, so eastern and western slopes may receive enough of a reduction in solar radiation within the Mount Isa Inlier to be ecologically equivalent to south-facing aspects. Alternatively, east- and south-facing slopes may also provide basking sites for reptiles, as well as some mammals (Geiser et al. 2002; Creese et al. 2019), during the cooler parts of the day towards sunrise and sunset respectively, leading to increased rates of use of these areas compared with north-facing slopes.
The importance of metamorphic and igneous lithologies compared with sedimentary ones, at both the community and species level, highlights the importance of these broad lithology groupings. This trend may reflect the proportional availability of finer-scale categories of lithology and the ages and varying propensity of these geologies to weather and break down to form suitable sheltering and denning sites within the study area (Twidale and Campbell 2005). Sedimentary landforms surveyed in this study are predominantly laterite jumpups, which are extremely old and weathered and provide few sheltering opportunities. By contrast, igneous and metamorphic landforms generally consist of complex, often deeply fissured habitat with an abundance of sheltering and denning opportunities (e.g. granite boulder piles and quartzite scree).
Our data suggest that all landforms across the bioregion are utilised at similar rates by the community, except for a small positive increase in rates of use of hills compared with jumpups. Additionally, there were significant effects of landform on nine specialists, but these effects were not consistent among specialists. Unfortunately, detailed analysis of the true importance of some landform types (notably ‘outcrop’ and ‘ravine’) was hampered by small sample sizes, resulting in large credible intervals overlapping zero, especially at the community level. As discussed above, jumpups in the bioregion are predominantly composed of laterite, which is extremely weathered and provides relatively few sheltering opportunities. Beyond this, it is difficult to comment on why certain landforms may increase or depress rates of use, but it is likely that it is a result of a combination of site characteristics missing from our broadscale assessment, such as the abundance of preferred dietary items, water availability, vegetative cover, and presence of shelter sites (e.g. Soderquist and Nally 2000; Blevins and With 2011; Hohnen et al. 2016a). Our study therefore highlights landforms that may be potentially important for a range of rocky specialists, but further research is required to determine exactly why they are important.
Ruggedness did not have a significant effect on rates of use by the community, indicating that areas of low, moderate and high ruggedness were utilised at similar rates across the study area. This was in part driven by the importance of ruggedness at the species level, with a positive effect on three species and a negative effect on a further five. Rugged areas provide higher habitat value than less rugged areas due to the increased availability of den sites (e.g. Hernandez-Santin et al. 2022) and protection from feral predators (McDonald et al. 2017; McDonald et al. 2020). It was therefore surprising to find a negative effect on five specialists, especially given four of these (spinifex pigeon, Geophaps plumifera leucogaster; Carpentarian pseudantechinus, Pseudantechinus mimulus; Slater’s ring-tailed dragon, Ctenophorus slateri; and ridge-tailed monitor, Varanus acanthurus) may at least occasionally form part of feral cats’ (Felis catus) diet (Doherty et al. 2015). This relationship may be explained by the fact that, as this study was focused on rocky landforms, we did not survey any areas that could truly be considered ‘not rugged’ (e.g. sandplains) for a true comparison. Feral cat occupancy decreases with increasing ruggedness and habitat complexity (Hohnen et al. 2016b; McDonald et al. 2020), and we recorded a generally low number of detections of cats throughout the study, possibly indicating avoidance of our camera stations by cats. Therefore, although five species may have higher rates of use within the less rugged parts of our study area, this may not be the case in truly non-rugged locations. Further comparative analysis of rates of use of non-rugged locations by rocky landform specialists and feral cats would provide a clearer picture of this hypothesis.
