Cross-pollination and pollen storage to assist conservation of Metrosideros bartlettii (Myrtaceae), a critically endangered tree from Aotearoa New Zealand
Karin van der Walt


A Ōtari Native Botanic Garden and Wilton’s Bush Reserve, Wellington, New Zealand.
B Massey University, Palmerston North, New Zealand.
C Victoria University of Wellington, Wellington, New Zealand.
D Museum of New Zealand Te Papa Tongarewa, Wellington, New Zealand.
Pacific Conservation Biology 29(2) 141-152 https://doi.org/10.1071/PC21054
Submitted: 13 August 2021 Accepted: 2 February 2022 Published: 1 March 2022
© 2023 The Author(s) (or their employer(s)). Published by CSIRO Publishing. This is an open access article distributed under the Creative Commons Attribution-NonCommercial-NoDerivatives 4.0 International License (CC BY-NC-ND)
Abstract
Context: Metrosideros bartlettii is one of the most threatened trees in New Zealand and with less than 14 individuals spread across three populations, the species is at high risk of extinction. Despite reproductive failure being identified as one of the factors contributing to population decline, little is known about its pollination biology.
Aim: The current study aimed to gain knowledge of the reproductive biology of M. bartlettii by using trees in cultivation of which origin is known.
Methods: We tested the effect of self-pollination, cross-pollination and hybridisation treatments on reproductive output. In addition, the viability of fresh pollen was determined for each tree and the impact of desiccation and storage temperature (5°C, −18°C and −196°C) on pollen viability assessed.
Key results: Metrosideros bartlettii was found to be highly self-incompatible with seed formed via autonomous self-pollination having low viability. Hybridisation with Metrosideros excelsa, another native species commonly found in cultivation, produced viable seeds, but seedlings failed to survive. Pollen viability differed significantly among trees, and pollen stored at −18°C and −196°C retained viability after 6 months.
Conclusion: Metrosideros bartlettii is self-incompatible and cross-pollination is required to increase seed production and supplement wild populations.
Implications: Trees in cultivation provide a valuable tool for the conservation of M. bartlettii. However, cross-pollination is essential to prevent hybridisation and ensure genetically robust seed. Long-term storage of pollen at the temperatures determined here will help to overcome challenges in cross-pollination of trees that are spatially and geographically isolated.
Keywords: botanic garden, breeding system, cryopreservation, extinction, hybridisation, integrated conservation, liquid nitrogen, long-term storage, myrtle rust, threatened.
Introduction
Worldwide, more than 26% of tree taxa are threatened with extinction due to forest clearing for agriculture, logging, climate change and the global spread of pathogens (Sharrock 2020; GTA 2021). Similar trends have been recorded for New Zealand (NZ) where half of the native vascular plants are at risk of extinction (de Lange et al. 2018). Large-scale deforestation, invasive pest animals, and weeds are the main threats to native trees in NZ. More recently, impacts from fungal pathogens such as myrtle rust (Austropuccinia psidii), resulted in all 29 Myrtaceae species classified as of conservation concern (de Lange et al. 2018). This family includes the genus Metrosideros, which is mostly found in the Pacific region and includes several emblematic and culturally important species for NZ. One of these species is the critically endangered Metrosideros bartlettii (Bartlett’s rātā, rātā moehau) (de Lange 2014). Despite its 30 m height, this endemic tree was recorded for the first time in 1975, with a second locality confirmed in 1984 (Dawson 1985). It is unlikely that M. bartlettii was ever common, and extensive surveys conducted in the 1990s found only 34 individuals in three populations located in the far north of NZ (Fig. 1). By 2016, 14 individuals in three localities remained in the wild (de Lange 2014). Although the biggest historical decline of M. bartlettii was due to land use changes following settlement, more recent reasons for population decline include reproductive failure and devastating effects of the introduced brushtail possums (Trichosurus vulpecula) on buds, flowers and young shoots (de Lange 2014). Metrosideros bartlettii has a high probability of extinction due to genetic, demographic, and environmental stochasticities, and urgent conservation measures are needed to increase the population size and genetic diversity (Melesse 2017). Unfortunately, despite flowering prolifically, fruit/seed set is low, and seedlings are rarely found in the wild (Drummond et al. 2000). Therefore, conservation of M. bartlettii requires urgent research focusing on its reproductive biology and seed ecology to understand the lack of recruitment in wild populations.
![]() |
Several threatened plant species are widely cultivated in botanic gardens and nurseries globally, often outnumbering wild populations (Asmussen-Lange et al. 2011). This is also the case for M. bartlettii with several trees present in botanic and private gardens throughout NZ. However, the suitability of ex situ material for recovery of threatened species has been limited in some cases (Abeli et al. 2020; Ensslin and Godefroid 2020) due to low genetic diversity (Christe et al. 2014), low or no seed production (Cochrane 2004) or negative consequences due to adaptations to ex situ conditions (Horiuchi et al. 2020). Spontaneous hybridisation between morphologically similar congeneric species can also occur in ex situ collections, further eroding the suitability of progeny for conservation (Volis 2017; Lozada-Gobilard et al. 2020). Despite this, re-establishment or de-extinction of plant species has been achieved where founder populations were limited (Abeli et al. 2020; Hitchcock et al. 2020) using methods such as micro-propagation and cross-pollination (Cibrian-Jaramillo et al. 2013; Hyvärinen 2020).
