Fire and geodiversity
Ruby O. Hoyland


A
B Present address:
Abstract
Geodiversity elements contribute significantly to local and global hydrological, biogeochemical and ecosystem services and as such, fire is a potentially disruptive force with long-term implications. from limiting karstic speleothems formation, to compounding impacts of peat-fire-erosion cycles. Geodiversity elements additionally possess important cultural, aesthetic, and environmental values, including the support of ecosystem services. Hence, assessments of potential fire damage should consider implications for land users, society, and culture, alongside the geomorphic impacts on geodiversity elements. With a view to providing a concise set of descriptors of the response of geodiversity elements to fire, we qualify and in places, quantify, how fire may degrade geosystem function. Where possible, we highlight the influence of fire intensity and frequency gradients, and cumulative fire, in the deterioration of geodiversity values. Geoconservation is integral to protected areas with implications from fire effected geodiversity functions and values presenting issues for management, with potential consequences extending through to delisting, degazetting, and resizing of protected areas. Future research in reserve systems should concentrate on understanding the synergistic and compounding effects of fire on the geophysical landscape.
Keywords: deposition, fire management, fluvial, geoheritage, geosystem services, karst, landform, post-fire impacts, soil, values.
Introduction
The concept of geodiversity is expansive (Boothroyd and McHenry 2019). It includes the natural range (diversity) of geological (rocks, minerals, fossils), geomorphological (landform, topography, physical processes), soil, and hydrological features including their assemblages, structures, systems, and role in landscapes (Gray et al. 2013). Geodiversity occurs at all scales, from the elemental to the global (Bétard and Peulvast 2019). Geodiversity is thus inclusive of the elements (i.e. tangible examples of geodiversity such as rocks and landforms), of ecosystem and geosystem functions, and their associated values (Fig. 1). Negative impacts upon geodiversity can have profound consequences, even at very localised scales of operation (Gray 2019). Potentially degrading processes and threats to geodiversity elements have only recently been envisaged as a collective (but see Kiernan 1996; Dixon et al. 1997; Shakesby and Doerr 2006). Now with rapidly accelerating climate change and the expansion of wildfire, it is timely to conduct a global synthesis and quantification of the potential impacts of fire on geodiversity.
Visages of geodiversity and geoheritage. (a) Rocks such as granodiorite of the Tasmanian Bay of Fires, (b) minerals such as crocite from the Dundas region of Tasmania, (c) fossil casts and rhizoliths such as the rhizoliths of Augusta, Western Australia, (d) soils, (e) landforms such as Uluru from the Northern Territory, and (f) their associated processes, such as the aeolian weathering of limestone in the Pinnacles, Western Australia. Image credit: Ruby Hoyland and Melinda McHenry.
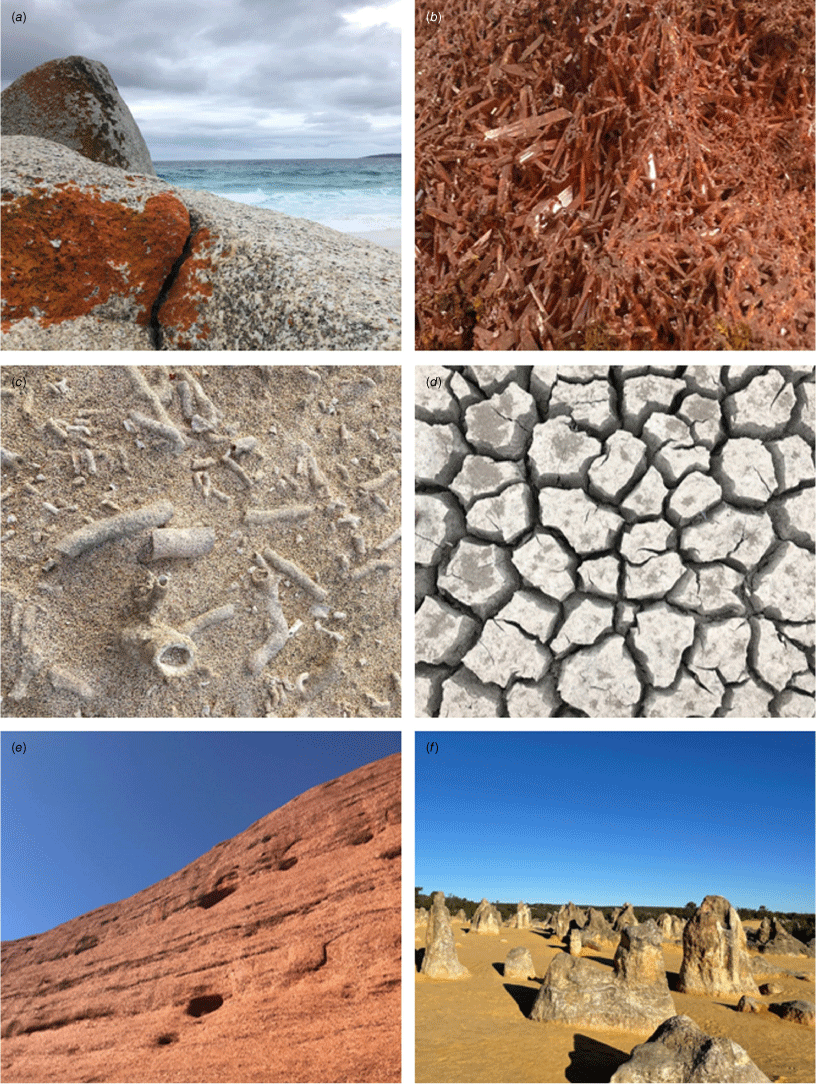
Fire is a geomorphic agent, whose impacts span from the local scale over hourly to annual interval to the global processes occurring over decades to millennia (Whitlock et al. 2010; Linley et al. 2022). Predicting fire behaviour and ecosystem-level response is complicated, with physical variables coalescing to influence ignition probability, fire severity and rate-of-spread (Moritz et al. 2010; Whitlock et al. 2010). The subsequent response of elements and values to fire is non-linear, and depending on severity and extent, can have cumulative and disruptive impacts on ecosystem processes and services. Fire intensity and fire heterogeneity influence the distribution and severity of impacts on geodiversity elements. Fire can modify element size, shape, appearance, function, capacity to support or regulate other ecosystem services and provisions.
Knowledge of the potential degradation and threats to geodiversity elements and values is important for protected areas governance and management, land and water restoration and for the benefit of society. Fire is both a function and consequence of geodiversity whereby elements and associated processes such as topography, soil moisture and structural landscape features influence fire intensity, severity, and extent. Additionally, fire is a perturbation that could potentially degrade geosystem processes such as volatilising soil carbon reservoirs, metamorphosing rocks and altering drainage patterns (González-Pérez et al. 2004).
Geological elements encompass the lithological landscape features that may be in the form of bedrock, outcrops, cliffs and exposures. Geological elements, as a source of soil parent material and species habitat, are the foundation upon which many significant natural values are supported. They facilitate hydrological and biochemical processes, and are highly valuable sources of scientific knowledge. Palaeontological features, for instance, contribute to the scientific value of a lithological geodiversity element via relative landscape or feature dating and estimates of landscape evolution. Meanwhile bedrock, outcrops or exposures can possess scenic, spiritual or natural values associated with representativeness, form, function and integrity.
Some geodiversity elements and values are recognised as ecosystem services (ES) in that they offer the monetary and functional value to living ecosystem components, and thus the broader environment and humans. Ecosystem services are defined as the goods and services derived from the (natural) environment, though have been criticised since their conceptualisation for being highly biocentric (Gray 2019). Ecosystem services are divided into four categories: (1) provisioning; (2) supporting; (3) regulating; and (4) cultural services.
Current ES frameworks omit key functions and processes derived from the abiotic environment; hence, there has been recent critiques of biocentrism (Gray 2019) and the disconnection between the contributions of biosphere and geosphere (van Ree and van Beukering 2016; van Ree et al. 2017). As a result, an emerging framework of geosystem services (GS), the abiotic counterpart to ES or the ‘goods and services derived from non-living ecosystem components’ has arisen to promote those aspects. Regardless of interpretation, it is evident that damage to elements or values by fire could also affect geosystem and ecosystem service provision.
The act of conserving or recognising the value of geodiversity, and geoscientific values and phenomena as geoheritage is referred to as ‘geoconservation’ (Gordon 2019). Representation and valuation of geodiversity elements in natural systems and protected areas is chronically underfunded and poorly articulated (but see Crofts et al. 2021). To date, most geoconservation priorities have been considered at the regional scale and have paid attention predominantly to geomorphological and morphometric parameters and patterns within a landscape (Bétard and Peulvast 2019), yet wildfire operates at increasingly larger scales (Moritz et al. 2010; Pezzatti et al. 2013; Higuera 2015).
Geoconservation is fundamental to all protected areas management and should be explicitly accounted for in management planning (Crofts 2018; Crofts et al. 2020; Gordon et al. 2019). Successful management should therefore include tools for fire managers to make decisions on when best to intervene in wildfires, including pre-emptive and operational management plans (Semeniuk and Semeniuk 2005). In many places, fire risk management already considers the exposure and sensitivity of common or charismatic elements (e.g. peatland soils, rock monuments) to fire. Unfortunately, likelihood and consequence assessment of fire as a key threatening process to geodiversity elements and values is presently constrained, notably by a lack of coherent statements and data that could be used to inform threshold risk modelling. Without this information, we lack collective insight into the potential cumulative impacts of wildfire in a time where inter-fire frequency, intensity and fire range has increase (Linley et al. 2022), and there has been significant refinement and expanded application of the concept of geodiversity. While fire impacts to geological elements and geomorphological processes have been largely conceptualised by Shakesby and Doerr (2006), in light of the need to understand value implications and consequences for protected areas, we present here not only the physical changes and functional disruption at the localised scale, but also where additional values such as the educational, scientific, and touristic uses of geodiversity elements, are altered and diminished by fire occurrence. A section is given to expanding this understanding on a larger scale through describing impacts which accumulate through the geo- and ecosystem.
Impacts of fire on geodiversity
Fire-induced changes affect all components of geodiversity, including the elements and the relationships between them and their associated values (Table 1). Human activities increasingly play a crucial role in shaping wildfire. From 1979 to 2013, fire-weather seasons lengthened by an average of 18.7%, though this inadequately captures the risk of economically and ecologically destructive fire events. Historical records from the western USA spanning the past 3000 years reveal that fire activity was initially driven by temperature and drought but shifted towards anthropogenic influence during the 19th century as human population increased and indigenous fire practices diminished (Bowman et al. 2020). Human variables, including ignition and suppression, are identified as significant predictors of fires, especially in the wildland–urban interface, where human-induced disturbances exceed historic variability, emphasising the critical role of human actions in fire regime changes (Hawbaker et al. 2013; Jones et al. 2022).
