Balancing future food security and greenhouse-gas emissions from animal-sourced protein foods in Southeast Asia
R. S. Hegarty


A
B
C
D
E
F
G
H
Abstract
Southeast Asia’s human population is expected to rise by 100 million between 2023 and 2050, with an associated rise in animal-product output in the region’s low- to middle-income countries. Countries with the largest population are forecast to continue their increasing poultry consumption, with regional pig meat consumption also to rise, but much less than in China to the north, and much less in Muslim-dominant countries. The forecast growth in the regional ruminant population is more modest and the farm-gate greenhouse gas (GHG) cost per unit of human food protein generated is much higher for ruminant meat (203–584 kg carbon dioxide equivalents (CO2e)/kg protein) than for pig meat (18 kg/kg) or poultry (4 kg/kg). Changing human diets away from ruminant or any animal-sourced protein, is being explored to increase the human food supply at a lower GHG cost. However, with small-holder livestock production systems dominant across many regional countries, the social, land-use and broader economic roles of ruminants need consideration. Strategies to expand ruminant production but with a reduced GHG unit cost (emission intensity) are being pursued. Increasing individual animal-product output, largely through simple animal health and nutritional management decisions, can allow future food targets to be met at a lower GHG emission than if this additional food was produced by business-as-usual livestock production systems. Because the Paris Agreement recognises the priority of food provision over emission abatement, it seems reasonable that much of Southeast Asia should pursue emission intensity targets more than absolute emission targets, and reflect this in their nationally determined contributions (NDCs). Emission-intensity intentions are already apparent not just in NDCs but in emerging carbon markets.
Keywords: emission intensity, enteric methane, food security, greenhouse gas, livestock, nutrition, productivity, Southeast Asia.
Introduction
Although the annual percentage growth of the global population is expected to decline between now and 2050, the total number of persons is expected to rise (UNDESA 2022), and in Southeast Asia (SE Asia), the current population of 620 million is expected to rise by approximately 100 million by 2050. This increasaed population will substantially increase regional food demand. Recent data (FAOSTAT 2024) show that SE Asia has 41.7 million (6.1%) people experiencing under-nutrition, with the majority of SE Asia peoples living in low- to middle-income countries (World Bank classification). The general trend of animal protein being of increasing importance as the per-capita income increases (the ‘nutrition transition’, or ‘protein transition’) is as real in SE Asia, as it is in the rest of the developing world (Drewnowski and Poulain 2018). There is generally a slow rise in total productivity by agriculture across SE Asian nations, but even so, agricultural growth lags behind the average economic growth in the region (Liu et al. 2020). Increased animal-product output is anticipated in major SE Asian nations (e.g. Tenrisanna and Kasim 2020) and particularly in the low- and low–middle-income countries (OECD/FAO 2023); so, increased emissions can also be anticipated from both ruminant and non-ruminant livestock systems. Animal production in low-income countries typically has a much higher emission intensity than that in high-income countries (Bateki et al. 2023; FAOSTAT 2023a); so, increasing animal production while reducing greenhouse-gas (GHG) emissions is a challenge for the region. The response will entail a move towards poultry and reduced growth in ruminant meat (OECD/FAO 2023), but the ruminant industries must work to reduce total climate-changing GHG emissions while increasing animal-product output. Whereas the effects of a changing climate on food security have been a focus for livestock development (Mbow et al. 2019), less effort has been invested in evaluating how the food-producing industries (especially livestock) in the global south can be managed to reduce their contribution to climate change, while increasing food supply (FAO 2023a). In this article, we explore the role and future of ruminants in SE Asian animal production, as well as management strategies to control enteric GHG emissions, and the importance of the emission-intensity metric in achieving both climate and food-security goals, while being consistent with emerging carbon-credit schemes.
Population and future food demand in Asia/Southeast Asia
Of the world’s population of 7.8 billion, 4.7 billion are in Asia, with 620 million living in SE Asia (ESCAP 2023; World Bank 2023), of which 41.7 million were reported to be undernourished in 2023 (FAOSTAT 2024). The global and SE Asian populations are expected to increase to 8.6 billion and 720 million respectively, by 2050. The increase in the population in SE Asia will directly affect the regional and, thus, global food demand in the future. In addition, the associated demographic changes will also influence future demand for food through income growth, driven by socio-cultural and lifestyle changes as a result of urbanisation. This will lead to an increase in the female workforce, strengthening household income and overall purchasing power.