Cross-pollination is a common conservation strategy for endangered plants in botanic gardens and private collections and has been successfully applied in cycads (Iglesias-Andreu et al. 2017), orchids (Del Vecchio et al. 2019), trees (Chen et al. 2016) and herbaceous species (Sudarmono et al. 2016). Controlled cross-pollination has also been recommended as a tool to obtain genetically diverse seeds of Metrosideros boninensis, an endangered tree endemic to the Bonin Islands in the western North Pacific Ocean (Kaneko et al. 2008). However, for cross-pollination to be successful, knowledge of the species’ reproductive biology, pollen viability and breeding system is essential particularly for species with small populations or limited founders (Chen et al. 2016). Where cross-pollination is conducted as part of an overall conservation plan, it is important that techniques are developed to store and test pollen viability to maximise chances of successful pollination and seed production (Towill 2004; Wheeler and McComb 2006). In vitro germination of pollen can provide an effective method for viability assessment, although the germination media and incubation time need to be optimised for each species (Page et al. 2006; Cruzatty et al. 2020). The ability to extend pollen longevity through short- or long-term storage can be used to overcome temporal and geographical separation of flowering plants (Page et al. 2006; Nadarajan et al. 2018) and has been instrumental in the conservation of threatened species such as Hymenoxys acaulis var. glabra, Brighamia insignis and Pittosporum halophilum (DeMauro 1993; Towill 2004). Although the creation of pollen banks is widely used in crop breeding, it is an underutilised tool for plant conservation and there is an urgent need for the development of species-specific pollen collection and storage protocols (Kormuťák et al. 2019).
The current study aimed to gain knowledge of the reproductive biology of M. bartlettii by using trees in cultivation for which the source population is known. The specific objectives of the study were to: (1) gain insight into the breeding system and reproductive success of selected individual trees in cultivation; (2) compare the effect of pollination treatments on seed characteristics, seed germination and seedling establishment; (3) compare pollen viability between trees and establish short-, medium- and long-term pollen storage protocols; and (4) advise feasible conservation actions for M. bartlettii which can be applied to plants in cultivation and in the wild.
Materials and methods
Study trees
All the trees used in this study are in cultivation, both in botanic and private gardens, in Wellington and Auckland, New Zealand. The trees were selected based on presence of flowers, known source population, accessibility, and landowner permission. A total of six individual trees, representing two wild populations (Kohuronaki and Radar Bush), were used in this study (Table 1). This included two trees at Ōtari Native Botanic Garden (ŌOI and ŌAL), one tree at Percy’s Reserve in Lower Hutt (PLH), two trees in private gardens in Wellington (TAW and WLH) and one tree at the University of Auckland which served as a pollen donor in 2017 (TUA).
![]() |
Pollination studies were conducted during the flowering period for M. bartlettii in November/December of 2017 and 2019, with capsules, seed set and seed viability assessed in March/April of 2018 and 2020.
Flower development
Ten flower clusters containing flowers in bud were randomly tagged among the two trees at Ōtari Native Botanic Garden (Ōtari). Flower development from bud to senescence was monitored daily and changes in the position of the style and stamens were recorded. Flowers were considered mature when all stamens were shedding pollen and the style was fully extended.
Breeding system
Pollination experiments were applied to randomly selected inflorescences on four trees (Table 1), these experiments included autonomous self-pollination (AS), emasculation and bagging to test for apomixis (APO), hand self-pollination (HS), natural pollination (N), cross-pollination between individuals from different source populations (×DS), cross-pollination between individuals from the same source population (×SS) and hybridisation with Metrosideros excelsa (HY). These treatments tested for capacity to self-pollinate, self-compatibility, interspecific compatibility (hybridisation) and apomixis (asexual reproduction in which seeds are produced from unfertilised ovules). Natural pollination, in which no flower manipulation was applied, represented the control. For pollination experiments, three to six flowering branches were randomly assigned to a treatment and marked with flagging tape. At each branch, floral buds were counted and then covered with a fine mesh bag to prevent introduction of any foreign pollen (Neal and Anderson 2004). Before anthesis, flowers assigned to hand pollination (e.g. HS, ×DS, ×SS and HY) and apomixis treatments were emasculated using tweezers and bagged again until the style had straightened indicating that the flower had reached maturity (Fig. 2). Flowers assigned to test for apomixis remained bagged until floral senescence. In other treatments, a clean paint brush was used to transfer pollen between mature flowers on the same tree (self-pollination), between flowers on different trees of the same species (cross-pollination) or between flowers of different species (hybridisation) (Table 2). The process was repeated 3–5 days later. Flowers remained bagged until the stigma wilted, which was approximately 3 weeks after pollination. Capsules were collected at maturity (between March and April). The outcome of each treatment was assessed by evaluating fruit set (ratio of capsules to pollinated flowers) and capsule size (length × width). Capsule size was also measured for 15 capsules produced outside pollination treatments. The viability of the pollen used in each treatment was verified as per methodology described below.
![]() |
Seed viability, germination potential and seedling development
The percentage of viable seed per treatment was determined by germinating 200 randomly selected seeds. In cases where no germination was observed, remaining seed from the treatment was scanned for seeds with a developed embryo using a stereo microscope (Fig. 3). If seeds containing an embryo (filled) were found, these were used to assess germination potential and seedling establishment.