Plant species richness had a significant effect on only a single species. Rates of use by Hosmer’s spiny-tailed skink (Egernia hosmeri) were positively affected by increasing plant richness, and this may be due to the species’ diet. While there are other herbivorous species within the community, E. hosmeri appears to have a more varied diet including leaves, stems, berries and seeds (Shea 1995) compared with the grass- and forb-dominated diets of the euro (O. r. erubescens) and P. purpureicollis (Telfer and Bowman 2006; Creese et al. 2019; Papp 2023) and the seed-dominated diets of G. p. leucogaster and Z. argurus (Frith and Barker 1975; Watts 1977; Begg and Dunlop 1985). Our limited data suggest that sites with increased plant richness may therefore be necessary for E. hosmeri to meet its dietary requirements, but further research is required in a range of areas, including further detailed dietary analysis, comprehensive floristic surveys, and phenological studies.
Our study is the first known attempt to quantify and explore the associations of the rocky landform specialist fauna community of the Mount Isa Inlier. It is difficult to assess how many studies have been conducted in the bioregion, as many are restricted to grey literature in relation to resource extraction and exploration and are therefore not publicly available. Of those studies that are accessible, they have typically been limited to single species and/or short study windows. Single season surveys may not provide a complete picture of a fauna community, especially if members of that community are rare or have low mobility (Thompson et al. 2010). Our study demonstrates this, with the Eromanga Basin knob-tailed gecko (Nephrurus eromanga) not being recorded until the sixth sampling period in January 2019. Even relatively long surveys such as ours may miss species. We know of several rocky landform specialists within the Mount Isa Inlier that were not recorded during our survey despite the significant amount of survey effort. In some instances, this may be random chance, especially if a species is rare or has low mobility, but in other instances our methodologies may have been unsuitable for recording all specialists. Camera traps are generally more effective at detecting a wide range of taxa compared with other census techniques (Wearn and Glover-Kapfer 2019), but our use of this single methodology almost certainly led to an underestimate of certain taxa such as bats and small, cryptic reptiles. Additionally, cameras were baited with peanut butter as it is a cheap and readily available product and is commonly utilised as a universal bait in camera trap studies. However, it is likely that the exclusive use of peanut butter biased our estimates towards those species that are known to be attracted by this type of bait, namely small- to medium-size mammals (Paull et al. 2011; Diete et al. 2016; Goldingay 2024). Other taxa may respond more favourably to other bait types, as shown by others (e.g. Davis et al. 2008; Sebastián-González et al. 2020). It is also possible that some taxa responded negatively to the peanut butter bait. Future studies of the community could explicitly test this hypothesis by comparing site utilisation estimates by using multiple bait types (including unbaited controls) and modelling this as a detection probability covariate.
Conclusions
The rocky landforms of the Mount Isa Inlier are a collection of complex, persistent habitats that support a unique rocky landform specialist community, as well as provide at least occasional utility for a range of non-specialist taxa. We provide the first evidence of how the community, as well as individual rocky landform specialists use this unique landscape through both time and space. Our results allow us to make some preliminary recommendations for future surveys or monitoring programs aimed at furthering our understanding of the community and/or the individual species of which the community is composed. Primarily, in instances where time, resources and personnel are limited (as they are for many fauna surveys) November (or, more broadly, the late dry season) appears to be the best time of year to maximise survey efficiency, as the greatest proportion of rocky landforms is being utilised. Targeted survey effort for individual species may be better suited to other times of year. Surveys will be less effective if conducted in April (or, more broadly, the late wet season) as utilisation rates are at their lowest for the community, and either at their lowest or not significantly different from other times of the year for individual species. Because western and southern slopes on metamorphic and igneous hills have a significant positive effect on community rates of use of the landscape, as well as for a majority of individual species, areas where these features predominate will be important for maximising future survey efficiency. Importantly, focusing on hillsides is likely to encompass other landform types, as these areas were typically mid-slope locations in our study, connecting the more complex scarps, ridges and ravines to lower-lying terrain. These areas should also be candidate locations for focused land management and biodiversity conservation through, for example, targeted invasive species control, exclusion of stock, and gazettal of protected areas with a view to maintain and enhance their value as refugia. While this study focused on factors affecting rates of use across the broader landscape, there is ample scope for future research to investigate finer-scale associations of the community and individual specialists, such as characterising shelter requirements and exploring niche overlap/separation.
