Fungal consumption by marsupials in southern Tasmania
Kelsey Elliott A , Michael M. Driessen B , Karl Vernes C D and Todd F. Elliott
A
B
C
D
Abstract
In Australia, many mycophagous (fungus feeding) mammals that disperse fungal spores are extinct or threatened throughout much of their historic range. Using live-trapping, we collected scats from eastern bettongs (Bettongia gaimardi), long-nosed potoroos (Potorous tridactylus), brush-tailed possums (Trichosurus vulpecula), eastern barred bandicoots (Perameles gunnii) and southern brown bandicoots (Isoodon obesulus) at two sites in southern Tasmania. Microscopic analysis of scats revealed that all species in this study consumed fungi (over 24 fungal taxa), and the composition varied between some species and sites. This study highlights the need for additional research to gain insight into the ecological implications of spore dispersal by native marsupials.
Keywords: critical weight range mammals, diets, fungal ecology, fungivory, mammal ecology, mycophagy, mycorrhizal fungi, Potoroidae, spore dispersal, truffle ecology.
Introduction
Globally, over 600 vertebrates contribute to fungal spore dispersal (Elliott et al. 2019a, 2019b, 2022a; Nest et al. 2023) by eating fungi that form mycorrhizal associations key to plant nutrient uptake (Claridge and May 1994; Johnson 1996). Many fungal species ingested by animals form sequestrate (enclosed) fruiting bodies that are hypogeous (fruit underground). These habits have likely developed in response to animal feeding behaviours, as strong aromas released by the fungi are attractive to animals (Donaldson and Stoddart 1994; Stephens et al. 2020). In the absence of the aid of animal dispersal, most fungi disperse spores by wind (Geml et al. 2008) or move vegetatively via mycelial spread (Jonsson et al. 1999). However, only a small percentage of spores disperse beyond a few metres of the parent fruiting body (Li 2005; Galante et al. 2011) and a variety of factors can impede mycelial spread. The excavation, consumption and subsequent dispersal of fungi provided by vertebrate mycophagists is therefore a key factor in the health of mycorrhizal forest systems.
There has been significant research focus on these animal–fungal interactions within Australia (Claridge and May 1994; Nuske et al. 2017) but these associations also are widespread beyond this region. Rodents have been shown to be the most diverse group of mycophagists globally, including many Australian taxa (Vernes et al. 2015; Elliott et al. 2022a). In Australia, potoroid marsupials are the most thoroughly studied obligate mycophagists. All eight extant members of this Australian endemic family eat a diversity of fungi (Taylor 1992; Claridge and Trappe 2005; Nuske et al. 2017; Elliott et al. 2022a).
The fungal foraging activities of potoroids are important to soil disturbance and organic matter decomposition (Garkaklis et al. 2004; Newell 2009; Fleming et al. 2014; Davies et al. 2019; Palmer et al. 2020). This ecosystem service is vital to soil hydration and organic matter decomposition (Fleming et al. 2014; Davies et al. 2019; Palmer et al. 2020). While estimating soil disturbance was beyond the scope of this study, Tasmanian potoroids and other mycophagists would be expected to play a similar role in bioturbation as has been shown for related species on mainland Australia. Since colonial invasion, Australia’s potoroid mammals have suffered serious population declines and extinctions (Short and Smith 1994; Short 1998; Vernes et al. 2021). This loss has led to a reduction in spore dispersal that in turn has impacted plant and fungal communities. Given the ecological significance of services provided by members of the Potoroidae, our aim was to explore the fungal diets of these mammals in an ecosystem in which the animals remain in healthy numbers.
