Prevalence of enteric pathogens in shellfish in Madang Province of Papua New Guinea
Sinafa Robby A B * , Monalisa P. Kas A , Kevin Soli A , Glennis Rai A , Andrew R. Greenhill A C , Lloyd Werry B , Stephanie Tringin B , Kathleen Diapong B , Aisak Pue B , William Pomat A , Kari Iamba B , Paul F. Horwood A D and Mohammad Yazid Abdad A D E FA
B
C
D
E
F
Abstract
Shellfish are natural reservoirs for enteric pathogens and are an important food source for some coastal communities in Papua New Guinea (PNG). Little is known about the food safety of shellfish in PNG. Salmonella spp. and Vibrio cholerae are common enteric pathogens known to cause moderate to severe gastrointestinal illnesses in humans, and there is a lack of information on the prevalence of these important bacterial pathogens in shellfish in PNG. The aim of this study was to gain preliminary data on the prevalence of these enteric pathogens in edible shellfish. A total of 400 shellfish samples were collected in estuarine habitats of Madang Province and screened for Salmonella spp. and V. cholerae by real-time polymerase chain reaction (PCR), yielding 0/400 (0%) and 17/400 (4.25%) positives, respectively. Further testing revealed that none of the V. cholerae detected in this study was a toxigenic strain, however the result does not necessarily indicate that there is no risk of cholera infections. Further investigation is warranted. This study provides the first detection of V. cholerae in shellfish in PNG and highlights the potential public health concerns of these natural reservoirs for enteric pathogens.
Keywords: clam, edible shellfish, gastrointestinal pathogens, mussel, real-time PCR, Salmonella spp., Vibrio cholerae.
Introduction
Shellfish are natural reservoirs for enteric pathogens and are an important food source for some coastal communities in Papua New Guinea (PNG).1 Shellfish may take in microorganisms such as enteric pathogens from surrounding water during their filter feeding process.2,3 Consequently, transmission of the pathogens to humans can occur through uncooked, insufficiently cooked or unprocessed shellfish.2–4 Furthermore, some coastal housing including toilet facilities are built on waterways and wastes are emptied directly into rivers and streams. This could potentially lead to waterway contamination providing high chances for shellfish exposure to sources of contamination and transmission of pathogen to humans.
Salmonella spp. and Vibrio spp. are key enteric pathogens in PNG. Both Salmonella spp. and Vibrio spp. are known to cause moderate to severe illnesses in humans, such as diarrhoeal diseases and septicemia.2,3,5–7 Infections caused by virulent strains may result in fatal outcomes, especially in patients who are immunocompromised or have liver diseases.8,9 Vibrio spp. are highly adaptive in the aquatic environment and live naturally in riverine and estuarine ecosystems including animal hosts.5 While the natural habitat of Salmonella spp. are not thought to be estuarine and marine environments, they can easily make their way into such environments through human and agricultural contamination. Seafood can be contaminated with Salmonella spp. particularly in those countries where sanitation and hygiene are lacking.10
An example of enteric infections in PNG was the cholera outbreak of 2009–2011 that rapidly spread throughout coastal areas of the country with more than 15,500 cases of cholera and over 500 deaths11,12 including two cases detected as part of a study in Central Province, PNG.13
While there is no evidence the consumption of shellfish played a major role in cholera in PNG, other studies have confirmed non-toxigenic strains of Vibrio cholerae have been associated with cases of diarrhoeal illness in multiple locations throughout the world.14–16 The 2009–2011 outbreak suggests a high risk of cholera transmission in the coastal regions of PNG.5 Salmonella spp. on the other hand are also known to be one of the most common food-borne pathogens globally and their detection in seafood highlights the need to investigate its prevalence in shellfish.2
This study aimed to investigate and establish preliminary information on Salmonella spp. and V. cholerae in shellfish and highlights the potential public health concerns of shellfish being natural reservoirs for enteric pathogens in PNG.