Geodiversity element | Functional attribute | Worst-case, direct impacts of fire | |||||
---|---|---|---|---|---|---|---|
Functional value | Cultural and aesthetic value | Economic value | Scientific and educational | Intrinsic value | |||
Exposed rock | Structure | Fracturing, weathering, and altered mineral aggregates decrease structural support (Shakesby and Doerr 2006; Storey 2010; Natural Values Conservation Branch 2017; Buckman et al. 2021). | Indigenous rock art and cultural signifiers damaged or destroyed (Pearson 2015; Allam 2020). | Decline in geotourism. | Degraded fossil deposits, speleothems, and slowly developed glacial and periglacial features (Natural Values Conservation Branch 2017). | Existence of geoheritage, landscape features, topography, and their integrity; altered state of existence (Gray 2005). | |
Vertical fracturing, ash deposits, fire-blackened surfaces, spalling impact: disruption of historical and folklore meaning; impact on artistic inspiration, sense of place, spiritual connection (Gray 2005; Shtober-Zisu et al. 2018). | Loss of construction materials and minerals. | Implications for cosmogenic isotope dating. | |||||
Soil | Structure | Altered aggregate stability and increased bulk density (Ice et al. 2004). | Reduced access to sites. | Reduced infrastructural support and reduced site access. | Reduced access to sites or site closure (Hilger and Englin 2009). | Existence of geoheritage, landscape features, topography, and their integrity; altered state of existence (Gray 2005). | |
Increased exposure to mechanical erosion processes (Ice et al. 2004). | Changes in colour and texture (Verma and Jayakumar 2012). | Modification of trails and tourism related infrastructure, including the economic value of experiences (Hilger and Englin 2009). | |||||
Increased susceptibility to slope failure (Li et al. 2021). | |||||||
Hydrology and watershed processes | Desiccation (Kennard and Gholz 2001). | Altered aesthetic value. | Nutrient volitisation reduces ecosystem service support (Tulau et al. 2019). | Disruption of system and properties. | |||
Reduced infiltration (Ice et al. 2004; Moody et al. 2016). | Altered cultural understandings of sites. | ||||||
Hydrophobicity (Certini 2005). | |||||||
Overland flow and rill formation (Shakesby and Doerr 2006). | |||||||
Mineralogy | Altered mineral assemblages, recrystallisation of Fe and Al oxide (Certini 2005; Shakesby and Doerr 2006). | Damage to sites of significance. | Reduced natural capital. | Reduction in undisturbed mineral samples. | |||
Reduced visual appeal. | |||||||
Geochemistry | Decreased total N and increased nitrification (Kutiel and Inbar 1993). | Damage to sites of significance. | Reduced suitability and sustainability of agriculture, viticulture, horticulture (Caon et al. 2014). | Reduced capacity for studies of undisturbed soils. | |||
Reduced rate of mineralisation and increased pH (Certini 2005). | Decline in land value. | Disruption or destruction of paleosols. | |||||
Organic matter | Transformation and reduction of organic matter, substrates, and residues (DeBano 1990; Certini 2005; Shakesby and Doerr 2006). | Altered landscape value and aesthetic (Caon et al. 2014). | Carbon storage and sequestration (Semeniuk and Semeniuk 2005; Natural Values Conservation Branch 2017; Mayer et al. 2020; Carroll et al. 2023). | Loss opportunity for studies of complex soil interactions. | |||
Altered biological assemblages (Shakesby and Doerr 2006). | Loss of biological assemblages and altered ecological succession (Marafa and Chau 1999; Williams-Jara et al. 2022). | Reduced primary productivity. | |||||
Cryosphere | Permafrost decline | Melting and sublimation; increased albedo accelerating melt rate. | Loss of permafrost environments and biome. | Altered carbon storage capacity. | Loss of biome. | Existence of geoheritage, landscape, features, topography, and their integrity, altered state of existence (Gray 2005). | |
Thaw slumping (Loranty et al. 2021). | Reduced access to sites. | Loss of/reduced access to climate proxy. | |||||
Glacier formations | Increased albedo; melt and sublimation (Molina et al. 2015; Aubry-Wake et al. 2022) | Altered aesthetics (black carbon accumulation; ash deposition; reduced size) (Kang et al. 2020). | Reduced touristic appeal. | Loss of climate proxy; loss of landscape feature. | |||
Reduction in climate regulation capacity. | Loss of landscape features (e.g. pingos, thermokarst, etc.) (Loranty et al. 2021). | ||||||
Depositional forms | Dune structure and transport mechanisms | Activation of dunes (Shumack et al. 2017; Fisher and Hesse 2019). | Alteration of cultural landscapes. | Reduced capacity to support ecosystem services in their current form. | Interference of long undisturbed sites. | Existence of geoheritage, landscape features, topography, and their integrity; altered state of existence (Gray 2005). | |
Increased wind erosion potential. | Decreased aesthetic appeal. | ||||||
Glacial deposits | Spalling, fracturing. | ||||||
Increased weathering and erosion potential (Shtober-Zisu et al. 2018). | |||||||
Fluvial landforms | Fluvial processes and structure | Increased debris torrents. | Decreased aesthetic and visitation appeal. | Altered capacity to support ecosystem services (Blackwood et al. 2021). | Destruction of channel and system properties (Ward et al. 2022). | Existence of geoheritage, landscape features, topography, and their integrity; altered state of existence (Gray 2005). | |
Increased channel erosion (Miller et al. 2003). | Altered sense of place. | Reduced irrigation potential. | |||||
Altered pool complexes. | Reduced landscape significance. | Sedimentation (Ward et al. 2022). | |||||
Modified sediment regimes (Warrick et al. 2012). | Increased likelihood of post-fire flood impacts (Jong-Levinger et al. 2022). | Decline in waterway capability. | |||||
Channel widening (Ielpi and Lapôtre 2023). | |||||||
Karst and Epikarst | Drip water geochemistry | Increased occurrence and variability of some elements and isotopes (Holland 1994; Nagra et al. 2016). | Altered cultural narratives of speleological landforms. | Reduced access to and safety in karsts means results in decreased suitability for visitation. | Incorrect speleothem interpretation for proxy records due (McDonough et al. 2022). | Existence of geoheritage, landscape features, topography, and their integrity; altered state of existence (Gray 2005). | |
Increase in some metal concentrations (Coleborn et al. 2018). | Discoloured channel walls and water lead to decreased aesthetic appeal (Holland 1994). | Decreased tourism potential from reduced cave feature visibility. | Reduced access to and safety within karsts. | ||||
Hydrology | Increased absorption of water into the system (Holland 1994; Coleborn et al. 2018). | ||||||
Depositional regime | Increased sedimentation and increased sediment supply from fire-induced spalling (Holland 1994; Storey 2010). |
Complicating matters is that anthropogenically-induced climate change adds great uncertainty to the identification of future fire behaviour and likely deems the application of previous fire histories as proxies for ‘acceptable’ fire regime tolerances, inappropriate (Gavin et al. 2007). Global climate models suggest fire severity and frequency will increase; however, at a regional and local scale, the implications of climate change may vary greatly, thus hindering the ability to generate appropriately scaled fire management planning strategies (Gordon et al. 2022a, 2022b). The emergence of a new dominant fire type, known as ‘megafires’, is already presenting formidable challenges for ecosystems, communities, and fire management agencies. Defined as wildfires exceeding 100,000 acres (405 km2) in size, megafires have become increasingly prevalent globally in the past two decades, with countries including Australia, Chile, Portugal, Russia, and California all having experienced megafires in the past 5 years (Khorshidi et al. 2020; Pliscoff et al. 2020; Collins et al. 2021; Varga et al. 2022; Ramos et al. 2023). The scale of megafire events in both area and intensity result in profound alterations to ecosystems including disruption to ecological and hydrogeological processes on an unprecedented scale; for example, the 2019/2020 Australian megafires extended to regions not anticipated to experience burns including World Heritage listed Gondwanan Rainforest (Ward et al. 2020). Future climate projections suggest that rising global temperatures, altered precipitation patterns, and increased frequency of extreme weather events will contribute to a heightened susceptibility of landscapes to megafire events. Coupled with expected prolonged droughts and extended fire seasons, conditions are becoming more conducive to the ignition and rapid spread of large-scale wildfires (Deb et al. 2020), albeit that the impacts of fire can be experienced at large and small spatial and temporal scales (Fig. 2).
Spatial and temporal impacts of fire on geodiversity elements. Fire impacts geodiversity elements and values in several ways. Each point on the above graph indicates an impact, with its location relative to the spatial and temporal range where it occurs. Note to reviewers, this is an interactive image, please download the file via this link and open to hover over diamonds: https://rpubs.com/RHoyland/1166910.
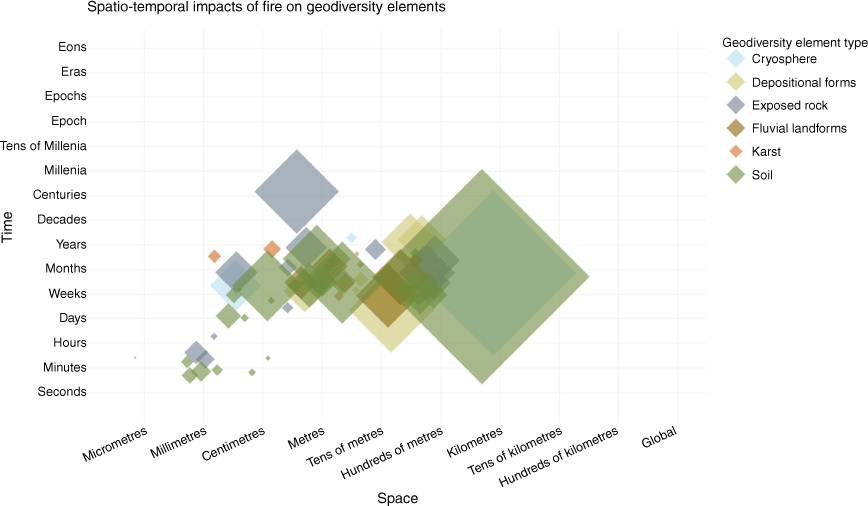
Rock
The structural integrity of geological elements can be compromised by fire through mechanical alteration to the mineralogical structure. Strong temperature changes create micro-fractures within individual minerals and throughout rock margins (Anderson 2019). Rapid, uneven heating and thermal expansion coupled with rapid endolithic dehydration may also result in fracturing and fissures throughout the outer rock and inwards, while accelerated thermal diffusion by intense heating increases rate of gas release from rock. These processes, coupled with climatic conditions that are conducive to rapid cooling following fire, induce spalling of the outer surface (Shakesby and Doerr 2006). As spalling is the primary result of weathering on rock, fire will accelerate the physical exfoliation that results in lensoid-shaped fragments up to several centimetres thick and become detached from the rock surface (Fig. 3).
Photographs of exposed lithological elements approximately 1 month after prescribed burns in Tasmania. (a) Granodiorite boulder with fire-induced spalled section; insert shows spalled fragment found below boulder. (b) Granodiorite boulder with surface cracking parallel to boulder surface. (c) Surface charring of granodiorite rock compared to natural state (0–4 cm) that was protected from fire by soil. (d) Siltstone rock with surface char and cracking, with small, spalled section. (e) Fossil specimen in siltstone charred by fire. (f) Charred and crumbling conglomerate rock after fire impact. Image credit: Ruby Hoyland.
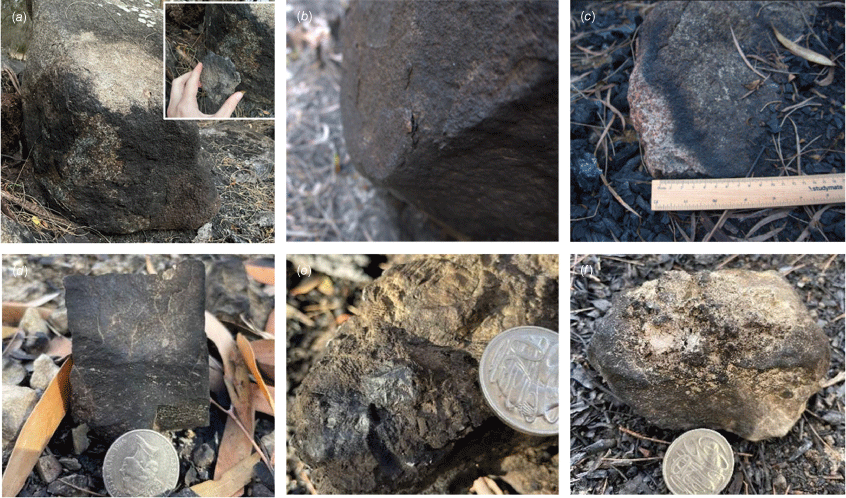
Spalling affects almost all rigid rock types with structural composition being a principal contributor to spalling potential (Shakesby and Doerr 2006; Buckman et al. 2021). Rocks with higher quartz composition are more likely to spall due to the expansive potential of quartz compared to other minerals, such as a 3.76% increase in volume when heated to 570°C from ambient temperature, which is twice as much as hornblende and four times greater than feldspar (Winkler 1975). Existing fractures and weathering rinds can induce further spalling through the alteration of surface expansion and contraction potential, such as in laminar structures of carbonate rocks (Cooper and Simmons 1977; Shtober-Zisu et al. 2015, 2018). Heterogeneous mineral compositions create variable thermal conductivity in rocks, which when coupled with sudden temperature differences from the initiation and/or the extinguishment of a fire, increase the likelihood of spalling (Zimmerman et al. 1994). Spalling of exposed lithological elements may be visible for upwards of 6 years, as spalled sections are often a lighter colour having undergone less weathering than non-spalled surfaces (Shtober-Zisu et al. 2018). These aesthetic consequences have further implications for touristic appeal; for example, a 2022 wildfire in the Bohemian Switzerland National Park in the Czech Republic resulted in diminished touristic interest due to altered visuals of the rock forms and landscape (Boháč and Drápela 2023). Spalled sections of rock may also act to accumulate ash post-fire for 2–3 decades, impacting aesthetics and ecohydrology (Shtober-Zisu et al. 2018).