Materials and methods
We established two trapping sites in the South East Tasmanian bioregion: The Peter Murrell Conservation Area and State Reserve near Huntingfield, Tasmania (43°00′S, 147°18′E) and on private property near The Lea, Tasmania (42°56′08.2″S, 147°18′55.9″E). These sites are distinct and ~7 km apart. The Peter Murrell Reserves are dominated by Eucalyptus amygdalina coastal woodland with a heathy understory on sandy soils and include stands of Acacia spp. and Allocasuarina sp. The Reserves also include heathlands and some wetland areas. The site near The Lea is dominated by Eucalyptus pulchella forest and woodland, pasture (~9 ha) and some Eucalyptus globulus dry forest and woodland.
We used standard cage trapping protocols approved by the University of New England Animal Ethics Committee (Approval Number AEC18-065) and the Department of Primary Industries, Parks, Water and Environment’s Standard Operating Procedures for Live-trapping and Handling of Wild Tasmanian Mammals 2013. Traps were baited with peanut butter and vanilla on bread, and set from dusk to dawn. Captured animals were transferred to clean cloth bags to be identified, weighed and sexed. To ensure identification of recaptures, we clipped a small patch of fur from the flank of each animal for temporary identification or used subcutaneous microchips for more permanent identification. Scats were collected from each trap and placed in 70% alcohol. Traps were cleaned after each capture to prevent contamination between captures. We had 120 trap nights in the Peter Murrell Conservation Area between 26 and 29 August 2019 and 160 trap nights at The Lea site between 29 August 2019 and 1 September 2019.
Scat samples were collected from each capture and multiple pellets (from the same individual) were collected when possible to ensure accurate assessment of which fungal taxa had been eaten. Samples were wet-mounted on glass slides and analysed at 400× magnification to determine presence and species richness of fungal spores (Fig. 1). To avoid reporting incidental consumption of fungi from spores that happened to be present in the environment, only spores found to occur frequently (a minimum of five times) in each sample were considered. Spores were identified based on standard microscopic characters. Ambiguous identities were confirmed by comparison with spores of identified fungal fruiting body collections made by the authors in the same or similar habitats. We generally did not attempt to refine fungal identification beyond family or genus level. We focused on the diversity of fungi consumed and therefore apply some older nomenclatural concepts that help to outline the diversity of taxa. For example, we mention the genera Thaxterogaster and Quadrispora and our use refers to sequestrate taxa allied with Cortinarius, a speciose genus that contains both epigeous and sequestrate hypogeous species. Many recent taxonomic studies of sequestrate fungi have shown that members of historically sequestrate genera are often synonymous with epigeous genera (Gasparini 2014; Elliott and Trappe 2018; Pastor et al. 2019; Elliott et al. 2020a; Nouhra et al. 2021). We agree with these taxonomic revisions but in the context of this study we mention Thaxterogaster and Quadrispora in reference to spores of the Cortinariaceae but have characters indicating that these likely belong to sequestrate taxa. The taxa we list as Cortinarius could also be sequestrate but we have no evidence to indicate the fruiting habits.
A micrograph showing a typical view of a diversity of fungal spores in the scat of a long-nosed potoroo.