Global demand for agricultural commodities (including for non-food uses) is projected to grow at 1.1% p.a. over the current decade (2022–2031), well below the 2% p.a. growth experienced over the previous decade (2012–2021), with global food demand projected to increase by 1.4% p.a. over this period, owing to population and per-capita income growth. However, growth will not be uniform across different economic groups, with most additional demand for food continuing to come from low- and middle-income countries in Asia, including most of the SE Asian countries. In low-income countries, the average food consumption per head per day is projected to increase by 7% over the decade to 2031, the largest gain of all the income groups. Average diets in low income countries will remain heavily based on staples (cereals, roots, tubers), which are projected to account for more than half of additional calories by 2031 (Fig. 1). However, middle-income countries are expected to increase their food consumption and diversify their diets in the coming decade. Staples and animal products will account for two-thirds of this increase, and fats for 18%. The projected increase in fat consumption in middle-income countries is underpinned by ongoing urbanisation and changing lifestyles (e.g. increasing tendency to eat outside the home), which favour higher consumption of processed and convenience foods. In upper-middle income countries, per capita food consumption is expected to increase by 4% by 2031 (Fig. 1; OECD/FAO 2023). Given the expected high income growth and the strong preference to consume more meat in several of these countries, including China to the north of SE Asia, 45% of additional calories will be provided by animal products and 20% by fats.
Per human-capita calorie availability in the main food groups by country income groupA at start and end of the decadeBC. Source: OECD/FAO (2022). FAOSTAT Food Balances Database, http://www.fao.org/faostat/en/#data/FBS; OECD/FAO (2023), ‘OECD–FAO Agricultural Outlook’, OECD Agriculture Statistics (database), http://dx.doi.org/10.1787/agr-outl-data-en. A‘Staple foods are those eaten regularly, and in such quantities as to constitute the dominant part of the diet and supply a major proportion of total dietary energy. The main kinds of staple foods are cereals (e.g. rice, maize, wheat, rye, barley, oats, millet, sorghum), roots and tubers (e.g. potatoes, cassava, yams) and legumes (e.g. beans, lentils, soybean).’ FAO glossary: https://openknowledge.fao.org/server/api/core/bitstreams/f1ee0c49-04e7-43df-9b83-6820f4f37ca9/content/state-food-security-and-nutrition-2023/annexes11.html#:~:text=Staple%20foods,proportion%20of%20total%20dietary%20energy. BRespective per-capita income in 2018. The applied thresholds are: low, <US$1550; lower-middle, <US$3895; upper-middle, <US$13,000; and high, >US$13,000. CFats are defined as butter and vegetable oils (OECD/FAO 2022).
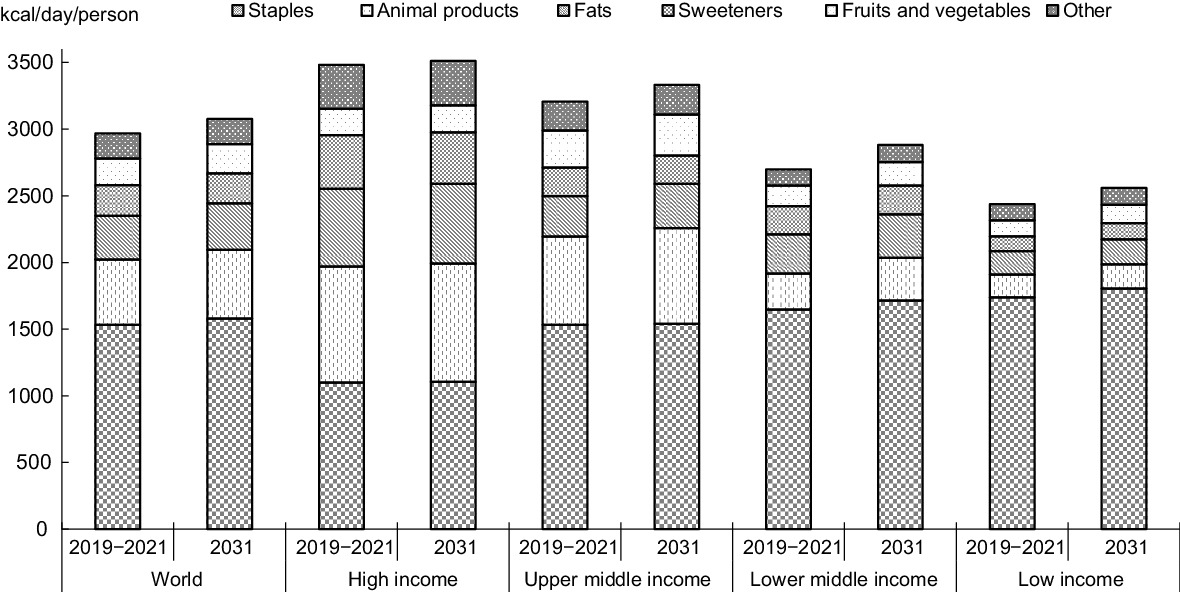
Although affluence and poverty are found in all countries, lower-middle-income countries (Indonesia, Vietnam, Philippines, Cambodia and Laos) are home to 82% of SE Asia’s 620 million people, whereas 17% of the population live in higher-middle-income countries (Malaysia and Thailand) and only 1% live in a high income-bracket country (Singapore). Thus, food demand from SE Asia will increase at a high rate over the next decade and beyond, until these countries enjoy the status of high-income countries. Indonesia is the most populated nation (273 million) followed by the Philippines (115 million), Vietnam (97.5 million), Thailand (71.6 million), with the remaining countries being less populated (Table 1). The global food-security index (GFSI) is a metric to compare food security across countries on the basis of