Values assessment of fossil deposits, petroglyphs, and glacial pavements can be diminished when fire compromises the quality of features for cation-radio dating and reducing the volume of viable specimens for research and conservation purposes. Further, spalled rocks may compromise the safety of geotourism activities including rock climbing and trail walking, as partially spalled fragments create unsafe climbing surfaces and detached debris can increase the risk of rock falls (Boháč and Drápela 2023). Micro-fracturing of minerals at the surface and sub-surface of landforms, exposures and clasts can reduce the structural integrity of rocks and decrease resistance to other weathering and erosion agents and processes, accelerating the deterioration of natural and cultural values (Tratebas et al. 2004).
Soil
Fire has the potential to modify or destroy soil attributes and functions (Agbeshie et al. 2022). Fire-induced changes to soil chemistry include nutrient volatilisation and modified elemental concentrations and nutrient availability (Kutiel and Inbar 1993; Kennard and Gholz 2001; Tulau 2015). Many soil interactions are affected synchronously due to the combustion of soil organic matter (DeBano 1990). For instance, combustion of leaf litter and organic matter in topsoil volatilises hydrophobic organic compounds, which are transported vertically along strong temperature gradients (DeBano 2000b). These compounds condense at lower temperatures, coating soil particles, which then form hydrophobic soil layers (DeBano 1990; Neary et al. 1999; DeBano 2000a; Shakesby and Doerr 2006; Stavi et al. 2017; Stavi 2019). Water that would normally permeate through the soil is deterred by the hydrophobic layer, increasing propensity for surface runoff, rill formation, and erosion susceptibility (DeBano 1981).
Hydrophobicity afflicts sandy soils more than other soil textual classes due to the low surface area to volume ratio of sediments. Soil water repellence is initiated at temperatures between 175°C and 200°C, and the response is asymptotic whereby at temperatures above 280°C, hydrophobicity no longer occurs (DeBano 1981). The quantity of organic content present in and on soils is also influential in determining the likelihood and extent of hydrophobic soils, which along with minimised soil porosity and condensing processes, has subsequent impacts for soil viability following fires (DeBano 2000a; Ice et al. 2004; Olorunfemi et al. 2014). Fire-induced soil water repellency may reduce splash erosion by forming a surface crust on soils of 2.0–5.0 mm size fractions, but the potential for accelerated wind erosion on sandy soils has not yet been thoroughly quantified and may be of concern (Vermeire et al. 2005; Fox et al. 2007). Greater magnitudes of hydrophobicity and severe erosion tend to occur following high severity fires, while the removal of organic matter and biomass, and disruptions to nutrient and chemical cycling can occur at much lower temperature thresholds (Fox et al. 2007; Mataix-Solera et al. 2011; Vieira et al. 2015). Consequently, management burns can remove organic material and volatilise organic compounds, which may also cement soil particles together resulting in further altered porosity and structural stability. This can lead to slumping, increased runoff and erosion, and at large scales, an increase in flood potential (Fig. 4).
Fire-induced hydrophobicity impacts on Geosystem Services. Change (negative or positive) to geosystem service provision induced by fire and consequent soil hydrophobicity.
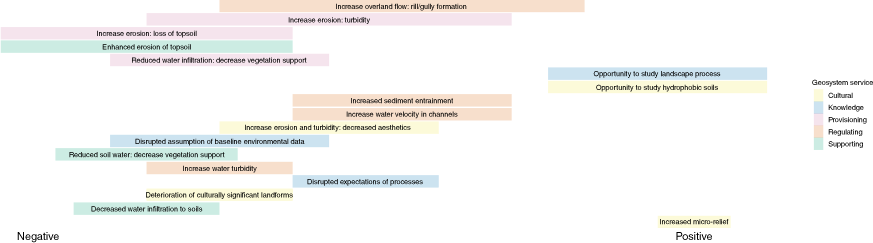
Organic nutrient losses from combustion of organic soil components of soils may occur as particulate matter and contribute to the composition of ash. The ratios of particulate and non-particulate nutrient losses in low-intensity fires in sub-alpine Eucalyptus forests were 57%:43% in fires that produced black ash, and 73%:27% in fires that produced fine grey ash (Boerner 2006). Particulate matter may be exported from the system via hydrological and aeolian transport processes or remain in situ post-fire depending on weather patterns, topography, and soil conditions (Boerner 2006). Studies observing nutrient transport post-fire noted the first precipitation event was highly significant in dissolving ash, with upwards of 90% of nutrients in organic particulate matter leached (Boerner 2006).
Whilst organic compounds containing nitrogen, phosphorus and sulfur volatilise at low temperature thresholds (200°C), inorganic compounds require higher temperatures (Boerner 2006). At temperature thresholds greater than 500°C, 11–17% of calcium, 9–46% of potassium, 13–17% of magnesium, and 10–46% of inorganic phosphorus were lost from various forest soils (Grier 1975; Harwood and Jackson 1975; Christensen 1977; Boerner 2006). Nitrogen volatilisation is highly dependent on the combustion of organic components, with an observed rate of 55 kg/ha of nitrogen from litter and wood, and 6 kg/ha of nitrogen from humus under prescribed burning scenarios in the USA (Hubbard et al. 2004). Exposure to high temperatures can also transform crystalline minerals, which regulate the sorption capacity of soils for phosphorus, resulting in a lower phosphorus content in soils and/or reduced bioavailability of soil phosphorus following fires. In the study of a prescribed moderate-high severity burn, a loss of approximately 7 kg/ha of phosphorus was observed in oligotrophic soil (Santín et al. 2018). The majority of the organic and bioavailable forms of phosphorus were removed from the soil, with implications for vegetation recovery and capacity in ecosystem support, as well as reduced resilience of soils to future stresses (Santín et al. 2018).
Short-term increases in post-fire soil microorganism biomass result from rapid growth of fire-resistant microorganism communities that utilise nutrients from dead microorganisms and labile carbon. Such occurrences are related to the quick recovery of soil respiration (1 month post-fire), yet denoted reduced biodiversity that persisted 2–6 months post-burn (Barreiro and Díaz-Raviña 2021). Long-term trends for microorganism recovery in soils post-fire events showed inconsistent results for biomass, nutrient cycling, and community diversity, with recorded recovery rates between 1 month and 50 years (Barreiro and Díaz-Raviña 2021).
The reduction or removal of soil biota can occur even at low to moderate temperatures (especially in soils rich in organic matter) causing immediate disruptions to the cyclic efficiency and structural integrity of these soils (Certini 2005; Shakesby and Doerr 2006). Microorganisms involved in the carbon cycle are highly sensitive to thermal changes and more likely to expire at lower temperatures compared to bacteria and fungi involved in nitrogen cycling (Barreiro and Díaz-Raviña 2021). Fires that heat soils in excess of 120°C result in the death of fungi and bacteria, whereas low temperature fires (<50°C) impede growth and activity, particularly of fungi, but may not necessarily result in broadscale microbial community extinction. Such changes have drastic impacts on bio-geo-hydrological interactions and can lead to the permanent loss of sensitive ecosystems include peat and wetlands (Semeniuk and Semeniuk 2005; Natural Values Conservation Branch 2017; Mayer et al. 2020). For example, an Alaskan fire in 2007 was estimated to have contributed to ~2 Tg of carbon, with 60% attributed to soil organic matter, an amount comparable with the average annual net sink for carbon in the Arctic tundra in the past 250 years (Mack et al. 2011 in Adams 2013). Such occurrences undermine both the economic value of these sites as carbon stores and as sequestration environments, as well as diminishing their intrinsic value (Belyea and Clymo 2001; Gao et al. 2022).
The burning of soils and organic materials can produce pyrogenic carbon. A positive change to soils, pyrogenic carbon has a longer mean residence time compared to other forms and may persist in the environment for multiple centuries under favourable conditions (Abney et al. 2017). Pyrogenic carbon is particularly important for peatlands as a relatively intractable form, and contributes to soil dynamics in other landscapes through carbon storage (Gao et al. 2022). Nevertheless, pyrogenic carbon is highly erodible due to properties of hydrophobicity and low-density (compared with other carbon forms), as well as being concentrated in the upper soil horizons, where it can be lost in post-fire erosive processes (Cotrufo et al. 2016). For instance, pyrogenic carbon was found in much higher concentrations in depositional landscapes versus erosional landscapes 1- year post-fire in California USA (160 and 84 g/kg, respectively) (Abney et al. 2017). The erosion and transport of pyrogenic carbon into locations with unfavourable conditions for preservation can result in shorter environmental persistence with implications for terrestrial carbon storage capacity.
Cumulative impacts of fires on soils include fire-induced erosion, which is largely attributed to and further exacerbated by the loss of protective vegetation cover which leaves soils exposed to mechanical erosion processes (frost-heave, wind, and water erosion) and thereafter frequently forms gullies and rills (Ice et al. 2004; Shakesby 2011). Rates of ongoing degradation are inversely correlated with regeneration of protective vegetation and re-regulation of hydrological processes within soil and at the soil surface. Coleborn et al. (2016) documented soil recovery via CO2 concentration and concluded it took 5–10 years post-fire event in an Australian southern temperate woodland for soil to recover, with rate of recovery largely dependent on vegetation revival. Similarly, fires that removed organic materials, volatilised essential nutrients, and destroyed seed stores had longer recovery time estimates due to exacerbated vegetation recovery time in the temperate Tasmanian wilderness woodlands forests and peatlands (Natural Values Conservation Branch 2017; Shumack et al. 2017).
Soil type and characteristics strongly influence their response to fire and subsequent recovery (Wall et al. 2012; Vacchiano et al. 2014). In the same fire, soils with higher soil moisture sustained lower overall in-fire temperatures than soils with low soil moisture prior to the fire (Busse et al. 2005). Based on the nature of their sensitivity to disturbances, soils with lower rates or prospects of regeneration following fire include calcareous/karstic soils and organic soils, based on their slow formation rate and high flammability, respectively (Liu et al. 2020). Recalcitrant soils with persisting low organic content (due to high severity fires causing slow vegetation regeneration or high rates of erosion) negatively impact microorganism recovery rates, with recorded effects on biomass lasting 5–10 years. In fact, in some scenarios the destruction was irreversible and soil recovery did not occur (Barreiro and Díaz-Raviña 2021).
Post-fire land management also alters the likelihood of cumulative degradative impacts and the recovery rate of soils. Practices that result in soil compaction or increased erosion further degrade soils, while practices including mulching have been shown to be beneficial in increasing microorganism biomass and reducing overland flow and soil erosion but have limitations in terms of which localities, and ecosystems, they are applicable in Pereira et al. (2018), Barreiro and Díaz-Raviña (2021). Land use practices that involve livestock have been related to an increased amount of mineral material dislodgment from soils and increased soil erodibility in recently burnt grasslands (Stavi 2019). Post-fire soil management needs to mitigate cumulative degradative impacts while enhancing soil recovery, highlighting the need for greater research in this sphere to inform context-specific strategies.
Fluvial systems
Existing hydrological regimes, geomorphic form and processes of catchments influence the type and severity of fire (Storey and Betts 2011). Fires create conditions favourable for erosion and transport of sediments to fluvial systems because post-fire soils are largely water-repellent or exhausted and therefore, post-fire rains runoff into fluvial channels in a higher volume and greater velocity (Shakesby and Doerr 2006). The removal of riparian vegetation through fire or management action increases the volume of precipitation contacting bare soil. For example, Pettit and Naiman (2007) note the non-linear relationship between fire in the riparian zone and flood events is characterised by changes to riparian vegetation and upstream infiltration regimes. The occurrence of concentrated water flow pathways was not modified by fire incidence, though the velocity of channels was observed to increase in burnt scenarios (Pierson et al. 2009). Pierson et al. (2009) also found that in burnt landscapes runoff occurred sooner and with an increased average runoff rate of 40% when compared to unburnt plots under simulated rainfall conditions. A separate study found that fire-accelerated erosion rates contributed 8 and 6%, respectively, to long-term sediments yields in two lakes in the north-west USA (Swanson 1981). Similarly, in a simulated example, the compound impacts of wildfire and rainfall was shown to increase peak flows by up to six times when compared with events where wildfire was not present (Bowman and Williamson 2021).