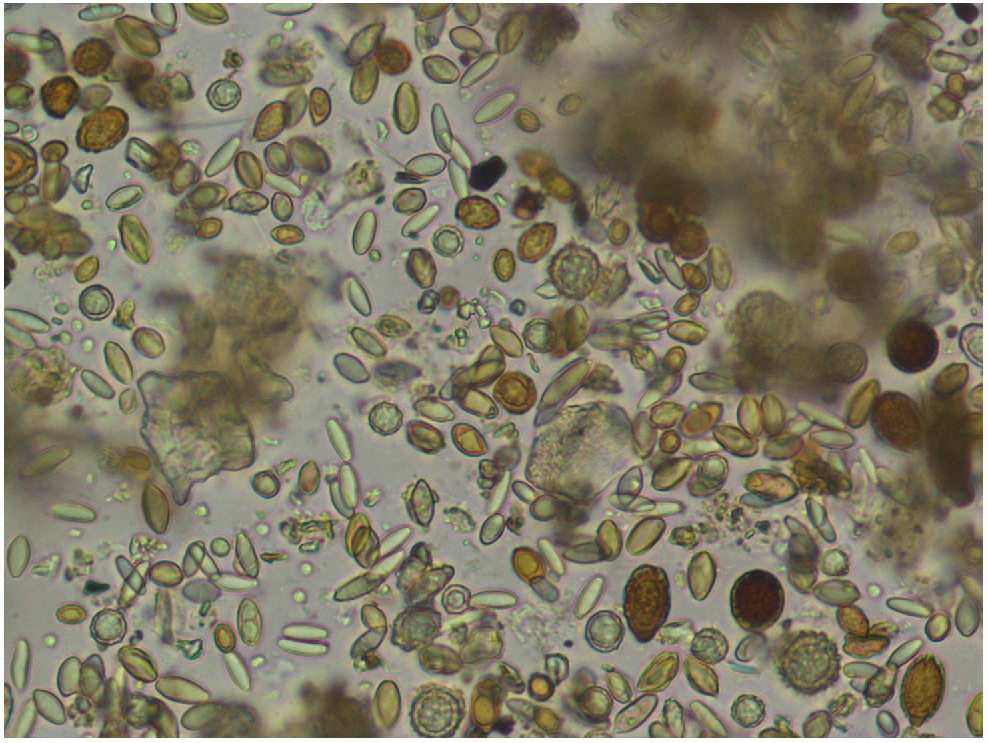
To compare fungal consumption between mammal species and sites, we conducted analysis of similarities (ANOSIM) in Primer 7 using presence/absence of fungal species in scat samples to generate Bray–Curtis similarity measures. ANOSIM returns an R-statistic that yields a measure of similarity between categories. R-values most commonly range from zero to one; the closer the R-value is to one, the more the categories differ; a value close to zero indicates that assemblages can barely be separated (Clarke and Warwick 2001). ANOSIM tests are robust to differences in the number of samples in factor groups (Clarke and Warwick 2001). To visualise multivariate patterns in vertebrate assemblage structure, multidimensional scaling was performed. Scats without fungi were excluded from the analysis, as was the single eastern barred bandicoot sample (see Table 1).
Fungal taxa | Mammal species | |||||
---|---|---|---|---|---|---|
Bettongia gaimardi (6) | Potorous tridactylus (31) | Trichosurus vulpecula (8) | Perameles gunnii (1) | Isoodon obesulus (3) | ||
Amylascus | 50.0 | 38.7 | – | – | – | |
Mesophelliaceae | 100.0 | 80.6 | 62.5 | – | – | |
Aroramyces 1 | – | 16.1 | – | – | – | |
Ascomycete (Dicina-like) | 16.7 | – | – | – | – | |
Austrogautieria | 66.7 | 35.5 | 12.5 | – | – | |
Boletoid | 100.0 | 58.1 | – | – | 33.3 | |
Cortinarius | 100.0 | 83.9 | 25.0 | – | – | |
Descomyces | – | – | – | 100.0 | – | |
Descomyces 2 | 50.0 | – | – | – | – | |
Descomyces 5 | 33.3 | 100.0 | 37.5 | – | – | |
Elaphomyces | – | 22.6 | – | – | – | |
Glomeromycota | 100.0 | 74.2 | – | – | – | |
Hydnangium | 66.7 | 19.4 | – | – | – | |
Hymenogaster | 16.7 | 9.7 | – | – | – | |
Hysterangium | 50.0 | 77.4 | 12.5 | – | – | |
Hymenogaster 2 | – | – | – | – | – | |
Labyrinthomyes | 100.0 | 96.8 | – | – | – | |
Octaviania | – | 3.2 | – | – | – | |
Quadrispora | 83.3 | 83.9 | – | – | – | |
Rossbeevera | 16.7 | – | – | – | – | |
Russulaceae | 100.0 | 87.1 | 50.0 | – | – | |
Scleroderma | – | 6.5 | – | – | – | |
Thaxterogaster | 66.7 | 96.8 | 12.5 | – | 66.7 | |
Unknown spore taxon | – | – | – | 100.0 | – | |
Number of scats examined | 6 | 31 | 8 | 1 | 3 | |
Number of fungal taxa | 17 | 18 | 7 | 2 | 2 | |
% occurrence of fungus | 100 | 100 | 75 |
Total taxa in diets and percentage occurrence of fungus in all scats (where sufficient samples were gathered) are also shown.
