Mobilisation and replenishment of phosphorus reserves in Bos indicus cows. 2. Mature lactating cows fed diets deficient or adequate in phosphorus
R. M. Dixon

A
B
C
D
E
F
G Present address:
Abstract
Pastures growing on low phosphorus (P) soils are often P deficient, particularly for lactating cows.
To examine the effects of P-deficient diets on the performance of lactating multiparous cows.
From parturition, mature Bos indicus cross cows (n = 32, initially 474 kg liveweight (LW) and body condition score 3.5) were fed ad libitum for 14 weeks one of four diets: (i) high P (HP-HCa), (ii) low P with low calcium (LP-LCa), (iii) low P with high Ca (LP-HCa), or (iv) the LP-LCa diet with ammonium chloride (LP-LCa+ac).
Voluntary feed intakes were higher for cows fed the HP-HCa than the three LP diets (23.1 vs 17.4–19.6 g DM/kg LW.day; P < 0.001). The HP-HCa cows gained 0.15 kg LW/day, whereas LP cows lost 0.14–0.51 kg LW/day; P < 0.05). The cows fed LP diets generally maintained milk production and calf growth comparable to that of HP-HCa cows (5.8 kg/day and 0.70 kg/day). The HP-HCa cows retained 6.0–6.4 g P/day from weeks 1–8, but P retention was negligible at week 14. The LP cows mobilised 4.9–9.1 g body P/day. Rib cortical bone shortly after parturition was 634 ± 127 (337–848) μg P/mm2 in the 12th rib, and indicated that the bone P reserves were deficient, marginal and adequate in 27, 37 and 37% of the cows, respectively. During lactation, rib bone P increased 23% in HP-HCa cows, changed little in the LP-LCa and LP-HCa cows (−4 and +7%), and decreased 13% (P < 0.05) in LP-LCa+ac cows. The change in rib cortical bone P during lactation was correlated with this measurement at parturition; the P per unit surface area of rib cortical bone of cows with low bone P at parturition did not change, but in cows with high bone P it decreased up to 16%. Plasma inorganic P averaged 1.49 mmol/L in the HP-HCa cows and <0.8 mmol/L in the LP cows. Plasma Ca, Ca/inorganic P ratio, 1,25-dihydroxy vitamin D3, bone alkaline phosphatase, and carboxy-terminal telepeptides of type 1 collagen increased during lactation (P < 0.05 to P < 0.001) in the LP cows.
When fed acutely P-deficient diets, mature cows of moderate LW and with body condition scores at parturition generally maintained milk secretion and calf growth by mobilisation of body reserves.
The capacity of beef cows to maintain lactation when ingesting P-deficient diets provides opportunities for improved herd management.
Keywords: beef cows, bone minerals, calf growth, diet calcium, lactation, mobilisation, phosphorus, phosphorus deficiency.
Introduction
Beef cattle production, especially in northern Australia, South America and Africa, often utilises rangelands with very low soil phosphorus (P) concentrations that lead to low pasture P concentrations and nutritional deficiencies of P in grazing cattle. In such rangelands, P deficiencies may be a major constraint to both production and welfare of animals. The bones and soft tissues of cattle that are replete in P contain ~8.9 g P/kg empty bodyweight, which in a 450-kg cow corresponds to ~2.7 g bone P and ~0.7 kg soft tissue P (ARC 1980). At least 30%, and in some circumstances up to 45%, of bone P may be mobilised during severe and extended P deficiencies in beef cows (Benzie et al. 1959; Little 1983; Read et al. 1986a, 1986b; Dixon et al. 2017). This is sufficient to substantially contribute to P requirements during dietary P deficiencies. In addition, numerous experiments have established that bone mineral reserves are mobilised during dietary P or Ca deficiency in lactating dairy cows (Knowlton and Herbein 2002; Salazar et al. 2013), dairy goats (Deitert and Pfeffer 1993) and sheep (Braithwaite 1986). The potential for such mobilisation is recognised in nutritional feeding standards, such as those reported by Suttle et al. (1991), CSIRO (2007) and NRC (2016). However, these nutritional recommendations do not provide quantitative estimates of the amounts, duration or circumstances when bone P and Ca are mobilised, or of the subsequent replenishment that will be necessary for ongoing growth and reproduction.
Phosphorus deficiencies of cattle grazing extensive rangelands are usually managed by providing P supplements. However, there are major challenges to achieving optimal and target intakes of a specific mineral when supplements are fed as mixtures of minerals or as feed blocks offered free-choice. These include achieving target intakes of the key supplementary nutrients and high variability in supplement intakes among individuals within a herd. Furthermore, P deficiencies in cattle grazing seasonally dry rangelands usually occur during the rainy season, when the pasture metabolisable energy, protein and P are at their highest during the annual cycle, but when demands for P for late pregnancy, lactation and growth of cows are also at their highest. Unfortunately, this is also the season when effective mineral supplementation is most difficult (McCosker and Winks 1994; Dixon et al. 2020a; Jackson et al. 2023). In these production systems, the mobilisation and subsequent replenishment of bone minerals are physiological mechanisms that often have important effects on the P nutrition of breeder cows, including in well managed production systems.
Management of the P nutrition of beef breeder cows grazing P-deficient rangelands requires knowledge of when, and to what extent, cows mobilise their body P reserves to provide for pregnancy and lactation when P is deficient in the diet, and the circumstances in which cows replenish these reserves. An experiment examined the effects of feeding three acutely P-deficient diets to mature Bos indicus cross cows during early lactation, and compared these with cows consuming a high-P diet. The hypotheses were that when fed acutely P-deficient diets, lactating beef cows would: (i) mobilise sufficient body P reserves to maintain milk production for calf growth, (ii) reduce bone P mobilisation when diet Ca is high, and (iii) increase bone P mobilisation when ingesting a more acidogenic diet. Cows in early lactation were fed a diet high in P (HP-HCa) or one of three diets low and deficient in P (LP diets). The effects on voluntary intake, mobilisation or replenishment of body P, liveweight (LW) change, milk secretion, calf growth, bone minerals, and plasma concentrations of plasma inorganic P (Pi), Ca and metabolic markers in the cows were measured.
Materials and methods
Cattle and diets
Mature Bos indicus × Bos taurus (~5/8–3/4 B. indicus) cows (6–11 years of age, n = 32) from a herd at the Queensland Department of Primary Industries Spyglass Research Station (100 km west of Townsville, Queensland, Australia, in the seasonally dry tropics) were used in the experiment. These cows had been relocated to the Brian Pastures Research Station, Gayndah, in the subtropics of Queensland in June 2014, and had been utilised in a previous experiment (June–September 2014) that examined the effects of P-deficient and P-adequate diets during the second trimester of pregnancy (Dixon et al. 2024). During an introductory interval for the present experiment, from the 29 September 2014 when the cows were 20–30 weeks pregnant, and until parturition, the cows were managed as a single group in a 9-ha paddock. During this phase (11–20 weeks, depending on the date of parturition of individuals), the cows were fed a high-P diet comprising oat hay ad libitum supplemented with 2.5 kg as-fed/cow.day of a mixture (g/kg, as fed) comprising 765 whole cottonseed, 191 cottonseed meal, 20 mono dicalcium phosphate, 20 limestone and 4 premix. The cows were weighed and blood sampled every 3 weeks, and calved in the paddock. Measurements of the rib cortical bone obtained by biopsy had been made at the end of the previous experiment. The experiment was approved by the Animal Ethics Committee of the Queensland Department of Primary Industries (Approvals SA 2014/12/490 and SA 2014/12/495).
Parturition of the cows was from 16 December 2014 to 18 February 2015, with the mean date 17 January 2015 (±17 days). Within 24 h of parturition, the cows were blood sampled, their LWs measured, and body condition scores (BCS; 1–5 scale (CSIRO 2007)) estimated by the same experienced person. The cows were (mean ± s.d. (range)) 474 ± 57 (372–598) kg LW, and 3.5 ± 0.55 (2.0–4.3) BCS immediately after parturition. The hip height was 137 ± 4.6 cm, the fat cover at the P8 rump and rib sites were 3.70 ± 2.48 and 2.00 ± 1.17 mm, respectively, and the eye muscle area was 51.0 ± 11.6 cm2. The P per unit surface area of rib cortical bone (PSACB) shortly after parturition was equivalent to 570 ± 117 (312–786) μg P/mm2 in the 12th rib. Based on the criteria that PSACB of <550, 550–650 and >650 μg P/mm2 in the 12th rib in mature cows are indicative of deficient, marginal and adequate bone mineral status, respectively, 27, 37 and 37% of the cows were in these respective classes at parturition (Dixon et al. 2019). The cows and calves were relocated to individual pens in a roofed, but open-sided, animal shed. Groups of four cows calving over several days were considered as a block, housed in adjoining pens and randomly allocated to one of the four diet treatments. The blocks thus encompassed variation both in date of parturition and the pen position in the shed. Half of each pen (33 m2) had a concrete floor, while the remainder was soil. Canvas sails provided additional shade and water was freely available from troughs.
The diets were: (1) high P and high Ca (HP-HCa), (2) low P and low Ca (LP-LCa), (3) low P and high Ca (LP-HCa), and (4) low P and low Ca with the addition of ammonium chloride (LP-LCa+ac; Table 1). The diets based on chopped wheat straw, wheat flour and sugar, with the addition of urea, minerals and vitamins, were prepared as total mixed rations. Mono dicalcium phosphate (Kynophos; KK Animal Nutrition) was included in the HP-HCa diet, and limestone in the HP-HCa and LP-HCa diets. Ammonium chloride (5 g/kg) was included to provide the mildly acidogenic LP-LCa+ac diet. The cows were introduced to the treatment diets over 4 days, during which additional straw was added to allow adaptation and to minimise the risk of acidosis. Batches of each diet were prepared weekly. Fresh feeds were offered daily at ~10% in excess of the previous intakes of individuals to achieve ad libitum intakes, and feed refusals were collected weekly.
Attribute | Treatment diets | ||||
---|---|---|---|---|---|
HP-HCa | LP-LCa | LP-HCa | LP-LCa+ac | ||
Ingredient (as fed, g/kg) | |||||
Wheat straw | 509 | 517 | 514 | 516 | |
Wheat flour | 280 | 285 | 283 | 284 | |
Sugar | 140 | 142 | 141 | 142 | |
Canola oil | 22.1 | 22.4 | 22.3 | 22.4 | |
Urea | 22.1 | 20.4 | 22.3 | 17.3 | |
Mono dicalcium phosphate | 9.4 | 0.0 | 0.0 | 0.0 | |
Limestone | 4.7 | 0.0 | 4.7 | 0.0 | |
Ammonium sulfate | 4.7 | 4.8 | 4.7 | 4.7 | |
Ammonium chloride | 0.0 | 0.0 | 0.0 | 5.0 | |
Sodium chloride | 4.7 | 4.7 | 4.7 | 4.7 | |
Magnesium oxide | 2.33 | 2.36 | 2.35 | 2.36 | |
Rumigro premix A | 0.762 | 0.767 | 0.769 | 0.773 | |
Elanco rumensin 100 B | 0.265 | 0.267 | 0.268 | 0.269 | |
Composition | |||||
DM (g/kg as fed) | 948 | 947 | 946 | 944 | |
Organic matter (g/kg DM) | 915 (3.3) | 923 (2.8) | 921 (5.5) | 925 (3.3) | |
Crude protein (g/kg DM) | 126 (8.7) | 120 (14.3) | 122 (15.4) | 113 (2.1) | |
Neutral detergent fibre (g/kg DM) | 388 (34) | 415 (25) | 396 (28) | 397 (5) | |
Acid detergent fibre (g/kg DM) | 294 (15) | 283 (20) | 286 (27) | 286 (11) | |
Lignin (g/kg DM) | 38 (5) | 31 (4) | 35 (9) | 27 (8) | |
Crude fat (g/kg DM) | 36 (3) | 34 (2) | 35 (2) | 35 (4) | |
Calcium (g/kg DM) | 4.63 (0.81) | 1.72 (0.73) | 2.79 (0.37) | 1.53 (0.16) | |
Phosphorus (g/kg DM) | 2.16 (0.51) | 0.57 (0.23) | 0.52 (0.07) | 0.53 (0.05) | |
Magnesium (g/kg DM) | 1.99 (0.37) | 1.89 (0.32) | 1.69 (0.34) | 1.77 (0.37) | |
Sulfur (g/kg DM) | 2.31 (0.19) | 3.00 (0.36) | 2.10 (0.36) | 2.18 (0.22) | |
Diet Ca/P | 2.24 (0.63) | 3.02 (0.55) | 5.49 (0.96) | 2.89 (0.30) | |
DCAD (mequivalents) C | −3 (3.0) | −1 (3.1) | −1 (4.1) | −10 (3.5) |
The diets comprised High P-High Ca, Low P-Low Ca, Low P-High Ca and Low P-Low Ca with addition of ammonium chloride (HP-HCa, LP-LCa, LP-HCa, LP-LCa+ac). The mean and s.d. (in parenthesis) are given for samples of the constituents. Organic constituents were the mean of four samples. Mineral constituents were sampled weekly (means of 20 samples).
Measurement and sampling procedures
The LWs of cows and calves were measured before feeding on the same day each week, and in addition within 24 h after parturition. Cow BCS was estimated weekly and by the same experienced person. Voluntary intakes were measured weekly from the feed offered and refused. Feed offered was sampled as each batch was prepared, and feed refused was sampled on the same day each week when feed refusals were collected, and DM contents were determined. During three 5-day total collections (Weeks 4, 8 and 14), all faeces were collected several times daily while the animals were constrained to the section of the pen with concrete flooring. A 10% subsample of the daily faecal excretion was stored at −20°C, and at the end of the collection interval, these subsamples were mixed and subsampled. During other intervals, samples of the feeds offered and refused were bulked monthly. Feed and faeces samples were dried at 60°C, ground (1-mm screen) in a laboratory mill (Christie and Norris Mill, Chelmsford, UK), and retained for analysis. Urine samples (~30–60 mL) were collected by stimulation of the vulva on days immediately preceding and following the Week 14 total collection, acidified (pH <3) with 4-M sulphuric acid, and stored at −20°C for subsequent analyses.
Jugular blood samples were obtained at fortnightly intervals before measuring LW and before feeding. Samples were obtained using vacutainers (BD Diagnostics, Plymouth, UK) containing lithium heparin as an anticoagulant, chilled in iced water, centrifuged (3000g for 10 min) and the plasma stored at −20°C. Milk secretion was measured seven times at fortnightly intervals by mechanical milking (DeLaval Model DVP 170/340) following IV injection of 10 i.u. oxytocin (Ilium Syntocin) to enhance milk letdown; each cow was milked twice with a 4-h interval. Samples of milk for subsequent analyses of composition were stored at −20°C. Biopsies of the outer cortical bone of the 11th rib (Little 1972; Dixon et al. 2024) were obtained 7 ± 4 and 95 ± 15 days after parturition. The 11th rib was sampled in the present experiment to avoid potential error from a repeated sampling of the 12th rib (Little and Minson 1977; Little and Ratcliff 1979). Real-time ultrasound scanning (Esaote Pie Medical Aquila with a 3.5-MHz linear array transducer (Pie Medical Imaging, Maastricht, The Netherlands) was used to measure fat depth at the rib and P8 rump sites, and eye muscle area, near the commencement and the end of the experiment.