Seeds were germinated in 90 mm Petri dishes containing 7% water agar using an incubator set at 15/25°C with a 16 h dark/8 h light cycle. To limit infection by fungi or bacteria, seeds were surface sterilised by washing in 5 g L−1 sodium dichloroisocyanurate (NaDCC) for 20 min followed by three rinses in sterile distilled water. Radicle protrusion of at least 2 mm was the criterion for germination, with seedling establishment classified as the development of cotyledons. Germination was scored three times a week for 6 weeks, which is the maximum time needed for seed germination. Non-germinated seeds were inspected under a stereo microscope to determine if they contain an embryo. To assess seedling survival and development, germinated seeds were transferred to a Petri dish containing soil collected from the forest at Ōtari and returned to the incubator. After 6 weeks in soil, seedling size was visually compared and then seedlings planted in pots containing commercial seed raising mix and transferred to the nursery at Ōtari.
Self-incompatibility
The index of self-incompatibility (ISI) was calculated based on Schmidt-Adam et al. (1999) and Koelling et al. (2011). Individuals with an ISI of 0.2 or less were considered self-incompatible:
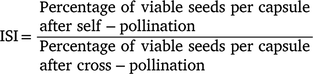
For ISI calculation only AS, HS and ×DS treatments were considered.
Pollen viability, desiccation and storage
Fresh pollen viability was assessed for four trees (ŌOI, PLH, TAW and WLH) in November 2019 and for two trees (ŌOI and PLH) in November 2020 (Table 1). Pollen collected from ŌOI in November 2020 was used to investigate the effect of desiccation and temperature on short- and medium-term storage of pollen. A clean paint brush was used to collect pollen from the anthers under a stereo microscope. Pollen viability was assessed through in vitro germination on semi-solid Brewbaker and Kwack pollen medium (Brewbaker and Kwack 1963) consisting of 300 mg L−1 Ca(NO3)2.4H2O (hydrated calcium nitrate), 200 ppm MgSO4.7H2O (hydrated magnesium sulfate), 150 ppm boric acid, 100 ppm KNO3 (potassium nitrate), supplemented with 20% sucrose. Pollen was considered germinated when the pollen tube length was greater than the diameter of the grain. To screen for desiccation sensitivity, freshly collected pollen was desiccated at 5°C for 3 days over a solution of lithium chloride (735 g L−1) generating a relative humidity (RH) of ∼15%. The impact of desiccation and storage temperature was assessed by storing non-desiccated and desiccated pollen at 20°C (room temperature), 5°C, −18°C (freezer) and −196°C (liquid nitrogen). Frozen pollen was thawed at room temperature for 2 h before slow rehydration in a high humidity chamber for 4 h at 25°C. Pollen was then germinated in vitro for 72 h at 25°C to assess its viability. All treatments consisted of five replicates containing a minimum of 100 pollen grains.
Statistical analysis
Differences in seed set, capsule size and seed viability between treatments, as well as variables associated with pollen viability between trees and across storage treatments, were analysed using a one-way ANOVA followed by pairwise comparisons using Fisher’s l.s.d. (data normally distributed) or Kruskal Wallis test (data not normally distributed). A general linear model (GLM) was used to analyse the pollen viability affected by three variables (time in storage, desiccation, and temperature) and their interactions. A two-way ANOVA was used to investigate the relationship between desiccation and storage temperature on pollen viability. Arcsine square root transformation was used to normalise where needed. Results are presented as the mean ± one s.d. All statistical analysis was conducted using XLStat Software ver. 1.3 (2021) and SAS/STAT ver. 14.2.
Results
Flower and fruit development
The flowering period for M. bartlettii in cultivation lasted approximately 3 weeks. In the 3 years of observations (2017, 2019 and 2020), the trees flowered from mid-November until the first week of December. Trees across Wellington were slightly out of sync; those in private gardens (TAW and WLH) were in peak flower between 19 and 29 November, while the trees at Ōtari (ŌOI and ŌAL) were lagging 7–10 days behind, with flowers fully open from 29 November until 10 December. Flower development from bud to the start of pollen shed was completed in 5 days (Fig. 4). The style emerged from the flower bud on Day 1, approximately 12–24 h before the anthers. At the end of Day 2, the style and anthers were all erect, but pollen shed was only initiated on Day 4. Flowers wilted approximately 7 days after pollen shedding was first recorded.
![]() |
The complete reproductive event, from buds to seed dispersal, took 5 months. Initial capsule development was visible 4 weeks after pollination (mid-December) and mature capsules split open to release seed late-March or early-April.
Despite using more than 1100 flowers in pollination treatments on the TAW tree, fruit set was only 2%. This contrasted with WLH in which all treatments resulted in similar fruit set (Fig. 5a). In 2020, ŌOI produced capsules on all treatments except by N, although capsules were also formed outside of pollination treatments (Fig. 5b). However, in 2018, the same tree produced capsules on ×DS exclusively (Fig. 5c). Pollination treatment had a significant impact on capsule size (P < 0.001) with the biggest capsules (17.2 ± 4.2 mm) obtained by HY while the smallest capsules were by AS treatments (10.8 ± 2.8 mm) (Fig. 5d). APO treatments did not produce any capsules in 2018.
Self-incompatibility
Metrosideros bartlettii was found to be highly self-incompatible with only one tree (WLH) producing viable seed from self-pollination treatments, but viability of these seeds barely reached 1% (Table 3). The ISI score for this tree was 0.06.
![]() |
Seed viability, germination potential and seedling development
Overall seed viability was low with the highest viability (21.5 ± 11.9%) observed in seeds produced by ×DS treatments (Table 3, ŌOI). Of the 43 flowers used for HY in ŌAL, 11.6% produced capsules although seed viability was very low (<1%) despite the presence of viable pollen on M. excelsa (data not included). Capsules from ×SS treatments did not contain any viable seed.