Data availability
The data that support this study are not publicly available but may be shared upon reasonable request to the corresponding author if appropriate.
Conflicts of interest
Christofer Clemente is a co-Editor-in-Chief of Australian Journal of Zoology. To mitigate this potential conflict of interest he had no editor-level access to this manuscript during peer review. The author(s) have no further conflicts of interest to declare.
Declaration of funding
Funding and in-kind support was provided by EcoSmart Ecology, MMG Ltd and Chinova Resources Pty Ltd.
Acknowledgements
We acknowledge Aboriginal and Torres Strait Islands peoples as the Traditional Owners and custodians of the land and water on which we live, work, and rely. This study was conducted on Kalkadoon and Yalarrnga Country, and we pay our respects to their Elders past, present and emerging. We thank landholders (Ian and Marianna Campbell at Rifle Creek Station, James Lord at May Downs Station, MDH Pty Ltd, Chatsworth Station, McMillan Pastoral Company Pty Ltd, MMG Ltd and Chinova Resources Pty Ltd) for providing access to their properties or leases. We particularly acknowledge the field assistance efforts provided by volunteers (Kirrin Brown, Lorraine Evans, Jake Gardos, Bryce Haberer, Emily Kirton, Lisa Swales, Anna Tadros and Elizabeth Wemyss). All field research was conducted under approval from Queensland Department of Environment and Science (WISP05097908), and the University of the Sunshine Coast (ANS1748).
References
Bale CL, Williams JB, Charley JL (1998) The impact of aspect on forest structure and floristics in some Eastern Australian sites. Forest Ecology and Management 110(1–3), 363-377.
| Crossref | Google Scholar |
Begg RJ (1981) The small mammals of little Nourlangie rock, N.T IV.* Ecology of Zyzomys woodwardia, the large rock-rat, and Z. argurus, the common rock-rat, (Rodentia: Muridae). Australian Wildlife Research 8(2), 307-320.
| Crossref | Google Scholar |
Begg RJ, Dunlop CJ (1985) The diets of the large rock-rat, Zyzomys woodwardi, and the common rock-rat, Z. argurus (Rodentia: Muridae). Australian Wildlife Research 12(1), 19-24.
| Crossref | Google Scholar |
Benwell A (2007) Response of rock-outcrop and fringing vegetation to disturbance by fire and drought. Australian Journal of Botany 55(7), 736-748.
| Crossref | Google Scholar |
Betts PG, Giles D, Mark G, Lister GS, Goleby BR, Aillères L (2006) Synthesis of the Proterozoic evolution of the Mt Isa Inlier. Australian Journal of Earth Sciences 53(1), 187-211.
| Crossref | Google Scholar |
Blevins E, With KA (2011) Landscape context matters: local habitat and landscape effects on the abundance and patch occupancy of collared lizards in managed grasslands. Landscape Ecology 26, 837-850.
| Crossref | Google Scholar |
Bradley AJ, Kemper CM, Kitchener DJ, Humphreys WF, How RA, Schmitt LH (1988) Population ecology and physiology of the common rock rat, Zyzomys argurus (Rodentia: Muridae) in tropical northwestern Australia. Journal of Mammalogy 69(4), 749-764.
| Crossref | Google Scholar |
Braithwaite RW, Muller WJ (1997) Rainfall, groundwater and refuges: predicting extinctions of Australian tropical mammal species. Australian Journal of Ecology 22(1), 57-67.
| Crossref | Google Scholar |
Bureau of Meteorology (2024) Climate data online: station IDs 029127, 029141, 037036. Available at http://www.bom.gov.au/climate/data/ [accessed April 2024]
Burke A (2002) Island–matrix relationships in Nama Karoo inselberg landscapes. Part I: do inselbergs provide a refuge for matrix species? Plant Ecology 160, 79-90.