Laboratory procedures
Organic matter of feed offered, feed refused and faeces was determined as the DM minus ash measured by incineration (550°C for 8 h). Samples of feed offered and refused, faeces, and milk, were digested in a nitric-perchloric acid mixture, and the concentrations of P, Ca, Mg and S were analysed using an inductively coupled plasma spectrometer (Optima 7300 DV; Perkin Elmer, Waltham, MA, USA). Samples of feed offered were also analysed for total N, neutral detergent fibre, acid detergent fibre, lignin and crude fat by a commercial laboratory using near-infrared reflectance spectroscopy with inhouse calibrations (Dairy One, Ithaca, USA). The concentrations of fat, protein and lactose in milk were measured by mid-infrared spectroscopy (Australian Herd Recording Services, Tasmania). Rib bone biopsy samples were carefully scraped to remove trabecular bone before measuring cortical bone thickness using vernier callipers, and specific gravity by gravimetric procedures. Plasma samples were analysed calorimetrically for plasma inorganic P (Pi), total Ca and Mg using a Beckman Coulter Au680 analyser with OSR6122, OSR 6189 and OSR6189 assay kits, respectively. The concentration of P in urine was analysed as described by Goodwin (1970). Plasma concentrations of hormones and bone metabolism markers were determined using commercial assay kits in accordance with the manufacturer’s instructions. Assays performed were parathyroid hormone (PTH; A11930; Beckman Coutler), 25-hydroxy vitamin D3 and 1,25-dihydroxy vitamin D3 (AA-35F1 and AA-54F2, Immunodiagnostic Systems), carboxy-terminal telepeptides of type 1 collagen (CTX-1; Crosslaps AC-02F1; Immunodiagnostic Systems), bone alkaline phosphatase (BAP; MicroVue 8012; Quidel), total osteocalcin (OCN; MicroVue 8002; Quidel) and insulin-like growth factor 1 (IGF-1; A15729; Beckman Coulter).
Calculations and statistical analyses
The requirements of the cows for P and Ca, and the amounts ingested in each of the diets, were calculated assuming a true absorption coefficient of 0.70 for P, as recommended by CSIRO (2007). In addition, the amount of absorbed P provided in the HP-HCa diet was calculated assuming a true absorption coefficient of 0.90 for the mono dicalcium phosphate included in this diet (Dixon et al. 2020a). During late pregnancy the conceptus-free LW (CF-LW) of the cows was calculated from the actual date of parturition and subtraction of the estimated conceptus weight (scaled to a 30-kg calf birthweight) from the cow total LW (O’Rourke et al. 1991). The LW change of the cows was calculated as: (1) the difference between the LWs on Day 1 and Day 98, and also (2) the linear regression of LW with time from Days 35 to 98. The LW gains of the calves were calculated by linear regression of LW from birth to Day 98. Apparent digestibility of DM and organic matter were calculated by conventional procedures. The metabolisable energy (ME) content of the diets was calculated from the in vivo organic matter digestibility (OMD) as (CSIRO 2007):
Daily milk production was calculated from the secretion during the 4-h intervals measured fortnightly. The P secreted in milk was calculated from the amount and its P concentration. The gross energy (GE) content of milk was calculated as:
with the concentrations as g/kg units (CSIRO 2007). Urinary P excretion was calculated from the creatinine and P concentrations, and was negligible. The P retentions were calculated from the P intakes minus the excretion of P in faeces and milk. The concentration of P in the outer cortical rib bone was calculated as:
The PSACB (μg P/mm2) was calculated as the product of P concentration and thickness of the cortical bone (Dixon et al. 2019). To facilitate comparisons of the PSACB measured in the 11th rib with previous measurements in the 12th rib bone in the same cows, and with other published reports, the PSACB values were also calculated with adjustment for the lower PSACB in the 11th rib than in the 12th rib (ratio = 1.078; Dixon et al. 2020b).
Statistical analyses by ANOVA used Genstat (release 16.1, VSN International Ltd, Hemel Hemstead, UK) to compare among the treatments. The effects of animal blocks at allocation and potential covariates (initial cow LW, BCS and age, date of parturition, rib bone cortical bone thickness, P concentration, and PSACB measured during the previous experiment and at parturition) were examined and included when significant at P < 0.05. Where measurements were made at intervals (intake, digestibility, concentrations of Pi, Ca, Pi/Ca and endocrine markers), the effects of diet and time were examined in a repeated measures ANOVA with effects of diet, time and the diet × time interaction. The plasma concentrations of PTH, precurser 25-dihydroxy vitamin D3 and active 1,25-dihydroxy vitamin D3 were transformed (log10 (X + 1)) before analyses to address their high variability. Pair-wise comparisons between means were made using Fisher’s protected least significant difference test.
Results
Health of the cows and their performance during late pregnancy
During late pregnancy through to parturition, the cows on average gained 0.36 ± 26 kg total LW/day, but only maintained their maternal LW (−0.1 ± 0.28 kg CF-LW/day). The Pi of cows that had been fed high-P and low-P diets during mid-pregnancy averaged 2.1 and 1.0 mmol/L, respectively, at the end of the previous experiment (Dixon et al. 2024). During Weeks 24–34 of pregnancy, the cows previously fed the high-P diet and low-P diet averaged 2.16 ± 0.69 and 1.80 ± 0.83 mmol/L, respectively. During Weeks 34–40, the cows previously fed high-P diets decreased to an average of 1.74 ± 0.29 mmol/L, whereas the cows previously fed the low-P diets averaged 1.71 ± 0.19 mmol/L (Fig. 1). At the commencement of the introductory interval, the PSACB in the 12th rib averaged 532 ± 126 μg P/mm2. Following adjustment for the difference between the 11th and 12th ribs, the PSACB increased during the introductory interval to 615 ± 127 (337–849) μg P/mm2 at parturition; that is, a 15% increase.
The plasma inorganic P (Pi) concentrations in the cows during the last 16 weeks of pregnancy. The cows had been fed high-P () or low-P (
) diets during the previous experiment, and then all were fed a high-P diet during late pregnancy.
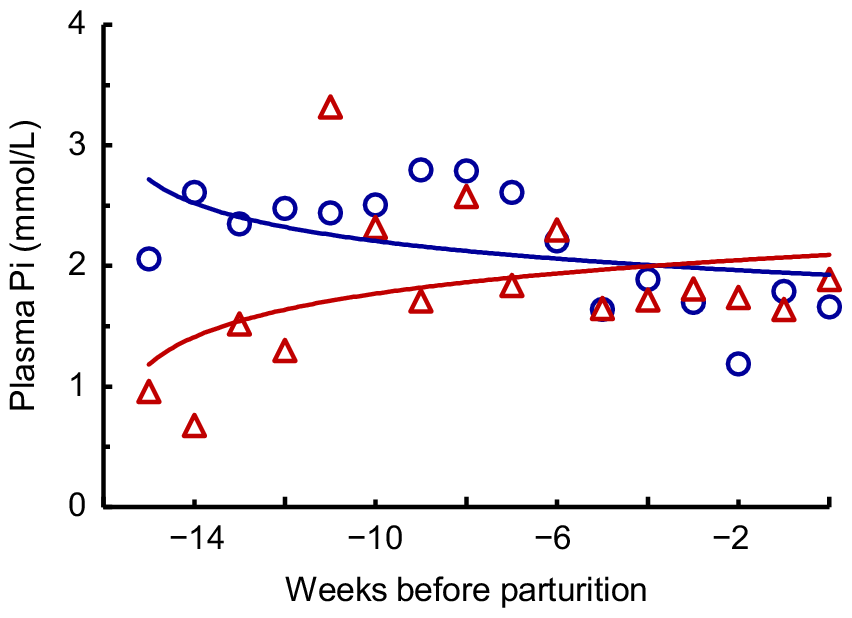
Two calves died of causes unrelated to the experiment, and these cow–calf pairs were removed from the experiment. For animal welfare reasons, one cow with low BCS was withdrawn from the experiment 1 week early, although the results for this cow–calf pair were included in the dataset. The cows and calves were otherwise in good health throughout the experiment, and no signs of osteomalacea, such as abnormal gait, were observed.
Intakes, organic matter digestibility and LW changes
The mean concentrations of P ranged from 0.52 to 0.57 g P/kg DM in the three LP diets, and 2.16 g P/kg DM in the HP-HCa diet (Table 1). The diet Ca/P ratios were 2.2, 3.0, 5.5 and 2.9 in the HP-HCa, LP-LCa, LP-HCa and LP-LCa+ac diets, respectively. When the conservative true absorption coefficient of 0.70 (CSIRO 2007) was adopted, the HP-HCa diet provided 1.05 of the calculated P requirements of the cows (Table 2). However, when a higher true absorption coefficient of 0.90 was adopted for the mono dicalcium phosphate (Dixon et al. 2020a), the HP-HCa diet was estimated to provide 1.27 of the absorbed P requirements. The three LP diets provided only 0.26–0.32 of the calculated P requirements.
Measurement | Diet treatment | cov | s.e.m. | Probability | ||||||||
---|---|---|---|---|---|---|---|---|---|---|---|---|
HP-HCa | LP-LCa | LP-HCa | LP-LCa+ac | Diet (d.f. 19) | Time (d.f. 46) | D × T (d.f. 51) | Diet | Time | D × T | |||
Total P, intake and requirements A | ||||||||||||
Intake (g P/day) | 23.1 | 4.7 | 4.1 | 4.8 | – | – | – | – | – | – | – | |
Requirement (g P/day) | 22.1 | 14.5 | 13.5 | 18.7 | – | – | – | – | – | – | – | |
Intake/requirement | 1.05 | 0.32 | 0.31 | 0.26 | – | – | – | – | – | – | – | |
Absorbed P, intake and requirements A | ||||||||||||
Intake (g P/day) | 19.6 | 3.3 | 2.9 | 3.3 | – | – | – | – | – | – | – | |
Requirement (g P/day) | 15.5 | 10.1 | 9.4 | 13.1 | – | – | – | – | – | – | – | |
Intake/requirement | 1.27 | 0.32 | 0.31 | 0.26 | – | – | – | – | – | – | – | |
Intake and digestibility | ||||||||||||
n | 8 | 7 | 8 | 7 | – | – | – | – | – | – | – | |
DM intake (kg/day) B | 10.7a | 8.2b | 7.9b | 9.0b | 1, 2 | 0.49 | 0.28 | 0.67 | <0.01 | 0.01 | n.s. | |
DM intake (g/kg LW.day) B | 23.1a | 18.3bc | 17.4c | 19.6b | 0.55 | 0.64 | 1.35 | <0.001 | <0.001 | n.s. | ||
OM digestibility (g/kg) | ||||||||||||
Week 4 | 699 | 693 | 698 | 727 | 1, 2 | 18 | – | – | 0.09 | – | – | |
Week 8 | 705 | 679 | 681 | 642 | – | 14 | – | – | 0.10 | – | – | |
Week 14 | 671 | 653 | 643 | 660 | – | 24 | – | – | n.s. | – | – | |
Mean | 692 | 674 | 674 | 676 | – | 13 | 9 | 13 | n.s. | <0.01 | n.s. | |
ME content (MJ ME/kg DM) | 9.73 | 9.40 | 9.47 | 9.44 | – | 0.22 | – | – | n.s. | – | – | |
ME intake (MJ ME/day) C | 98.8a | 69.5c | 69.9c | 80.6b | 2 | 3.3 | – | – | <0.001 | – | – | |
ME intake (kJ ME/kg LW.day) C | 210a | 161b | 158b | 175b | – | 6.4 | – | – | <0.001 | – | – | |
Cow liveweight | ||||||||||||
Day 1 (kg) | 459 | 483 | 483 | 473 | – | 10.3 | – | – | n.s. | – | – | |
Day 98 (kg) | 474 | 433 | 449 | 456 | 1,24 | 10.5 | – | – | <0.01 | – | – | |
Change, days 1–98 (kg) | +15c | −50a | −34a | −14a | 3, 4 | 10.1 | – | – | <0.001 | – | – | |
Change, days 1–98 (kg/day) | +0.15a | −0.51b | −0.35b | −0.14a | 3, 4 | 0.115 | – | – | <0.001 | – | – | |
Change, Regn (kg/day) D | +0.45a | −0.23b | −0.20b | +0.20a | 3, 4 | 0.106 | <0.001 | |||||
Cow body condition score | ||||||||||||
Initial | 3.4 | 3.8 | 3.4 | 3.4 | – | 0.22 | – | – | – | – | – | |
Final | 3.5 | 2.5 | 2.3 | 2.6 | – | 0.24 | <0.01 | |||||
Change | +0.1a | −1.2b | −1.1b | −0.8b | 5 | 0.22 | – | – | <0.01 | – | – |
The means adjusted for covariates of the measurements voluntary intake; organic matter digestibility during three total collection intervals after 4, 8 and 14 weeks of lactation; cow liveweight changes; and in lactating cows fed four diets are given. The diets comprised High P-High Ca, Low P-Low Ca, Low P-High Ca and Low P-Low Ca with the addition of ammonium chloride (HP-HCa, LP-LCa, LP-HCa, LP-LCa+ac).
Means within rows with different lowercase letters are different at P < 0.05. Covariates (cov): 1, calving date; 2, initial cow liveweight; 3, carry-over effect of P treatment in the previous experiment; 4, age of cow; 5, initial cow body condition score.
The average LW of the cows measured shortly after parturition was 474 ± 57 kg (Table 2). During the first 3 weeks of lactation, the cows fed the HP-HCa, LP-LCa and LP-HCa diets lost 22–29 kg LW, and those fed the LP-LCa+ac treatment lost 56 kg, before the cows in each treatment had regained much of this LW (Fig. 2a). By Week 5, the LW of cows on each diet had stabilised to 11–32 kg lower than the LW immediately after parturition. Voluntary intakes were low in Week 1 (10.9–13.0 g DM/kg LW.day), increased during Weeks 2–4 and then stabilised (Fig. 2b). The inclusion of additional straw during Days 1–4 to reduce the risk of acidosis likely contributed to the low initial intakes. The average voluntary intakes from Weeks 5–14 were 23.1 g DM/kg LW.day in the HP-HCa cows, but were lower (P < 0.05) in the LP diet cows (17.4–19.6 g DM/kg LW.day; Table 2). Also, the voluntary intake was lower in the LP-HCa cows than in the LP-LCa+ac cows (P < 0.05). The losses and regains in LW during the first 4 weeks were likely due primarily to the lower feed intakes and changes in digesta load in the gastrointestinal tract and in body fluids. The changes in LW between Days 1 and 98 should have provided the most reliable measure for the entire experimental interval, and these indicated that the cows fed the HP-HCa diet gained on average 0.15 kg/day, whereas the cows fed the three LP diets lost LW (−0.14 to −0.51 kg LW/day; Table 2). In contrast, the LW change calculated by regression from Weeks 5–14 indicated that after adaptation, the HP-HCa cows gained 0.45 kg/day, the LP-LCa+ac cows gained 0.20 kg/day, and the LP-LCa and LP-HCa cows lost LW slowly (−0.23 and −0.20 kg/day). The HP-HCa cows maintained BCS, whereas the cows fed each of the LP diets lost 0.8–1.2 BCS units. The fat thickness at the P8 rump and rib sites decreased during the 14 weeks by 0.7–2.1 mm and 0.1–0.4 mm, respectively (data not shown). The HP-HCa cows gained 4.9 mm2 eye muscle area, whereas the changes with the LP diets ranged from −1.3 to +1.7 mm2. There were no significant differences (P > 0.05) in these attributes among the diets.
(a) Liveweights and (b) voluntary intakes (g DM/kg LW.day) of mature cows fed ad libitum on four diets with high P (HP-HCa () or LP-LCa (
), low P (LP-HCa (
) and LP-LCa+ac (
) during lactation.