affordability, availability, quality, safety, sustainability and adaptation (GFSI 2022). The index accounts not just for current supply, but for the vulnerability to price and supply-chain risks associated with that supply, hence reflecting food security, not just availability, with a high index being desirable. In 2022, only one regional country (Singapore) scored within the top one-third of the 113 participating countries, two countries (Malaysia and Vietnam) were within the top 50%, whereas the remaining countries were within the top 75%. Most often ASEAN countries scored very poorly for food availability (more than for food affordability or quality and safety). This indicates the importance of addressing food security in SE Asia in the coming decade.
Country | Population (million) | Income level | GFSI rank (score) | Livestock-related emissions (kt CO2e) | ||
---|---|---|---|---|---|---|
Enteric | Manure | |||||
Singapore | 5.9 | High | 28 (73.1) | 0.3 | 19.3 | |
Malaysia | 33.6 | Higher-middle | 41 (69.9) | 1161 | 2213 | |
Thailand | 71.6 | Higher-middle | 64 (60.1) | 7668 | 5904 | |
Vietnam | 97.5 | Lower-middle | 46 (67.9) | 13,115 | 12,110 | |
Indonesia | 273 | Lower-middle | 63 (60.2) | 31,555 | 28,257 | |
Philippines | 115 | Lower-middle | 67 (59.3) | 8767 | 6308 | |
Cambodia | 16.7 | Lower-middle | 78 (55.7) | 4731 | 2368 | |
Laos | 7.4 | Lower-middle | 81 (53.1) | 1564 | 2893 | |
Brunei | 0.45 | No data | No data | 3.8 | 86.9 | |
Myanmar | 53.8 | Lower middle | 72 (57.6) | 19,385 | 10,145 | |
Timor-Leste | 1.32 | Lower-middle | No data | No data | No data |
Income-level data are from https://blogs.worldbank.org/opendata/new-world-bank-country-classifications-income-level-2022-2023.
GFSI source: GFSI-UN (2023).
Livestock related emission data are from FAOSTAT (AR5) data for 2021. Manure emission include manure managed + left on pasture + applied to soils. CO2e, carbon dioxide equivalents.
As in many low- and middle-income countries of other continents, urban migration for economic reasons is common in SE Asia. For example, in Indonesia (the most populated country in SE Asia), the urban population made up only 22.4% of the total population in 1980 but grew to 50.3% in 2006 and is predicted to reach 60% by 2025 (Widarjono and Rucbha 2016). Because of its rapid growth in population and purchasing power, Indonesia expects its food demand to quadruple by 2050, particularly for higher-value foods such as meat, dairy, fruit, and vegetables. To achieve food self-sufficiency, Indonesia calls for a reduction in most food imports, reflecting a rejection of the call for free and internationalised markets by economic liberalism. Food self-sufficiency policy in the Indonesian context is a national-level objective that is silent about distribution, individual access to food, food affordability or other domestic distributional considerations, focussing on the goal of increased national production and reduced reliance on imported food (Hamilton-Hart 2019).
Recent and future role of animal products in the Southeast Asian food basket
Presently, 5 of the 10 ASEAN countries import more than US$1 billion of meat and edible meat offal (excluding live animal imports) annually. For these countries, the value of their meat and edible meat offal imports is from 9 (Singapore) to 912 (Philippines) times the value of meat and edible meat-offal exports, showing their national dependence on meat imports (ASEANstats 2023). As lower-middle-income countries work to increase the availability of food to meet the expected increased future demand, it is assumed that the structure of diets in lower-middle-income countries will change as food consumption increases, with a growing share of animal-source foods in their diet as part of the protein transition.