Results
Fungi were a major component of the diets of the five mammal species trapped during this study. A total of 24 fungal spore types was recognised in the scats (Table 1 and Fig. 1). We had eight captures of brush-tailed possums (Trichosurus vulpecula), one of eastern barred bandicoot (Perameles gunnii) and three of southern brown bandicoots (Isoodon obesulus) (Table 1). We had six captures of eastern bettongs (Bettongia gaimardi) and 31 captures of long-nosed potoroos (Potorous tridactylus), and every scat from these two species contained fungal spores (Table 1 and Fig. 2).
Ordination of individual mammal diets based on presence/absence of fungal taxa. Brown triangles = southern brown bandicoot, light blue circles = long-nosed potoroo, dark blue squares = eastern bettong and red inverted triangles = brush-tailed possum.
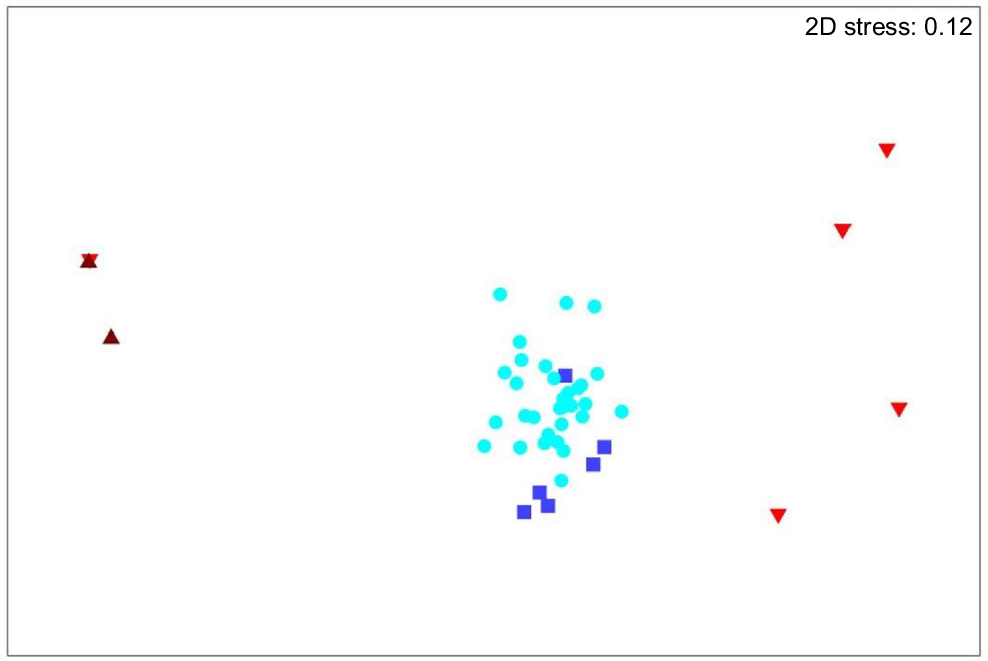
We found significant differences in fungal diet among species (ANOSIM R = 0.698, P = 0.001, Fig. 2). Composition of fungi in the long-nosed potoroo samples was well separated from that in southern brown bandicoots (R = 1.00) and brush-tailed possums (R = 0.97) but not well separated from eastern bettongs (R = 0.29). Fungi composition in eastern bettongs was well separated from that in southern brown bandicoots (R = 1.00) and moderately separated from that in brush-tailed possums (R = 0.59). Fungi composition in brush-tailed possums was moderately separated from that in southern brown bandicoots (R = 0.47).