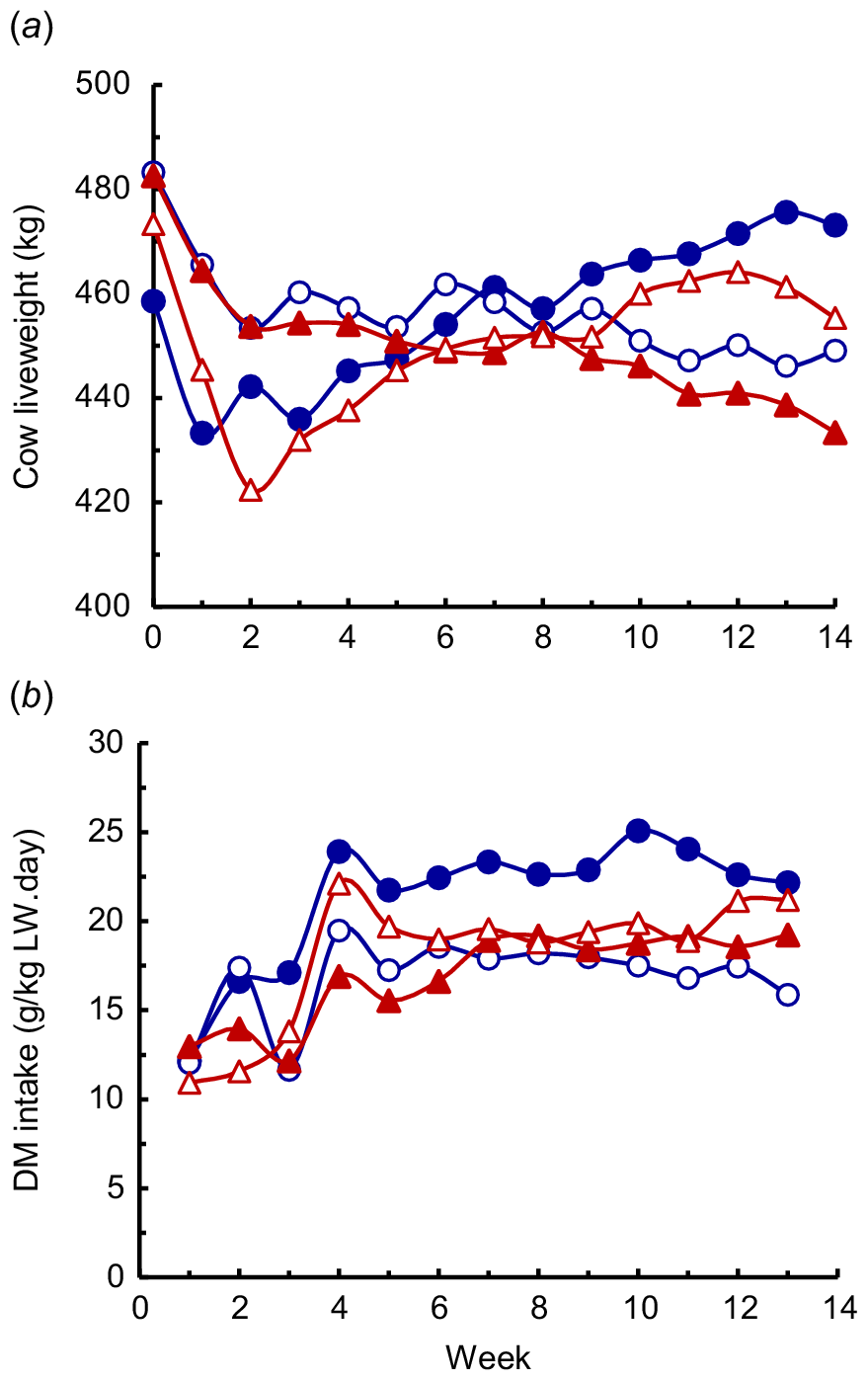
The organic matter digestibility (mean 679 g/kg) did not differ among the diets, but was higher (P < 0.05) during Week 4 (704 g/kg) than during Weeks 8 and 14 (676 and 657 g/kg, respectively; Table 2). Intake of metabolisable energy was higher (P < 0.001) in the HP-HCa diet cows than the three LP diets (210 vs 158–175 kJ ME/kg LW.day).
Milk production, calf birth weight and calf growth
Milk production averaged 5.40 kg/day (Table 3). At Week 2 of lactation, the milk production of the LP-LCa cows (7.61 kg/day) was higher (P < 0.05) than in the other two LP diets (4.12 and 4.35 kg/day in the LP-HCa and the LP-LCa+ac diets; Fig. 3), whereas the amount of milk secreted by the cows fed the HP-HCa diet (5.73 kg/day) did not differ from the other diets. Throughout the 14 weeks of the experiment, the milk production changed little in the HP-HCa cows, but decreased linearly (P < 0.05) in the LP-LCa cows. However, in the LP-HCa cows, milk production increased from Weeks 2 to 4, and then declined linearly from Weeks 4 to 14, and in the LP-LCa+ac cows increased from Weeks 2 to 8, and thereafter declined linearly. The rates of decrease in milk secretion from the respective peaks in the cows fed the LP diets were greater (P < 0.05) in the LP-LCa and LP-LCa+ac cows (−0.26 and −0.31 kg/week) than in the LP-HCa diet (−0.17 kg/week).
Measurement | Diet treatments | s.e.m. (d.f. 19) | Probability | ||||||
---|---|---|---|---|---|---|---|---|---|
HP-HCa | LP-LCa | LP-HCa | LP-LCa+ac | Diet | Time | D × T | |||
n | 8 | 7 | 8 | 7 | – | – | – | – | |
Production (kg/day) | 5.81 | 5.93 | 4.57 | 5.31 | 0.46 | n.s. | <0.05 | <0.05 | |
(MJ/day) | 18.1 | 17.7 | 12.6 | 15.3 | 1.43 | 0.05 | <0.05 | 0.05 | |
(g P/day) | 5.29 | 5.11 | 4.00 | 4.59 | 0.39 | n.s. | <0.05 | 0.08 | |
Milk composition | |||||||||
Fat (g/kg) | 41.5a | 40.5ab | 32.2c | 36.8bc | 2.15 | <0.05 | <0.001 | n.s. | |
Protein (g/kg) | 26.7a | 24.0b | 24.0b | 26.4a | 1.11 | n.s. | <0.05 | <0.05 | |
Lactose (g/kg) | 51.1 | 50.4 | 51.3 | 51.4 | 0.45 | n.s. | n.s. | n.s. | |
Ca (g/kg) | 1.23 | 1.22 | 1.23 | 1.24 | 0.024 | n.s. | 0.10 | n.s. | |
P (g/kg) | 0.90 | 0.87 | 0.86 | 0.85 | 0.019 | n.s. | 0.05 | n.s. | |
Mg (g/kg) | 0.096 | 0.093 | 0.095 | 0.095 | 0.0034 | n.s. | <0.01 | n.s. | |
Energy (MJ/kg) | 3.08a | 2.96a | 2.66b | 2.90ab | 0.096 | <0.05 | <0.001 | n.s. | |
Calf growth | |||||||||
Birthweight (kg) A | 27.8 | 31.9 | 29.9 | 31.1 | 1.64 | n.s. | – | – | |
Liveweight at 98 days (kg) | 96.7 | 95.1 | 86.2 | 92.8 | 5.2 | n.s. | – | – | |
Liveweight gain (kg/day) | 0.70 | 0.66 | 0.59 | 0.63 | 0.048 | n.s. | – | – |
The calf birthweights and growth are given. The diets comprised High P-High Ca, Low P-Low Ca, Low P-High Ca and Low P-Low Ca with the addition of ammonium chloride (HP-HCa, LP-LCa, LP-HCa, LP-LCa+ac). Means within rows with different lowercase letters are different at P < 0.05.
D × T, diet × time interaction.
Milk production measured at fortnightly intervals in cows fed the four diets comprising HP-HCa (), LP-LCa (
), LP-HCa (
) and LP-LCa+ac (
). The linear decreases in milk secretion from Week 2 of lactation in cows fed HP-HCa and the LP-LCa cows, and from the peak milk secretion in the cows fed LP-HCa and LP-LCa+ac diets, were as follows: HP-HCa, Weeks 2–14, Y = 5.84 − 0.005X (R2 = 0.002); LP-LCa, Weeks 2–14, Y = 8.02 − 0.26X (R2 = 0.94); LP-HCa, Weeks 4–14, Y = 6.20 − 0.17X (R2 = 0.97); and LP-LCa+ac, Weeks 8–14, Y = 8.90 − 0.31X (R2 = 0.99). The slopes of these linear regressions of the HP-HCa, LP-LCa and LP-HCa diets differed (P < 0.05), but the LP-LCa was not different to the LP-LCa+ac diet.
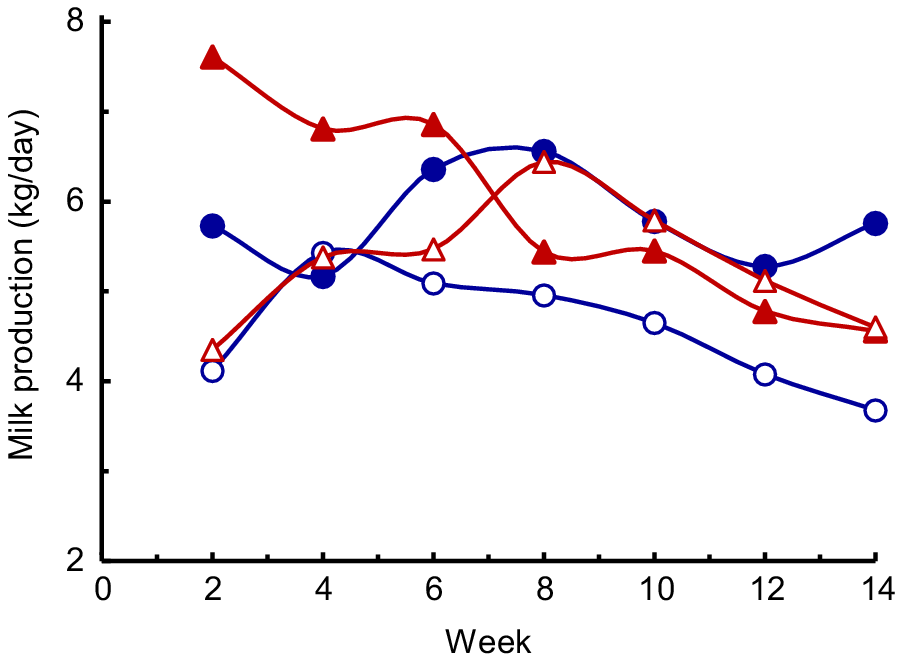
Milk energy content (mean 2.90 MJ/kg) was affected by the diet (P < 0.05) and decreased with time from 3.28 to 2.84 MJ/kg (P < 0.001). The secretion of milk energy also decreased with the declines in milk production, and in the cows fed the LP diets, the declines in milk energy were greater than in the amount of milk (28 and 15%, respectively). Milk P concentration was not affected by diet, but tended (P = 0.05) to decrease from 0.93 to 0.87 g P/kg from Week 2 to Week 14 during lactation. Milk P secretion (g P/day) was closely related to the amount of milk secreted (kg/day) and was described by the equation:
Birthweight of the calves averaged 30.2 ± 5.2 kg (Table 3). Calf growth rates were well described by the regression of LW with time (R2 ≥ 0.97), averaged 0.64 kg/day and were not affected (P > 0.05) by the diets. Calf growth rate was only moderately correlated with the milk energy production (r = 0.61–0.68) measured by the fortnightly machine milking.
Phosphorus intakes, faecal excretion and retention
During the total collection intervals, the mean P intake and faecal P excretion were higher for the HP-HCa diet (25.2 and 15.7 g P/day, respectively) than for each of the three LP diets (mean intakes 4.0–5.0 g P/day and faecal excretion 6.0–7.5 g P/day; P < 0.001; Table 4). This was expected, given the differences in dietary P concentrations. The intakes of P minus P in faeces in the three total collection intervals averaged +9.1 g P/day in the HP-HCa cows and −1.7 to −2.6 g P/day in the three LP diets. Furthermore, the P intake minus P in faeces in the HP-HCa cows during Week 14 (+5.1 g P/day) was lower (P < 0.05) than during Weeks 4 and 8 (+10.5 and +12.1 g P/day, respectively). Urinary excretion of P was negligible, averaging ≤0.05 g P/day in the LP diet cows and 0.21 g P/day in the HP-HCa cows.
Measurement | Diet treatments | cov | s.e.m. | Probability | ||||||||
---|---|---|---|---|---|---|---|---|---|---|---|---|
HP-HCa | LP-LCa | LP-HCa | LP-LCa+ac | Diet (D) (d.f. 19) | Time (T) (d.f. 46) | D × T (d.f. 51) | Diet | Time | D × T | |||
n | 8 | 7 | 8 | 7 | – | – | – | – | – | – | ||
P intake (g P/day) | ||||||||||||
Week 4 | 23.4a | 3.2b | 5.9b | 4.4b | 3,5 | 0.98 | – | – | <0.001 | – | – | |
Week 8 | 27.3a | 4.7b | 4.2b | 5.6b | 6 | 0.92 | – | – | <0.001 | – | – | |
Week 14 | 24.2a | 4.5b | 3.2b | 4.4b | – | 1.17 | – | – | <0.001 | – | – | |
Mean | 25.2a | 4.3b | 4.0b | 5.0b | 6 | 0.77 | 0.48 | 0.87 | <0.001 | n.s. | n.s. | |
P in faeces (g P/day) | ||||||||||||
Week 4 | 13.2aA | 6.3b | 6.3b | 7.5b | – | 0.64 | – | – | <0.001 | – | – | |
Week 8 | 15.0aB | 5.7b | 6.8b | 8.1b | 5,6 | 0.91 | – | – | <0.001 | – | – | |
Week 14 | 19.1aB | 6.7b | 4.8b | 6.6b | – | 1.89 | – | – | <0.001 | – | – | |
Mean | 15.7a | 6.3b | 6.0b | 7.5b | – | 0.96 | 0.56 | 1.32 | <0.001 | n.s. | <0.05 | |
P (intake – faecal excretion) (g P/day) | ||||||||||||
Week 4 | +10.5aB | −2.1b | −1.8b | −2.9c | – | 1.07 | – | – | <0.001 | – | – | |
Week 8 | +12.1aB | −1.6b | −2.1b | −2.8b | – | 1.23 | – | – | <0.001 | – | – | |
Week 14 | +5.1aA | −2.1b | −1.6b | −2.3b | – | 1.14 | – | – | <0.001 | – | – | |
Mean | +9.1a | −1.7b | −1.9b | −2.6b | 6 | 0.70 | 0.62 | 1.24 | <0.001 | n.s. | <0.05 | |
P in milk (g P/day) | ||||||||||||
Week 4 | 4.8ab | 6.3aB | 4.2b | 4.4b | 1 | 0.49 | – | – | <0.05 | – | – | |
Week 8 | 5.2 | 5.1A | 4.4 | 4.9 | 1,5 | 0.39 | – | – | n.s. | – | – | |
Week 14 | 4.5 | 3.9A | 3.7 | 4.6 | 1,4 | 0.49 | – | – | n.s. | – | – | |
Mean | 5.0 | 5.0 | 4.0 | 4.6 | 1,4 | 0.34 | 0.19 | 0.52 | n.s. | <0.01 | <0.05 | |
P retention (g P/day) | ||||||||||||
Week 4 | +6.0aB | −9.1b | −5.8b | −7.5b | 1,2,3 | 1.17 | – | – | <0.001 | – | – | |
Week 8 | +6.4aB | −6.6c | −6.2c | −7.7c | – | 1.12 | – | – | <0.001 | – | – | |
Week 14 | −0.6bA | −6.2c | −4.9c | −6.4c | – | 1.28 | – | – | <0.01 | – | – | |
Mean | +3.6b | −6.0a | −5.7a | −7.1a | – | 0.81 | 0.59 | 1.26 | <0.001 | n.s. | <0.01 | |
Means of the variables measured at the three intervals (mg P/kg LW.day) | ||||||||||||
P intake | 54.8a | 10.3b | 9.4b | 10.4b | – | 1.3 | – | – | <0.001 | n.s. | n.s. | |
P faeces | 34.9a | 14.1b | 13.6b | 16.6b | – | 1.9 | – | – | <0.001 | n.s. | <0.05 | |
P (intake – faeces) | +21.3a | −3.8b | −4.3b | −6.3b | – | 1.4 | – | – | <0.001 | – | – | |
P milk | 10.7 | 10.9 | 8.7 | 10.0 | – | 0.8 | 0.4 | 1.2 | <0.001 | n.s. | <0.05 | |
P retention, Week 4 | +10.4aB | −18.8b | −12.9b | −16.5b | – | 2.4 | – | – | <0.001 | – | – | |
P retention, Week 8 | +13.6aB | −13.3b | −14.1b | −17.4b | – | 2.5 | – | – | <0.001 | – | – | |
P retention, Week 14 | +0.8aA | −14.3b | −9.2b | −14.2b | – | 2.6 | – | – | <0.01 | – | – | |
P retention | +8.3c | −15.5ab | −12.1b | −16.5a | – | 1.56 | 1.28 | 2.61 | <0.001 | n.s. | <0.05 |
The diets comprised High P-High Ca, Low P-Low Ca, Low P-High Ca and Low P-Low Ca with the addition of ammonium chloride (HP-HCa, LP-LCa, LP-HCa, LP-LCa+ac). P retention was calculated as the (P intake – P in faeces – in P milk).