Modifications to the volume of water and sediments and their velocity can induce morphological changes in fluvial systems. Channel widening increased 130% following fire events in North America (Ielpi and Lapôtre 2023). Increased sediment yield also has implications for bedloads of streams and rivers. For example, the varve thickness of lake sediments increased in the 15 years following fire events by 35% and 25% where fire recurrence was 60 years and 80 years, respectively (Swanson 1981). Further, stream-bound logs and woody debris influence downstream flow by collecting and depositing sediments and partially or fully restricting flow at multiple stream points (Praskievicz and Sigdel 2021). At the watershed-scale, this can impact the redistribution of sediments and depositional landforms, particularly where hydro-geomorphological processes have been altered, albeit there can be micro-topographical variance on finer scales where impacts to localised dependencies are equally important to understanding and accounting for shifts in fluvial environments. Furthermore, destabilisation of the riparian zone following fire events can be exacerbated by the resulting changes to stream flow processes, as stream channel affects velocity and therefore the scour potential of water (Swanson 1981).
Wildfires produce ash and sediment that can transport undesired elements and compounds to the fluvial environment. The accumulation of post-fire metal contaminants has severe ecotoxicological impacts on pelagic and benthic aquatic species in fluvial systems, contributing to mutations, mortality, and bioaccumulation. For instance, water and sediment samples from a burnt region of a river and a stream contained zinc, nickel, and copper concentrations that were seven-times, eight-times, and 1.5-times greater, respectively, than those taken outside the burnt zone. Water samples from the burnt river region also had a higher total suspended solids count with a higher percentage of fine particles and a greater organic content load compared to the unburnt river region (Ré et al. 2021). Conversely, fires can sometimes increase the availability of inorganic nutrients in the environment. For example, sub-alpine lake sediments revealing a relationship between high severity fire events and increased levels of rock-derived nutrients over a 6200-year period (Leys et al. 2016). Such changes in nutrients may have implications for the succession of biotic communities through potentially favouring certain species.
Cryosphere
The cryosphere encompasses the frozen aspects of geodiversity, including glaciers, ice caps and sheets, permafrost, and sea ice, and is critical in regulating global climate patterns and ecosystems. The cryosphere is directly impacted by fires producing black carbon, which results from the imperfect combustion of organic matter (Kang et al. 2020). Black carbon reduces albedo of surfaces when it accumulates, accelerating thawing and sublimation as the higher solar radiation absorption is converted to heat (Molina et al. 2015; Kang et al. 2020; Aubry-Wake et al. 2022). For instance, on the Tibetan Plateau, black carbon concentrations in snow and ice were attributed to a 20% decrease in albedo during the melt season (Kang et al. 2020). Additionally, black carbon is insoluble in water and accumulates on the surface of snow and ice as it melts or sublimates, compounding the effect.
Fires in the Arctic are particularly impactful as fires have the capacity to melt permafrost and generate thermokarst, which increase near-surface temperatures by up to 10°C through altering the localised albedo (Loranty et al. 2021). Jones et al. (2015) followed the progression of thermokarst formation in the 7 years following a burn in the Arctic, revealing both retrogressive thaw slumps and active layer detachment slides that occurred because of the fire, causing a 340% increase in microtopography. Similarly, Loranty et al. (2021) found a reduction in shade provided by vegetation in a Siberian forest fire in low and high density stands. There was a correlation between low-density tree cover and warmer soil temperatures, which could exacerbate future permafrost thawing. Permafrost thawing may also be coupled with slope failure as structural changes occur, further exposing features to increased erodibility (Li et al. 2021). Implications of these consequences extend to diminished opportunities for cultural connection through leisure activities and tourism due to reduced accessibility of sites.
Depositional landscapes
Post-fire erosion can occur within systems comprising all inorganic sediment types including sands, talus and alluvium. Frequency and intensity of changes to these systems following fire are influenced by climatic and geomorphological factors that determine the type and extent of sediment and debris mobilisation. The removal of protective vegetation increases the shear stress of wind on the boundary layer of deposits in aeolian environments, resulting in decreased surface stability and therefore increased weathering and erosion potential (Roehner et al. 2020). A study observing dune crest activity in the Simpson Desert, Australia identified dune activation only occurring at sites that had experienced a burn and where non-photosynthetic vegetation cover remained below 16% for 4 years (Fisher and Hesse 2019). Depending on land use, dune activation can be a negative or positive outcome for land management (e.g. foundation stability) and geoconservation (e.g. ‘process in action’).
Though direct impacts of fire on dune system stability are not yet comprehensively documented, it is likely that the cumulative effects of gravity-driven or water-driven erosion may impact larger debris that is exposed or deconsolidated following fire. The availability of colluvium and weathered material and the frequency and magnitude of post-fire rainfall events are important controls on the likelihood of significant mass-movement events such as dry ravel and rock falls (Swanson 1981; Florsheim et al. 2016). Destabilisation of dunes has implications for the redistribution of fine through coarse sediments in arid environments with consequences for the aesthetic and cultural values associated with landscape change, and opportunistic desert plant nutrition.
Karsts
Despite the relative protection of karsts resulting from their largely subterranean positioning, karstic geodiversity elements can still be affected by fire through surface changes. Modifications to epikarst biogeochemistry and hydrology are often immediately associated with changes to soils but also by means of altered components of input channels and areas of karst-surface interface. Epikarst soil conditions have a strong influence on hydrology and subsequently drive dripwater geochemistry, speleothem formation and karst morphology (Bian et al. 2019). The primary control on speleothem formation is soil CO2 concentrations that drive the rate of CaCO3 dissolution downward through the vadose zone. Abundance of CaCO3-bearing compounds within established soils is relative to the level of biochemical dissolution and subsequent transport of products via leaching. Speleothem growth is therefore strongly influenced by this dissolution and transport regime, which is disrupted by fire through reduction or complete elimination of soil biomass high in the soil profile resulting from soil desiccation (Bian et al. 2019) (Fig. 5). In the 2 months following a low-intensity prescribed burn, isotopic composition of dripwater was observed to be altered due to soil water evaporation, with elements including boron, silicon, and lead increasing in concentration (Coleborn et al. 2018).
Impacts of fire on karst systems and processes. (a) Before a fire, Water seeping through soil and epikarst delivers chemical constituents that deposit at the base of speleothems, contributing to their growth. (b) Following a burn, altered dripwater geochemistry from impacted soils and lack of vegetation result in changed colour and growth rate of speleothems. Schematic credit: Ruby Hoyland.
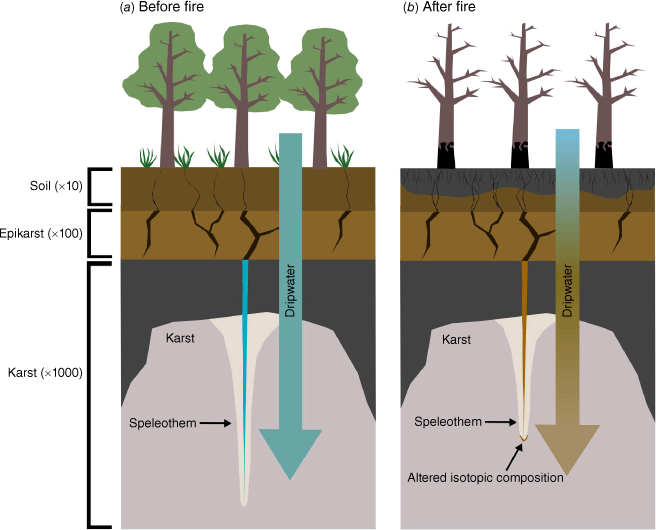
Cumulative impacts of fires are evident even in karstic environments. For example, within 15 months of a fire event, the accumulation of charcoal and ash, and the presence of soot stains in caves reduced the aesthetic appeal of karst tourism (Heap 1999). The safety and accessibility of caves was reduced by altered structural stability and increased risk of inundation following fire events, further contributing to the reduction of site economic potential. Further, coal and hydrogen stores in karst systems may be combusted during fire events, eliminating their viability as future economic and fuel sources, as well as diminishing opportunity to view such features in situ for educational and scientific purposes.
Karst depth has a significant influence on impact intensity with shallower caves more affected than deeper ones (Coleborn et al. 2018). Though fire damage in the pedosphere may be evident immediately, the cumulative impacts of modified nutrient and water chemistry and availability may not be evident for some time given cave depth. Much of the resultant impact on karst geodiversity integrity and value is therefore deferred and predicting such effects requires knowledge of the variable sensitivity of elements and their exposure to change. Additional factors to consider in the management of karst post-fire include weather conditions, which shape the intensity and longevity of impacts, and changes to the vegetation assemblage overlying karst, that can alter the hydrology by increasing infiltration over a relatively short time (McDonough et al. 2022).
Implications for ecosystem and geosystem services
The preservation of ES is a key function of protected areas, with the safeguarding of system-wide functions and processes of relevance for sustainable land management and in ensuring the provision of future avenues for landscape-scale evolution (Gordon and Barron 2012; Stolton et al. 2015). Values and processes associated with geodiversity underpin ES (Gray 2005). Thus, impacts from fire on geodiversity values have cascading impacts on the functionality of ecosystems (Fig. 6). Importantly, there are also services provided by geodiversity elements that are not directly valued because of their more charismatic and emblemic biotic dependencies. Examples include culturally- and touristically-significant unique landforms, stratigraphic units that provide geological history and landform knowledge, and the occurrence of fossil-bearing stone building materials (Gray 2011).
Cumulative impact of fire on geosystem and ecosystem services. (a) Five geosystem services (as compared with four ecosystem services, not shown) more accurately describe the value of geodiversity elements to humans and the environment. Geosystem services are the goods and services provided by geodiversity elements and include the categories of regulating, supporting, provisioning, cultural and knowledge services (adapted from Gray 2011). (b) The influence of fire on a specific service provided by the ecosystem and/or geosystem has consequences for other services, resulting in a cascade of indirect effects on the provision of ecosystem and geosystem services.
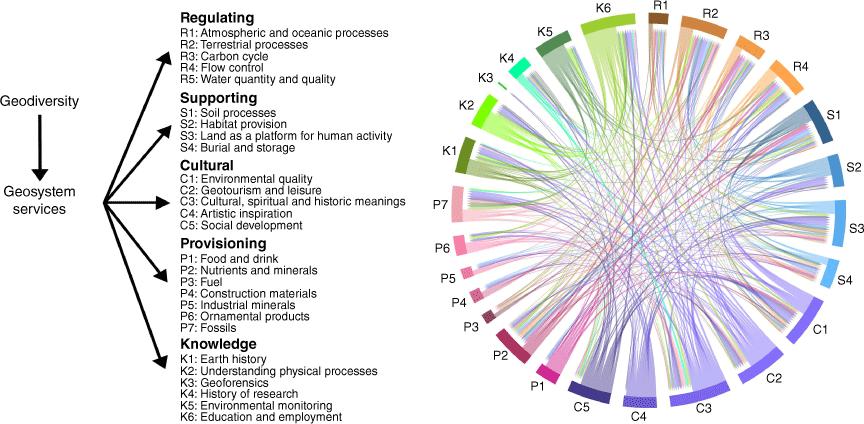
Fire events that degrade or diminish geodiversity elements with such values can directly and indirectly reduce the capacity for geosystem services provision. As the synthesis of even basic direct worst-case effects of fire on geodiversity elements is complex, it is too difficult to provide specifics for all possible fire trajectories on these elements. Nonetheless, geosystem services are indeed important to the ecosystem and therefore, it should be considered a priority to understand how these affect service provision to humans and the environment.
It should be noted that there is current debate surrounding the delineation of living and non-living ecosystem components, including confusion over whether water and ice should be accounted for in ES or GS. There is also deliberation in which framework certain biotic-abiotic interactions, such as soils with their high biodiversity but (often) abiotic parent-material, should be included. The divide between ES and GS is often given a spatial limit at the pedosphere, the layer of soil where organic activity begins to drastically decrease towards the lithosphere. The pedosphere is largely regarded as a combination zone where services, particularly ‘supporting’ services, are highly interrelated between the biotic and abiotic components of both systems. van Ree and van Beukering (2016) include organisms important for functional subsurface processes, such as stygofauna and bacteria, in GS. Hereafter, we use the geosystem services model to describe landscape-scale effects of fire on geodiversity as it is more inclusive of subterranean and pedospheric processes.
Regulating services are provided by processes in the ecosystem and geosystem that maintain hydrological, geological and geomorphic cycles (Gray 2011). Fires affect regulating services, for example, via reduced soil moisture and soil cover following fires. This reduction has been linked to enhanced dust emissions, which influence atmospheric processes including cloud formation, as dust particles serve as cloud condensing nuclei. Atmospheric dust also influences the global radiation budget through altering the absorption and scattering of light entering the atmosphere and has consequences for human health due to reduced air quality and the transmission of combustion residuals (Yu and Ginoux 2022).