The single brush-tailed possum sample from the Peter Murrell Reserves is clustered with that of the southern brown bandicoots from the same site (Fig. 2). The other brush-tailed possum samples are from The Lea. Eastern bettong samples are all from The Lea. The long-nosed potoroos are the only mammal for which we had sufficient data to compare between the two study sites. There was no significant difference in fungal consumption between The Lea and the Peter Murrell Reserves (R = 0.08, P = 0.23, Fig. 3).
Discussion
This study builds on existing knowledge about mycophagy in Australia and highlights the ecological importance of conservation of potoroids and other critical weight range mammals. Mycophagy levels among potoroids have been shown to vary between seasons and with the availability of fungi (Taylor 1992; Johnson 1994; Johnson and McIlwee 1997; Tory et al. 1997; Vernes 1999; Robley et al. 2001; Vernes et al. 2015). Our study is a temporal snapshot and does not represent consumption throughout the entire year but many studies of this group have shown these to be regular fungal consumers (Nuske et al. 2017; Elliott et al. 2022a). Our study supports the idea that obligate mycophagists such as our two potoroids share similar fungal dietary components. Our observation of mycophagy among brush-tailed possums, eastern barred bandicoots and southern brown bandicoots supports more robust mycophagy data sets about the diets of these marsupials (see: Nuske et al. 2019; Elliott et al. 2022a, 2023).
Testing viability of spores after transit through the digestive systems was beyond the scope of our study. However, among the more than 40 mammal species that have been studied – including members of Peramelidae, Phalangeridae and Potoroidae – all have been shown to pass viable fungal spores and some have been shown to increase spore germination rates (Elliott et al. 2022a). Although we did not test for evidence of detrimental degradation of spores, no evidence of this was observed.
Accurately estimating spore dispersal potential requires data on digestive transit time and telemetry data on movement. These data were lacking, therefore we could not model dispersal potential in this study. However, for comparison, modeling of swamp wallabies (Wallabia bicolor) has shown that these animals frequently disperse mycorrhizal fungal spores hundreds of metres and possibly as far as 1265 m from the point of consumption (Danks et al. 2020). Dispersal estimates based on home range size indicate that some murid rodents could also move spores hundreds and possibly over 1000 m (Elliott et al. 2020b, 2022b), and bandicoots likely hundreds of metres (Elliott et al. 2022c).
Spores often linger longer than other dietary items in digestive systems and therefore have a longer period of potential dispersal (Danks 2012). Ultimately, spore dispersal potential is dependent upon movement patterns, home range size and gut passage rate. No studies have assessed passage rate of fungal spores through potoroid digestive systems but based on what is known of passage rates of other animals discussed in the aforementioned studies, maximum retention times are likely to be 2 days. Mean home range sizes vary significantly between the two potoroids in this study. Mean home range size is 61 ha for the eastern bettong (Taylor 1993), and 4 ha among males and 1.9 ha among females for long-nosed potoroos (Long 2001). The differences in home range size suggest that bettongs may contribute to longer distance dispersal than potoroos. Another variable not considered in this study is secondary dispersal that occurs when these prey species are ingested by a predator or scavenger. In mainland Australia, dingoes (Canis familiaris) contribute to spore dispersal by eating primary mycophagists (including long-nosed potoroos), with modelling suggesting that dingoes potentially disperse fungal spores over more than 10 km (Elliott et al. 2024). Avian raptors, Tasmanian devils (Sarcophilus harrisii) and quolls (Dasyurus spp.) likely provide – and at one point the Tasmanian tiger (Thylacinus cynocephalus) provided – a similar function of long-distance spore dispersal.