Means within rows with different lowercase letters are different at P < 0.05.
Means within columns with different upper case letters (A and B) are different at P < 0.05.
cov, covariates: 1, carry-over effect of P treatment in the previous experiment; 2, carry-over effect of energy treatment in the previous experiment; 3, calving date; 4, age of cow; 5, initial cow body condition score; 6, initial cow liveweight.
D × T, diet × time interaction.
The P retention (dietary P – faecal P – milk P) was affected by the dietary P concentrations (P < 0.001) and there was a diet × time interaction (P < 0.01; Table 4). Cows fed the HP-HCa diet retained 6.0 and 6.4 g P/day (10.4 and 13.6 mg P/kg LW.day) during the Week 4 and 8 total collections, but retained negligible P (−0.6 g P/day) during the Week 14 total collection. Thus, during the first 8 weeks of lactation, the HP-HCa cows were ingesting sufficient P to provide for their maintenance, growth and milk, and to also replenish 6.2 g body P/day (12.0 mg P/kg LW.day). However, by Week 14, the P replenishment in these HP-HCa cows was apparently complete, and surplus dietary P was being excreted in faeces. During lactation the LW change of the HP-HCa diet cows (Y; kg/day) was related to the P retention (X; g P/day) as follows:
In contrast to the HP-HCa cows, the LP cows exhibited negative P retentions in each of the three total collection intervals, ranging from −4.9 to −9.1 g P/day, and −9.2 to −18.8 mg P/kg LW.day (means −5.7 to −7.1 g P/day and −12.1 to −16.5 mg P/kg). Thus these P-deficient LP diet cows were mobilising substantial body P. During the Week 4 total collection, the LP-LCa cows secreted more P in milk (6.3 g P/day) (P < 0.05) than the cows fed the other two LP diets (4.2 and 4.4 g P/day). However, these LP-LCa cows were not able to maintain this secretion, and the the amount of milk P decreased to 5.1 and 3.9 g P/day during the Week 8 and 14 total collections, respectively (Table 4). These results suggest that the maximum P mobilisation in the cows was initially ~9.1 g P/day (18.8 mg P/kg LW.day), and decreased to 6.2 g P/day (14.3 mg P/kg LW.day) as lactation progressed and body P reserves declined in these LP-LCa cows. There was no such decline in the amounts of P mobilised through the 14 weeks of lactation in the LP-HCa and LP-LP-LCa+ac cows. There was a tendency, albeit not statistically significant, for the inclusion of ammonium chloride in the diet to increase P mobilisation.
The secretion of milk P by the cows fed the three LP diets, and also in the cows fed the HP-HCa diet, was related to P retention with separate relationships for the cows fed the low P and high P diets (Fig. 4a). These regressions indicated that in the P deficient cows, 57% of mobilised body P was secreted as milk P. Among cows ingesting the HP-HCa diet that provided in excess of their P requirements, milk P secretion decreased by 24% of the increases in P retention. Phosphorus in excess of the P requirements was secreted as milk P. As there was little variation in the concentration of milk P, this regression also indicated the close associations between P retention and the amounts of milk produced. Also, as expected, the growth of calves sucking cows fed the LP diets (kg/day) was negatively related to the P retention (g P/day) (P < 0.05), whereas the growth of calves sucking cows fed the HP-HCa diet tended to be positively related to P retention (Fig. 4b).
The relationships between mean milk P production and mean P retention (a) in cows fed the HP-HCa diet (), or the three LP diets (LP-LCa (
), LP-HCa (
) and LP-LCa+ac (
)). The relationships were: HP-HCa diet Y = 6.12 − 0.24X (n = 8, r = 0.68, P > 0.05); the three LP diets Y = 0.75 − 0.57X (n = 24, r = 0.78, P < 0.01). The relationships between mean calf growth and mean P retention (b) in cows fed these diets were: HP-HCa diet; Y = 0.62 + 0.018X (n = 8, r = 0.56, n.s); the three LP diets Y = 0.42 − 0.31X (n = 21, r = 0.49, P < 0.05).
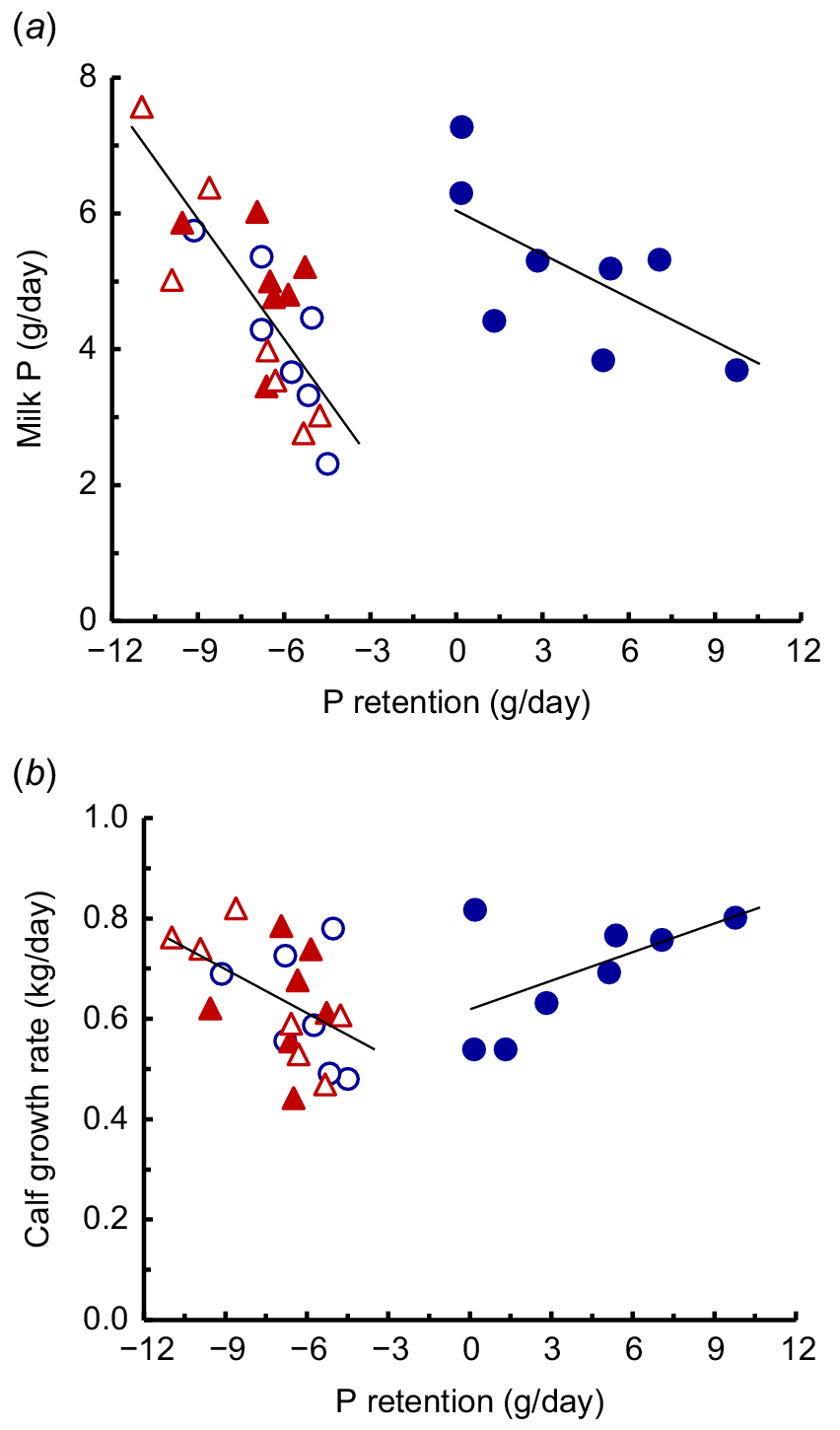
Changes in rib bone
In the cows fed the HP-HCa diet, there was a large increase during lactation in the PSACB that was equivalent to an increase from 634 to 729 μg P/mm2 (15%) in the 12th rib (Table 5). This increase in bone during lactation was in addition to the approximate 15% increase in bone during the introductory interval when the cows were in late pregnancy, as described above. In contrast, the LP-HCa cows tended to increase PSACB by only 6.9% during lactation, whereas the PSACB decreased in the LP-LCa and LP-LCa+ac diets by −4.1 and −13.2%, respectively. In general, the changes in PSACB in the LP diet cows were associated with decreases in rib cortical bone P concentration rather than in the cortical bone thickness. Overall, the results indicated that the HP-HCa cows gained substantial rib cortical bone, the LP-HCa cows gained a small amount of bone, and the LP-LCa and LP-LCa+ac cows lost bone. The PSACB also indicated that by Week 14 of lactation, six out of eight of the HP-HCa cows were adequate, and two out of eight were marginal in their bone mineral reserves and P status. In contrast, 10 out of 22, seven out of 22 and five out of 22 of the LP cows were classed as deficient, marginal and adequate, respectively, in bone mineral reserves.
Measurement | Diet treatments | cov A | s.e.m. (d.f. 21) | Prob | ||||
---|---|---|---|---|---|---|---|---|
HP-HCa | LP-LCa | LP-HCa | LP-LCa+ac | |||||
n | 8 | 7 | 8 | 7 | – | – | – | |
Cortical bone thickness (mm) | ||||||||
Initial | 3.79 | 3.69 | 3.99 | 3.39 | – | 0.231 | n.s. | |
Final | 4.40a | 3.72b | 4.02ab | 3.79b | 1 | 0.162 | <0.05 | |
Change | +0.73b | +0.01a | +0.35ab | +0.09a | 1 | 0.173 | <0.05 | |
Change (%) | 23.3b | 3.2a | 12.3ab | 1.9a | 1 | 4.51 | <0.01 | |
P concentration of cortical bone (mg P/cc) | ||||||||
Initial Pconc (mg P/cc) | 155 | 147 | 155 | 153 | – | 4.52 | n.s. | |
Final Pconc (mg P/cc) | 153b | 142ab | 140a | 135a | – | 3.86 | <0.05 | |
Change P conc (mg P/cc) | −0.8b | −9.6ab | −13.5a | −19.4a | 2 | 4.15 | <0.05 | |
Change P conc (%) | −0.1b | −6.4ab | −8.1a | −12.5a | 2 | 2.81 | <0.05 | |
PSACB (μg P/mm2) | ||||||||
Initial | 587 | 541 | 624 | 517 | – | 41 | n.s. | |
Final | 676c | 525ab | 577b | 491a | – | 22 | <0.001 | |
Change | +110c | −42b | +4ab | −73a | 3 | 24 | <0.001 | |
Change (%) | +22.8c | −4.1a | +6.9b | −13.2a | 3 | 3.8 | <0.001 |
The cows were fed ad libitum on diets comprising High P-High Ca, Low P-Low Ca, Low P-High Ca and Low P-Low Ca with addition of ammonium chloride (HP-HCa, LP-LCa, LP-HCa, LP-LCa+ac). The measurements were cortical bone thickness, concentration of P in outer cortical bone and PSACB in the 11th rib bone. The PSACB in the 12th rib was estimated as 1.078 times these values for the 11th rib. Means within rows with different lowercase letters are different at P < 0.05.
There was a wide range in the PSACB of the 11th rib at parturition (569 ± 119 (312–786) μg P/mm2), and the changes in PSACB through lactation were linearly related to the PSACB at parturition (Fig. 5). Furthermore, there were separate relationships for the cows fed the three LP diets and the HP-HCa diet. The elevation of the regression line for the cows fed the HP-HCa diet was higher (P < 0.05) than that of the cows fed the LP treatments, but the slopes did not differ. The LP diet cows with PSACB >650 μg P/mm2 at parturition lost on average 18% of their PSACB, whereas the cows with PSACB <450 μg P/mm2 increased their PSACB by on average 25%. The HP-HCa cows gained on average 19 ± 23 μg P/mm2, and there was the same pattern where the cows with PSACB <550 μg P/mm2 at parturition gained 31–44%, and the cows with PSACB >650 μg P/mm2 at parturition lost 7% of their PSACB. In addition, in these HP-HCa cows (n = 8), the change in PSACB during lactation was positively correlated with cow LW change (r = 0.80, P < 0.05) and with P retention (r = 0.73, P < 0.05).
The relationships between the change in P per unit surface area (PSACB) in rib cortical bone and the PSACB at parturition, during the 14 weeks of lactation in cows fed the HP-HCa diet () or the three LP diets (LP-LCa (
), LP-HCa (
) and LP-LCa+ac (
)). The relationships were as follows: The HP-HCa diet Y = 685 − 1.017X (n = 7, r = 0.89, P > 0.01); the three LP diets Y = 340 − 0.668X (n = 21, r = 0.85, P < 0.001). The elevation of the regression line for the cows fed the HP-HCa diet was higher (P < 0.05) than that of the cows fed the LP treatments.

Plasma P, Ca and Mg
During lactation, there were effects of diet, time and diet × time (P < 0.001) on Pi (Fig. 6a). The HP-HCa cows maintained Pi to an average of 1.49 mmol/L during the 14 weeks. However, the Pi in LP-LCa and LP-HCa cows decreased sharply by Week 2, and continued to decline slowly to an average of 0.37–0.46 mmol/L from Weeks 12 to 14. The Pi of the LP-LCa+ac cows declined more slowly than in the other two LP diets, was higher (P < 0.05) than in the LP-LCa cows from Weeks 2 to 8 and averaged 0.48 mmol/L from Weeks 12 to 14. In addition, the average Pi was related to the P retention (P < 0.001; Fig. 7).
The plasma concentrations of (a) inorganic P (Pi), (b) total Ca and (c) the Pi/Ca ratio in cows fed four diets comprising HP-HCa (), LP-LCa (
), LP-HCa (
) and LP-LCa+ac (
) during lactation. (a) The diet × time interaction was significant (P < 0.01) and the least significant differences (l.s.d.; 5%) = 0.43. (b) Both diet and time were significant (P < 0.01 and P < 0.001) and the l.s.d. (5%) = 0.10 and 0.07, respectively. (c) The diet × time interaction was significant (P < 0.01) and the l.s.d.(5%) = 2.01.