| Crossref | Google Scholar |
Burke A (2003) Inselbergs in a changing world – global trends. Diversity and Distributions 9(5), 375-383.
| Crossref | Google Scholar |
Burnett BN, Meyer GA, McFadden LD (2008) Aspect-related microclimatic influences on slope forms and processes, northeastern Arizona. Journal of Geophysical Research 113, F03002.
| Crossref | Google Scholar |
Buschke FT, Coetzer C, Pinceel T, Mehlomakhulu Z, Moreels N, du Randt L, Vanschoenwinkel B (2020) Mountains and rocky outcrops as ecological refuges in a high biodiversity working landscape. Biological Conservation 250, 108759.
| Crossref | Google Scholar |
Clarke PJ (2002) Habitat islands in fire-prone vegetation: do landscape features influence community composition? Journal of Biogeography 29(5–6), 677-684.
| Crossref | Google Scholar |
Couper PJ, Hoskin CJ (2008) Litho-refugia: the importance of rock landscapes for the long-term persistence of Australian rainforest fauna. Australian Zoologist 34(4), 554-560.
| Crossref | Google Scholar |
Creese S, Davies SJJF, Bowen BJ (2019) Comparative dietary analysis of the black-flanked rock-wallaby (Petrogale lateralis lateralis), the euro (Macropus robustus erubescens) and the feral goat (Capra hircus) from Cape Range National Park, Western Australia. Australian Mammalogy 41(2), 220-230.
| Crossref | Google Scholar |
Davis C, Fleming P, Craig M, Grigg A, Hardy G (2008) A funnel trap for capture of small arboreal reptiles. Amphibia-Reptilia 29, 413-423.
| Crossref | Google Scholar |
Dickman CR, Predavec M, Downey FJ (1995) Long-range movements of small mammals in arid Australia: implications for land management. Journal of Arid Environments 31(4), 441-452.
| Crossref | Google Scholar |
Dickman CR, Mahon PS, Masters P, Gibson DF (1999) Long-term dynamics of rodent populations in arid Australia: the influence of rainfall. Wildlife Research 26(4), 389-403.
| Crossref | Google Scholar |
Diete RL, Meek PD, Dixon KM, Dickman CR, Leung LK-P (2016) Best bait for your buck: bait preference for camera trapping north Australian mammals. Australian Journal of Zoology 63(6), 376-382.
| Crossref | Google Scholar |
Doherty TS, Davis RA, Van Etten EJB, Algar D, Collier N, Dickman CR, Edwards G, Masters P, Palmer R, Robinson S (2015) A continental-scale analysis of feral cat diet in Australia. Journal of Biogeography 42(5), 964-975.
| Crossref | Google Scholar |
Efford MG, Dawson DK (2012) Occupancy in continuous habitat. Ecosphere 3(4), 1-15.
| Crossref | Google Scholar |
Fitzsimons JA, Michael DR (2017) Rocky outcrops: a hard road in the conservation of critical habitats. Biological Conservation 211, 36-44.
| Crossref | Google Scholar |
Freeland WJ, Winter JW, Raskin S (1988) Australian rock-mammals: a phenomenon of the seasonally dry tropics. Biotropica 20(1), 70-79.
| Crossref | Google Scholar |
Frith HJ, Barker RD (1975) Food of the plumed pigeons, Geophaps plumifera and G. ferruginea. Australian Wildlife Research 2(1), 63-76.
| Crossref | Google Scholar |
Gallant JC, Dowling TI, Read AM, Wilson N, Tickle P, Inskeep C (2011) 1 second SRTM derived digital elevation models user guide. Available at www.ga.gov.au/topographic-mapping/digital-elevation-data.html [accessed 8 December 2020]
García MB, Domingo D, Pizarro M, Font X, Gómez D, Ehrlén J (2020) Rocky habitats as microclimatic refuges for biodiversity. A close-up thermal approach. Environmental and Experimental Botany 170, 103886.