Our data show that possums, bandicoots, bettongs and potoroos consume a diversity of mycorrhizal fungi in Tasmania. We hope this study will stimulate further research into viability, passage rates and dispersal estimation of fungal spores, and provide an additional ecological rationale for the value of conserving Australian mammal communities.
Data availability
All relevant data are presented in the paper but clarification or notes are available upon request.
Declaration of funding
This project was made possible by funding provided to the corresponding author by two Holsworth Wildlife Research Endowment Grants from the Ecological Society of Australia and an In Situ Science Research Excellence Award. The trapping program at Peter Murrell Reserves by the second author was funded by the Tasmanian Government.
Acknowledgements
We are grateful to Carly Lambert for assisting with trapping at The Lea site and the Walch family for allowing us to trap on their private property. Peter J. Jarman also provided assistance with trapping at the Peter Murrell sites. The School of Environmental and Rural Science at the University of New England provided facilities and an International Postgraduate Research Scholarship to the corresponding author.
References
Claridge AW, May TW (1994) Mycophagy among Australian mammals. Australian Journal of Ecology 19(3), 251-275.
| Crossref | Google Scholar |
Danks MA (2012) Gut-retention time in mycophagous mammals: a review and a study of truffle-like fungal spore retention in the swamp wallaby. Fungal Ecology 5(2), 200-210.
| Crossref | Google Scholar |
Danks MA, Simpson N, Elliott TF, Paine CET, Vernes K (2020) Modeling mycorrhizal fungi dispersal by the mycophagous swamp wallaby (Wallabia bicolor). Ecology and Evolution 10(23), 12920-12928.
| Crossref | Google Scholar |
Davies GTO, Kirkpatrick JB, Cameron EZ, Carver S, Johnson CN (2019) Ecosystem engineering by digging mammals: effects on soil fertility and condition in Tasmanian temperate woodland. Royal Society Open Science 6, 180621.
| Crossref | Google Scholar |
Donaldson R, Stoddart M (1994) Detection of hypogeous fungi by Tasmanian bettong (Bettongia gaimardi: Marsupialia; Macropodoidea). Journal of Chemical Ecology 20, 1201-1207.
| Crossref | Google Scholar |
Elliott TF, Trappe JM (2018) A worldwide nomenclature revision of sequestrate Russula species. Fungal Systematics and Evolution 1(1), 229-242.
| Crossref | Google Scholar |
Elliott TF, Jusino MA, Trappe JM, Lepp H, Ballard G-A, Bruhl JJ, Vernes K (2019a) A global review of the ecological significance of symbiotic associations between birds and fungi. Fungal Diversity 98, 161-194.
| Crossref | Google Scholar |
Elliott TF, Bower DS, Vernes K (2019b) Reptilian mycophagy: a global review of mutually beneficial associations between reptiles and macrofungi. Mycosphere 10(1), 776-797.
| Crossref | Google Scholar |
Elliott TF, Nelsen DJ, Karunarathna SC, Stephenson SL (2020a) Entoloma sequestratum, a new species from northern Thailand, and a worldwide key to sequestrate taxa of Entoloma (Entolomataceae). Fungal Systematics and Evolution 6(1), 253-263.
| Crossref | Google Scholar |
Elliott TF, Townley S, Johnstone C, Meek P, Gynther I, Vernes K (2020b) The endangered Hastings River mouse (Pseudomys oralis) as a disperser of ectomycorrhizal fungi in eastern Australia. Mycologia 112(6), 1075-1085.
| Crossref | Google Scholar |
Elliott TF, Truong C, Jackson SM, Zúñiga CL, Trappe JM, Vernes K (2022a) Mammalian mycophagy: a global review of ecosystem interactions between mammals and fungi. Fungal Systematics and Evolution 9(1), 99-159.
| Crossref | Google Scholar |
Elliott TF, Elliott K, Vernes K (2022b) The fungal rat race: mycophagy among rodent communities in eastern Australia. Wildlife Research 50(7), 526-536.