Mean germination of filled seeds was highly variable ranging from 20.5 ± 6.3% for seeds from randomly selected capsules (those outside of the pollination treatments) to 93.1 ± 11.4% in ×DS treatments (Fig. 6a). All seedlings transferred to soil survived (Fig. 6b) but after 6 weeks in soil, seedlings from randomly selected capsules were smaller than those from ×DS treatments (Fig. 6c). Seedlings from ×DS treatments established successfully in nursery conditions (Fig. 6d) but those from randomly selected capsules failed to survive in nursery conditions. Although germination rate was similar across treatments, seedlings from the HY treatments failed to survive for longer than 4 weeks in vitro compared to high survival from other treatments.
Pollen viability, desiccation and storage
Pollen viability differed significantly between individual trees with the highest viability recorded in PLH (78.4 ± 7.2%) and WLH (74 ± 8.6%) and lowest viability associated with TAW (50.6 ± 28.4%). Viability also differed significantly between years for the same tree with higher viability associated with pollen collected in 2019 for both ŌOI (P = 0.009; 58.9 ± 7.3%) and PLH (P = 0.038; 78.4 ± 7.2%) compared to 2020 when viability was 37.8 ± 7.5% and 67.7 ± 6.4%, respectively.
Desiccating pollen to ∼15% equilibrated RH did not significantly impact viability (P = 0.848) but pollen stored at room temperature (20°C) lost all viability within 7 days irrespective of desiccation treatment (Fig. 7a). No viability loss was recorded in pollen from ŌOI, which was desiccated and stored for 6 months at −18°C (42.2 ± 2.7%) and −196°C (43.6 ± 4.0%) (Fig. 7b) compared to very low viability for pollen stored at 5°C (Fig. 7c).
Discussion
This study has demonstrated that M. bartlettii is a highly self-incompatible tree where the production of viable seeds is significantly increased by cross-pollination with pollen from trees from a different source population. This finding is not unexpected as self-incompatibility is common in Myrtaceae and it has been previously reported in other Metrosideros species in NZ and overseas (Carpenter 1976; Schmidt-Adam et al. 1999; Hanna et al. 2013). In all known examples, however, a mosaic of self-compatibility and partially self-incompatible individuals occur within the same population. The differences in self-compatibility observed here between the trees TAW and WLH may be an indication that this mosaic also occurs in M. bartlettii. In the case of Metrosideros collina, a species from Hawai’i, differences in self-compatibility are also linked with differences in flower colour (Carpenter 1976). Such flower colour polymorphism has not been reported in M. bartlettii and we did not observe any other less noticeable morphological differences between trees, but our study included only a small number of individuals and they may not reflect the morphological diversity occurring in natural populations. Future studies should investigate morphological diversity of each of the 14 remnant trees and their mating system as a bias towards self-incompatibility, along with the lack of suitable pollinators to move pollen between compatible trees, may be responsible for the poor recruitment reported for this species (Drummond et al. 2000).
Fertility in Metrosideros species is generally very low and this appears to be genetically determined rather than caused by pollinator or resource limitation (Schmidt-Adam et al. 1999). It is likely that low fertility, along with self-incompatibility, may help to promote heterozygosity within populations by favouring the development of ovules fertilised by only a distinct genotype. It is known that outcrossing is paramount to the reproductive success in M. excelsa, with progeny originating from self-pollination unlikely to survive in natural populations (Schmidt-Adam et al. 2009). As with other Myrtaceae species, flower development in M. bartlettii is characterised by a temporal separation of stigma receptivity and pollen shed (i.e. dichogamy) a well-known mechanism to prevent self-pollination (Beardsell et al. 1993). In our study, most of the pollination treatments applied to M. bartlettii triggered capsule formation, but seed viability was greater than 15% only in those treatments that used pollen obtained from trees at different source populations (Table 3). This outcome was however not consistent as the ŌOI tree failed to produce viable seeds in the second flowering season (Table 3) even though the pollen donor was from a different source population. The pollen donors for ŌOI were, however, different trees in 2017 (TUA) and 2020 (WLH). Meanwhile, the tree TAW also failed to produce viable seeds after using pollen from different source population (Table 3). Since none of these trees produce viable seeds by any other treatment in that season (2020), it is possible that resource limitation or environmental conditions, rather than pollination treatment, affected the production of viable seeds. These hypotheses are supported by the results from ×DS treatments on ŌOI in 2018, which produced the highest percentage viable seed (21.5%) in this study (Table 3). An alternative explanation is that some of these trees are genetically too similar for effective pollination and future genetic studies will be useful to determine this. Finally, a somewhat unexpected result was that M. bartlettii also readily produced capsules outside of ×DS treatments (i.e. N and AS treatments, as well as outside of treatments), but the seed viability associated with these capsules was very low (<1.6%) and seedlings failed to survive under nursery conditions. It is likely these seeds have originated by hybridisation. We have experimentally confirmed hybridisation between M. bartlettii and M. excelsa results in capsule formation, but these seeds have very low viability (Table 3). Birds and insects (honeybees, bumble bees, native solitary bees and flies) were commonly observed feeding on M. bartlettii and these may facilitate transport of pollen from other species of Metrosideros flowering within the gardens/neighbourhood. An alternative explanation is that these capsules were formed via self-pollination. Similar scenarios have been reported in many other mass flowering Myrtaceae species in which wind and rain may be responsible for self-pollination, but the production of viable seed is low or unlikely (Griffin 1980; Beardsell et al. 1993).