| Crossref | Google Scholar |
Geiser F, Goodship N, Pavey CR (2002) Was basking important in the evolution of mammalian endothermy? Naturwissenschaften 89, 412-414.
| Crossref | Google Scholar | PubMed |
Geyle HM, Guillera-Arroita G, Davies HF, Firth RSC, Murphy BP, Nimmo DG, Ritchie EG, Woinarski JCZ, Nicholson E (2019) Towards meaningful monitoring: a case study of a threatened rodent. Austral Ecology 44(2), 223-236.
| Crossref | Google Scholar |
Goldingay RL (2024) The influence of bait and camera type on detection of a spectrum of medium-sized Australian mammals. Australian Mammalogy 46, AM23020.
| Crossref | Google Scholar |
Goodyear SE, Pianka ER (2011) Spatial and temporal variation in diets of sympatric lizards (genus Ctenotus) in the Great Victoria Desert, Western Australia. Journal of Herpetology 45(3), 265-271.
| Crossref | Google Scholar |
Harrington G, McKeown A, Venables B (2017) The range and habitat of the Kalkadoon grasswren Amytornis ballarae. Australian Field Ornithology 34, 12-17.
| Crossref | Google Scholar |
Henle K (1990) Population ecology and life history of the arboreal gecko Gehyra variegata in arid Australia. Herpetological Monographs 4, 30-60.
| Crossref | Google Scholar |
Hernandez-Santin L, Goldizen AW, Fisher DO (2022) Northern quolls in the Pilbara persist in high-quality habitat, despite a decline trajectory consistent with range eclipse by feral cats. Conservation Science and Practice 4(8), e12733.
| Crossref | Google Scholar |
Hohnen R, Tuft K, Legge S, Walters N, Johanson L, Carver S, Radfod IJ, Johnson CN (2016a) The significance of topographic complexity in habitat selection and persistence of a declining marsupial in the Kimberley region of Western Australia. Australian Journal of Zoology 64(3), 198-216.
| Crossref | Google Scholar |
Hohnen R, Tuft K, McGregor HW, Legge S, Radford IJ, Johnson CN (2016b) Occupancy of the invasive feral cat varies with habitat complexity. PLoS ONE 11, e0152520.
| Crossref | Google Scholar | PubMed |
Horsup A (1994) Home range of the allied rock-wallaby, Petrogale assimilis. Wildlife Research 21(1), 65-84.
| Crossref | Google Scholar |
How RA, Dell J, Wellington BD (1990) Reproductive and dietary biology of Nephrurus and Underwoodisaurus (Gekkonidae) in Western Australia. Records of the Western Australian Museum 14(4), 449-459.
| Google Scholar |
Huey RB, Peterson CR, Arnold SJ, Porter WP (1989) Hot rocks and not-so-hot rocks: retreat-site selection by garter snakes and its thermal consequences. Ecology 70(4), 931-944.
| Crossref | Google Scholar |
James CD (1991) Temporal variation in diets and trophic partitioning by coexisting lizards (Ctenotus: Scincidae) in central Australia. Oecologia 85, 553-561.
| Crossref | Google Scholar | PubMed |
James C, Shine R (1985) The seasonal timing of reproduction: a tropical–temperate comparison in Australian lizards. Oecologia 67, 464-474.
| Crossref | Google Scholar | PubMed |
James CD, Losos JB, King DR (1992) Reproductive biology and diets of goannas (Reptilia: Varanidae) from Australia. Journal of Herpetology 26(2), 128-136.
| Crossref | Google Scholar |
Johnstone RE (1981) Notes on the distribution, ecology and taxonomy of the partridge pigeon (Geophaps smithii) and spinifex pigeon (Geophaps plumifera) in Western Australia. Records of the Western Australian Museum 9, 49-64.