Growth in global consumption of meat is projected to increase by 14% by 2030, compared with the average of 2018–2020, driven largely by income and population growth (Fig. 2). In the Asia and Pacific region, meat consumption is projected to grow by four percentage points more (18%) than that globally. Globally, poultry meat is expected to represent 41% of all the protein from meat sources in 2030, an increase of two percentage points when compared with 2020 (Fig. 3). The global shares of other meat products are lower, with pork at 34%, and ruminant sourced meat at 25%, of which beef is 20% and sheep and goat meat together are 5%.
Growth in meat production and per human-capita consumed on a protein basis, 2019–2021 to 2031, by countries for different income groups. Note: the 38 individual countries and 11 regional aggregates in the baseline are classified into the four income groups according to their respective per-capita income in 2018. The applied thresholds are: low <US$1550, lower-middle: <US$3895, upper-middle: <US$13,000, high >US$13,000. Source: OECD/FAO (2022), ‘OECD–FAO Agricultural Outlook’, OECD Agriculture Statistics (database). http://dx.doi.org/10.1787/agr-outl-data-en.
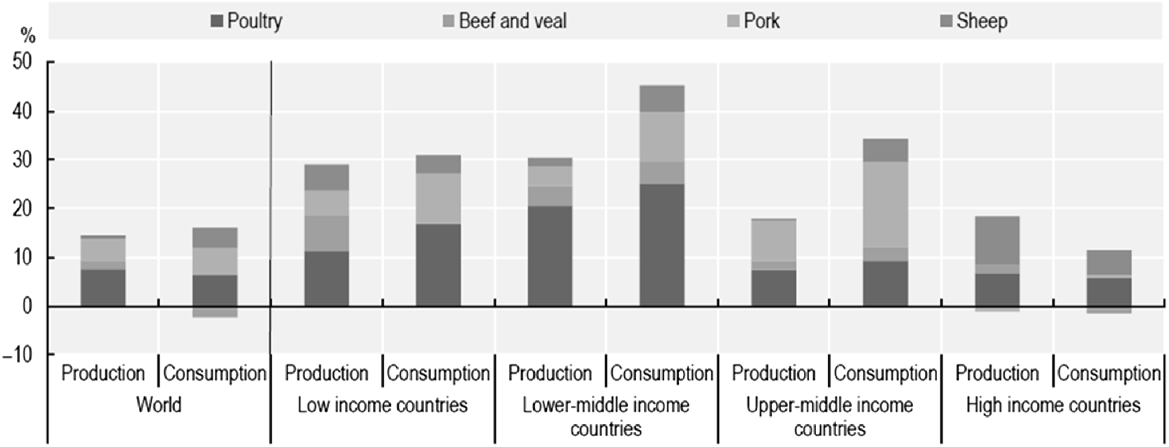
Projected meat consumption per human capita by global region, showing a continued rise of poultry and pig meat consumption relative to beef and sheep meat. Source: OECD/FAO (2022), ‘OECD–FAO Agricultural Outlook’, OECD Agriculture Statistics (database). http://dx.doi.org/10.1787/agr-outl-data-en. Note: Per-capita consumption is expressed in retail weight.
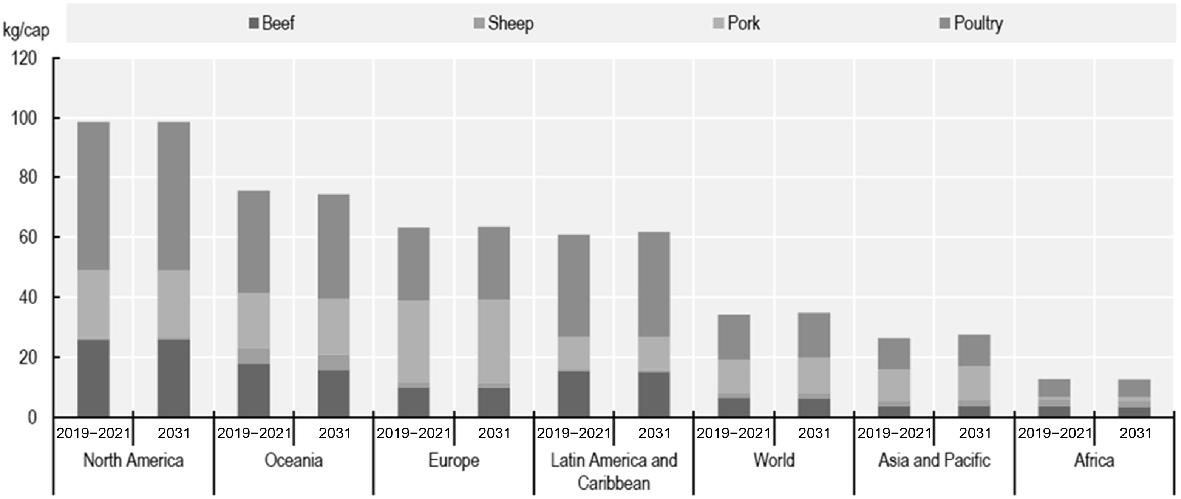
A clear trend is the rise of poultry meat consumption in virtually all countries and regions. On a per-capita basis, the expected robust growth rates in poultry consumption reflect the significant role of poultry in the national diets of several populous developing countries, including China, India and the SE Asian countries. In lower-income countries, the lower price of poultry than other meats is pivotal, whereas in high-income countries, an increased preference for white meats reflects the fact that they are more convenient to prepare and are being perceived as a healthier food choice (OECD/FAO 2023).