Methodology
Sampling location
Shellfish sample collection was conducted from the estuaries and coastal habitat within the township of Madang with varying distribution of shellfish across the sampling sites (Fig. 1). A total of 11 sites were selected based on accessibility of the principal investigator who is from Madang province. The province is in the Momase Region of PNG and Madang is located at latitude of 5°13′S and longitude 145°48′E, with an elevation of 3 m above sea level and is generally characterised by a tropical climate with a mean monthly temperature of 32°C and average monthly rainfall of 259 mm.17 The population size of Madang province was about 493,906 according to 2011 PNG National Report.17
Map showing different shellfish collection sites within the Madang town. Site 1 = Airport Area 1, Site 2 = Airport Area 2, Site 3 = Airport Area 3, Site 4 = DCA Land 1, Site 5 = DCA Land 2, Site 6 = DCA Land 3, Site 7 = DCA Land 4, Site 8 = Nui Timin Island, Site 9 = Wagol Creek, Site 10 = Yamilong Round. Wara, Site 11 = Yamilong Lagoon. DCA = Department of Civil Aviation. Source: Google Maps.
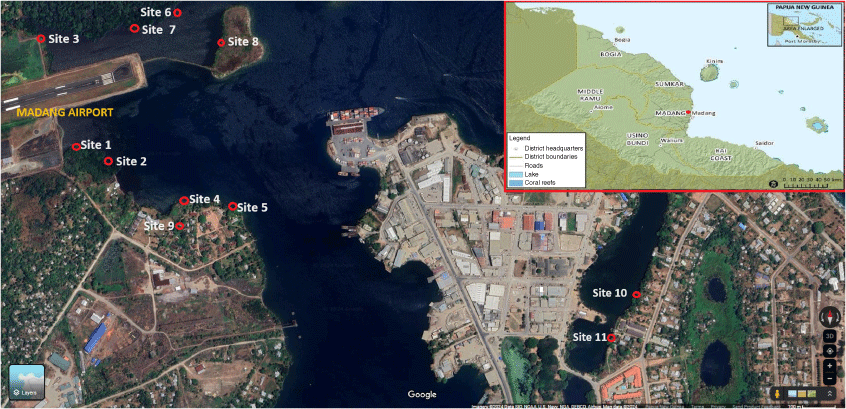
Sampling techniques, transportation and storage
Sampling was conducted by the principal investigator (MPK) with the assistance of local people from each sampling site. Locals engaged in the shellfish sampling were given a brief explanation and short training on what types of edible shellfish to collect. The collectors were remunerated. Recording of the collection was maintained by the principal investigator (MPK).
Samples from the 11 sites were collected in the morning hours of each day over a 3-day period. Any edible shellfish sighted in each sampling site were hand collected and placed in buckets filled with water collected from site river system. The number of shellfishes collected from each site were recorded.
At the end of each day, collected samples were transported from the sampling sites to the Papua New Guinea Institute of Medical Research (PNGIMR) branch in Madang which is less than an hour’s drive from each of the sampling sites. At the Madang laboratory, the shellfish were cleaned and placed into plastic bags, labelled according to date and site of collection and temporarily stored in a −20°C chest freezer. A total of 400 shellfish were collected in the 3-day sampling period.
In the early morning hours of the fourth day, the samples were repacked in cooler boxes with ice packs and transported by road from Madang laboratory to PNGIMR Goroka branch laboratory in the Eastern Highlands Province (EHP). The samples reached Goroka within 6–7 h. Upon arrival the shellfish samples were immediately stored in −20°C freezers.
DNA extraction and molecular detection
After a week of storage in the −20°C freezer, the shellfish samples were thawed, and images of each shellfish were captured for identification purposes using a digital camera. Tissue of each shellfish was then removed aseptically from the cleaned shell and placed in sterile falcon tubes and stored in the −80°C freezer until required for DNA extraction.
DNA was later aseptically extracted in a type A2 biosafety cabinet from each shellfish tissue using the DNeasy Blood and Tissue Kit (Qiagen, Hilden, Germany), according to the manufacturer’s instructions. Two grams of shellfish tissue was used for each extraction. The final extracted DNA from each sample was aliquoted into 2 mL screwcap tubes and stored in the −80°C freezer until required for quantitative real-time polymerase chain reaction (qPCR) testing.