Our study has confirmed cross-pollination is the only pollination method to obtain viable seeds of M. bartlettii to supplement wild populations. Hand pollination has been successfully applied to critically endangered tree species such as Magnolia sinica, a pollinator dependant species in China (Chen et al. 2016) and Ocotea catharinensis in Brazil (Montagna et al. 2018). For the critically endangered Morus boninensis, controlled crossing between genotypes is now the only way to obtain pure seedlings due to extensive hybridisation with the invasive species Morus accidosa (Tani et al. 2006). Spontaneous hybridisation in ex situ collections can result in maladaptive changes in life-history traits, fitness, and genetic diversity, rendering plant material unsuitable for restoration (Lozada-Gobilard et al. 2020). Genetic studies have confirmed hybridisation between Metrosideros species in New Zealand (Gardner et al. 2004; Melesse 2017) and as mentioned earlier, our study found that hybridisation with co-flowering M. excelsa produced viable seed. However, seedlings of hybrid origin were less vigorous than those seedlings generated via ×DS treatments and failed to survive beyond 4 weeks. It should be noted that the hybridisation treatment was limited to a single tree. It is recommended that future studies investigate fruit set, seed viability and seedling vigour from hybridisation using a larger sample size and including Metrosideros robusta another co-flowering species. This will provide fundamental information for the collection of seed from trees in cultivation and their potential use to reinforce extant wild populations.
The self-incompatible breeding system, isolation of individuals and out of sync flowering associated with M. bartlettii makes the storage of pollen an essential part of its overall conservation strategy. Short- and long-term storage of pollen allows for cross pollination between trees that flower at different times or grow in different areas (Cruzatty et al. 2020). Studies investigating pollen viability and long-term storage for Myrtaceae have mostly focused on species of commercial interest such as various Eucalyptus species (e.g. Wheeler and McComb 2006) and Kunzea pomifera (Page et al. 2006). Information for Metrosideros pollen is limited to two studies, both on M. excelsa in cultivation (Schmidt-Adam et al. 1999; Nadarajan et al. 2021). Our study addressed this research need and our findings will guide handling of M. bartlettii pollen during pollination and inform short- and long-term storage. For instance, it was found that the viability of pollen kept at room temperature rapidly declines while it remains consistent for up to 4 weeks when stored in the fridge (5°C) but then it rapidly declines. Desiccation and freezing of pollen are essential to maintain viability for longer than 4 weeks, with pollen stored at −196°C (liquid nitrogen) producing the best results. Our next step towards developing this technique is to verify moisture content of fresh pollen and measure pollen vigour after long-term storage by evaluating its ability to produce viable seeds. Another unexpected finding was detecting significant variation in fresh pollen viability between trees and years, ranging from 37 to 78%. Variation in pollen viability across individuals of the same species is not unique to M. bartlettii and it has been detected in Castanea sativa and Picea abies (Nikkanen et al. 2000; Beyhan and Serdar 2008) where there was up to a 10-fold difference in pollen viability between genotypes. These studies suggest variation in pollen viability among trees indicates a potential for male gametophyte competition, a strategy to maintain genetic diversity. However, this also means some trees are better pollen donors than others and this should be considered when conducting controlled pollination of M. bartlettii.
Conclusion and recommendations for conservation
The continued decline, extremely small population size, reproductive failure and threats from myrtle rust mean that without urgent conservation interventions, extinction of M. bartlettii is highly likely (Drummond et al. 2000; Melesse 2017). We have shown that the breeding system of M. bartlettii favours cross-pollination, supporting results from a previous genetic study that showed inbreeding in M. bartlettii is very low (Melesse 2017). Although cultivated specimens can provide material to support in situ recovery, careful consideration should be given to provenance, genetic diversity, and hybridisation risks. The lack of genetic diversity associated with the current stock of M. bartlettii in cultivation may limit contribution to the overall conservation of the species. However, in this study we identified key individual trees from different source populations that can be outcrossed to increase seed viability and offspring fitness, and therefore can make a significant contribution towards decreasing the risk of extinction. We have also outlined pollen handling and storage protocols that will make it possible to overcome barriers caused by different flowering times or large distance between trees.
Recommendations for the conservation of M. bartlettii:
-
Develop a pollination network that utilises pollen banks to optimise reproductive output through cross-pollination of trees of known provenance/sources.
-
Secure germplasm of the remaining genotypes through living collections and/or cryopreservation.
-
Create a seed bank for seed obtained from wild populations and cross-pollination of trees in cultivation.
-
Assist Ngāti Kuri to develop a reintroduction/enhancement program to increase population sizes in the wild.
Data availability
The data that support this study will be shared upon reasonable request to the corresponding author.
Conflicts of interest
The authors declare no conflicts of interest.
Declaration of funding
This research did not receive any specific funding.
Acknowledgements
Ngā mihi ki a koutou o Ngāti Kuri, ngā kaitiaki o Rata Moehau. We acknowledge and thank Ngāti Kuri for their guidance and support of our work to assist with the conservation their incredible taonga (treasure). The authors thank the contributions made by Jeremy Rolfe (Department of Conservation), Revel Drummond (Plant and Food Research Limited) and Gary Houliston (Manaaki Whenua – Landcare Research). Special thanks to Peter de Lange (Unitec Institute of Technology) for sharing knowledge on many aspects of M. bartlettii. Bruce Burns (The University of Auckland) and Emma Bodley (Auckland Botanic Garden) for collecting flowers for pollination studies in 2017. Access to trees were provided by Percy’s Reserve (Jonathan Frericks – Lower Hutt City Council), Kris Ericksen (private garden) as well as Bill Fox and Angela Silbery-Fox (private garden). Zhao He from Massey University aided with statistical analysis.