Approximately one-third of the overall increase in meat consumption projected until 2030 is attributed to pig meat, with China accounting for 70% of this decadal increase in pig meat consumption (OECD/FAO 2022). Although declining in most developed countries, in developing countries, the per-capita consumption of pig meat, which is half that in developed countries, is expected to increase marginally. Several Asian countries where pork is traditionally consumed, are projected to increase consumption on a per-capita basis, whereas the majority of the population in Indonesia and Malaysia (making up about half of the population in SE Asia) are Muslim who do not consume pork. Whereas pork consumption in these two countries is expected to remain low relative to other major meat sources and unchanged, it is a policy of some governments to increase cattle production to supply the expected increase in local demand for beef and milk (Pham-Thanh et al. 2020; MAFI 2021; Nasution et al. 2021).
Production of ruminants, particularly cattle, in most SE Asian countries is less efficient and does not fulfil the local meat demand, as compared with local poultry and pig production. To meet the current and future local demand for beef, most of these countries depend on importation (Fig. 4).
Balance of past and future national domestic beef production and beef imports across SE Asia. Source: OECD/FAO (2022), ‘OECD–FAO Agricultural Outlook’, OECD Agricultural Statistics (database). http://dx.doi.org/10.1787/agr-outl-data-en.
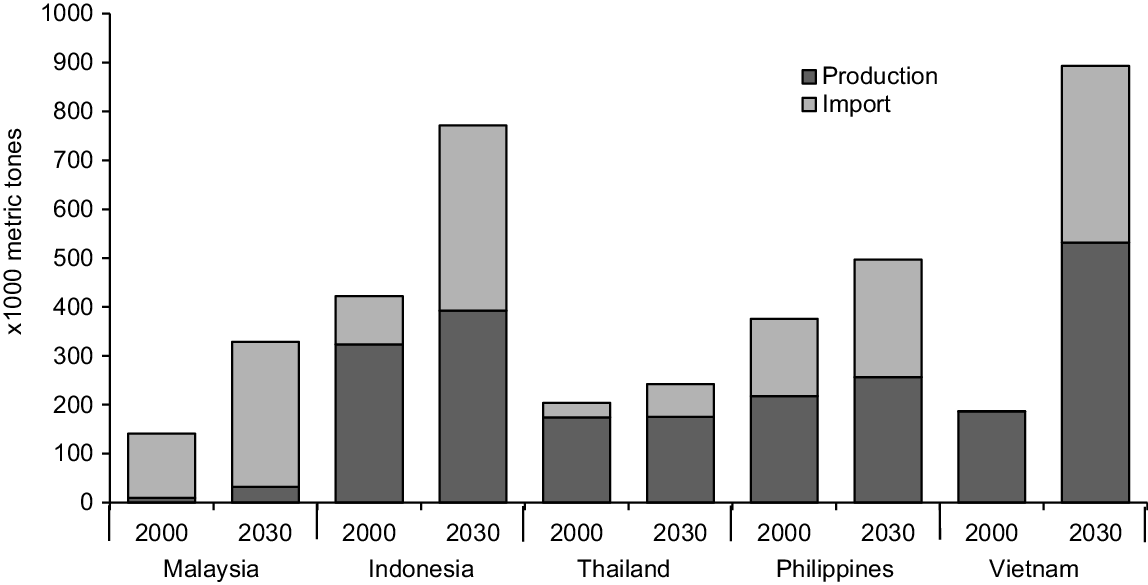
Roles of livestock beyond animal-sourced food
As in many low- and lower-middle-income countries in other continents (e.g. Africa), livestock in SE Asia are raised for roles besides supplying meat and milk, including providing land management, draught and income/security (FAO 2018). Because the majority of the population living in rural areas in SE Asia rely on farming, many of these smallholder farmers integrate ruminants (cattle, goats and sheep) with their arable-farming activities. Keeping cattle and small ruminants is traditionally considered as an investment for the small farmers, with livestock being sold when there is a need for cash for the family (Schmidt 1992). Some farmers consider that owning cattle provides a sense of pride and position, with the more cattle they have, the higher is their social status in the village (Rustinsyah 2019).