For qPCR screening, the stored DNA extracts were thawed and specific genes of interest were targeted to determine the presence of pathogens (Salmonella spp. and V. cholerae) according to the qPCR protocols described in the literature.9,18,19 Table 1 shows the specific primers and the probes used in the qPCR analysis. The primers and probes including positive controls were obtained from previous studies conducted at PNGIMR.7
Primer | Sequence | Target gene | Reference | |
---|---|---|---|---|
Forward Primer SAomp-F | CCTGGCAGCGGTGATCC | Salmonella spp. ompF gene | Tatavarthy and Cannons (2010) | |
Reverse Primer SAomp-R | AAATTTCTGCTGCGTTTGCG | |||
Probe SAomp-P | 6FAM-TGCCCTGCTGGCTGCTGCA-MGBNFQ | |||
Forward Primer VC hly-F | GGCAAACAGCGAAACAAATACC | Vibrio cholerae hlyA gene | Rivera et al. (2001) | |
Reverse Primer VC hly-R | CTCAGCGGGCTAATACGGTTTA | |||
Probe VC hly-P | AGCCAAACTAGAGGCGAGAGCAAGTTATAC | |||
Forward Primer VCctxF | TTTGTTAGGCACGATGATGGAT | Vibrio cholerae ctx A gene | Blackstone et al. (2007) | |
Reverse Primer VCctxR | ACCAGACAATATAGTTTGACCCACTAAG | |||
Probe VCctxP | ACTGGACTTCTTATTRCCGTTCTATG |
The qPCRs were then performed on a CFX96 real-time thermocycler (Bio-Rad, USA). PCR cycling parameters for Salmonella spp. amplification were set as follows: initial activation at 95°C for 15 min, with 40 cycles of denaturation at 94°C for 60 s and annealing/extension at 60°C for 60 s.18
PCR cycling parameters for V. cholerae amplification were set as follows: initial activation at 60°C for 2 min, 95°C for 15 min followed by 40 cycles of denaturation at 94°C for 60 s and annealing/extension at 60°C for 60 s.9
Vibrio spp. positives were tested for toxigenic gene. The PCR cycling parameters for the V. cholerae toxigenic gene was set as follows: initial activation at 50°C for 2 min, 95°C for 15 min followed by 40 cycles of denaturation at 94°C for 60 s and annealing/extension at 60°C for 60 s.19
Shellfish identification
The shellfish specimens collected in the study were identified to species level based on morphological characteristics using a standard shellfish identification chart20,21 and identification methods used.22 Images of each shellfish captured using a digital camera were sent to the Department of Fisheries and Marine Resources at PNG University of Natural Resources and Environment (UNRE) for further verification and confirmation.
Data analysis
Quantitative data generated from the qPCR were entered into Microsoft Excel spreadsheets (Microsoft, Redmond, USA) and utilised to determine the proportion of the pathogen in the population of shellfish collected. The main variable for analysis was the determination of pathogen prevalence in shellfish across the sampling sites. The presence (positive) and absence (negative) of the enteric pathogens was computed from the original qPCR data. Graphical representation comparing the presence or absence of the two enteric pathogens and their prevalence was done with the ggplot2 package in RStudio (https://github.com/kari983/ShellfishRcode/blob/main/README.md?plain=1).
Quality management
Quantification of extracted DNA and measurement of impurities was done using a spectrophotometer (Nanodrop) as part of quality assessments. Positive controls (positive V. Cholerae and Sallmonella spp. samples from previous studies) and negative controls (nuclease free water) were included in all qPCR runs to ensure quality control. DNA extracts from each sample were stored in the −80°C freezer and easily accessed and retested when required to ensure the validity and reliability of the assays.
Results
We collected a total of 400 shellfish samples across 11 sites in the Madang area. Seven samples of shellfish were collected in Airport Area 1 (Site 1), 62 in Airport Area 2 (Site 2), 73 in Airport Area 3 (Site 3), 21 in DCA Land 1 (Site 4), 16 in DCA Land 2 (Site 5), 59 in DCA Land 3 (Site 6), 40 in DCA Land 4 (Site 7), 46 in Nui Timin Island (Site 8), 22 in Wagol Creek (Site 9), 20 in Yamilong Round Wara (Site 10) and 34 in Yamilong Lagoon (Site 11) (Fig. 2).