| Google Scholar |
Keppel G, Van Niel KP, Wardell-Johnson GW, Yates CJ, Byrne M, Mucina L, Schut AGT, Hopper SD, Franklin SE (2012) Refugia: identifying and understanding safe havens for biodiversity under climate change. Global Ecology and Biogeography 21(4), 393-404.
| Crossref | Google Scholar |
King D (2008) The diet and foraging strategy of Varanus acanthurus. Biawak 2(1), 11-17.
| Google Scholar |
Mackenzie DI (2005) Was it there? Dealing with imperfect detection for species presence/absence data. Australian & New Zealand Journal of Statistics 47(1), 65-74.
| Crossref | Google Scholar |
McDonald PJ, Nano CEM, Ward SJ, Stewart A, Pavey CR, Luck GW, Dickman CR (2017) Habitat as a mediator of mesopredator-driven mammal extinction. Conservation Biology 31(5), 1183-1191.
| Crossref | Google Scholar | PubMed |
McDonald PJ, Stewart A, Jensen MA, McGregor HW (2020) Topographic complexity potentially mediates cat predation risk for a critically endangered rodent. Wildlife Research 47(8), 643-648.
| Crossref | Google Scholar |
Michael DR, Lindenmayer DB, Cunningham RB (2010) Managing rock outcrops to improve biodiversity conservation in Australian agricultural landscapes. Ecological Management & Restoration 11(1), 43-50.
| Crossref | Google Scholar |
Michael DR, Cunningham RB, Lindenmayer DB (2011) Regrowth and revegetation in temperate Australia presents a conservation challenge for reptile fauna in agricultural landscapes. Biological Conservation 144(1), 407-415.
| Crossref | Google Scholar |
Millar CI, Hickman KT (2021) Camera traps provide insights into American pika site occupancy, behavior, thermal relations, and associated wildlife diversity. Western North American Naturalist 81(2), 141-170.
| Crossref | Google Scholar |
Niedballa J, Sollmann R, Courtiol A, Wilting A (2016) camtrapR: an R package for efficient camera trap data management. Methods in Ecology and Evolution 7(12), 1457-1462.
| Crossref | Google Scholar |
Noble C, Laver RJ, Rosauer DF, Ferrier S, Moritz C (2017) Phylogeographic evidence for evolutionary refugia in the Gulf sandstone ranges of northern Australia. Australian Journal of Zoology 65(6), 408-416.
| Crossref | Google Scholar |
Nordberg EJ, Murray P, Alford R, Schwarzkopf L (2018) Abundance, diet and prey selection of arboreal lizards in a grazed tropical woodland. Austral Ecology 43(3), 328-338.
| Crossref | Google Scholar |
Noske RA (1992) The status and ecology of the white-throated grasswren Amytornis woodwardi. Emu 92(1), 39-51.
| Crossref | Google Scholar |
Nyman P, Metzen D, Noske PJ, Lane PNJ, Sheridan GJ (2015) Quantifying the effects of topographic aspect on water content and temperature in fine surface fuel. International Journal of Wildland Fire 24(8), 1129-1142.
| Crossref | Google Scholar |
Oliver PM, Ashman LG, Bank S, Laver RJ, Pratt RC, Tedeschi LG, Moritz CG (2019) On and off the rocks: persistence and ecological diversification in a tropical Australian lizard radiation. BMC Ecology and Evolution 19, 81.
| Crossref | Google Scholar |
Oliver PM, Donnellan SC, Gunn BF (2021) Plio–Pleistocene vicariance across arid Australia in the ‘spiny knob-tailed geckos’ (Nephrurus asper group), with the description of a new species from western Queensland. Australian Journal of Zoology 69(6), 216-228.
| Crossref | Google Scholar |
Paull DJ, Claridge AW, Barry SC (2011) There’s no accounting for taste: bait attractants and infrared digital cameras for detecting small to medium ground-dwelling mammals. Wildlife Research 38(3), 188-195.