Draught has been a major use of large ruminants in some industries but has much decreased in SE Asia in the rice industry because of mechanisation, although this change is poorly documented (Mota-Rojas et al. 2021). In palm oil production, of which Indonesia and Malaysia are the world’s principal providers, buffalo remains important for draught. In Malaysia, nearly 27% of the Malaysian oil palm plantations are owned by 440,000 smallholder farmers. Harvesting and transporting oil palm fruit bunches to the collection points within the plantation are tedious tasks. Using water buffaloes and cattle to assist farmers in transporting the harvested fruit bunches increased harvesters’ income by 30% (Sarmin and Liang 1992). In addition, this system can foster agricultural and livestock entrepreneurship among smallholder farmers to increase their income. In addition to income generation, integrating palm oil production and livestock may offer several benefits; including carbon sequestration, topsoil restoration, improved ecosystem biodiversity, and reduced pesticide and fertilizer use (Henson et al. 2012; Besar et al. 2020; Álvarez et al. 2024).
Silvopastoral systems with tree crops properly integrated with livestock at appropriate stocking densities and feed availability can mitigate soil degradation, restore a healthy ecosystem and allow carbon to be sequestered deep in the soil (Torres et al. 2017).
Non-ruminant livestock are increasingly also serving multiple farm roles in SE Asia because circular agriculture is increasingly being promoted to deliver on multiple sustainable-development goals (FAO 2018; UNDESA 2021). Pig manure is increasingly being viewed as a fertilizer asset in smallholder systems and for biogas in smallholder and commercial systems (Tiemann and Douxchamps 2023). Raising black soldier fly larvae for fish or poultry feed on waste from household, chicken, pig or plant wastes (da Silva and Hesselberg 2020) is also diversifying income streams for smallholders, enabling greater financial security and business assurance.
GHG footprint of ruminants in Asia/Southeast Asia
Tubiello et al. (2022) reported that one-third of all anthropogenic GHG emissions can be attributed to agrifood systems. This GHG emissions are allocated to categories of farm gate (e.g. crop and livestock production activities), land-use change (e.g. deforestation and peatland drainage for agriculture), and pre- and post-production processes (e.g. food manufacturing, retail, household consumption and food disposal). Overall, Asian food-system emissions are 46% farm gate, 13% land-use change (LUC) and 42% pre- and post-production process sources (Tubiello et al. 2022). Globally, the LUC emissions from food system are declining, whereas in Indonesia the LUC emissions at 51% of total food-system emissions still exceed farm-gate emissions. Hence, there is a clear case for considering livestock’s role in LUC, especially in cases such as grazing cattle under coconut or oil palms on recently cleared forest country (Álvarez et al. 2024). However, because LUC emissions are not ascribed to livestock in inventory, discussion below is largely restricted to farm-gate emissions, which includes methane from livestock enteric and manure sources and nitrous oxide from manure, managed, as well as that left on pasture or applied to soils. In some cases, reference is made to publications referring only to enteric methane emissions. Emission intensity (EI; units GHG/unit animal product) is based on farm-gate emissions (including nitrous oxide, N2O), unless restricted to methane, in which case it is called methane intensity.
Total farm-gate GHG emissions (Tg CO2e) allocated to livestock-sourced commodities in SE Asia doubled over the past four decades, from 93 Tg CO2e in 1980 to 186 Tg CO2e in 2020 (1000 Tg = 1 Gg; FAOSTAT 2023a; Fig. 5a), mainly from meat-producing livestock (note that all FAOSTAT estimates cited rely on using AR5 global-warming potentials and 100-year time horizon). The large ruminants (cattle and buffaloes) were the main contributors of livestock GHG, together contributing 86.4% and 72.2% of CO2e of livestock emissions for 1980 and 2020 respectively, with cattle being the primary contributors. It is noted that that proportion of livestock GHG contributed by buffaloes declined by 22.6 percentage points, from 37.7% in 1980 to 15.1% of CO2e livestock emissions in 2020 reflecting the decline in buffalo population in almost all the SE Asian countries (16.75 million head in 1980 to 11.42 million in 2020; FAOSTAT 2023a). This decline is because their main function as draught power for rice production has largely been replaced by modern farm machinery. The overall decline in the buffalo population in SE Asia (Fig. 5a), could be viewed as a positive factor in reducing GHG emission from livestock in the region because of the high EI of buffaloes, but they are culturally important, especially in the Philippines.