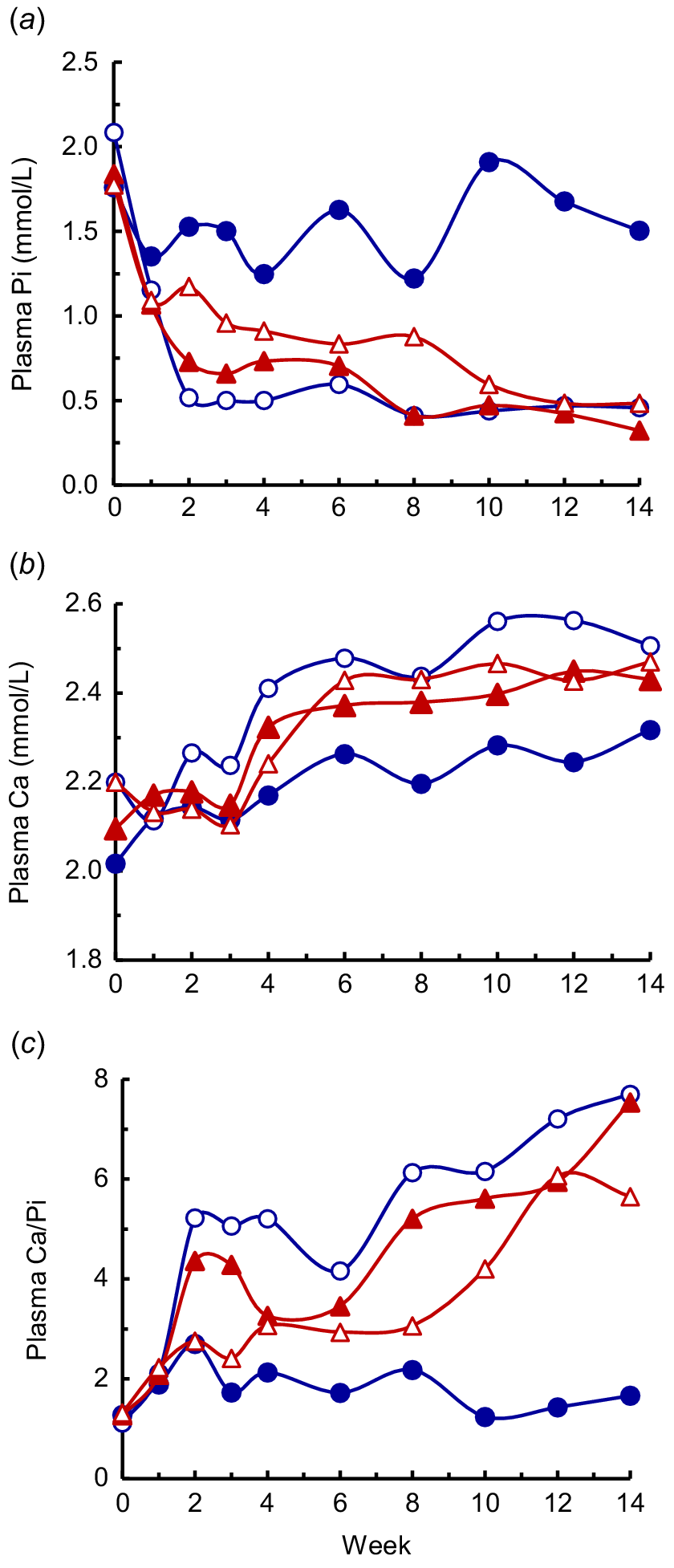
The relationship between the mean plasma Pi and mean P retention in the cows fed the HP-HCa diet (), or the three LP diets LP-LCa (
), LP-HCa (
) and LP-LCa+ac (
). The relationship was as follows: Y = 1.14 − 0.059X (n = 29, r = 0.78, P > 0.001).
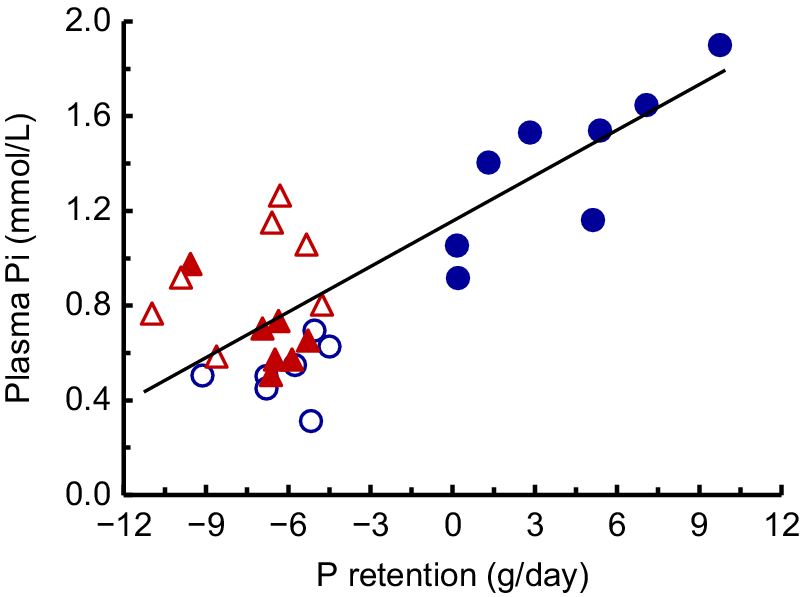
The plasma Ca concentrations (Fig. 6b) were affected by both diet and time (P < 0.01 or lower), but not by the diet × time interaction. Plasma Ca was 2.13 mmol/L immediately after parturition, and increased progressively in each of the diets during lactation to an average of 2.32 mmol/L in the HP-HCa cows and 2.43–2.51 mmol/L in the LP cows at Week 14 (P < 0.05; Fig. 6b). However, there were no consistent differences between the LP diets. Plasma Mg concentrations (data not shown) were not affected by diet, but increased (P < 0.001) from 0.80 mmol/L at Week 1 to 0.92 mmol/L at Week 14.
The plasma Ca/Pi ratio (Fig. 6c) accentuated the differences between the diets in Pi and Ca during P deficiency. There were effects of diet, time and the diet × time interaction (P < 0.01 or lower) during lactation. The Ca/Pi ratio in the HP-HCa cows was stable and averaged 1.8 during the experiment. The Ca/Pi ratio increased in each of the LP diets (P < 0.05) as lactation progressed, although more rapidly in the LP-LCa and LP-HCa cows than the LP-LCa+ac cows. From Week 2, the Ca/Pi ratio was generally higher (P < 0.05) in the LP-HCa and LP-LCa cows than the HP-HCa cows, and was higher in all the LP diet cows than in the HP-HCa cows during Weeks 10–14. From Weeks 10 to 14, the Ca/Pi ratio in the three LP diets averaged 6.4, and was almost five times that in the HP-HCa cows.
Plasma endocrine and bone markers
Plasma PTH concentrations in the various treatments were affected by both the diet and time (P < 0.001), but there was no diet × time interaction (P < 0.05; Fig. 8a). Across all of the diets, the PTH decreased progressively, averaging 208 pg/mL shortly after parturition to 131 pg/mL during Week 1–3, then decreasing (P < 0.05) to an average of 71 pg/mL during Week 4–14. The concentrations in the LP-HCa cows (mean 35 pg/mL) were lower (P < 0.05) than in the other three diets (mean 97 pg/mL). Plasma concentrations of the precursor 25-dihydroxy vitamin D3 (data not shown) did not differ between the treatments (P > 0.05), averaged 105 ng/mL shortly at parturition and decreased (P < 0.001) to 85 ng/mL at Week 2. The concentration of active 1,25-dihydroxy vitamin D3 (Fig. 8b) averaged 245 pg/mL shortly after parturition, and was affected by diet, time and the diet × time interaction (P < 0.05 or lower). Active 1,25-dihydroxy vitamin D3 concentrations in the HP-HCa cows declined during the experiment, being lower (P < 0.05) after 8 weeks than during Weeks 1–3, and decreased further by Week 14. During Weeks 1–8 the 1,25-dihydroxy vitamin D3 concentrations did not differ between the HP-HCa, LP-LCa and LP-HCa cows, whereas concentrations in the LP-LCa+ac cows were higher than in the HP-HCa cows at Weeks 2, 8 and 14. At Week 14, the 1,25-dihydroxy vitamin D3 concentrations in cows fed each of the LP diets was higher (P < 0.05) than the cows fed the HP-HCa diet.
The plasma concentrations of (a) parathyroid hormone (PTH) and (b) 1,25 dihydroxy vitamin D3 in cows fed four diets comprising HP-HCa (), LP-LCa (
), LP-HCa (
) and LP-LCa+ac (
) during lactation. Following log10 transformation of the PTH in (a) the diet and time were significant (both P < 0.001) and the l.s.d. (5%) = 0.25 and 0.27, respectively. Following log10 transformation of the 1,25 dihydroxy vitamin D3 in (b) the diet × time interaction was significant (P < 0.05) and the l.s.d. (5%) = 0.22.
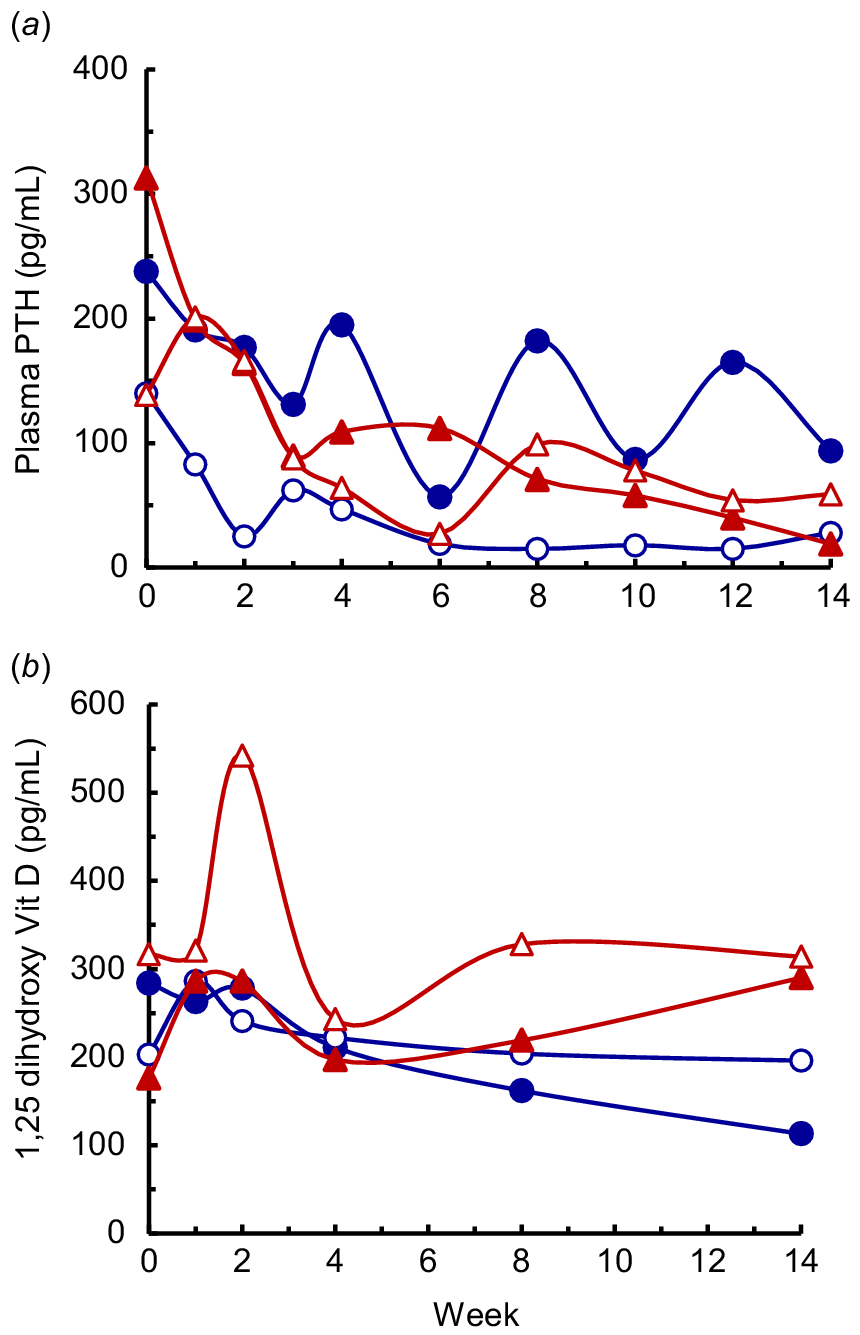
Plasma CTX-1 concentrations were very low at parturition (0.32 ± 0.02 ng/mL), and increased markedly in all diets during the first 3 weeks of lactation (Fig. 9a). There were effects of diet, time and the diet × time interaction (all P < 0.001). The CTX-1 concentrations in HP-HCa cows reached a maximum at Week 3 of lactation (2.0 ± 0.51 ng/mL), and then gradually declined to 0.6 ng/mL at Week 14. In contrast, in cows fed each of the LP diets, the CTX-1 concentrations continued to increase until Week 6, and then plateaued until Week 14 (mean ≥4.7 ng/mL). Among these LP diets, the CTX-1 concentrations from Weeks 2 to 14 were generally higher (P < 0.05) in the LP-LCa+ac cows than the LP-HCa cows, whereas concentrations in the LP-LCa cows were intermediate and not different (P > 0.05) to the other LP diets.
The plasma concentrations of (a) carboxy-terminal telepeptides of type 1 collagen (CTX-1), (b) osteocalcin (OCN), (c) the ratio of CTX-1 to OCN ×100 (CTX-1/OCN) and (d) bone alkaline phosphatase (BAP) in lactating cows fed four diets comprising HP-HCa (), LP-LCa (
), LP-HCa (
) and LP-LCa+ac (
) during lactation. (a) The diet, time, and diet × time interaction were significant (all P < 0.001), and the l.s.d.( 5%) of the diet × time interaction = 1.3. (b) The effects of diet, time and diet × time interaction were all significant (P < 0.05 or lower), and the l.s.d. (5%) of the diet × time interaction = 11. (c) The effects of diet, time and diet × time interaction were all significant (P < 0.05 or lower), and the l.s.d. (5%) of the diet × time interaction = 65. (d) The effects of diet, time and diet × time interaction were all significant (P < 0.05 to P < 0.001), and the l.s.d.(5%) of the diet × time interaction = 12.
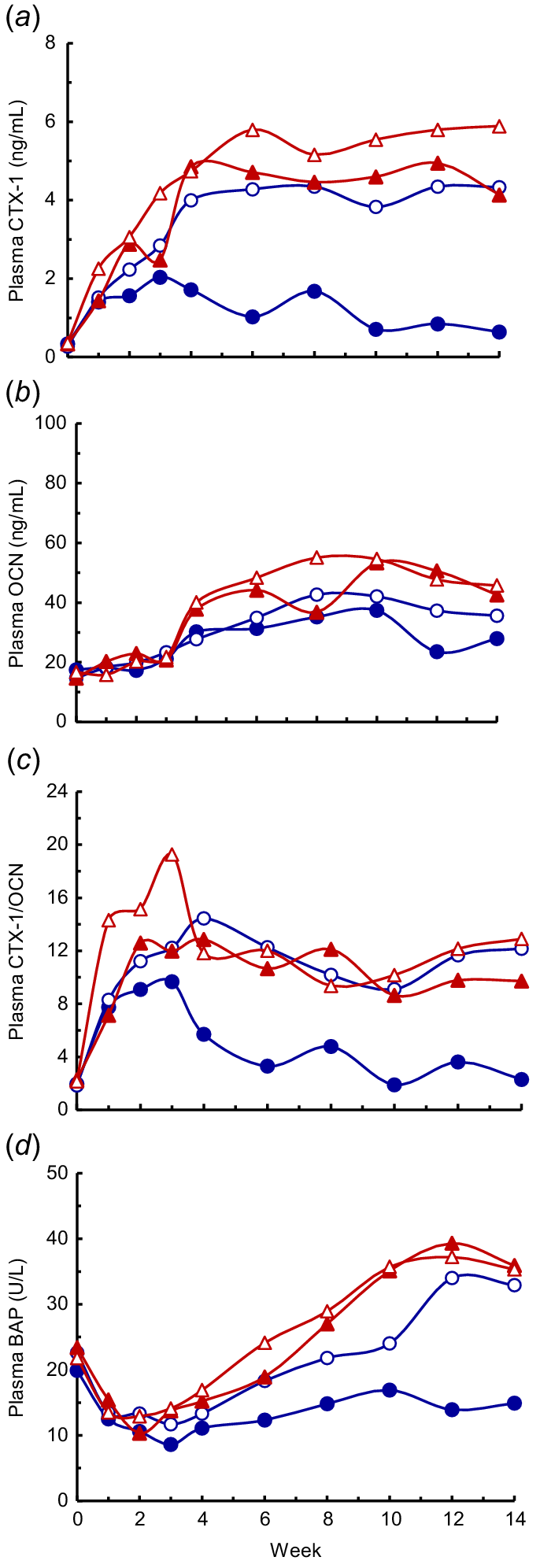
At parturition, plasma OCN concentrations were low (mean 16 ng/mL) in all the diets, and increased gradually to an average of 35 ng/mL across all diets at Week 4 (Fig. 9b). From Weeks 6 to 14, the OCN concentrations were always lower in the HP-HCa cows than LP-LCa+ac cows (means 30 and 49 ng/mL, respectively; P < 0.05), whereas the LP-LCa and LP-HCa cows were intermediate, averaging 46 and 40 ng/mL.