The number of shellfish samples collected at each site. The distribution of shellfish by sampling sites, we used the sites as a factor while shellfish species type as a categorical variable and species counts as response.
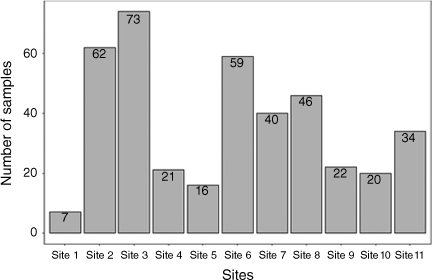
A total of 11 shellfish species were found across the 11 sites (Table 2). The highest number of shellfish species was found at Site 5 (n = 7) followed by Site 1 (n = 3), Site 3 (n = 2) and Site 10 (n = 2). The other seven sites hosted a single species each.
Species ID | Common name | Family | Species | |
---|---|---|---|---|
SP 1 | Toothless clam | Lucinidae | Anodontia edentula | |
SP 2 | Punctate codakia | Lucinidae | Codakia punctata | |
SP 3 | Common basket lucina | Fimbridae | Fimbria fimbriatus | |
SP 4 | Fan mussel | Pinnidae | Atrina fragilis | |
SP 5 | Freshwater mussel | Unionidae | Haasodonta vanheurni | |
SP 6 | Magical cone | Conidae | Conus magus | |
SP 7 | Tiger cowrie | Cypraeidae | Cypraea tigris | |
SP 8 | Tapestry turban | Turbinidae | Turbo petholatus | |
SP 9 | Giant clam | Tridacnidae | Tridacna gigas | |
SP 10 | Prickly spotted drupe | Muricidae | Drupa ricinus | |
SP 11 | Trochus shell | Trochidae | Trochus niloticus |
Only Anodontia edentula was collected in Site 2 (Airport Area 2), Site 4 (DCA Land 1), Site 6 (DCA Land 3), Site 7 (DCA Land 4), Site 8 (Nui Timin Island) and Site 9 (Wagol Creek). Site 11 (Yamilong Lagoon) was dominated entirely by Codakia punctata (Fig. 3). The proportion of C. punctata (57%) was higher at Site 1 (Airport Area 1) than Haasodonata vanheumi (29%) and Atrina fragilis (14%). Two species collected at Site 3 (Airport Area 3) were C. punctata (70%) and Conus magus (30%). Seven species collected at Site 5 (DCA Land 2) include Cypraea tigris (37%), Fimbria fimbriatus (19%), Tridacna gigas (13%), Turbo petholatus (13%), Trochus niloticus (6%), Drupa ricinus (6%) and A. edentula (6%).
The distribution of 11 shellfish species across sampling sites. The coloured legend shows the proportion of shellfish species type at each site. Values within the coloured columns are percentage (%) of each species collected at specific sites.
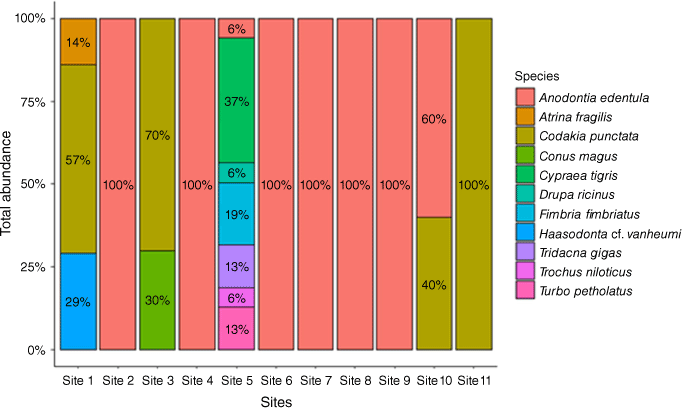
V. cholerae was only detected in two shellfish species, C. punctata and C. magus. However, no Salmonella spp. outer membrane protein (OMP) was detected in the species collected. Fifteen shellfish of C. punctata tested positive for V. cholerae. Two C. magus shellfish also tested positive for V. cholerae while the rest produced negative results. The vibrio positives were tested for the V. cholera toxin gene however all results came up negative. The results showed that Salmonella spp. OMP was not prevalent in shellfish found in the sampling sites in Madang. Salmonella spp. OMP was absent in the 400 samples collected while only 4% of the shellfish samples tested positive for V. cholerae.