Percentages of the GHG emissions arising from Southeast Asian livestock (a) by source animal, (b) by source of meat and (c) by source of milk and egg production, in 1980 and 2020. (FAOSTAT 2023a).
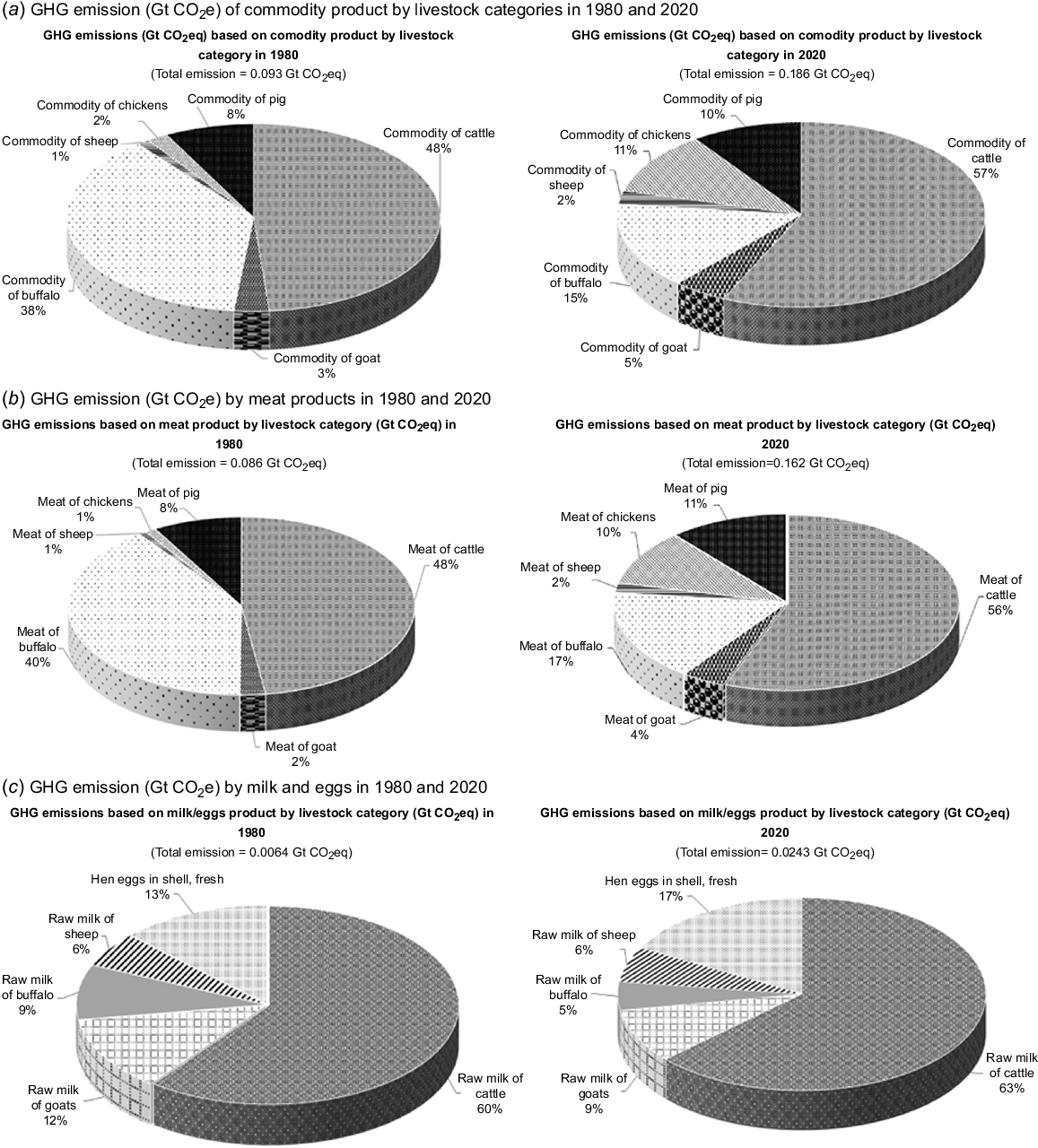
Total farm-gate GHG emissions from small ruminants, particularly goats, increased from 4.1% to 7.1% of CO2e over the past 40 years. Of total SE Asian livestock GHG emissions, the proportion contributed by non-ruminant livestock (pigs and chickens) was 9.5% of livestock CO2e emissions in 1980 and 21.5% of CO2e in 2020 (Fig. 5a–c). The increase was primarily from expansion of the poultry sector, which recorded a 9.3 percentage point rise in emissions, consistent with the increased consumption of poultry meat products as the economic status of low- and middle-income countries improves. This trend is also expected to continue into the coming century (FAO 2023a). SE Asian emissions (CO2e) from pig production recorded only a 2.1 percentage point increase over the past 40 years, reflecting that the majority of the population of two agriculture-based SE Asian countries Indonesia (with the largest human population in SE Asia) and its neighbour, Malaysia, are predominantly Muslims, and so consume little pork, leading to almost no rise in pork consumption in these two countries.