References
Abeli, T, Dalrymple, S, Godefroid, S, Mondoni, A, Müller, JV, Rossi, G, and Orsenigo, S (2020). Ex situ collections and their potential for the restoration of extinct plants. Conservation Biology 34, 303–313.| Ex situ collections and their potential for the restoration of extinct plants.Crossref | GoogleScholarGoogle Scholar | 31329316PubMed |
Asmussen-Lange, CB, Maunder, M, and Fay, MF (2011). Conservation genetics of the critically endangered Round Island bottle palm, Hyophorbe lagenicaulis (Arecaceae): can cultivated stocks supplement a residual wild population? Botanical Journal of the Linnean Society 167, 301–310.
| Conservation genetics of the critically endangered Round Island bottle palm, Hyophorbe lagenicaulis (Arecaceae): can cultivated stocks supplement a residual wild population?Crossref | GoogleScholarGoogle Scholar |
Beardsell, DV, Knox, RB, and Williams, EG (1993). Breeding system and reproductive success of Thryptomene calycina (Myrtaceae). Australian Journal of Botany 41, 333–353.
| Breeding system and reproductive success of Thryptomene calycina (Myrtaceae).Crossref | GoogleScholarGoogle Scholar |
Beyhan, N, and Serdar, U (2008). Assessment of pollen viability and germinability in some European chestnut genotypes (Castanea sativa L.). Horticultural Science 35, 171–178.
| Assessment of pollen viability and germinability in some European chestnut genotypes (Castanea sativa L.).Crossref | GoogleScholarGoogle Scholar |
Brewbaker, JL, and Kwack, BH (1963). The essential role of calcium ion in pollen germination and pollen tube growth. American Journal of Botany 50, 859–865.
| The essential role of calcium ion in pollen germination and pollen tube growth.Crossref | GoogleScholarGoogle Scholar |
Carpenter, FL (1976). Plant-pollinator interactions in Hawaii: pollination energetics of Metrosideros collina (Myrtaceae). Ecology 57, 1125–1144.
| Plant-pollinator interactions in Hawaii: pollination energetics of Metrosideros collina (Myrtaceae).Crossref | GoogleScholarGoogle Scholar |
Chen, Y, Chen, G, Yang, J, and Sun, W (2016). Reproductive biology of Magnolia sinica (Magnoliaecea), a threatened species with extremely small populations in Yunnan, China. Plant Diversity 38, 253–258.
| Reproductive biology of Magnolia sinica (Magnoliaecea), a threatened species with extremely small populations in Yunnan, China.Crossref | GoogleScholarGoogle Scholar | 30159474PubMed |
Christe, C, Kozlowski, G, Frey, D, Fazan, L, Bétrisey, S, Pirintsos, S, Gratzfeld, J, and Naciri, Y (2014). Do living ex situ collections capture the genetic variation of wild populations? A molecular analysis of two relict tree species, Zelkova abelica and Zelkova carpinifolia. Biodiversity and Conservation 23, 2945–2959.
| Do living ex situ collections capture the genetic variation of wild populations? A molecular analysis of two relict tree species, Zelkova abelica and Zelkova carpinifolia.Crossref | GoogleScholarGoogle Scholar |
Cibrian-Jaramillo, A, Hird, A, Oleas, N, Ma, H, Meerow, AW, Francisco-Ortega, J, and Griffith, MP (2013). What is the conservation value of a plant in a botanic garden? Using indicators to improve management of ex situ collections. The Botanical Review 79, 559–577.
| What is the conservation value of a plant in a botanic garden? Using indicators to improve management of ex situ collections.Crossref | GoogleScholarGoogle Scholar |
Cochrane A (2004) Western Australia’s ex situ program for threatened species: a model integrated strategy for conservation. In ‘Ex situ plant conservation: Supporting Species Survival in the Wild’. (Eds EO Guerrant, K Havens, M Maunder) pp. 40–66. (Island Press: Washington, DC)
Cruzatty, LCG, Droppelmann, F, and Izaguirre-Mayoral, ML (2020). New protocol for storage of viable pollen of Lapageria rosea (Philesiaceae), an endangered plant species endemic to temperate forests of Chile. Plant Species Biology 35, 332–337.
| New protocol for storage of viable pollen of Lapageria rosea (Philesiaceae), an endangered plant species endemic to temperate forests of Chile.Crossref | GoogleScholarGoogle Scholar |
Dawson, JW (1985). Metrosideros bartlettii (Myrtaceae) a new species from North Cape, New Zealand. New Zealand Journal of Botany 23, 607–610.
| Metrosideros bartlettii (Myrtaceae) a new species from North Cape, New Zealand.Crossref | GoogleScholarGoogle Scholar |
de Lange P (2014) ‘Metrosideros bartlettii. The IUCN Red List of Threatened Species 2014. Vol. 2020.’ (International Union for the Conservation of Nature)
de Lange PJ, Rolfe JR, Barkla JW, Courtney SP, Champion PD, Perrie LR, Beadel SM, Ford KA, Breitwieser I, Schonberger I, Hindmarsh-Walls R, Heenan PB, Landley K (2018) ‘Conservation status of New Zealand indigenous vascular plants, 2017.’ (Department of Conservation: Wellington)
Del Vecchio, S, Pierce, S, Fantinato, E, and Buffa, G (2019). Increasing the germination percentage of a declining native orchid (Himantoglossum adriaticum) by pollen transfer and outbreeding between populations. Plant Biology 21, 935–941.