Discussion
We conducted a survey of shellfish in the Madang coastal area to determine the proportion of shellfish with either V. cholerae or Salmonella spp. to investigate the potential risk of enteric pathogens and disease to Madang residents consuming local shellfish.
We found V. cholerae in 17 of the 400 shellfish samples collected although none of these contained the toxic gene associated with the severe disease and epidemic strain. V. cholerae was only detected in two species of shellfish, C. punctata and C. magus found around the Airport Area 1 (Site 1) and Yamilong Round Wara (Site 10) and Yamilong Lagoon (Site 11). However, no Salmonella spp. were detected in the 400 samples of shellfish collected.
The presence of V. cholerae confirms the risk of shellfish acting as potential natural reservoirs for enteric pathogens,23 thus being a potential public health concern. The absence of Salmonella spp. was pleasing from a public health perspective, as they have been shown to survive in aquatic habitats and have been detected in high numbers in dried oysters and shellfish.24 It is therefore important that shellfish and other seafood are properly cooked before consumption.25 Less than half the population of PNG have access to safe waste and sanitation facilities and safe drinking water with no appreciable improvements over recent years, leading to greater risk of enteric infections and outbreaks.12
The distributional trend of shellfish species (Fig. 3) in the sampling sites also suggests the potential prevalence of enteric pathogens and risks for future cases of food borne illness. A lucinid bivalve mollusc (C. punctata) and a coned mollusc (C. magus) that yielded positive to V. cholerae showed a discernible distributional pattern; C. punctata is more widely distributed across the four sampling sites and C. magus was localised to two sites. C. punctata is commonly collected for consumption both locally and elsewhere.1,2
The risk of potential infections by V. cholerae to the population of Madang town increased with the ease of accessibility to collection sites of the shellfish for consumption and to sell at local markets. Such estuarine environments may potentially facilitate the transmission of pathogens.11,12 This supports the World Health Organization (WHO) report that water samples collected from rivers in coastal provinces in PNG during the 2009–2011 cholera outbreak indicated high levels of contamination with V. cholerae.26
Limitations
Samples were collected from a single location (Madang), and the results cannot be extrapolated to the rest of the country. In addition, small numbers of samples were collected for each shellfish species which may not be representative of the true prevalence of V. cholerae and Salmonella spp. in those shellfish species.
Seasonal variation was not assessed, and this could have impacts on the detection rate due to rainfall and temperature differences. Further research on climatic and environmental factors that may facilitate proliferation, transmission and spread of enteric pathogens in shellfish may be beneficial. Weather phenomena such as increased rainfalls and elevated sea surface temperatures are considered important factors in the increased risk of cholera outbreaks for coastal areas.27 The 2009–2011 cholera outbreak in PNG5,11,12 may have been introduced through an infectious traveller or by ballast water from a ship,28 however climatic factors may have initiated plankton blooms, the abundance of which have also been associated with an increased presence of V. cholerae. Sea and estuarine waters of these coastal villages are possible sources of introduction.29 In brief, we know relatively little about PNG’s marine habitats and their interactions with human health.
Conclusion
This study provides the first report on the prevalence of V. cholerae and Salmonella spp. in shellfish in PNG, which could potentially act as a reservoir for enteric pathogens. The results demonstrate there may be a moderate risk of food borne illness associated with consumption of shellfish in PNG. However, there is insufficient data at this point to be able to ascertain the magnitude of the risk, or to develop evidence-based, informed decision making in the handling and processing of shellfish and other seafood before consumption. Future studies may focus on Vibrio spp. and indicator organisms such as thermotolerant coliforms. Vibrio spp. should be investigated as they are natural inhabitants of aquatic environments and important human pathogens. Indicator organisms such as thermotolerant coliforms are indicative of faecal contamination, including various bacterial and viral gastrointestinal pathogens. In addition, further research is needed to establish baseline data on the prevalence of other enteric pathogens in shellfish and other seafood in PNG. Studies may also look into continuous monitoring and surveillance of shellfish population including water monitoring and testing for other enteric pathogens.