Terrestrial processes like the rock cycle are also influenced by the occurrence of fire, as spalling creates sediment and increases the surface area of rock faces to future weathering potential. This allows the cycle of rock breakdown to (sedimentary) rock formation to occur. Further, altered overland flow mechanics also related to fire events (like hydrophobicity) enhance erosion susceptibility, allowing increased sediment movement that carries the nutrients derived from terrestrial areas to fluvial and aquatic systems. This alters the concentrations of organic and inorganic nutrient levels in these ecosystems, thereby affecting nutrient cycles in source and depositional areas (Miller et al. 2003; Leys et al. 2016; Natural Values Conservation Branch 2017).
Geomorphological processes are similarly impacted by fire events as the reduction in the stability of riverbanks and altered stream channels impede flow control, including reduced capacity for flood mitigation. For example, Seibert et al. (2010) found a mean post-fire increase in peak flows of 120% with both simulated and observed runoff changes persisting for half a decade following the wildfire compared to pre-fire parameters. Similarly, Mahat et al. (2016) observed peak stream flow variability was dramatically altered by post-fire events, with higher mean annual water yield up to twice as high in burnt catchments compared to those left unburned. Other natural hazards may be indirectly initiated by fire events, including debris flows; hence reduced infiltration resulting from fire can increase the likelihood of such events (McGuire and Youberg 2019). The results of these changes to flow regimes include higher propensity for flash flooding, lower runoff tolerance thresholds and ongoing changes to stream sediment budget (Storey and Betts 2011; Moody et al. 2016).
Furthermore, the regulation of water quality by the geosystem involves natural physical filtration. This service may be diminished by thermally induced fracturing of rock materials that increases porosity and thereby decreases capacity for impurities from water to be trapped in porous spaces of rocks (Brotóns et al. 2013). Additionally, the cascading impact of increased turbidity of waterways due to reduced aggregate stability and increased sediment movement following fire events consequently reduces the capacity to obtain freshwater from the ecosystem (White et al. 2006; Warrick et al. 2012). This has implications for the capture and treatment of drinking water where, for example, water sources may become contaminated with volatile organic compounds and high sediment loads, which pose a threat to human health (Solomon et al. 2021).
Supporting services refer to the platform where geodiversity provides for other systems and services to exist, including the occurrence of soils, their processes, and utilitarian uses (Gray 2011, 2019). Fire directly impacts the capacity of soils to support life through altered physiochemical properties, potentially reducing the suitability of fire-effected areas for certain species. Such changes may facilitate the settlement of new or opportunistic species to an area with further implications for local ecology (Williams-Jara et al. 2022). Where fertility and water retention capacity of soils have decreased following fires, repeated events preferentially facilitate the growth and proliferation of fire-tolerant soil microbial species, altering the organic component and nutrient cycles of soils (Muñoz-Rojas et al. 2016; Lombao et al. 2020; Sulaeman et al. 2021). Profound soil microbial change can equal plant community change.
Geodiversity also provides a supporting service through the provision of habitat. Fire events are part of the formation of inselbergs in inland and arid regions due to fire-induced rock spalling of exposed outcrops. These formations increase the availability of sediments, simultaneously generating important ecological niches and thus provisioning habitat (Buckman et al. 2021). Conversely, fire events can also degrade habitats, through a deterioration of water quality (Ré et al. 2021). Carbon capture and storage is an additional supporting service provided by the geosystem, and as noted earlier, is directly impacted through the destruction of geodiversity elements like peatlands, as well as through the combustion of coal, oil, and gas reservoirs.
Cultural services are intangible benefits humans garner from ecosystems and include aesthetic, educational, touristic and knowledge values (Millennium Ecosystem Assessment Program 2005). Landscape character shapes the connection that people feel towards places that provides spiritual and cultural benefit. Therefore, alterations to or loss of elements or features of landscapes are detrimental to the provision of cultural services. For example, a 1997 wildfire in Indonesia, which indirectly caused the death of the Mentawai Island coral reef ecosystem, disrupted the local narrative and connection to the reef landscape (Abram et al. 2003). Abram et al. (2003) proposed the smoke produced by the Indonesian wildfire led to atmospheric fallout of dust over the reef, which aided in the growth of a red algae bloom and subsequently asphyxiated the reef. This impact to the environmental quality of the local landscape had implications for the recreational, leisure and touristic opportunities available in the Mentawai Island region as well as cascading negative impacts for habitat, biodiversity, and natural wave barrier protection.
Similarly, the indirect impact of fires on vegetation recovery through altered soil properties may result in ecological succession in landscapes that is dissimilar to previous states (Marafa and Chau 1999). For example, fire events have been identified as responsible for shifts between mature ecosystems of conifer forests to shrublands in Mediterranean ecosystems, as these systems have lower requirements for soil nutrients (Caon et al. 2014). Such changes impact the aesthetics of a landscape and can therefore alter the sense of place felt, which may be particularly detrimental when the prior landscape has significant spiritual value.
Geotourism is also directly and indirectly affected by fire events; for example, the integrity of rock-climbing routes may be damaged due to spalling rock surfaces, thereby creating hazardous climbing conditions (Yeste-Lizán et al. 2023).
Provisioning services are goods and services obtained from ecosystems and geosystem, including critical services like food, fresh and mineral water, and utilitarian goods such as fuel and fibre (Millennium Ecosystem Assessment Program 2005; Gray 2011). Provisioning geosystem services are more utilitarian than the former categories, though nonetheless provide several critical services for the environment, such as processes involved in nutrient and water provision. Fire modifies water provision through changes to turbidity and chemical and nutrient concentrations; however, fuel, construction materials (stone, gravel, sand), and industrial minerals (metals) that are provisioned through geosystem services are largely unaffected by fires due to their non-flammable nature. Instead, the occurrence of fires may impact the infrastructure required to utilise these services or their availability. For instance, wildfires may cause the shutdown of production plants or roads.
As part of geosystem services only, knowledge services encompass the information and knowledge provided by geodiversity which contributes to science, education, and understanding (Gray 2011). Ice cores are valuable archives offering insights into historical climate patterns, atmospheric composition, and anthropogenic influences, as well as provide information about past fire occurrence and severity (Mayewski et al. 2017; Sierra-Hernández et al. 2022). Fires contribute to the degradation of ice zones through the release of black carbon and aerosols, which along with direct heat transfer, accelerate the melting of ice zones including glaciers and permafrost regions. Furthermore, the accelerated melting of glaciers hinders efforts to precisely reconstruct past glacial dynamics which consequences for the scientific understanding of geomorphological processes.
Fires that alter the physical and chemical properties of geodiversity elements may degrade their scientific integrity. For example, thermal alterations to sediments and rocks can compromise the reliability of dating methods including thermoluminescence and radiocarbon dating, which has implications for accurately understanding the ages of formations and processes (Brown 2020). Further, the increased frequency and intensity of fire events, and the emerging occurrence of megafires, is disruptive to our understanding of past climates and their applicability to current and future scenarios. As a case in point, a 2010 study of paleontological evidence in Alaska’s tundra revealed no fire events in the 5000-year period leading up to an unprecedented wildfire in 2007 (Hu et al. 2010). The reliability of knowledge gained from geological records is being compromised by accelerating and intensifying fires, with implications extending to our understanding of fire-climate interactions and ability to produce accurate models. Hence, the knowledge services provided by geosystems may be undermined by the occurrence of fire.
Implications for management
A constraint for current fire managers is the inability to anticipate and plan for when fire will be impactful at scale or will cause ongoing disruption to functions and values through time. Fire is a key agent in the (re)structuring of natural terrestrial systems and results in transformations that occur across a complex spatio-temporal distribution, including beyond the scales of contemporary geoconservation (Moritz et al. 2010; Whitlock et al. 2010; Pezzatti et al. 2013; Higuera 2015). Managing geodiversity requires a valuation of geodiversity elements, recognition of potential and actual threats, and effective action to mitigate and manage degradative pressures (Brilha et al. 2018). Fire has the potential to damage geodiversity elements, with the likelihood of fire occurrence, fire severity, geodiversity element vulnerability and sensitivity, and post-fire processes all influential in determining the occurrence of a negative impact.
Severe or total loss of values can present management implications for geoheritage, as it may undermine the justification for the protection of the geodiversity element or process. Where geodiversity elements impacted by fire have lost significant, important, or unique values and functions, recovery is paramount, particularly for geoheritage sites that may require the recovery of values in order to retain certain statutory protections (e.g. World Heritage Areas, loss of outstanding universal values associated with criterion VIII Earths History, could jeopardise the listing).
The escalating threat of wildfires with climate changes poses a significant risk to the integrity of protected areas, potentially leading to downgrading, downsizing or even degazetting of these critical conservation zones. The intensity and scale of megafires in particular can undermine the capacity of protected areas to sustain their management objectives and ecological functions. The destruction of habitats, alteration of vegetation dynamics and soil degradation resulting from intense fires can compromise their integrity as geoheritage. Moreover, the increasing frequency and severity of wildfires may necessitate a re-evaluation of the boundaries and management strategies of protected areas. This may involve resizing protected areas to better align with the changing ecological conditions, modifying management plans to incorporate new fire regimes, or in extreme cases, considering the degazetting of certain areas no longer viable for conservation.
The International Union for the Conservation of Nature (IUCN) guidelines for applying protected area management categories explicitly consider among the objectives common to all protected areas the need to: (1) maintain diversity of landscape or habitat; (2) conserve significant landscape features, geomorphology and geology; and (3) conserve natural and scenic areas of national and international significance for cultural, spiritual and scientific purposes (IUCN 2008). To date, the authors are unaware of an instance in which fire, wild or otherwise, has led to the delisting or loss of protected area status because of a total loss of geodiversity elements and/or their associated listing values. This does not mean that such a scenario could not happen. For example, high-value peatlands and their organic soils can be completely lost to fire (e.g. Turetsky et al. 2015; Davies et al. 2016; Kiely et al. 2021). As fire increases in severity and frequency globally, curators and managers of geoheritage and geodiversity may find themselves reflecting on how to exclude fire to maintain integrity and value of elements in protected areas. For now, the learning framework offered by adaptive management enables land managers to consider deleterious changes in condition and make decisions on when it may be more appropriate to direct resources to fire exclusion, instead of post-fire recovery efforts.
Conclusion
Ultimately, fire has the potential to disrupt functions and values associated with geodiversity, resulting in cumulative impacts on vast spatial and temporal scales. Geodiversity is valuable in its contribution to natural systems including the provisioning of ecosystem and geosystem services, and it holds associated socio-cultural, economic, and educational values, which benefit protected areas. Where fire events devalue geodiversity elements, the protection of the element may be threatened, thereby presenting management implications for the recovery of function and value. Our ability to preserve, protect, and recover values diminished by fire events is limited by our understanding of the extent of impacts, owing to limited research on the geosystem-wide implications of fire and cumulative impacts over extended periods. Therefore, effective, adaptive management practices are required to conserve functions and values of geodiversity in the face of degradative fire events.
Data availability
This review article synthesises and analyses existing literature, reports, and data sources that are publicly accessible and referenced in the citations.
Acknowledgements
The authors acknowledge Kathryn Storey and Luke Wallace for their comments on earlier drafts of this manuscript.
Author contributions
All authors researched data for the article, wrote the article, made a substantial contribution to discussion of content, and reviewed and/or edited the manuscript before submission.