| Increasing the germination percentage of a declining native orchid (Himantoglossum adriaticum) by pollen transfer and outbreeding between populations.Crossref | GoogleScholarGoogle Scholar | 30907053PubMed |
DeMauro, MM (1993). Relationship of breeding system to rarity in the lakeside daisy (Hymenoxys acaulis var. glabra). Conservation Biology 7, 542–550.
| Relationship of breeding system to rarity in the lakeside daisy (Hymenoxys acaulis var. glabra).Crossref | GoogleScholarGoogle Scholar |
Drummond, RSM, Keeling, DJ, Richardson, TE, Gardner, RC, and Wright, SD (2000). Genetic analysis and conservation of 31 surviving individuals of a rare New Zealand tree, Metrosideros bartlettii (Myrtaceae). Molecular Ecology 9, 1149–1157.
| Genetic analysis and conservation of 31 surviving individuals of a rare New Zealand tree, Metrosideros bartlettii (Myrtaceae).Crossref | GoogleScholarGoogle Scholar |
Ensslin, A, and Godefroid, S (2020). Ex situ cultivation impacts on plant traits and drought stress response in a multi-species experiment. Biological Conservation 248, 108630.
| Ex situ cultivation impacts on plant traits and drought stress response in a multi-species experiment.Crossref | GoogleScholarGoogle Scholar |
Gardner, RC, De Lange, PJ, Keeling, DJ, Bowala, T, Brown, HA, and Wright, SD (2004). A late Quaternary phylogeography for Metrosideros (Myrtaceae) in New Zealand inferred from chloroplast DNA haplotypes. Biological Journal of the Linnean Society 83, 399–412.
| A late Quaternary phylogeography for Metrosideros (Myrtaceae) in New Zealand inferred from chloroplast DNA haplotypes.Crossref | GoogleScholarGoogle Scholar |
Griffin, AR (1980). Floral phenology of a stand of mountain ash (Eucalyptus regnans F. Muell.) In Gippsland, Victoria. Australian Journal of Botany 28, 393–404.
| Floral phenology of a stand of mountain ash (Eucalyptus regnans F. Muell.) In Gippsland, Victoria.Crossref | GoogleScholarGoogle Scholar |
GTA (2021) ‘Global tree assessment.’ (Botanic Gardens Conservation International)
Hanna, C, Foote, D, and Kremen, C (2013). Invasive species management restores a plant–pollinator mutualism in Hawaii. Journal of Applied Ecology 50, 147–155.
| Invasive species management restores a plant–pollinator mutualism in Hawaii.Crossref | GoogleScholarGoogle Scholar |
Hitchcock, A, Williams, J, and Cowell, C (2020). Lessons learned as Erica turgida is returned. Journal for Nature Conservation 56, 125858.
| Lessons learned as Erica turgida is returned.Crossref | GoogleScholarGoogle Scholar |
Horiuchi, Y, Kamijo, T, and Tanaka, N (2020). Biological and ecological constraints to the reintroduction of Eriocaulon heleocharioides (Eriocaulaceae): a species extinct in the wild. Journal for Nature Conservation 56, 125866.
| Biological and ecological constraints to the reintroduction of Eriocaulon heleocharioides (Eriocaulaceae): a species extinct in the wild.Crossref | GoogleScholarGoogle Scholar |
Hyvärinen, M-T (2020). Rubus humulifolius rescued by narrowest possible margin, conserved ex situ, and reintroduced in the wild. Journal for Nature Conservation 55, 125819.
| Rubus humulifolius rescued by narrowest possible margin, conserved ex situ, and reintroduced in the wild.Crossref | GoogleScholarGoogle Scholar |
Iglesias-Andreu, LG, Octavio-Aguilar, P, Vovides, AP, Meerow, AW, de Cáceres-González, FN, and Galván-Hernández, DM (2017). Extinction risk of Zamia inermis (Zamiaceae): a genetic approach for the conservation of its single natural population. International Journal of Plant Sciences 178, 715–723.
| Extinction risk of Zamia inermis (Zamiaceae): a genetic approach for the conservation of its single natural population.Crossref | GoogleScholarGoogle Scholar |
Kaneko, S, Isagi, Y, and Nobushima, F (2008). Genetic differentiation among populations of an oceanic island: the case of Metrosideros boninensis, an endangered endemic tree species in the Bonin Islands. Plant Species Biology 23, 119–128.
| Genetic differentiation among populations of an oceanic island: the case of Metrosideros boninensis, an endangered endemic tree species in the Bonin Islands.Crossref | GoogleScholarGoogle Scholar |
Koelling, VA, Hamrick, JL, and Mauricio, R (2011). Genetic diversity and structure in two species of Leavenworthia with self-incompatible and self-compatible populations. Heredity 106, 310–318.
| Genetic diversity and structure in two species of Leavenworthia with self-incompatible and self-compatible populations.Crossref | GoogleScholarGoogle Scholar | 20485327PubMed |
Kormuťák, A, Galgóci, M, Boleček, P, and Gőmőry, D (2019). Effect of storage on pollen viability in Pinus sylvestris L., Pinus mugo turra and their hybrid swarms. Dendrobiology 82, 43–51.
| Effect of storage on pollen viability in Pinus sylvestris L., Pinus mugo turra and their hybrid swarms.Crossref | GoogleScholarGoogle Scholar |
Lozada-Gobilard, S, Pánková, H, Zhu, J, Stojanova, B, and Münzbergová, Z (2020). Potential risk of interspecific hybridization in ex situ collections. Journal for Nature Conservation 58, 125912.
| Potential risk of interspecific hybridization in ex situ collections.Crossref | GoogleScholarGoogle Scholar |
Melesse K (2017) Molecular phylogeny of the genus Metrosideros and population genetics of some New Zealand species within the genus. Doctor of Philosophy in Biology, University of Canterbury.