The plasma CTX-1/OCN ratio (×100; Fig. 9c) increased markedly from a mean of 2.1 at parturition to 8–10 in the HP-HCa cows from Weeks 1 to 3, and then declined to 2.3 by Week 14. The cows fed each of the LP diets increased to 7–19 during Weeks 1–3 before stabilising to the range 9–14 from Weeks 2 to 14. Plasma BAP concentrations (Fig. 9d) decreased shortly after parturition until Week 3 across all treatments (means 22 and 12 U/L, respectively), but thereafter followed a similar pattern to that of OCN concentrations. There were effects of diet, time and the diet × time interaction (P < 0.05, P < 0.001 and P = 0.05, respectively). As lactation progressed, BAP concentrations increased (P < 0.01) in each of the LP diets, and during Week 10 and 12, BAP were higher (P < 0.05) in the LP-LCa than the LP-HCa diet. During Weeks 10–14, the BAP concentrations were lower (P < 0.05) in the HP-HCa diet (mean 16 U/L) than in all three LP diets (means 27–39 U/L).
The interrelationships between changes in animal performance, P kinetics and plasma metabolites
In the correlation matrix among the measured variables (Table 6) there were numerous significant (P < 0.05 or lower) correlations between the intakes of P and metabolisable energy, P retention, changes in cow LW, rib bone PSACB, and plasma metabolites. These correlations were generally in accord with the expectations that across the treatment diets, the higher intakes of P and metabolisable energy would be associated with higher P retention and Pi, lower CTX-1 and Ca/Pi, and that milk production and calf growth would be poorly related to intakes and metabolite concentrations. The correlations among plasma CTX-1, Ca/Pi and BAP (Table 6) were expected, as each is indicative of bone mobilisation or deposition (Anderson et al. 2017). The multiple regression relationships (Table 7) supported the hypothesis that P intake was a primary driver of P retention, plasma Pi and cow LW change, although a number of other measurements also contributed to the prediction of the dependent variables (Table 7). For example, the magnitude of milk P secretion, bone mineral status measured as the PSACB at parturition, and plasma Pi and CTX-1 concentrations contributed to the prediction of P retention. In the multiple regression modelling to predict the P retention from metabolisable energy intake and various measurements in plasma, there was a better prediction from metabolisable energy intake and plasma CTX-1 (R2 = 0.82) than from metabolisable energy intake and plasma Ca/Pi or BAP (R2 = 0.72 and 0.73, respectively).
Variables | Variables | ||||||||||||||
---|---|---|---|---|---|---|---|---|---|---|---|---|---|---|---|
1 | 2 | 3 | 4 | 5 | 6 | 7 | 8 | 9 | 10 | 11 | 12 | 13 | |||
Cow LW(p) | P-intake | ME intake | Cow LW (Δ) | Pi | P-ret | Milk PA | Calf LWG | PSACB (p) | PSACB (Δ) | CTX-1 | Ca/Pi | BAP | |||
1 | Cow LW (p) | – | |||||||||||||
2 | P-intake | −0.08 | – | ||||||||||||
3 | ME intake | −0.25 | 0.79*** | – | |||||||||||
4 | Cow LW (Δ) | −0.39* | 0.58** | 0.42* | – | ||||||||||
5 | Pi | −0.15 | 0.75*** | 0.67** | 0.55** | – | |||||||||
6 | P-ret | −0.17 | 0.90*** | 0.80** | 0.52** | 0.80** | – | ||||||||
7 | Milk PA | 0.03 | 0.18 | 0.02 | −0.25 | −0.07 | −0.12 | – | |||||||
8 | Calf LWG | 0.12 | 0.26 | 0.37 | −0.36 | 0.14 | 0.18 | 0.55** | – | ||||||
9 | PSACB (p) | 0.00 | 0.04 | −0.08 | −0.17 | 0.02 | 0.22 | −0.09 | −0.01 | – | |||||
10 | PSACB (Δ) | −0.28 | 0.48* | 0.37 | 0.53** | 0.26 | 0.29 | 0.25 | 0.05 | −0.70*** | – | ||||
11 | CTX-1 | 0.19 | −0.85*** | −0.58** | −0.52** | −0.58** | −0.82** | −0.11 | −0.19 | −0.07 | −0.52** | – | |||
12 | Ca/Pi | 0.08 | −0.77*** | −0.50** | −0.54** | −0.68** | −0.71** | −0.05 | 0.00 | −0.17 | −0.31 | 0.62*** | – | ||
13 | BAP | −0.07 | −0.53** | −0.21 | −0.31 | −0.44* | −0.49* | −0.20 | −0.08 | −0.32 | −0.14 | 0.46* | 0.57** | – |
Variables: Cow LW(p), cow liveweight immediately after parturition (kg); P-intake, intake of P (g/day); ME intake, intake of metabolisable energy (MJ ME/day); Cow LW(Δ), cow liveweight change during lactation (kg/day); Pi, concentration of inorganic P in plasma (mmol/L); P-ret, P retention (g P/day); Milk P, P secreted in milk (g P/day); Calf LWG, calf liveweight gain (kg/day); PSACB(p), P per unit surface area of cortical rib bone at parturition (μP/mm2); PSACB(Δ), change in P per unit surface area of cortical rib bone during lactation (μg P/mm2); CTX-1, carboxy-terminal telepeptides of type 1 collagen; Ca/Pi, ratio of calcium to inorganic P in plasma; BAP, bone alkaline phosphatase in plasma.
Milk PA: As the concentration of P in milk (mean 0.87 ± 0.12 g P/kg) was not significantly affected (P ≥ 0.05) by the diet treatments or by the week of lactation, these correlation coefficients are approximations for the correlation coefficients with the amount of milk produced.
*, P < 0.05; **, P < 0.01; ***, P < 0.001.
Dependent variables | Independent variables | R2 | |||||||||
---|---|---|---|---|---|---|---|---|---|---|---|
Constant | P intake (g P/day) | PSACB at parturition (μg P/mm2) | MEI (kJ ME/kg LW.day) | Milk P (g P/day) | CTX-1 (ng/mL) | Pi (mmol/L) | Calf LWG (kg/day) | P retention (g P/day) | |||
P retention (g P/day) | |||||||||||
Y1 | −9.26*** | 0.614*** | – | – | – | – | – | – | – | 0.79 | |
Y1 | −13.9*** | 0.609*** | 0.00815* | – | – | – | – | – | – | 0.82 | |
Y1 | −3.58* | 0.656*** | – | – | −1.27*** | – | – | 0.88 | |||
Y1 | −16.2*** | – | – | 0.102*** | −1.49*** | – | – | – | 0.82 | ||
Y1 | −4.11* | – | – | – | – | −1.50*** | 6.48*** | – | – | 0.81 | |
Pi (mmol/L) | |||||||||||
Y2 | 0.552*** | 0.0391*** | – | – | – | – | – | – | – | 0.56 | |
Y2 | 1.123*** | – | – | – | – | – | – | – | 0.0596*** | 0.59 | |
Cow LWG (kg/day) | |||||||||||
Y3 | −0.038n.s. | 0.0325*** | – | – | −0.0993* | – | – | – | – | 0.41 | |
Y3 | 0.541(−) | 0.0364*** | – | – | – | – | – | – | – | 0.54 | |
Y3 | 0.125n.s. | – | – | – | – | 0.632*** | −1.414** | – | 0.46 | ||
Calf LWG (kg/day) | |||||||||||
Y4 | 1.128n.s. | – | – | 0.00163* | 0.0493*** | – | – | – | – | 0.43 | |
– |
LWG, liveweight gain; PSACB, phosphorus per unit area of outer cortical bone at parturition; MEI, metabolisable energy intake.
n.s., not significant; (−), P < 0.10; *, P < 0.05; **, P < 0.01; ***, P < 0.001.
Discussion
The performance and P replenishment of the cows fed the high P diet
The performance of the HP-HCa diet cows was generally as expected for their genotype, physiological status, quality of their diet, and that they were in moderate LW and BCS at parturition. As the estimated intake of absorbed P by these HP-HCa cows was substantially higher (1.27) than their calculated requirements, the replenishment of bone minerals should not have been limited by the availability of Pi as a substrate. The increase in PSACB in these HP-HCa cows from 532 μg P/mm2 in mid-pregnancy to 634 μg P/mm2 at parturition, and the further increase to 729 μg P/mm2 by Week 14 of lactation (Table 5; i.e. approximately a 32% increase over 7–8 months) showed that extensive bone repletion occurred during late pregnancy and early lactation in these cows fed adequate dietary P. Furthermore, this occurred when the cows were at approximately maintenance CF-LW during late pregnancy, and gaining a modest 0.15 kg LW/day during early lactation. The 15% gain in PSACB in the HP-HCa cows during lactation may have been partly associated with their consuming a high-P diet during late pregnancy, as well as during lactation. Cows that are P deficient, including during late pregnancy, may need time to adapt to commence bone growth when fed adequate P, and exhibit lower bone growth in early lactation than that observed in the HP-HCa cows during the present experiment.
Observations that the cows fed the HP-HCa diet had high voluntary intakes of DM and metabolisable energy (23.1 g DM/kg LW.day and 210 kJ ME/kg LW.day) and, after adaptation, gained 0.45 kg LW/day, were consistent with previous reports of the intake and LW gain of mature cows of similar genotype during early lactation when fed a diet containing adequate metabolisable energy, protein and minerals (Holroyd et al. 1979; Dixon et al. 2007; Coates et al. 2019; Dixon and Mayer 2021). The retention of ~6 g P/day (or ~12 mg P/kg LW.day) for at least the first 8 weeks of lactation, and the increase in PSACB to the equivalent of 729 μg P/mm2 in the 12th rib indicated that these mature cows substantially replenished their bone P reserves to a P replete status. This PSACB was also indicative of adequate bone P status (>650 μg P/mm2). This rate of replenishment for 8–13 weeks would correspond to 350–500 g body P, or 13–18% of the estimated 2.8 kg of bone P in a 470 kg P replete cow (ARC 1980). The calf birthweight (mean 30.2 kg) was low within the expected range of 30–35 kg for mature Droughtmaster cows. Low birthweights may have been associated with the low nutrition of these cows during their first and second trimesters of pregnancy. The cows in the present experiment had grazed P-deficient native pasture during the rainy and rainy-dry transition seasons during their previous lactation, and then post-weaning during their second trimester in the previous experiment (Dixon et al. 2024) most of the cows had ME intakes that allowed only slow gain (0.07 to 0.32 kg/day) in CF-LW. The milk secretion and calf growth rates in the HP-HCa cows in the present experiment (5.81 kg/day and 0.70 kg/day) corresponded to an 8.3:1 conversion of milk to calf growth. This is similar to the 8.9:1 conversion of milk to calf growth in Bos indicus cows in early lactation reported by Lampkin and Lampkin (1960). The calf growth in these HP-HCa diet cows (mean 0.70 kg/day) was in the lower range (~0.6–0.9 kg/day) reported for mature Droughtmaster cows fed a high-quality diet in pens or grazing high-quality rainy season pasture (Holroyd et al. 1979, 1988; Dixon et al. 2007, 2011; Dixon and Mayer 2021).
The replenishment rates of P in these HP-HCa cows of 6.2 g P/day (8.3 mg P/kg LW) for the first 8–13 weeks of lactation were comparable with the 3–7 g P/day replenishment of acutely P-deficient recently-weaned Droughtmasters cows receiving P supplements reported by Miller et al. (1998). Also Schild et al. (2023) reported rapid bone P replenishment in Bos taurus beef cows that were initially exhibiting osteomalacia; cows were able to substantially recover bone P when fed 8 g supplementary P/day for 3 months post-weaning. Higher rates of P replenishment (up to 30–40 mg P/kg LW) have been reported in high-producing dairy cows (Knowlton et al. 2001; Knowlton and Herbein 2002) and in dairy goats (Deitert and Pfeffer 1993; Pfeffer et al. 1993), but capacity for rapid replenishment of bone minerals would be an expected adaptation of dairy breed ruminants selected for high milk production.
The observation in the present experiment that P retention was negligible (−0.6 g P/day) in the HP-HCa cows by Week 14 of lactation indicated that these lactating Bos indicus cross cows replenished the majority of their body P reserves within several months, even when intakes of metabolisable energy and protein were sufficient for only moderate growth. The P retention measurements were consistent with the high PSACB (estimated as 729 μg P/mm2 in the 12th rib) that classed most (6/8) of these HP-HCa diet cows in adequate bone status at the end of the experiment. Importantly, the changes in PSACB during lactation were related to the PSACB at parturition (Fig. 5). It was also consistent with higher Pi during Weeks 10–14 (1.70 ± 0.20 mmol/L) than during Weeks 2–8 (1.40 ± 0.16 mmol/L; Fig. 6a); decreasing demand for Pi as a substrate for soft tissue and bone growth after Week 10 was consistent with the increased Pi. These concentrations compare with the Pi averaging 2.15 mmol/L after 10–14 weeks observed in this same group of cows when fed high-P diets during mid-pregnancy (Dixon et al. 2024), and also during the introductory interval when the cows were consuming a high-P diet. Comparable high-Pi concentrations have been reported in similar genotype cows during late pregnancy and early lactation ingesting diets adequate in P (Coates et al. 2019; Dixon et al. 2020b). More generally, replenishment of bone P can be expected in cows with PSACB indicative of deficient or marginal bone mineral status, and when they are ingesting a high-P diet and sufficient metabolisable energy for moderate LW gain.
High-producing dairy cows and ewes often exhibit obligatory body P mobilisation during early lactation (Braithwaite 1986; Knowlton and Herbein 2002). In the present experiment, the increases in plasma CTX-1 from a mean 0.3 ng/mL at parturition to ≥1.4 and ≥2.0 ng/mL at Weeks 1 and 3 of lactation in the HP-HCa cows, and even higher concentrations in the cows fed the LP diets, support the hypothesis that obligatory body P mobilisation also occurs in beef genotype cows shortly after parturition. However, the plasma CTX-1 concentrations in the cows in the present experiment fed the HP-HCa diet were much lower than those reported in high-producing dairy cows in early lactation fed adequate/high P diets of 3–4 ng/mL (Ekelund et al. 2006; Puggaard et al. 2014; Gaignon et al. 2019). In addition, the marked increases in OCN concentrations in early lactation in the present study indicate increased bone deposition, and the measured retention of 6.0 g P/day (10.4 mg P/kg LW.day) during Week 4 of lactation, clearly showed that there was substantial net bone mineral deposition despite the increases in CTX-1 concentrations in these cows fed the HP-HCa diet. This evidence for increased mobilisation and deposition of bone is in accord with generally higher bone turnover in lactating than non-lactating animals. However, it has been suggested (Liesegang et al. 1998, 2000) that in dairy cows in early lactation, bone mobilisation and subsequent bone formation are less closely linked, and also that the magnitude of this effect depends on the level of milk production.
The Pi of the HP-HCa cows was lower than the 1.8–2.2 mmol/L expected for mature non-lactating cows ingesting P in excess of their immediate requirements (Anderson et al. 2017; Dixon et al. 2020b, 2024). However, the Pi in the HP-HCa cows in the present experiment was in accord with observations that the Pi in high-producing dairy cows fed P-adequate diets in late pregnancy and early lactation are often reduced to the range of 1.4–1.7 mmol/L (Peterson et al. 2005; Taylor et al. 2009; Keanthao et al. 2021), apparently due to the high demand for Pi substrate in lactating cows. The concentrations of plasma Ca and of the other measured metabolites during the present experiment were generally comparable to those of these cows in mid-pregnancy, and the changes that did occur were consistent with the increased demands of lactation for P. The increased plasma Ca concentrations during lactation in the HP-HCa cows were consistent with bone mobilisation together with normal intake of Ca, and notably, Ca concentrations were within the normal physiological range. The plasma 1,25-dihydroxy vitamin D3 and PTH in the HP-HCa cows were comparable with concentrations of these hormones in the same cows fed HP-HCa diets during pregnancy (Dixon et al. 2024). The plasma BAP concentrations during Weeks 1–3 of lactation were low in the present experiment, but increased from Week 4 in the LP cows to be comparable with the cows fed LP diets in mid-pregnancy reported by Dixon et al. (2024). The results support the hypothesis that, following suppression in the early post-partum interval, bone formation recovers in early lactation in cows fed an adequate P diet. Overall the bone endocrine marker results (CTX-1, BAP, OCN) in HP-HCa cows support the hypothesis that bone turnover generally increases during lactation.