Data availability
The data that supports this study will be shared upon reasonable request to the corresponding author.
Declaration of funding
Funding support for this work was received from the PNG Institute of Medical Research, research grants.
References
1 Swadling P, Chowning A. Shellfish gathering at Nukalau Island, West New Britain Province, Papua New Guinea. J Soc Océan 1981; 72–73: 159-167.
| Google Scholar |
2 Dutta C, Panigrahi AK, Sengupta C. Prevalence of Pathogenic Bacteria in Finfish and Shellfish Obtained from Domestic Markets of West Bengal, India. Front Environ Microbiol 2015; 1: 14-18.
| Crossref | Google Scholar |
3 Panicker G, Call DR, Krug MJ, Bej AK. Detection of Pathogenic Vibrio spp. in Shellfish by Using Multiplex PCR and DNA Microarrays. Appl Environ Microbiol 2004; 70: 7436-7444.
| Crossref | Google Scholar | PubMed |
4 Yam WC, Chan CY, Ho Bella SW, Tam TY, Kueh C, Lee T. Abundance of clinical enteric bacterial pathogens in coastal waters and shellfish. Water Res 2000; 34: 51-56.
| Crossref | Google Scholar |
5 Horwood P, Greenhill A. Cholera in Papua New Guinea and the importance of safe water sources and sanitation. Western Pac Surveill Response J 2012; 3: 3-5.
| Crossref | Google Scholar | PubMed |
6 Sjöling Å, Sadeghipoorjahromi L, Novak D, Tobias J. Detection of major diarrheagenic bacterial pathogens by multiplex PCR panels. Microbiol Res 2015; 172: 34-40 . Epub 12 December 2014.
| Crossref | Google Scholar | PubMed |
7 Soli KW, Maure T, Kas MP, Bande G, Bebes S, Luang-Suarkia D, Siba PM, Morita A, Umezaki M, Greenhill AR, Horwood PF. Detection of enteric viral and bacterial pathogens associated with paediatric diarrhoea in Goroka, Papua New Guinea. Int J Infect Dis 2014; 27: 54-58 . Epub 1 September 2014.
| Crossref | Google Scholar | PubMed |
8 Issa H, Shorman M, Bseiso B, Al-Salem AH. A Case of O1 Vibrio Cholera Bacteremia and Primary Peritonitis in a Patient with Liver Cirrhosis. Gastroenterol Res 2009; 2: 358-360.
| Crossref | Google Scholar |
9 Rivera ING, Chun J, Huq A, Sack RB, Colwell RR. Genotypes Associated with Virulence in Environmental Isolates of Vibrio cholerae. Appl Environ Microbiol 2001; 67: 2421-2429.
| Crossref | Google Scholar | PubMed |
10 Heinitz ML, Ruble RD, Wagner DE, Tatini SR. Incidence of Salmonella in fish and seafood. J Food Prot 2000; 63: 579-592.
| Crossref | Google Scholar | PubMed |
11 Horwood PF, Karl S, Mueller I, Jonduo MH, Pavlin BI, Dagina R, Ropa B, Bieb S, Rosewell A, Umezaki M, Siba PM, Greenhill AR. Spatio-temporal epidemiology of the cholera outbreak in Papua New Guinea, 2009–2011. BMC Infect Dis 2014; 14: 449.
| Crossref | Google Scholar | PubMed |
12 Greenhill AR, Mutreja A, Bulach D, Belousoff MJ, Jonduo MH, Collins DA, Kas MP, Wapling J, Seemann T, Lafana A, Dougan G, Brown MV, Horwood PF. Wave 2 strains of atypical Vibrio cholerae El Tor caused the 2009–2011 cholera outbreak in Papua New Guinea. Microb Genom 2019; 5: e000256.
| Crossref | Google Scholar | PubMed |
13 Abdad MY, Soli KW, Pham B, Bande G, Maure T, Jonduo M, Kisa D, Rai G, Phuanukoonnon S, Siba PM, Horwood PF, Greenhill AR. Diarrhoeal disease surveillance in Papua New Guinea: findings and challenges. Western Pac Surveill Response J 2020; 11: 7-12.