Montagna, T, Silva, JZ, Pikart, TG, and Reis, MS (2018). Reproductive ecology of Ocotea catharinensis, an endangered tree species. Plant Biology 20, 926–935.
| Reproductive ecology of Ocotea catharinensis, an endangered tree species.Crossref | GoogleScholarGoogle Scholar | 29786924PubMed |
Nadarajan, J, Benson, EE, Xaba, P, Harding, K, Lindstrom, A, Donaldson, J, Seal, CE, Kamoga, D, Agoo, EMG, Li, N, King, E, and Pritchard, HW (2018). Comparative biology of cycad pollen, seed and tissue - a plant conservation perspective. The Botanical Review 84, 295–314.
| Comparative biology of cycad pollen, seed and tissue - a plant conservation perspective.Crossref | GoogleScholarGoogle Scholar |
Nadarajan, J, van der Walt, K, Lehnebach, CA, Saeiahagh, H, and Pathirana, R (2021). Integrated ex situ conservation strategies for endangered New Zealand Myrtaceae species. New Zealand Journal of Botany 59, 72–89.
| Integrated ex situ conservation strategies for endangered New Zealand Myrtaceae species.Crossref | GoogleScholarGoogle Scholar |
Neal, RP, and Anderson, GJ (2004). Does the ‘old bag’ make a good ‘wind bag’?: comparison of four fabrics commonly used as exclusion bags in studies of pollination and reproductive biology. Annals of Botany 93, 603–607.
| Does the ‘old bag’ make a good ‘wind bag’?: comparison of four fabrics commonly used as exclusion bags in studies of pollination and reproductive biology.Crossref | GoogleScholarGoogle Scholar |
Nikkanen, T, Aronen, T, Häggman, H, and Venäläinen, M (2000). Variation in pollen viability among Picea abies genotypes – potential for unequal paternal success. Theoretical and Applied Genetics 101, 511–518.
| Variation in pollen viability among Picea abies genotypes – potential for unequal paternal success.Crossref | GoogleScholarGoogle Scholar |
Page, T, Moore, GM, Will, J, and Halloran, GM (2006). Pollen viability in Kunzea pomifera (Myrtaceae) as influenced by sucrose concentration and storage. Australian Journal of Botany 54, 553–558.
| Pollen viability in Kunzea pomifera (Myrtaceae) as influenced by sucrose concentration and storage.Crossref | GoogleScholarGoogle Scholar |
Schmidt-Adam, G, Gould, KS, and Murray, BG (1999). Floral biology and breeding system of pohutukawa (Metrosideros excelsa, Myrtaceae). New Zealand Journal of Botany 37, 687–702.
| Floral biology and breeding system of pohutukawa (Metrosideros excelsa, Myrtaceae).Crossref | GoogleScholarGoogle Scholar |
Schmidt-Adam, G, Murray, BG, and Young, AG (2009). The relative importance of birds and bees in the pollination of Metrosideros excelsa (Myrtaceae). Austral Ecology 34, 490–498.
| The relative importance of birds and bees in the pollination of Metrosideros excelsa (Myrtaceae).Crossref | GoogleScholarGoogle Scholar |
Sharrock S (2020) Plant Conservation Report 2020. A review of progress in implementation of the Global Strategy for Plant Conservation 2011-2020. Secretariat of the Convention on Biological Diversity, Montreal, Canada and Botanic Gardens Conservation International, Richmond, UK.
Sudarmono, LD, Hartini, S, and Wawangningrum, H (2016). Hand-pollination of the giant corpse flower in the Bogor Botanic Gardens. International Journal of Conservation Science 7, 1153–1160.
Tani, N, Yoshimaru, H, Kawahara, T, Hoshi, Y, Nobushima, F, and Yasui, T (2006). Determination of the genetic structure of remnant Morus boninensis Koidz. trees to establish a conservation program on the Bonin Islands, Japan. BMC Ecology 6, 14.
| Determination of the genetic structure of remnant Morus boninensis Koidz. trees to establish a conservation program on the Bonin Islands, Japan.Crossref | GoogleScholarGoogle Scholar | 17034624PubMed |
Towill LE (2004) Pollen storage as a conservation tool. In ‘Ex situ plant conservation: supporting species survival in the wild’. (Eds E Guerrant, K Havens, M Maunder) pp. 180–188. (Island Press: Washington, USA)
Volis, S (2017). Conservation utility of botanic garden living collections: setting a strategy and appropriate methodology. Plant Diversity 39, 365–372.
| Conservation utility of botanic garden living collections: setting a strategy and appropriate methodology.Crossref | GoogleScholarGoogle Scholar | 30159530PubMed |
Wheeler, MA, and McComb, JA (2006). In vitro pollen viability and pollen storage in Eucalyptus marginata (Myrtaceae). Australian Forestry 69, 32–37.
| In vitro pollen viability and pollen storage in Eucalyptus marginata (Myrtaceae).Crossref | GoogleScholarGoogle Scholar |