| Crossref | Google Scholar |
Elliott TF, Travouillon KJ, Warburton NM, Danks MA, Vernes K (2022c) New Guinean bandicoots: new insights into diet, dentition and digestive tract morphology and a dietary review of all extant non-Australian Peramelemorphia. Australian Mammalogy 44(2), 266-279.
| Crossref | Google Scholar |
Elliott TF, Rainbird J, Vernes K (2023) Tasmanian bandicoots as fungal dispersers: a comparison in mycophagy between the southern brown bandicoot (Isoodon obesulus) and the eastern barred bandicoot (Perameles gunnii). Australian Mammalogy 46, AM23018.
| Crossref | Google Scholar |
Elliott TF, Paine CET, Ballard G-A, Milne H, Van der Eyk J, Elliott K, Meek P, Bruhl JJ, Vernes K (2024) The dingo (Canis familiaris) as a secondary disperser of mycorrhizal fungal spores. Wildlife Research 51, WR22057.
| Crossref | Google Scholar |
Fleming PA, Anderson H, Prendergast AS, Bretz MR, Valentine LE, Hardy GES (2014) Is the loss of Australian digging mammals contributing to a deterioration in ecosystem function? Mammal Review 44(2), 94-108.
| Crossref | Google Scholar |
Galante TE, Horton TR, Swaney DP (2011) 95% of basidiospores fall within 1 m of the cap: a field-and modeling-based study. Mycologia 103(6), 1175-1183.
| Crossref | Google Scholar |
Garkaklis MJ, Bradley JS, Wooller RD (2004) Digging and soil turnover by a mycophagous marsupial. Journal of Arid Environments 56(3), 569-578.
| Crossref | Google Scholar |
Gasparini B (2014) Cortinarius (Agaricales) revised taxonomy: new species names or combinations. Mycosphere 5(4), 541-544.
| Crossref | Google Scholar |
Geml J, Tulloss RE, Laursen GA, Sazanova NA, Taylor DL (2008) Evidence for strong inter- and intracontinental phylogeographic structure in Amanita muscaria, a wind-dispersed ectomycorrhizal basidiomycete. Molecular Phylogenetics and Evolution 48(2), 694-701.
| Crossref | Google Scholar |
Johnson CN (1994) Nutritional ecology of a mycophagous marsupial in relation to production of hypogeous fungi. Ecology 75(7), 2015-2021.
| Crossref | Google Scholar |
Johnson CN (1996) Interactions between mammals and ectomycorrhizal fungi. Trends in Ecology & Evolution 11(12), 503-507.
| Crossref | Google Scholar |
Johnson CN, McIlwee AP (1997) Ecology of the northern bettong, Bettongia tropica, a tropical mycophagist. Wildlife Research 24(5), 549-559.
| Crossref | Google Scholar |
Jonsson L, Dahlberg A, Nilsson M-C, Kårén O, Zackrisson O (1999) Continuity of ectomycorrhizal fungi in self-regenerating boreal Pinus sylvestris forests studied by comparing mycobiont diversity on seedlings and mature trees. New Phytologist 142(1), 151-162.
| Crossref | Google Scholar |
Li D-W (2005) Release and dispersal of basidiospores from Amanita muscaria var. alba and their infiltration into a residence. Mycological Research 109(11), 1235-1242.
| Crossref | Google Scholar |
Long KI (2001) Spatio-temporal interactions among male and female long-nosed potoroos, Potorous tridactylus (Marsupialia: Macropodoidea): mating system implications. Australian Journal of Zoology 49(1), 17-26.
| Crossref | Google Scholar |
Nest C, Elliott TF, Cooper T, Vernes K (2023) Seasonal consumption of mycorrhizal fungi by a marsupial-dominated mammal community. Fungal Ecology 64, 101247.
| Crossref | Google Scholar |
Newell J (2009) The role of the reintroduction of greater bilbies (Macrotis lagotis) and burrowing bettongs (Bettongia lesueur) in the ecological restoration of an arid ecosystem: foraging diggings, diet, and soil seed banks. PhD dissertation, University of Adelaide, Adelaide, South Australia, Australia.