| Crossref | Google Scholar |
Pianka ER (2014) Notes on the ecology and natural history of Ctenophorus caudicinctus (Agamidae) in Western Australia. Western Australian Naturalist 29(3), 226-230.
| Google Scholar |
Puckey H, Lewis M, Hooper D, Michell C (2004) Home range, movement and habitat utilisation of the Carpentarian rock-rat (Zyzomys palatalis) in an isolated habitat patch. Wildlife Research 31(3), 327-337.
| Crossref | Google Scholar |
QGIS Development Team (2020) QGIS Geographic Information System. Version 3.14.16. [Software]. Available at http://qgis.osgeo.org (accessed October 2022)
Read JL, Moseby KE (2001) Factors affecting pitfall capture rates of small ground vertebrates in arid South Australia. I. The influence of weather and moon phase on capture rates of reptiles. Wildlife Research 28(1), 53-60.
| Crossref | Google Scholar |
Riley SJ, DeGloria SD, Elliot R (1999) A terrain ruggedness index that quantifies topographic heterogeneity. Intermountain Journal of Sciences 5(1–4), 23-27.
| Google Scholar |
Rovero F, Zimmermann F, Berzi D, Meek P (2013) “Which camera trap and how many do I need?” A review of camera features and study designs for a range of wildlife research applications. Hystrix, the Italian Journal of Mammalogy 24(2), 148-156.
| Crossref | Google Scholar |
Sebastián-González E, Morales-Reyes Z, Naves-Alegre L, Alemañ CJD, Lima LG, Lima LM, Sánchez-Zapata JA (2020) Which bait should I use? Insights from a camera trap study in a highly diverse cerrado forest. European Journal of Wildlife Research 66, 99.
| Crossref | Google Scholar |
Sharp A (2009) Home range dynamics of the yellow-footed rock-wallaby (Petrogale xanthopus celeris) in central-western Queensland. Austral Ecology 34(1), 55-68.
| Crossref | Google Scholar |
Shea GM (1995) Herbivory in Egernia hosmeri (Squamata: Scincidae). Herpetofauna 25, 8-11.
| Google Scholar |
Shi H, Paull D, Broome L, Bates H (2015) Microhabitat use by mountain pygmy-possum (Burramys parvus): implications for the conservation of small mammals in alpine environments. Austral Ecology 40(5), 528-536.
| Crossref | Google Scholar |
Shine R (1977) Habitats, diets, and sympatry in snakes: a study from Australia. Canadian Journal of Zoology 55(7), 1118-1128.
| Crossref | Google Scholar |
Shine R, Slip DJ (1990) Biological aspects of the adaptive radiation of Australasian pythons (Serpentes: Boidae). Herpetologica 46(3), 283-290.
| Google Scholar |
Si AY, Ross AK, Crisp HA (2023) Trapping efficiency of common brushtail possums is affected by rainfall. Australian Mammalogy 45(2), 246-250.
| Crossref | Google Scholar |
Smith RS, Sage LW (2006) Vegetation on and around granite rock outcrops in the Wellington National Park. Conservation Science Western Australia 5(3), 259-268.
| Google Scholar |
Soderquist TR, Nally RM (2000) The conservation value of mesic gullies in dry forest landscapes: mammal populations in the box–ironbark ecosystem of southern Australia. Biological Conservation 93(3), 281-291.
| Crossref | Google Scholar |
Spence-Bailey LM, Nimmo DG, Kelly LT, Bennett AF, Clarke MF (2010) Maximising trapping efficiency in reptile surveys: the role of seasonality, weather conditions and moon phase on capture success. Wildlife Research 37(2), 104-115.
| Crossref | Google Scholar |
Speziale KL, Ezcurra C (2015) Rock outcrops as potential biodiversity refugia under climate change in north Patagonia. Plant Ecology & Diversity 8(3), 353-361.