References
Abney RB, Sanderman J, Johnson D, Fogel ML, Berhe AA (2017) Post-wildfire rosion in mountainous terrain leads to rapid and major redistribution of soil organic carbon. Frontiers in Earth Science 5, 99.
| Crossref | Google Scholar |
Abram NJ, Gagan MK, Mcculloch MT, Chappell J, Hantoro WS (2003) Coral reef death during the 1997 Indian Ocean dipole linked to Indonesian wildfires. Science 301(5635), 952-955.
| Crossref | Google Scholar | PubMed |
Adams MA (2013) Mega-fires, tipping points and ecosystem services: managing forests and woodlands in an uncertain future. Forest Ecology and Management 294, 250-261.
| Crossref | Google Scholar |
Agbeshie AA, Abugre S, Atta-Darkwa T, Awuah R (2022) A review of the effects of forest fire on soil properties. Journal of Forestry Research 33, 1419-1441.
| Crossref | Google Scholar |
Allam L (2020) Grave fears held for thousands of rock art sites after bushfires lay bare irrevocable damage. The Guardian, 2 February. Available at http://www.theguardian.com/artanddesign/2020/feb/02/grave-fears-held-for-thousands-of-rock-art-sites-after-bushfires-lay-bare-irrevocable-damage [verified 24 November 2023]
Anderson SP (2019) Breaking it down: mechanical processes in the weathering engine. Elements 15, 247-252.
| Crossref | Google Scholar |
Aubry-Wake C, Bertoncini A, Pomeroy JW (2022) Fire and ice: the impact of wildfire-affected albedo and irradiance on glacier melt. Earth’s Future 10, e2022EF002685.
| Crossref | Google Scholar |
Barreiro A, Díaz-Raviña M (2021) Fire impacts on soil microorganisms: mass, activity, and diversity. Current Opinion in Environmental Science & Health 22, 100264.
| Crossref | Google Scholar |
Belyea LR, Clymo RS (2001) Feedback control of the rate of peat formation. Proceedings of the Royal Society. Series B: Biological Sciences 268, 1315-1321.
| Crossref | Google Scholar | PubMed |
Bétard F, Peulvast J-P (2019) Geodiversity hotspots: concept, method and cartographic application for geoconservation purposes at a regional scale. Environmental Management 63, 822-834.
| Crossref | Google Scholar | PubMed |
Bian F, Coleborn K, Flemons I, Baker A, Treble PC, Hughes CE, Baker A, Andersen MS, Tozer MG, Duan W, Fogwill CJ, Fairchild IJ (2019) Hydrological and geochemical responses of fire in a shallow cave system. Science of the Total Environment 662, 180-191.
| Crossref | Google Scholar | PubMed |
Blackwood St A, Lawrence A, Wright IA, Smith G, Fitzgerald G (2021) Fire and water in the Blue Mountains: lessons from a long term monitoring program. In ‘Proceedings of the 10th Australian Stream Management Conference’, 2–4 August 2021. pp. 87–94. Available at https://researchdirect.westernsydney.edu.au/islandora/object/uws%3A62811/ [verified 24 November 2023]
Boerner REJ (2006) Soil, Fire, Water, and Wind: How the elements conspire in the forest context. In ‘Fire in eastern oak forests: delivering science to land managers: Proceedings of a conference’, 15–17 November 2005, Ohio State University, Columbus, Ohio. (Ed. MB Dickenson) (USDA Forest Service, Northern Research Station)
Boháč A, Drápela E (2023) Present climate change as a threat to geoheritage: the wildfire in Bohemian Switzerland National Park and its use in place-based learning. Geosciences 13(12), 383.
| Crossref | Google Scholar |
Boothroyd A, McHenry M (2019) Old processes, new movements: the inclusion of geodiversity in biological and ecological discourse. Diversity 11, 216.
| Crossref | Google Scholar |
Bowman DMJS, Williamson GJ (2021) River flows are a reliable index of forest fire risk in the temperate Tasmanian wilderness world heritage area, Australia. Fire 4, 22.
| Crossref | Google Scholar |
Bowman DMJS, Kolden CA, Abatzoglou JT, Johnston FH, van der Werf GR, Flannigan M (2020) Vegetation fires in the Anthropocene. Nature Reviews Earth & Environment 1, 500-515.
| Crossref | Google Scholar |
Brilha J, Gray M, Pereira DI, Pereira P (2018) Geodiversity: an integrative review as a contribution to the sustainable management of the whole of nature. Environmental Science & Policy 86, 19-28.
| Crossref | Google Scholar |
Brotóns V, Tomás R, Ivorra S, Alarcón JC (2013) Temperature influence on the physical and mechanical properties of a porous rock: San Julian’s calcarenite. Engineering Geology 167, 117-127.
| Crossref | Google Scholar |
Brown ND (2020) Which geomorphic processes can be informed by luminescence measurements? Geomorphology 367, 107296.
| Crossref | Google Scholar |
Buckman S, Morris RH, Bourman RP (2021) Fire-induced rock spalling as a mechanism of weathering responsible for flared slope and inselberg development. Nature Communications 12, 2150.
| Crossref | Google Scholar | PubMed |
Busse MD, Hubbert KR, Fiddler GO, Shestak CJ, Powers RF (2005) Lethal soil temperatures during burning of masticated forest residues. International Journal of Wildland Fire 14, 267-276.
| Crossref | Google Scholar |
Caon L, Vallejo VR, Ritsema CJ, Geissen V (2014) Effects of wildfire on soil nutrients in Mediterranean ecosystems. Earth-Science Reviews 139, 47-58.
| Crossref | Google Scholar |
Carroll R, Wright IA, Reynolds JK (2023) Loss of soil carbon in a world heritage peatland following a bushfire. International Journal of Wildland Fire 32, 1059-1070.
| Crossref | Google Scholar |
Certini G (2005) Effects of fire on properties of forest soils: a review. Oecologia 143, 1-10.
| Crossref | Google Scholar | PubMed |
Christensen NL (1977) Fire and soil-plant nutrient relations in a pine-wiregrass savanna on the coastal plain of North Carolina. Oecologia 31, 27-44.
| Crossref | Google Scholar | PubMed |
Coleborn K, Spate A, Tozer M, Andersen MS, Fairchild IJ, MacKenzie B, Treble PC, Meehan S, Baker A, Baker A (2016) Effects of wildfire on long-term soil CO2 concentration: implications for karst processes. Environmental Earth Sciences 75, 330.
| Crossref | Google Scholar |
Coleborn K, Baker A, Treble PC, Andersen MS, Baker A, Tadros CV, Tozer M, Fairchild IJ, Spate A, Meehan S (2018) The impact of fire on the geochemistry of speleothem-forming drip water in a sub-alpine cave. Science of the Total Environment 642, 408-420.
| Crossref | Google Scholar | PubMed |
Collins L, Bradstock RA, Clarke H, Clarke MF, Nolan RH, Penman TD (2021) The 2019/2020 mega-fires exposed Australian ecosystems to an unprecedented extent of high-severity fire. Environmental Research Letters 16, 044029.
| Crossref | Google Scholar |
Cooper HW, Simmons G (1977) The effect of cracks on the thermal expansion of rocks. Earth and Planetary Science Letters 36, 404-412.
| Crossref | Google Scholar |
Cotrufo MF, Boot CM, Kampf S, Nelson PA, Brogan DJ, Covino T, Haddix ML, MacDonald LH, Rathburn S, Ryan‐Bukett S, Schmeer S, Hall E (2016) Redistribution of pyrogenic carbon from hillslopes to stream corridors following a large montane wildfire. Global Biogeochemical Cycles 30, 1348-1355.
| Crossref | Google Scholar |
Crofts R (2018) Putting geoheritage conservation on all agendas. Geoheritage 10, 231-238.
| Crossref | Google Scholar |
Crofts R, Gordon JE, Brilha J, Gray M, Gunn J, Larwood J, Santucci V, Tormey D, Worboys GL (2020) Guidelines for geoconservation in protected and conserved areas. In ‘Best Practice Protected Area Guidelines Series’. (Ed. C Groves) pp. 1–160. (IUCN: Gland, Switzerland) 10.2305/IUCN.CH.2020.PAG.31.en
Crofts R, Tormey D, Gordon JE (2021) Introducing new guidelines on geoheritage conservation in protected and conserved areas. Geoheritage 13, 33.
| Crossref | Google Scholar |
Davies GM, Kettridge N, Stoof CR, Gray A, Ascoli D, Fernandes PM, Marrs R, Allen KA, Doerr SH, Clay GD, McMorrow J, Vandvik V (2016) The role of fire in UK peatland and moorland management: the need for informed, unbiased debate. Philosophical transactions of the Royal Society. Series B, Biological Sciences 371(1696), 20150342.
| Crossref | Google Scholar | PubMed |
Deb P, Moradkhani H, Abbaszadeh P, Kiem AS, Engström J, Keellings D, Sharma A (2020) Causes of the widespread 2019-2020 Australian bushfire season. Earth’s Future 8, e2020EF001671.
| Crossref | Google Scholar |
DeBano LF (1981) Water repellent soils: a state-of-the-art. Research Paper PSW-GTR-46. (USDA Forest Service, Pacific Southwest Forest and Range Experiment Station: Berkeley, CA) 10.2737/PSW-GTR-46
DeBano LF (1990) The effect of fire on soil properties. USDA Forest Service Rocky Mountain Research Station. In ‘Symposium on Management and Productivity of Western-Montane Forest Soils’, 10–12 April 1990, Boise, ID. Available at https://forest.moscowfsl.wsu.edu/smp/solo/documents/GTRs/INT_280/DeBano_INT-280.php [verified 24 November 2024]
DeBano LF (2000a) The role of fire and soil heating on water repellency in wildland environments: a review. Journal of Hydrology 231–232, 195-206.
| Crossref | Google Scholar |
DeBano LF (2000b) Water repellency in soils: a historical overview. Journal of Hydrology 231–232, 4-32.
| Crossref | Google Scholar |
Dixon G, Tasmanian Regional Forest Agreement Environment and Heritage Technical Committee Australian Heritage Commission (1997) ‘National Estate Values (geoconservation). Conservation management guidelines for geodiversity: report to the Tasmanian RFA Environment and Heritage Technical Committee.’ (Tasmanian Regional Forest Agreement Environment and Heritage Technical Committee: Hobart, Tas.)
Fisher A, Hesse PP (2019) The response of vegetation cover and dune activity to rainfall, drought and fire observed by multitemporal satellite imagery. Earth Surface Processes and Landforms 44, 2957-2967.
| Crossref | Google Scholar |
Florsheim JL, Chin A, O’Hirok LS, Storesund R (2016) Short-term post-wildfire dry-ravel processes in a chaparral fluvial system. Geomorphology 252, 32-39.
| Crossref | Google Scholar |
Fox DM, Darboux F, Carrega P (2007) Effects of fire-induced water repellency on soil aggregate stability, splash erosion, and saturated hydraulic conductivity for different size fractions. Hydrological Processes 21, 2377-2384.
| Crossref | Google Scholar |
Gao C, Cong J, Sun Y, Han D, Wang G, Gao C, Cong J, Sun Y, Han D, Wang G (2022) Variability in pyrogenic carbon properties generated by different burning temperatures and peatland plant litters: implication for identifying fire intensity and fuel types. International Journal of Wildland Fire 31, 395-408.
| Crossref | Google Scholar |
Gavin DG, Hallett DJ, Hu FS, Lertzman KP, Prichard SJ, Brown KJ, Lynch JA, Bartlein P, Peterson DL (2007) Forest fire and climate change in western North America: insights from sediment charcoal records. Frontiers in Ecology and the Environment 5, 499-506.
| Crossref | Google Scholar |
González-Pérez JA, González-Vila FJ, Almendros G, Knicker H (2004) The effect of fire on soil organic matter—a review. Environment International 30, 855-870.
| Crossref | Google Scholar | PubMed |
Gordon JE (2019) Geoconservation principles and protected area management. International Journal of Geoheritage and Parks 7, 199-210.
| Crossref | Google Scholar |
Gordon JE, Barron HF (2012) Valuing geodiversity and geoconservation: developing a more strategic ecosystem approach. Scottish Geographical Journal 128, 278-297.
| Crossref | Google Scholar |
Gordon JE, Tormey D, Wignall R, Brazier V, Crofts R (2022a) Climate change will challenge the management of geoheritage in protected and conserved areas. Parks Stewardship Forum 38, 56-63.
| Crossref | Google Scholar |
Gordon JE, Wignall RML, Brazier V, Crofts R, Tormey D (2022b) Planning for climate change impacts on geoheritage interests in protected and conserved areas. Geoheritage 14, 126.
| Crossref | Google Scholar |
Gray M (2005) Geodiversity and geoconservation: what, why, and how? The George Wright Forum 22, 4-12 Available at https://www.jstor.org/stable/43597951 [verified 11 April 2023].
| Google Scholar |
Gray M (2011) Other nature: geodiversity and geosystem services. Environmental Conservation 38, 271-274.
| Crossref | Google Scholar |
Gray M (2019) Geodiversity, geoheritage and geoconservation for society. International Journal of Geoheritage and Parks 7, 226-236.
| Crossref | Google Scholar |
Gray M, Gordon JE, Brown EJ (2013) Geodiversity and the ecosystem approach: the contribution of geoscience in delivering integrated environmental management. Proceedings of the Geologists Association 124, 659-673.