Nouhra E, Kuhar F, Truong C, Pastor N, Crespo E, Mujic A, Caiafa MV, Smith ME (2021) Thaxterogaster revisited: a phylogenetic and taxonomic overview of sequestrate Cortinarius from Patagonia. Mycologia 113(5), 1022-1055.
| Crossref | Google Scholar |
Nuske SJ, Vernes K, May TW, Claridge AW, Congdon BC, Krockenberger A, Abell SE (2017) Redundancy among mammalian fungal dispersers and the importance of declining specialists. Fungal Ecology 27, 1-13.
| Crossref | Google Scholar |
Nuske SJ, Anslan S, Tedersoo L, Congdon BC, Abell SE (2019) Ectomycorrhizal fungal communities are dominated by mammalian dispersed truffle-like taxa in north-east Australian woodlands. Mycorrhiza 29, 181-193.
| Crossref | Google Scholar |
Palmer BJ, Valentine LE, Page M, Hobbs RJ (2020) Translocations of digging mammals and their potential for ecosystem restoration: a review of goals and monitoring programmes. Mammal Review 50(4), 382-398.
| Crossref | Google Scholar |
Pastor N, Chiapella J, Kuhar F, Mujic AB, Crespo EM, Nouhra ER (2019) Unveiling new sequestrate Cortinarius species from northern Patagonian Nothofagaceae forests based on molecular and morphological data. Mycologia 111(1), 103-117.
| Crossref | Google Scholar |
Robley AJ, Short J, Bradley S (2001) Dietary overlap between the burrowing bettong (Bettongia lesueur) and the European rabbit (Oryctolagus cuniculus) in semi-arid coastal Western Australia. Wildlife Research 28(4), 341-349.
| Crossref | Google Scholar |
Short J (1998) The extinction of rat-kangaroos (Marsupialia: Potoroidae) in New South Wales, Australia. Biological Conservation 86(3), 365-377.
| Crossref | Google Scholar |
Short J, Smith A (1994) Mammal decline and recovery in Australia. Journal of Mammalogy 75(2), 288-297.
| Crossref | Google Scholar |
Stephens RB, Trowbridge AM, Ouimette AP, Knighton WB, Hobbie EA, Stoy PC, Rowe RJ (2020) Signaling from below: rodents select for deeper fruiting truffles with stronger volatile emissions. Ecology 101(3), e02964.
| Crossref | Google Scholar |
Taylor RJ (1992) Seasonal changes in the diet of the Tasmanian bettong (Bettongia gaimardi), a mycophagous marsupial. Journal of Mammalogy 73(2), 408-414.
| Crossref | Google Scholar |
Taylor RJ (1993) Home range, nest use and activity of the Tasmanian bettong, Bettongia gaimardi. Wildlife Research 20(1), 87-95.
| Crossref | Google Scholar |
Tory MK, May TW, Keane PJ, Bennett AF (1997) Mycophagy in small mammals: a comparison of the occurrence and diversity of hypogeal fungi in the diet of the long-nosed potoroo Potorous tridactylus and the bush rat Rattus fuscipes from southwestern Victoria, Australia. Australian Journal of Ecology 22(4), 460-470.
| Crossref | Google Scholar |
Vernes K, Cooper T, Green S (2015) Seasonal fungal diets of small mammals in an Australian temperate forest ecosystem. Fungal Ecology 18, 107-114.
| Crossref | Google Scholar |
Vernes K, Elliott TF, Jackson SM (2021) 150 years of mammal extinction and invasion at Koonchera Dune in the Lake Eyre Basin of South Australia. Biological Invasions 23, 593-610.
| Crossref | Google Scholar |