The performance and P mobilisation of the cows fed the low P diets
The rapid decline in plasma Pi in cows fed each of the LP diets, and the very low concentrations from Weeks 10 to 14 (mean 0.37–0.48 mmol/L) were consistent with these cows being acutely P deficient (Wadsworth et al. 1990; Dixon et al. 2020a). The concurrent increases in plasma Ca to an average of 2.47 mmol/L during Weeks 10–14 were in accord with previous reports of increased plasma Ca during bone mobilisation, and increased 1,25-dihydroxy vitamin D3 stimulating Ca absorption from the gastrointestinal tract (Anderson et al. 2017). The increases in plasma concentrations of 1,25-dihydroxy vitamin D3 in response to the LP-LCa and LP-LCa+ac diets after 14 weeks of lactation were consistent with low-P diets increasing the active form of this hormone in our other studies with growing steers, and pregnant and lactating primiparous and multiparous cows of similar genotype (Anderson et al. 2016, 2018a, 2018b). Although increased 1,25-dihydroxy vitamin D3 in response to severely P-deficient diets is commonly observed in monogastrics (Fraser 1980), it apparently does not always occur in ruminants. Some studies with sheep and beef cattle have reported increases similar to the present study (Blair-West et al. 1992; Kohler et al. 2020), whereas other studies with sheep (Breves et al. 1985; Mauder et al. 1986) and dairy cows (Cohrs et al. 2018; Wächter et al. 2021) have observed no such effects. Differences between studies in activation of active vitamin D by low-P diets probably reflect the duration and degree of phosphorus deficiency compared with that in dairy cows in early lactation.
The progressive increases in plasma CTX-1 concentrations in the LP cows until Weeks 4–6, and the high concentrations thereafter, were in accord with marked bone mobilisation in early lactation in these cows. The CTX-1 concentrations in these LP cows (~4–6 ng/mL) in lactation were higher than when these same cows were acutely P deficient during mid-pregnancy (~2.5–3.5 ng/mL; Dixon et al. 2024). This was consistent with higher body P mobilisation in the cows during early lactation in the present experiment (~6–7 g P/day) than during their second trimester of pregnancy (~4–5 g P/day). Furthermore, the increases in plasma Ca/Pi ratio are in agreement with the elevated CTX-1 concentrations, and the higher plasma Ca/Pi ratio (~5.5–7.5) in these lactating cows than during pregnancy (~3.0–5.0).
These measurements of CTX-1 and Ca/Pi in plasma are in accord with the proposal of Anderson et al. (2017) that a high plasma Ca/Pi ratio provides a marker of bone mobilisation in lactating, as well as non-lactating, P-deficient cattle. Interestingly, the mechanism driving bone resorption in LP cows is unlikely to be PTH, as this hormone is markedly suppressed. Possibly mammary-derived PTH-related protein drives bone resorption in lactation and is also responsible for increased bone resorption observed here in response to low-P diets, but such roles for PTH-related protein remain to be firmly established in cattle (Anderson et al. 2017). Supporting the concept that bone turnover is increased during lactation, both OCN and BAP as markers of bone formation increased during lactation in P-deficient cows after being suppressed soon after parturition. Similarly, Ekelund et al. (2006) reported higher OCN concentrations in high-producing dairy cows given low-P diets, although this was observed from Weeks 17 to 24 of lactation, and thus, later in lactation than in the present study. The increase in BAP in the LP cows is in accord with the P deficiency of the LP cows. Overall, these results support the hypothesis that there is a general effect of lactation to increase bone turnover, and during P deficiency net mobilisation of bone, that alleviate the effects of dietary P deficiency.
Even though the LP diet cows consumed only ~0.3 of their calculated P requirements, had Pi 0.37–0.48 mmol/L after 12–14 weeks, consumed 21% less DM than the HP-HCa cows and were losing LW (−0.14 to −0.51 kg LW/day), they were nevertheless able to largely maintain milk secretion and a calf growth rate (0.59–0.66 vs 0.70 kg/day) similar to that of the HP-HCa cows during the 14 weeks. Clearly, the LP cows were able to maintain milk production in early lactation only because they mobilised 6–7 g body P reserves/day (12–16 mg P/kg LW.day) and also body energy reserves, as represented by the cow LW loss. However, a further consideration is the because milk production was decreasing in the LP cows, but not in the HP-HCa cows (Fig. 3), it is likely that with continuation of lactation after 14 weeks to weaning at 4–6 months, the differences between the LP and the HP-HCa cows in calf growth would increase. The absence of change in the concentration of P in the milk in the cows fed the LP diets in the present study is in accord with observations that milk P is maintained, or even increased slightly, as lactation progresses in dairy cows, even when fed P-deficient diets (Forar et al. 1982; Manuelian et al. 2018; Valk et al. 2002).
As the P concentration of soft tissues (normally 1.2 g P/kg; Suttle et al. 1991) can be decreased by 30–35% during acute P deficiency in sheep (Field et al. 1975; Ternouth and Sevilla 1990), mobilisation of soft tissue P in the LP cows could have provided up to ~3.0 g P/day of the mobilised of ~6.0 g P/day. In addition, the LW loss should have provided up to ~0.3 g P/day. Therefore, at least ~2.7 g P/day must have been mobilised from bone minerals. The measured 4.1% decrease in PSACB of rib cortical bone of the LP-LCa cows, if representative of the entire skeleton (Dixon et al. 2017), corresponded to only 1.5 g P/day and, hence, underestimated the bone mineral loss. However, as rapid changes in bone occur primarily in trabecular bone in association with its very large surface area, rather than from cortical bone, this was not unexpected (Seeman 2013; Winter et al. 2020). More generally, changes in PSACB are likely to underestimate short-term changes in bone P of the entire skeleton, and it is likely that mobilisation of trabecular bone, not measured in the present experiment, contributed substantially to the body P that was mobilised by the LP diet cows.
The 12–16 mg P/kg LW.day P mobilised in the LP-LCa lactating multiparous cows in the present experiment compares with reported mobilisation of 11–15 mg P/kg LW.day in primiparous lactating cows, and 20 mg P/kg LW.day in older cows, of similar genotype when grazing acutely P-deficient pastures (Ternouth and Coates 1997; Miller et al. 1998). High rates of P mobilisation have been reported during early lactation in P-deficient dairy cows (15–25 mg P/kg LW; Knowlton and Herbein 2002; Taylor et al. 2009; Salazar et al. 2013), dairy goats (25–40 mg P/kg LW; Deitert and Pfeffer 1993; Rodehutscord et al. 1994) and ewes (35 mg P/kg LW; Braithwaite 1986). It appears that the capacity for rapid bone mobilisation during lactation is lower in beef genotype cows than in dairy cows or goats selected for high milk production. Furthermore, the rates of body P mobilisation appear to be somewhat higher in lactating cows than in non-lactating cows; Dixon et al. (2024) reported mobilisation of 9–11 mg P/kg LW in these same cows when fed acutely P-deficient diets during mid-pregnancy, whereas Tuen et al. (1984) and Bortolussi et al. (1996) reported mobilisation of up to ~10 mg P/kg LW.day in young growing Bos indicus cross cattle.
The performance and P mobilisation of the cows fed the LP-HCa and the LP-LCa+ac diets
The LP-HCa treatment examined whether a modest increase in the diet Ca (diet Ca/P ratio increased from 3.0/1 to 5.5/1) in a low-P diet affected the availability of absorbed P and cow performance. High dietary Ca can reduce the absorption of P from the gastrointestinal tract, and the deposition of P into bone in growing sheep (Young et al. 1966; Field et al. 1975; Boxebeld et al. 1983) and goats (Pfeffer et al. 1995). Also, high dietary Ca can reduce bone mineral mobilisation in cattle (Theiler et al. 1927, 1937; Otto 1938), likely by depressing PTH and 1,25-dihydroxy vitamin D3. High dietary Ca/P ratios often occur in the diets of cattle grazing rangelands with calcareous soils, at least in northern Australia, and hence are potentially important for the management of P-deficient cattle. In this context, Cohen (1975) argued that large responses of grazing cattle to P supplements in some studies were likely associated with calcareous soils and high pasture Ca. High Ca concentrations and high Ca/P ratios often occur in senesced C4 grasses, and also in the widely used tropical pasture legumes, Stylosanthes hamata cv verano and S. scabra cv seca, when growing on low-P soils (Coates 1994). In addition, many commercial P supplements include limestone, which is intended to balance the Ca/P ratio of the supplement regardless of the Ca concentrations in pastures, and also to alleviate rain damage to calcium phosphate supplements fed in open troughs.
In the present experiment, the decrease in the PSACB in the LP-LCa cows, but not in the LP-HCa cows (Table 5), provided clear evidence that the small increase in diet Ca concentration and increase in Ca intake from 14 to 22 g Ca/day did reduce bone P mobilisation. Furthermore, the higher Ca intake reduced milk energy output (P < 0.05, P < 0.10; Table 4, Fig. 3). Other measurements also supported the hypothesis that supply of P as a substrate at the tissue level was lower in LP-HCa than LP-LCa cows. These included tendencies for lower mobilisation of LW and of P from body reserves, lower concentrations of plasma Pi, PTH, 1,25-dihydroxy vitamin D3, CTX-1, OCN and BAP, together with higher plasma Ca and Ca/Pi ratio, in the LP-HCa cows. The present experiment did not definitively establish that a dietary Ca concentration of 2.8 g/kg DM and Ca/P ratio of 5.5/1 in P-deficient diets had adverse effects in lactating cows, but did indicate that caution is warranted in the management of P deficiency in cows ingesting high Ca/P ratio diets.
The LP-LCa+ac diet treatment demonstrated that the inclusion of a low concentration of ammonium choride in the diet that caused only a small decrease in the diet cation–anion difference (from −1 to −10 mequivalents/kg DM) modified the effects of dietary P deficiency. Acidogenic diets fed for several weeks prepartum and postpartum to high-producing dairy cows have often increased bone mineral mobilisation, and reduced the incidence of hypocalcemia and milk fever (Goff et al. 1991; Abu Damir et al. 1994; van Mosel et al. 1994; Goff 2000; Gaignon et al. 2019). Furthermore, diets that included ammonium chloride or were mildly acidogenic fed for extended periods have increased voluntary intake, LW gain and bone mineral mobilisation in lactating dairy cows, goats and ewes (Espino et al. 2003; Liesegang 2008), mature non-lactating ewes (MacLeay et al. 2004), and growing calves and lambs (Beighle et al. 1988; Abu Damir et al. 1991). In the present experiment, the higher intakes of DM and metabolisable energy, improved LW performance following adaptation, and higher bone mobilisation in the LP-LCa+ac than in the LP-LCa cows (Tables 2 and 5) showed that the inclusion of a low concentration of ammonium chloride did improve the performance of the cows during dietary P deficiency. Furthermore, although generally not statistically significant (P > 0.05), the observations that plasma concentrations of Pi, PTH, 1,25-dihydroxy vitamin D3, CTX-1, OCN and BAP were generally higher, and plasma Ca and plasma Ca/Pi ratio were generally lower, in the LP-LCa+ac cows than in the LP-LCa cows support the hypothesis that the inclusion of the small amount of ammonium chloride in the diet increased bone mobilisation. Improved understanding of the effects of acidogenic diets on bone mineral mobilisation in beef cows is desirable, as pastures and supplements vary in their cation–anion difference. Mildly acidogenic diets can be easily implemented during supplementation of rangeland cattle, but caution will be needed to avoid excessive bone loss in cattle already in low bone mineral status.
Conclusions
The experiment demonstrated that when ingesting acutely P-deficient diets, mature Bos indicus cross beef breeder cows calving in moderate LW and body condition (mean 474 kg and BCS 3.5), and with moderate bone mineral reserves, were able to mobilise sufficient body P to generally maintain milk secretion and calf growth during early lactation. However, this was associated with substantial losses in LW, body condition and bone mineral reserves. High dietary Ca tended to reduce bone mobilisation and milk secretion, while following adaptation a mildly acidogenic diet increased voluntary intake and bone mobilisation to alleviate the effects of the severe P deficiency.
Data availability
The data that support this study may be shared upon reasonable request to the corresponding author.
Conflicts of interest
The authors declare no conflict of interest. Dr Rob Dixon is an Associate Editor of Animal Production Science, but had no role in the review or evaluation of the manuscript.
Declaration of funding
Funding for this work was provided by Meat and Livestock Australia, by the Queensland Alliance for Agriculture and Food Innovation (QAAFI), the University of Queensland, and by the Queensland Department of Primary Industries.
Acknowledgements
We thank Tracy Longhurst, Don Cherry and the DPI team at the Brian Pastures Research Station for their assistance with the care, handling and sampling of the cattle in the experiment. We also thank Jeremy Spiers and Nick Corbet (UQ), Michael Gravel, and DPI technical staff for undertaking laboratory analyses.
References
Abu Damir H, Scott D, Loveridge N, Buchan W, Milne J (1991) The effects of feeding diets containing either NaHCO3 or NH4Cl on indices of bone formation and resorption and on mineral balance in the lamb. Experimental Physiology 76, 725-732.
| Crossref | Google Scholar | PubMed |
Abu Damir H, Phillippo M, Thorp BH, Milne J, Dick L, Nevison IM (1994) Effects of dietary acidity on calcium balance and mobilisation, bone morphology and 1,25 dihydroxyvitamin D in prepartal dairy cows. Research in Veterinary Science 56, 310-318.
| Crossref | Google Scholar | PubMed |
Anderson ST, McNeill DM, Castells L, Spiers JG, Kidd LJ, Goodwin K, Fletcher MT, Dixon RM (2016) Novel physiological responses in phosphorus deficient beef cows. In ‘67th Annual Meeting of the European Federation of Animal Science’, 29 August−2 September 2016, Belfast, United Kingdom. (Wageningen Academic Publishers: Wageningen, Netherlands)
Anderson ST, Kidd LJ, Benvenutti MA, Fletcher NT, Dixon RM (2017) New candidate markers of phosphorus status in beef breeder cows. Animal Production Science 57, 2291-2303.
| Crossref | Google Scholar |
Anderson ST, Benvenutti MA, Steiger N, Goodwin KL, Kidd LJ, Fletcher MT, Dixon RM (2018a) Effects of phosphorus (P) and energy intakes on plasma markers of P deficiency in pregnant heifers. In ‘69th Annual Meeting of the European Federation of Animal Production’, 27–31 August 2018, Dubrovnik, Croatia. (Wageningen Academic Publishers: Wageningen, Netherlands)
Beighle DE, Tucker WB, Hemken RW (1988) Interactions of dietary cation-anion balance and phosphorus: Effects on growth and serum inorganic phosphorus in dairy calves. Journal of Dairy Science 71, 3362-3368.
| Crossref | Google Scholar | PubMed |
Benzie D, Boyne AW, Dalgarno AC, Duckworth J, Hill R (1959) Studies of the skeleton of the sheep. III. The relationship between phosphorus intake and resorption and repair of skeleton in pregnancy and lactation. Journal of Agricultural Science, Cambridge 52, 1-12.
| Crossref | Google Scholar |
Blair-West JR, Denton DA, McKinley MJ, Radden BG, Ramshaw EH, Wark JD (1992) Behavioral and tissue responses to severe phosphorus depletion in cattle. The American Journal of Physiology 263, R656-R663.
| Crossref | Google Scholar | PubMed |
Bortolussi G, Ternouth JH, McMeniman NP (1996) Dietary nitrogen and phosphorus depletion in cattle and their effects on liveweight gain, blood metabolite concentrations and phosphorus kinetics. Journal of Agricultural Science, Cambridge 126, 493-501.
| Crossref | Google Scholar |
Boxebeld A, Guéguen L, Hannequart G, Durand M (1983) Utilization of phosphorus and calcium and minimal maintenance requirement for phosphorus in growing sheep fed a low-phosphorus diet. Reproduction, Nutrition and Development 23, 1043-1053.
| Crossref | Google Scholar |
Braithwaite GD (1986) Phosphorus requirements of ewes in pregnancy and lactation. Journal of Agricultural Science, Cambridge 106, 271-278.