| Crossref | Google Scholar |
Grier CC (1975) Wildfire effects on nutrient distribution and leaching in a coniferous ecosystem. Canadian Journal of Forest Research 5, 599-607.
| Crossref | Google Scholar |
Harwood CE, Jackson WD (1975) Atmospheric losses of four plant nutrients during a forest fire. Australian Forestry 38, 92-99.
| Crossref | Google Scholar |
Hawbaker TJ, Radeloff VC, Stewart SI, Hammer RB, Keuler NS, Clayton MK (2013) Human and biophysical influences on fire occurrence in the United States. Ecological Applications 23, 565-582.
| Crossref | Google Scholar | PubMed |
Heap D (1999) Human impact on the Mount Cripps Karst. ACKMA Journal 37, 4-11 Available at https://ackma.org/journal/37/Human%20Impact%20on%20the%20Mount%20Cripps%20Karst%20-%20David%20Heap.pdf [verified 24 November 2023].
| Google Scholar |
Higuera PE (2015) Taking time to consider the causes and consequences of large wildfires. Proceedings of the National Academy of Sciences 112, 13137-13138.
| Crossref | Google Scholar | PubMed |
Hilger J, Englin J (2009) Utility theoretic semi-logarithmic incomplete demand systems in a natural experiment: forest fire impacts on recreational values and use. Resource and Energy Economics 31, 287-298.
| Crossref | Google Scholar |
Holland E (1994) The effects of fire on soluble rock landscapes. Helictite Journal of Australasian Cave Research 32, 3. Available at https://helictite.caves.org.au/pdf5/32.01.Issue.Screen.pdf [verified 24 November 2023].
| Google Scholar |
Hu SF, Higuera PE, Walsh JE, Chapman WL, Duffy PA, Brubaker LB, Chipman ML (2010) Tundra burning in Alaska: Linkages to climatic change and sea ice retreat. JGR Biogeosciences 115(G4), G04002.
| Crossref | Google Scholar |
Hubbard RM, Vose JM, Clinton BD, Elliott KJ, Knoepp JD (2004) Stand restoration burning in oak–pine forests in the southern Appalachians: effects on aboveground biomass and carbon and nitrogen cycling. Forest Ecology and Management 190, 311-321 Available at https://www.academia.edu/15968519/Stand_restoration_burning_in_oak_pine_forests_in_the_southern_Appalachians_effects_on_aboveground_biomass_and_carbon_and_nitrogen_cycling [verified 15 June 2023].
| Crossref | Google Scholar |
Ice GG, Neary DG, Adams PW (2004) Effects of wildfire on soils and watershed processes. Journal of Forestry 102, 16-20.
| Crossref | Google Scholar |
Ielpi A, Lapôtre MGA (2023) Modelling fire-induced perturbations in sediment flux based on stream widening and accelerated bank migration. CATENA 228, 107173.
| Crossref | Google Scholar |
IUCN (2008) Resolutions and recommendations adopted at the 4th IUCN world conservation congress. Resolution 4.040: Conservation of geodiversity and geological heritage. Available at https://portals.iucn.org/library/node/44190 [verified 25 January 2024]
Jones BM, Grosse G, Arp CD, Miller E, Liu L, Hayes DJ, Larsen CF (2015) Recent Arctic tundra fire initiates widespread thermokarst development. Scientific Reports 5, e15865.
| Crossref | Google Scholar | PubMed |
Jones MW, Abatzoglou JT, Veraverbeke S, Andela N, Lasslop G, Forkel M, Smith AJP, Burton C, Betts RA, van der Werf GR, Sitch S, Canadell JG, Santín C, Kolden C, Doerr SH, Le Quéré C (2022) Global and regional trends and drivers of fire under climate change. Reviews of Geophysics 60, e2020RG000726.
| Crossref | Google Scholar |
Jong-Levinger A, Banerjee T, Houston D, Sanders BF (2022) Compound post-fire flood hazards considering infrastructure sedimentation. Earth’s Future 10, e2022EF002670.
| Crossref | Google Scholar |
Kang S, Zhang Y, Qian Y, Wang H (2020) A review of black carbon in snow and ice and its impact on the cryosphere. Earth‐Science Reviews 210, 103346.
| Crossref | Google Scholar |
Kennard DK, Gholz HL (2001) Effects of high- and low-intensity fires on soil properties and plant growth in a Bolivian dry forest. Plant and Soil 234, 119-129.
| Crossref | Google Scholar |
Khorshidi MS, Dennison PE, Nikoo MR, AghaKouchak A, Luce CH, Sadegh M (2020) Increasing concurrence of wildfire drivers tripled megafire critical danger days in Southern California between 1982 and 2018. Environmental Research Letters 15, 104002.
| Crossref | Google Scholar |
Kiely L, Spracklen DV, Arnold SR, Papargyropoulou E, Conibear L, Wiedinmyer C, Knote C, Adrianto HA (2021) Assessing costs of Indonesian fires and the benefits of restoring peatland. Nature Communications 12(1), 7044.
| Crossref | Google Scholar | PubMed |
Kutiel P, Inbar M (1993) Fire impacts on soil nutrients and soil erosion in a Mediterranean pine forest plantation. CATENA 20, 129-139.
| Crossref | Google Scholar |
Leys B, Higuera PE, McLauchlan KK, Dunnette PV (2016) Wildfires and geochemical change in a subalpine forest over the past six millennia. Environmental Research Letters 11, 125003.
| Crossref | Google Scholar |
Li X-Y, Jin H-J, Wang H-W, Marchenko SS, Shan W, Luo D-L, He R-X, Spektor V, Huang Y-D, Li X-Y, Jia N (2021) Influences of forest fires on the permafrost environment: a review. Advances in Climate Change Research 12, 48-65.
| Crossref | Google Scholar |
Linley GD, Jolly CJ, Doherty TS, Geary WL, Armenteras D, Belcher CM, Bliege Bird R, Duane A, Fletcher MS, Giorgis MA, Haslem A, Jones GM, Kelly LT, Lee CKF, Nolan RH, Parr CL, Pausas JG, Price JN, Regos A, Ritchie EG, Ruffault J, Williamson GJ, Wu Q, Nimmo DG (2022) What do you mean, ‘megafire’? Global Ecology and Biogeography 31, 1906-1922.
| Crossref | Google Scholar |
Liu Y, Liu C, Rubinato M, Guo K, Zhou J, Cui M (2020) An assessment of soil’s nutrient deficiencies and their influence on the restoration of degraded karst vegetation in southwest China. Forests 11, 797.
| Crossref | Google Scholar |
Lombao A, Barreiro A, Fontúrbel MT, Martín A, Carballas T, Díaz-Raviña M (2020) Key factors controlling microbial community responses after a fire: importance of severity and recurrence. Science of the Total Environment 741, 140363.
| Crossref | Google Scholar | PubMed |
Loranty MM, Alexander HD, Kropp H, Talucci AC, Webb EE (2021) Siberian ecosystems as drivers of cryospheric climate feedbacks in the terrestrial Arctic. Frontiers in Climate 3, 730943.
| Crossref | Google Scholar |
Mack MC, Bret-Harte MS, Hollingsworth TN, Jandt RR, Schuur EAG, Shaver GR, Verbyla DL (2011) Carbon loss from an unprecedented Arctic tundra wildfire. Nature 475, 489-492.
| Crossref | Google Scholar | PubMed |
Mahat V, Silins U, Anderson A (2016) Effects of wildfire on the catchment hydrology in southwest Alberta. CATENA 147, 51-60.
| Crossref | Google Scholar |
Marafa LM, Chau KC (1999) Effect of hill fire on upland soil in Hong Kong. Forest Ecology and Management 120, 97-104.
| Crossref | Google Scholar |
Mataix-Solera J, Cerdà A, Arcenegui V, Jordán A, Zavala LM (2011) Fire effects on soil aggregation: a review. Earth-Science Reviews 109, 44-60.
| Crossref | Google Scholar |
Mayer M, Prescott CE, Abaker WEA, Augusto L, Cécillon L, Ferreira GWD, James J, Jandl R, Katzensteiner K, Laclau J-P, Laganière J, Nouvellon Y, Paré D, Stanturf JA, Vanguelova EI, Vesterdal L (2020) Tamm Review: Influence of forest management activities on soil organic carbon stocks: a knowledge synthesis. Forest Ecology and Management 466, 118127.
| Crossref | Google Scholar |
Mayewski PA, Carleton AM, Birkel SD, Dixon D, Kurbatov AV, Korotkikh E, McConnell J, Curran M, Cole-Dai J, Jiang S, Plummer C, Vance T, Maasch KA, Sneed SB, Handley M (2017) Ice core and climate reanalysis analogs to predict Antarctic and Southern Hemisphere climate changes. Quaternary Science Reviews 155, 50-66.
| Crossref | Google Scholar |
McDonough LK, Treble PC, Baker A, Borsato A, Frisia S, Nagra G, Coleborn K, Gagan MK, Zhao J, Paterson D (2022) Past fires and post-fire impacts reconstructed from a southwest Australian stalagmite. Geochimica et Cosmochimica Acta 325, 258-277.
| Crossref | Google Scholar |
McGuire LA, Youberg AM (2019) Impacts of successive wildfire on soil hydraulic properties: implications for debris flow hazards and system resilience. Earth Surface Processes and Landforms 44, 2236-2250.
| Crossref | Google Scholar |
Miller D, Luce C, Benda L (2003) Time, space, and episodicity of physical disturbance in streams. Forest Ecology and Management 178, 121-140.
| Crossref | Google Scholar |
Moody JA, Ebel BA, Nyman P, Martin DA, Stoof C, McKinley R (2016) Relations between soil hydraulic properties and burn severity. International Journal of Wildland Fire 25, 279-293.
| Crossref | Google Scholar |
Molina LT, Gallardo L, Andreade M, Baumgardner D, Borbor‐Córdova M, Bórquez R, Casassa G, Cereceda‐Balic F, Dawidowski L, Garreaud R, Huneeus N, Lambert F, McCarty JL, Mc Phee J, Mena‐Carrasco M, Raga GB, Schmitt C, Schwarz JP (2015) Pollution and its impacts on the South American cryosphere. Earth’s Future 3, 345-369.
| Crossref | Google Scholar |
Moritz MA, Krawchuk M, Parisien M-A (2010) Pyrogeography: understanding the ecological niche of fire. PAGES News 18, 83-85.
| Crossref | Google Scholar |
Muñoz-Rojas M, Erickson TE, Martini D, Dixon KW, Merritt DJ (2016) Soil physicochemical and microbiological indicators of short, medium and long term post-fire recovery in semi-arid ecosystems. Ecological Indicators 63, 14-22.
| Crossref | Google Scholar |
Nagra G, Treble PC, Andersen MS, Fairchild IJ, Coleborn K, Baker A (2016) A post-wildfire response in cave dripwater chemistry. Hydrology and Earth System Sciences 20, 2745-2758.
| Crossref | Google Scholar |
Natural Values Conservation Branch (2017) Assessment of the ecological impacts of the 2016 Mersey Forest Fire Complex. Nature Conservation Report No. 17/5. (Department of Primary Industries, Parks, Water and Environment: Hobart, Tas.) Available at https://dpipwe.tas.gov.au/Documents/Mersey_Forest_Fire_Impact_Report_Final_170802.pdf [verified 24 November 2023]
Neary DG, Klopatek CC, DeBano LF, Ffolliott PF (1999) Fire effects on belowground sustainability: a review and synthesis. Forest Ecology and Management 122, 51-71.
| Crossref | Google Scholar |
Olorunfemi IE, Ogunrinde TA, Fasinmirin JT (2014) Soil hydrophobicity: an overview. Journal of Scientific Research and Reports 3, 1003-1037.
| Crossref | Google Scholar |
Pearson M (2015) Climate Change, Fire and Cultural Heritage in Australia. Heritage at Risk: ICOMOS World Report 2006-2007 on Monuments and Sites in Danger. 10.11588/hr.2008.0.19895
Pereira P, Francos M, Brevik EC, Ubeda X, Bogunovic I (2018) Post-fire soil management. Current Opinion in Environmental Science & Health 5, 26-32.
| Crossref | Google Scholar |
Pettit NE, Naiman RJ (2007) Fire in the Riparian Zone: characteristics and ecological consequences. Ecosystems 10, 673-687.