| Crossref | Google Scholar |
Storr GM (1967) Geographic races of the agamid lizard Amphibolurus caudicinctus. Journal and Proceedings of the Royal Society of Western Australia 50, 49-56.
| Google Scholar |
Storr GM (1978) Notes on the Ctenotus (Lacertilia, Scincidae) of Queensland. Records of the Western Australian Museum 6(3), 319-332.
| Google Scholar |
Sweet SS (1999) Spatial ecology of Varanus glauerti and V. glebopalma in northern Australia. Mertensiella 11, 317-366.
| Google Scholar |
Tapper S-L, Byrne M, Yates CJ, Keppel G, Hopper SD, Van Niel K, Schut AGT, Mucina L, Wardell-Johnson GW (2014a) Prolonged isolation and persistence of a common endemic on granite outcrops in both mesic and semi-arid environments in south-western Australia. Journal of Biogeography 41(11), 2032-2044.
| Crossref | Google Scholar |
Tapper S-L, Byrne M, Yates CJ, Keppel G, Hopper SD, Van Niel K, Schut AGT, Mucina L, Wardell-Johnson GW (2014b) Isolated with persistence or dynamically connected? Genetic patterns in a common granite outcrop endemic. Diversity and Distributions 20(9), 987-1001.
| Crossref | Google Scholar |
Telfer WR, Bowman DMJS (2006) Diet of four rock-dwelling macropods in the Australian monsoon tropics. Austral Ecology 31(7), 817-827.
| Crossref | Google Scholar |
Telfer WR, Griffiths AD, Bowman DMJS (2008) The habitat requirements of four sympatric rock-dwelling macropods of the Australian monsoon tropics. Austral Ecology 33(8), 1033-1044.
| Crossref | Google Scholar |
Thomas H, Cameron SF, Campbell HA, Micheli-Campbell MA, Kirke EC, Wheatley R, Wilson RS (2021) Rocky escarpment versus savanna woodlands: comparing diet and body condition as indicators of habitat quality for the endangered northern quoll (Dasyurus hallucatus). Wildlife Research 48(5), 434-443.
| Crossref | Google Scholar |
Thompson GG, Thompson SA, Finlayson GR (2010) Spatial and temporal variations in the trapped terrestrial vertebrate fauna of the Hamersley Range, Western Australia. Journal of the Royal Society of Western Australia 93(2), 51-64.
| Google Scholar |
Twigg LE, How RA, Hatherly RL, Dell J (1996) Comparison of the diet of three sympatric species of Ctenotus skinks. Journal of Herpetology 30(4), 561-566.
| Crossref | Google Scholar |
Watts CHS (1977) The foods eaten by some Australian rodents (Muridae). Australian Wildlife Research 4(2), 151-157.
| Crossref | Google Scholar |
Wearn OR, Glover-Kapfer P (2019) Snap happy: camera traps are an effective sampling tool when compared with alternative methods. Royal Society Open Science 6, 181748.
| Crossref | Google Scholar | PubMed |
Woinarski JCZ, Braithwaite RW, Menkhorst KA, Griffin S, Fishe R, Preece N (1992) Gradient analysis of the distribution of mammals in Stage III of Kakadu National Park, with a review of the distribution patterns of mammals across north-western Australia. Wildlife Research 19(3), 233-262.
| Crossref | Google Scholar |
Woolley L-A, Geyle HM, Murphy BP, Legge SM, Palmer R, Dickman CR, Augusteyn J, Comer S, Doherty TS, Eager C, Edwards G, Harley DKP, Leiper I, McDonald PJ, McGregor HW, Moseby KE, Myers C, Read JL, Riley J, Stokeld D, Turpin JM, Woinarski JCZ (2019) Introduced cats Felis catus eating a continental fauna: inventory and traits of Australian mammal species killed. Mammal Review 49(4), 354-368.
| Crossref | Google Scholar |