| Crossref | Google Scholar |
Breves G, Ross R, Höller H (1985) Dietary phosphorus depletion in sheep: effects on plasma inorganic phosphorus, calcium, 1,25-(OH)2-Vit.D3 and alkaline phosphatase and on gastrointestinal P and Ca balances. Journal of Agricultural Science 105, 623-629.
| Crossref | Google Scholar |
Coates DB (1994) The effect of phosphorus as fertiliser or supplement on pasture and cattle productivity in the semi-arid tropics of north Queensland. Tropical Grasslands 28, 90-108.
| Google Scholar |
Coates DB, Dixon RM, Mayer RJ (2019) Between-year variation in the effects of phosphorus deficiency in breeder cows grazing tropical pastures. Tropical Grasslands-Forajes Tropicales 7, 223-233.
| Crossref | Google Scholar |
Cohen RDH (1975) Phosphorus and the grazing ruminant. World Review of Animal Production 11, 27-43.
| Google Scholar |
Cohrs I, Wilkens MR, Grünberg W (2018) Effect of dietary phosphorus deprivation in late gestation and early lactation on the calcium homeostasis of periparturient dairy cows. Journal of Dairy Science 101, 9591-9598.
| Crossref | Google Scholar | PubMed |
Deitert C, Pfeffer E (1993) Effects of reduced P supply in combination with adequate or high Ca intake on performance and mineral balances in dairy goats during pregnancy and lactation. Journal of Animal Physiology and Animal Nutrition 69, 12-21.
| Crossref | Google Scholar |
Dixon RM, Mayer R (2021) Effects of a nitrogen-based supplement on intake, live weight and body energy reserves in breeding Bos indicus cows. Tropical Grasslands – Forrajes Tropicales 9(1), 70-80.
| Crossref | Google Scholar |
Dixon RM, Smith DR, Coates DB (2007) Using faecal NIRS to improve nutritional management of breeders in the seasonally dry tropics. Recent Advances in Animal Nutrition in Australia 16, 123-134.
| Google Scholar |
Dixon RM, Playford C, Coates DB (2011) Nutrition of beef breeder cows in the dry tropics. 2. Effects of time of weaning and diet quality on breeder performance. Animal Production Science 51, 529-540.
| Crossref | Google Scholar |
Dixon RM, Kidd LJ, Coates DB, Anderson ST, Benvenutti MA, Fletcher MT, McNeill DM (2017) Utilising mobilisation of body reserves to improve management of phosphorus nutrition of breeder cows. Animal Production Science 57, 2280-2290.
| Crossref | Google Scholar |
Dixon RM, Coates DB, Mayer RJ, Miller CP (2019) Alternative rib bone biopsy measurements to estimate changes in skeletal mineral reserves in cattle. Animal 13, 119-126.
| Crossref | Google Scholar | PubMed |
Dixon RM, Anderson ST, Kidd LJ, Fletcher MT (2020a) Management of phosphorus nutrition of beef cattle grazing seasonally dry rangelands: a review. Animal Production Science 60, 863-879.
| Crossref | Google Scholar |
Dixon RM, Castells L, Goodwin KL, Kidd LJ, Anderson ST, Mayer RJ, Isherwood P, McNeill DM, Fletcher MT (2024) Mobilisation and replenishment of phosphorus reserves in Bos indicus cows. 1. Mid-pregnant mature cows post-weaning. Animal Production Science 64, AN24213.
| Crossref | Google Scholar |
Ekelund A, Spörndly R, Holtenius K (2006) Influence of low phosphorus intake during early lactation on apparent digestibility of phosphorus and bone metabolism in dairy cows. Livestock Science 99, 227-236.
| Crossref | Google Scholar |
Espino L, Guerrero F, Suarez ML, Santamarina G, Goicoa A, Fidalgo LE (2003) Long-term effects of dietary anion-cation balance on acid-base status and bone morphology in reproducing ewes. Journal of Veterinary Medicine Series A 50, 488-495.
| Crossref | Google Scholar | PubMed |
Field AC, Suttle NF, Nisbet DI (1975) Effect of diets low in calcium and phosphorus on the development of growing lambs. Journal of Agricultural Science, Cambridge 85, 435-442.
| Crossref | Google Scholar |
Forar KL, Kincaid RL, Preston RL, Hillers JK (1982) Variation of inorganic phosphorus in blood plasma and milk of lactating cows. Journal of Dairy Science 65, 760-763.
| Crossref | Google Scholar | PubMed |
Fraser DR (1980) Regulation of the metabolism of vitamin D. Physiological Reviews 60, 551-613.
| Crossref | Google Scholar | PubMed |
Gaignon P, Le Grand K, Laza-Knoerr AL, Hurtaud C, Boudon A (2019) Effect of calcium intake and the dietary cation-anion difference during early lactation on the bone mobilization dynamics throughout lactation in dairy cows. PLoS ONE 14, e0218979.
| Crossref | Google Scholar |
Goff JP (2000) Pathophysiology of calcium and phosphorus disorders. Veterinary Clinics of North America: Food and Animal Practice 16, 319-337.
| Crossref | Google Scholar |
Goff JP, Horst RL, Mueller FJ, Miller JK, Kiess GA, Dowlen HH (1991) Addition of chloride to a prepartal diet high in cations increases 1,25-dihydroxyvitamin D response to hypocalcemia preventing milk fever. Journal of Dairy Science 74, 3863-3871.
| Crossref | Google Scholar | PubMed |
Goodwin JF (1970) Quantification of serum inorganic phosphorus, phosphatase, and urinary phosphate without preliminary treatment. Clinical Chemistry 16, 776-780.
| Crossref | Google Scholar | PubMed |
Holroyd RG, O’Rourke PK, Allan PJ (1979) Effects of pasture type and supplementary feeding on the milk yield of Shorthorn and Brahman cross cows and the growth rate of their progeny in the dry tropics of north Queensland. Australian Journal of Experimental Agriculture 19, 389-394.
| Crossref | Google Scholar |
Holroyd RG, Mason GWJ, Loxton ID, Knights PT, O’Rourke PK (1988) Effects of weaning and supplementation on performance of Brahman cross cows and their progeny. Australian Journal of Experimental Agriculture 28, 11-20.
| Crossref | Google Scholar |
Keanthao P, Goselink RMA, Dijkstra J, Bannink A, Schonewille JT (2021) Effects of dietary phosphorus concentration during the transition period on plasma calcium concentrations, feed intake, and milk production in dairy cows. Journal of Dairy Science 104, 11646-11659.
| Crossref | Google Scholar | PubMed |
Knowlton KF, Herbein JH (2002) Phosphorus partitioning during early lactation in dairy cows fed diets varying in phosphorus content. Journal of Dairy Science 85, 1227-1236.
| Crossref | Google Scholar | PubMed |
Knowlton KF, Herbein JH, Meister-Weisbarth MA, Wark WA (2001) Nitrogen and phosphorus partitioning in lactating Holstein cows fed different sources of dietary protein and phosphorus. Journal of Dairy Science 84, 1210-1217.
| Crossref | Google Scholar | PubMed |
Kohler OM, Grunberg W, Schnepel N, Muscher-Banse AS, Rajaeerad A, Hummel J, Breves G, Wilkens MR (2020) Dietary phosphorus restriction affects bone metabolism, vitamin D metabolism and rumen fermentation traits in sheep. Journal of Animal Physiology and Animal Nutrition 105(1), 35-50.
| Crossref | Google Scholar |
Lampkin K, Lampkin GH (1960) Studies on the production of beef from zebu cattle in East Africa II. Milk production in suckled cows and its effect on calf growth. Journal of Agricultural Science 55, 233-239.
| Crossref | Google Scholar |
Liesegang A (2008) Influence of anionic salts on bone metabolism in periparturient dairy goats and sheep. Journal of Dairy Science 91, 2449-2460.
| Crossref | Google Scholar |
Liesegang A, Sassi ML, Risteli J, Eicher R, Wanner M, Riond J-L (1998) Comparison of bone resorption markers during hypocalcaemia in dairy cows. Journal of Dairy Science 81, 2614-2622.
| Crossref | Google Scholar | PubMed |
Liesegang A, Eicher R, Sassi ML, Risteli J, Kraenzlin M, Riond J-L, Wanner M (2000) Biochemical markers of bone formation and resorption around parturition and during lactation in dairy cows with high and low standard milk yields. Journal of Dairy Science 83, 1773-1781.
| Crossref | Google Scholar | PubMed |
Little DA (1972) Bone biopsy in cattle and sheep for studies of phosphorus status. Australian Veterinary Journal 48, 668-670.
| Crossref | Google Scholar | PubMed |
Little DA, Minson DJ (1977) Variation in the phosphorus content of bone samples obtained from the last three ribs of cattle. Research in Veterinary Science 23, 393-394.
| Crossref | Google Scholar | PubMed |
Little DA, Ratcliff D (1979) Phosphorus content of bovine rib: influence of earlier biopsy of the same rib. Research in Veterinary Science 27, 239-241.
| Crossref | Google Scholar | PubMed |
MacLeay JM, Olsen JD, Turner AS (2004) Effect of dietary-induced metabolic acidosis and ovariectomy on bone mineral density and markers of bone turnover. Journal of Bone Mineral Metabolism 22, 561-568.
| Crossref | Google Scholar | PubMed |
Manuelian CL, Penasa M, Visentin G, Zidi A, Cassandro M, Marchi MD (2018) Mineral composition of cow milk from multibreed herds. Animal Science Journal 89, 1622-1627.
| Crossref | Google Scholar | PubMed |
Mauder EM, Pillay AV, Care AD (1986) Hypophosphataemia and vitamin D metabolism in sheep. Quarterly Journal of Experimental Physiology 71, 391-399.
| Google Scholar |
Otto JS (1938) The assimilation of calcium and phosphorus by the growing bovine. Onderstepoort Journal of Veterinary Science and Animal Industry 10, 281-364.
| Google Scholar |
O’Rourke PK, Entwistle KW, Arman C, Esdale CR, Burns BM (1991) Fetal development and gestational changes in Bos taurus and Bos indicus genotypes in the tropics. Theriogenology 36, 839-853.
| Google Scholar | PubMed |
Peterson AB, Orth MW, Goff JP, Beede DK (2005) Periparturient responses of multiparous Holstein cows fed different dietary phosphorus concentrations prepartum. Journal of Dairy Science 88, 3582-3594.
| Crossref | Google Scholar | PubMed |
Pfeffer E, Pauen A, Haverkamp R (1993) Changes in retention of P and Ca and courses of blood plasma concentrations of inorganic phosphate and calcium in dairy goats following a change in P supply from reduced to adequate in combination with adequate or high Ca intake. Journal of Animal Physiology and Animal Nutrition 69, 22-28.
| Crossref | Google Scholar |
Pfeffer E, Rodehutscord M, Breves G (1995) Effects of reducing dietary calcium and/or phosphorus on performance and body composition in male kids. Journal of Animal Physiology and Animal Nutrition 74, 243-252.
| Crossref | Google Scholar |
Puggaard L, Lund P, Liesegang A, Schested J (2014) Long term effect of reduced dietary phosphorus on feed intake and milk yield in dry and lactating dairy cows. Livestock Science 159, 18-28.
| Crossref | Google Scholar |
Read MVP, Engels EAN, Smith WA (1986a) Phosphorus and the grazing ruminant. 2. The effects of supplementary P on cattle at Glen and Armoedsvlakte. South African Journal of Animal Science 16, 7-12.
| Google Scholar |
Read MVP, Engels EAN, Smith WA (1986b) Phosphorus and the grazing ruminant. 3. Rib bone samples as an indicator of the P status of cattle. South African Journal of Animal Science 16, 13-17.
| Google Scholar |
Rodehutscord M, Pauen A, Windhausen P, Pfeffer E (1994) Balances of phosphorus and calcium in dairy goats during periods of phosphorus depletion and subsequent phosphorus repletion. Journal of Animal Physiology and Animal Nutrition 72, 57-64.
| Crossref | Google Scholar |
Salazar JAE, Ferguson JD, Beegle DB, Remsburg DW, Wu Z (2013) Body phosphorus mobilization and deposition during lactation in dairy cows. Animal Physiology and Nutrition 97, 502-514.
| Crossref | Google Scholar |
Schild CO, Boabaid FM, Olivera LGS, Armendano JI, Saravia A, Custodio A, Algorta J, Alvarez C, Jaurena M, Dixon RM, Riet-Correa F (2023) Response of cows with osteomalacia grazing sub-tropical native pastures to phosphorus supplementation with loose mineral mix or feed blocks. The Veterinary Journal 298–299, 106013.
| Crossref | Google Scholar | PubMed |
Seeman E (2013) Age- and menopause-related bone loss compromise cortical and trabecular microstructure. Journal of Gerontology A. Biological Sciences and Medical Sciences 68, 1218-1225.
| Crossref | Google Scholar |
Suttle NF, Armstrong DG, Braithwaite GD, Field AC, Scott D, Thompson JK, Woolliams J (1991) A reappraisal of the calcium and phosphorus requirements of sheep and cattle. In ‘Agricultural and Food Research Council: Technical Committee on Responses to Nutrients Report No. 6. pp. 573–612. Nutrition Abstracts and Reviews B: Feeds and Feedstuffs. (CAB International)
Taylor MS, Knowlton KF, McGilliard ML, Swecker WS, Ferguson JD, Wu Z, Hanigan MD (2009) Dietary calcium has little effect on mineral balance and bone mineral metabolism through twenty weeks of lactation in Holstein cows. Journal of Dairy Science 92, 223-237.
| Crossref | Google Scholar | PubMed |
Ternouth JH, Coates DB (1997) Phosphorus homoeostasis in grazing breeder cattle. Journal of Agricultural Science 128, 331-337.
| Crossref | Google Scholar |
Ternouth JH, Sevilla CC (1990) The effects of low levels of dietary phosphorus upon the dry matter intake and metabolism of lambs. Australian Journal of Agricultural Research 41, 175-184.
| Crossref | Google Scholar |
Theiler A, Green HH, Du Toit PJ (1927) Minimum mineral requirements in cattle. Journal of Agricultural Science 17, 291-314.
| Crossref | Google Scholar |
Theiler A, Du Toit PJ, Malan AI (1937) Studies in mineral metabolism. XXXVII. The influence of variations in the dietary phosphorus and in the Ca:P ratio on the production of rickets in cattle. Onderstepoort Journal of Veterinary Science and Animal Industry 8, 375-414.
| Google Scholar |
Tuen AA, Wadsworth JC, Murray M (1984) Absorption of calcium and phosphorus by growing cattle during dietary protein deficiency. Proceedings of the Nutrition Society of Australia 9, 144-147.
| Google Scholar |
Valk H, Sebek LBJ, Beynen AC (2002) Influence of phosphorus intake on excretion and blood plasma and saliva concentrations of phosphorus in dairy cows. Journal of Dairy Science 85, 2642-2649.
| Crossref | Google Scholar |
Van Mosel M, Wouterse HS, van’t Klooster AT (1994) Effects of reducing dietary ([Na+ + K+] – [Cl− + SO4=]) on bone in dairy cows at parturition. Research in Veterinary Science 56, 270-276.
| Crossref | Google Scholar | PubMed |
Wächter S, Cohrs I, Golbeck L, Wilkens MR, Grünberg W (2021) Effects of restricted dietary phosphorus supply to dry cows on periparturient calcium status. Journal of Dairy Science 105, 748-760.
| Crossref | Google Scholar | PubMed |
Wadsworth JC, McLean RW, Coates DB, Winter WH (1990) Phosphorus and beef production in northern Australia. 5. Animal phosphorus status and diagnosis. Tropical Grasslands 24, 185-196.
| Google Scholar |
Winter EM, Ireland A, Butterfield NC, Haffner-Luntzer M, Horcajada M-N, Veldhuis-Vlug AG, Oei L, Colaianni G, Bonnet N (2020) Pregnancy and lactation, a challenge for the skeleton. Endocrine Connections 9(6), R143–R157. 10.1530/EC-20-0055
Young VR, Richards WP, Lofgreen GP, Luick JR (1966) Phosphorus depletion in sheep and the ratio of calcium and phosphorus in the diet with reference to calcium and phosphorus absorption. British Journal of Nutrition 20, 783-794.
| Crossref | Google Scholar | PubMed |