| Crossref | Google Scholar |
Pezzatti GB, Zumbrunnen T, Bürgi M, Ambrosetti P, Conedera M (2013) Fire regime shifts as a consequence of fire policy and socio-economic development: an analysis based on the change point approach. Forest Policy and Economics 29, 7-18.
| Crossref | Google Scholar |
Pierson FB, Moffet CA, Williams CJ, Hardegree SP, Clark PE (2009) Prescribed-fire effects on rill and interrill runoff and erosion in a mountainous sagebrush landscape. Earth Surface Processes and Landforms 34, 193-203.
| Crossref | Google Scholar |
Pliscoff P, Folchi M, Aliste E, Cea D, Simonetti JA (2020) Chile mega-fire 2017: an analysis of social representation of forest plantation territory. Applied Geography 119, 102226.
| Crossref | Google Scholar |
Praskievicz S, Sigdel R (2021) Loading of stream wood following the 2016 Chimney Tops 2 Wildfire: Great Smoky Mountains National Park, Tennessee, USA. River Research and Applications 37, 475-483.
| Crossref | Google Scholar |
Ramos AM, Russo A, DaCamara CC, Nunes S, Sousa P, Soares PMM, Lima MM, Hurduc A, Trigo RM (2023) The compound event that triggered the destructive fires of October 2017 in Portugal. iScience 26(3), 106141.
| Crossref | Google Scholar | PubMed |
Ré A, Campos I, Keizer JJ, Gonçalves FJM, Pereira JL, Abrantes N (2021) Effects of post-fire contamination in sediment-dwelling species of riverine systems. Science of the Total Environment 771, 144813.
| Crossref | Google Scholar | PubMed |
Roehner C, Pierce JL, Yager EM (2020) Spatial and temporal changes in aeolian redistribution of sediments and nutrients following fire. Earth Surface Processes and Landforms 45, 2556-2571.
| Crossref | Google Scholar |
Santín C, Otero XL, Doerr SH, Chafer CJ (2018) Impact of a moderate/high-severity prescribed eucalypt forest fire on soil phosphorous stocks and partitioning. Science of the Total Environment 621, 1103-1114.
| Crossref | Google Scholar | PubMed |
Seibert J, McDonnell JJ, Woodsmith RD (2010) Effects of wildfire on catchment runoff response: a modelling approach to detect changes in snow-dominated forested catchments. Hydrology Research 41, 378-390.
| Crossref | Google Scholar |
Semeniuk V, Semeniuk CA (2005) Wetland sediments and soils on the Swan Coastal Plain, southwestern Australia: types, distribution, susceptibility to combustion, and implications for fire management. Journal of the Royal Society of Western Australia 88, 91-120 Available at https://www.researchgate.net/publication/287639691_Wetland_sediments_and_soils_on_the_Swan_Coastal_Plain_southwestern_Australia_Types_distribution_susceptibility_to_combustion_and_implications_for_fire_management [verified 24 November 2023].
| Google Scholar |
Shakesby RA (2011) Post-wildfire soil erosion in the Mediterranean: review and future research directions. Earth-Science Reviews 105, 71-100.
| Crossref | Google Scholar |
Shakesby R, Doerr S (2006) Wildfire as a hydrological and geomorphological agent. Earth-Science Reviews 74, 269-307.
| Crossref | Google Scholar |
Shtober-Zisu N, Tessler N, Tsatskin A, Greenbaum N (2015) Accelerated weathering of carbonate rocks following the 2010 wildfire on Mount Carmel, Israel. International Journal of Wildland Fire 24, 1154-1167.
| Crossref | Google Scholar |
Shtober-Zisu N, Brook A, Kopel D, Roberts D, Ichoku C, Wittenberg L (2018) Fire induced rock spalls as long-term traps for ash. CATENA 162, 88-99.
| Crossref | Google Scholar |
Shumack S, Hesse P, Turner L (2017) The impact of fire on sand dune stability: surface coverage and biomass recovery after fires on Western Australian coastal dune systems from 1988 to 2016. Geomorphology 299, 39-53.
| Crossref | Google Scholar |
Sierra-Hernández MR, Beaudon E, Porter SE, Mosley‐Thompson E, Thompson LG (2022) Increased fire activity in Alaska since the 1980s: evidence from an ice core-derived black carbon record. JGR Atmospheres 127(2), e2021JD035668.
| Crossref | Google Scholar |
Solomon GM, Hurley S, Carpenter C, Young TM, English P, Reynolds P (2021) Fire and water: assessing drinking water contamination after a major wildfire. ACS EST Water 1, 1878-1886.
| Crossref | Google Scholar | PubMed |
Stavi I (2019) Wildfires in grasslands and shrublands: a review of impacts on vegetation, soil, hydrology, and geomorphology. Water 11, 1042.
| Crossref | Google Scholar |
Stavi I, Barkai D, Knoll YM, Glion HA, Katra I, Brook A, Zaady E (2017) Fire impact on soil-water repellency and functioning of semi-arid croplands and rangelands: implications for prescribed burnings and wildfires. Geomorphology 280, 67-75.
| Crossref | Google Scholar |
Stolton S, Dudley N, Çokçalışkan BA, Hunter D, Ivanić K-Z, Kanga E, Kettunen M, Kumagai Y, Maxted N, Senior J, Wong M, Keenleyside K, Mulrooney D, Waithaka J (2015) Values and Benefits of Protected Areas. In ‘Protected Area Governance and Management’. (Eds GL Worboys, M Lockwood, A Kothari, S Feary, I Pulsford) pp. 145–168. (ANU Press: ACT, Australia) Available at https://www.jstor.org/stable/j.ctt1657v5d.13 [verified 5 April 2023]
Storey K (2010) A review of the potential interactions between fire, soil, hydrology and geomorphology of buttongrass moorland. In ‘Proceedings of the 2007 Buttongrass Moorland Management Workshop’. (Ed. J Balmer) pp. 10–21. (Department of Primary Industries, Parks, Water, and Environment: Hobart, Tas.) Available at https://nre.tas.gov.au/Documents/Buttongrass-Workshop.pdf [verified 31 January 2024]
Storey K, Betts E (2011) Fluvial geomorphology and hydrology of small buttongrass moorland streams: the Gelignite Creek case study. Geodiversity Conservation and Management Section, Department of Primary Industries, Parks, Water and Environment, Nature Conservation Report 11/05, p. 122. Available at https://nre.tas.gov.au/Documents/Fluvial-Geomorphology-and-Hydrology-of-Small-Buttongrass-Moorland-Streams.pdf [verified 28 March 2024]
Sulaeman D, Sari ENN, Westhoff TP (2021) Effects of peat fires on soil chemical and physical properties: a case study in South Sumatra. IOP Conference Series: Earth and Environmental Science 648, 012146.
| Crossref | Google Scholar |
Swanson FJ (1981) Fire and geomorphic processes. (USDA Forest Service, Pacific Southwest Forest and Range Experiment Station) Available at https://wpg.forestry.oregonstate.edu/sites/wpg/files/seminars/81_swanson.pdf [verified 14 June 2023]
Tratebas AM, Cerveny NV, Dorn RI (2004) The effects of fire on rock art: microscopic evidence reveals the importance of weathering rinds. Physical Geography 25, 313-333.
| Crossref | Google Scholar |
Tulau MJ (2015) ‘Fire and Soils. A review of the potential impacts of different fire regimes on soil erosion and sedimentation, nutrient and carbon cycling, and water quantity and quality.’ (Office of Environment and Heritage: NSW, Australia). Available at https://www.environment.nsw.gov.au/~/media/6676FDEC72B546F5B849301424B29835.ashx
Tulau MJ, McInnes‐Clarke SK, Yang X, McAlpine RA, Karunaratne SB, Zhu Q, Morand DT (2019) The Warrumbungle Post‐Fire Recovery Project—raising the profile of soils. Soil Use and Management 35, 63-74.
| Crossref | Google Scholar |
Turetsky MR, Benscoter B, Page S, Rein G, Van Der Werf GR, Watts A (2015) Global vulnerability of peatlands to fire and carbon loss. Nature Geoscience 8(1), 11-14.
| Crossref | Google Scholar |
Vacchiano G, Stanchi S, Marinari G, Ascoli D, Zanini E, Motta R (2014) Fire severity, residuals and soil legacies affect regeneration of Scots pine in the Southern Alps. Science of the Total Environment 472, 778-788.
| Crossref | Google Scholar | PubMed |
van Ree CCDF, van Beukering PJH (2016) Geosystem services: a concept in support of sustainable development of the subsurface. Ecosystem Services 20, 30-36.
| Crossref | Google Scholar |
van Ree CCDF, van Beukering PJH, Boekestijn J (2017) Geosystem services: a hidden link in ecosystem management. Ecosystem Services 26, 58-69.
| Crossref | Google Scholar |
Varga K, Jones C, Trugman A, Carvalho LMV, McLoughlin N, Seto D, Thompson C, Daum K (2022) Megafires in a warming world: what wildfire risk factors led to California’s largest recorded wildfire. Fire 5(1), 16.
| Crossref | Google Scholar |
Verma S, Jayakumar S (2012) Impact of forest fire on physical, chemical and biological properties of soil: a review. Proceedings of the International Academy of Ecology and Environmental Sciences 2, 168-176.
| Google Scholar |
Vermeire LT, Wester DB, Mitchell RB, Fuhlendorf SD (2005) Fire and grazing effects on wind erosion, soil water content, and soil temperature. Journal of Environmental Quality 34, 1559-1565.
| Crossref | Google Scholar | PubMed |
Vieira DCS, Fernández C, Vega JA, Keizer JJ (2015) Does soil burn severity affect the post-fire runoff and interrill erosion response? A review based on meta-analysis of field rainfall simulation data. Journal of Hydrology 523, 452-464.
| Crossref | Google Scholar |
Ward M, Tulloch AIT, Radford JQ, Williams BA, Reside AE, Macdonald SL, Mayfield HJ, Maron M, Possingham HP, Vine SJ, O’Connor JL, Massingham EJ, Greenville AC, Woinarski JCZ, Garnett ST, Lintermans M, Scheele BC, Carwardine J, Nimmo DG, Lindenmayer DB, Kooyman RM, Simmonds JS, Sonter LJ, Watson JEM (2020) Impact of 2019-2020 mega-fires on Australian fauna habitat. Nature Ecology & Evolution 4, 1321-1326.
| Crossref | Google Scholar | PubMed |
Ward M, Southwell D, Gallagher RV, Raadik TA, Whiterod NS, Lintermans M, Sheridan G, Nyman P, Suárez‐Castro AF, Marsh J, Woinarski J, Legge S (2022) Modelling the spatial extent of post-fire sedimentation threat to estimate the impacts of fire on waterways and aquatic species. Diversity and Distributions 28, 2429-2442.
| Crossref | Google Scholar |
Warrick JA, Hatten JA, Pasternack GB, Gray AB, Goni MA, Wheatcroft RA (2012) The effects of wildfire on the sediment yield of a coastal California watershed. Geological Society of America Bulletin 124, 1130-1146.
| Crossref | Google Scholar |
White I, Wade A, Worthy M, Mueller N, Daniell T, Wasson R (2006) The vulnerability of water supply catchments to bushfires: impacts of the January 2003 wildfires on the Australian Capital Territory. Australasian Journal of Water Resources 10, 179-194.
| Crossref | Google Scholar |
Whitlock C, Higuera PE, McWethy DB, Briles CE (2010) Paleoecological perspectives on fire ecology: revisiting the fire-regime concept. The Open Ecology Journal 3, 6-23.
| Crossref | Google Scholar |
Williams-Jara GM, Espinoza-Tenorio A, Monzón-Alvarado C, Posada-Vanegas G, Infante-Mata D (2022) Fires in coastal wetlands: a review of research trends and management opportunities. Wetlands 42, 56.
| Crossref | Google Scholar |
Yeste-Lizán P, Gomez-Heras M, García-Rodríguez M, Pérez-López R, Carcavilla L, Ortega-Becerril JA (2023) Surface mechanical effects of wildfires on rocks in climbing areas. Fire 6, 46.
| Crossref | Google Scholar |
Yu Y, Ginoux P (2022) Enhanced dust emission following large wildfires due to vegetation disturbance. Nature Geoscience 15, 878-884.
| Crossref | Google Scholar |
Zimmerman SG, Evenson EB, Gosse JC, Erskine CP (1994) Extensive boulder erosion resulting from a range fire on the Type-Pinedale Moraines, Fremont Lake, Wyoming. Quaternary Research 42, 255-265.
| Crossref | Google Scholar |