| Crossref | Google Scholar | PubMed |
14 Wang H, Yang C, Sun Z, Zheng W, Zhang W, Yu H, Wu Y, Didelot X, Yang R, Pan J, Cui Y. Genomic epidemiology of Vibrio Cholerae reveals the regional and global spread of the two epidemic non-toxigenic lineages. PLoS Negl Trop Dis 2020; 14: e000046.
| Crossref | Google Scholar |
15 Morita D, Morita M, Alam M, Mukhopadhyay AK, Johura F-T, Sultana M, Monira S, Ahmed N, Chowdhury G, Dutta S, Ramamurthy T, Samanta P, Takahashi E, Okamoto K, Izumiya H, Ohnishi M. Whole-Genome Analysis of Clinical Vibrio cholerae O1 in Kolkata, India, and Dhaka, Bangladesh, Reveals Two Lineages of Circulating Strains, Indicating Variation in Genomic Attributes. mBio 2020; 11: e01227-20.
| Crossref | Google Scholar | PubMed |
16 Chowdhury G, Joshi S, Bhattacharya S, Sekar U, Birajdar B, Bhattacharyya A, Shinoda S, Ramamurthy T. Extraintestinal Infections Caused by Non-toxigenic Vibrio cholerae non-O1/non-O139. Front Microbiol 2016; 7: 144.
| Crossref | Google Scholar | PubMed |
17 Papua New Guinea National Statistical Office. Papua New Guinea 2011 National report/National Statistical Office. Port Moresby; 2015. 96 pp. Available at https://png-data.sprep.org/system/files/2011%20Census%20National%20Report.pdf
18 Tatavarthy A, Cannons A. Real-time PCR detection of Salmonella species using a novel target: the outer membrane porin F gene (ompF). Lett Appl Microbiol 2010; 50: 645-652.
| Crossref | Google Scholar |
19 Blackstone GM, Nordstrom JL, Bowen MD, Meyer RF, Imbro P, DePaola A. Use of a real time PCR assay for detection of the ctxA gene of Vibrio cholerae in an environmental survey of Mobile Bay. J Microbiol Methods 2007; 68: 254-259 . Epub 10 October 2006.
| Crossref | Google Scholar | PubMed |
20 Thurston T. Bivalve Shellfish Identification. Washington Department of Fish & Wildlife, Washington State Department of Health. DOH 332-087. 2023. Available at www.doh.wa.gov/Portals/1/Documents/4400/332-087-Ahellfish-ID.pdf
22 Tan KS, Kastoro WW. A small collection of Gastropods and Bivalves from the Anambas and Natuna Islands, South China sea. Raffles Bull Zool 2004; 11: 47-54.
| Google Scholar |
23 Haddock RL, Nocon FA. NAG Vibrio cholerae isolated from imported shellfish in Guam. Southeast Asian J Trop Med Public Health 1985; 16: 113-116.
| Google Scholar | PubMed |
24 Wilson IG, Moore JE. Presence of Salmonella spp. and Campylobacter spp. in shellfish. Epidemiol Infect 1996; 116: 147-153.
| Crossref | Google Scholar | PubMed |
25 Flick GJ, Jr, Granata AL. Biological Safety of Fresh and Processed Shellfish. South Regi Aquacult Center 2010; 4901: 8.
| Google Scholar |
27 Christaki E, Dimitriou P, Pantavou K, Nikolopoulos GK. The impact of climate change on cholera: A review on the global status and future challenges. Atmosphere 2020; 11: 449.
| Crossref | Google Scholar |
28 Horwood PF, Greenhill AR. Cholera in Papua New Guinea: observations to date and future considerations. PNG Med J 2013; 56: 162-165.
| Google Scholar | PubMed |
29 Rosewell A, Clark G, Mabong P, Ropa B, Posanai E, Man NWY, Dutta SR, Wickramasinghe W, Qi L, Ng JC, Mola G, Zwi AB, MacIntyre CR. Concurrent outbreaks of cholera and peripheral neuropathy associated with high mortality among persons internally displaced by a volcanic eruption. PLoS One 2013; 8: e72566.
| Crossref | Google Scholar | PubMed |