Emission intensities of livestock products by category for Southeast Asia
One of the great weaknesses of the lower-income countries is the high GHG cost of producing animal products, especially meat, being most apparent for beef. Historically EI of beef and veal production by low-income countries is approximately seven times that of the high-income countries (Fig. 6; GHG included are enteric CH4 and CH4 and N2O from managed, on-pasture and spread manures), identifying a large scope for practice change to reduce EI that is completely consistent with improving production efficiency of SE Asian animals. The EI of non-ruminant meat is much lower, being 1.1 kg CO2e for poultry meat compared with 57.0 for beef and veal produced in lower-middle-income countries in 2022 OECD/FAO (2022).
Average emission intensity of beef and veal produced in countries differing in national income. Source: OECD/FAO (2022), ‘OECD–FAO Agricultural Outlook’, OECD Agriculture Statistics (database). http://dx.doi.org/10.1787/agr-outl-data-en.
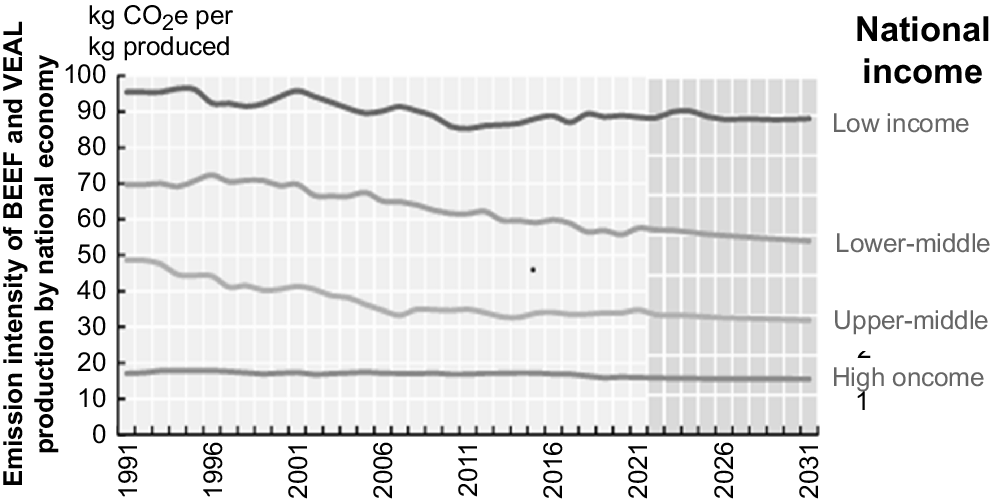
Human nutritionists moderate the EI to report emissions per kilogram of food protein. Chang et al. (2021) used data from 2014 to 2018 to show that methane intensity per kilogram of protein produced was much lower for poultry meat and eggs (<0.1 kg CH4/kg protein) or for pigs (0.3–0.5 kg CH4/kg protein) than the high 3.5–5.5 kg CH4/kg protein for ruminant meat protein. In this paper, GHG-emission (kilogram CO2e; farm gate) data for SE Asia (total data) were acquired from FAOSTAT production statistics for meat, milk and eggs (FAOSTAT 2023a) to study the change over recent decades of animal food-protein EI, with emissions for the single gas components for carbon dioxide (CO2), methane (CH4), and N2O, as well as their combined measurement as CO2e. This FAOSTAT domain of emission intensities (including enteric fermentation and manure-management emissions) encompasses analytical data on the intensity of GHG by agricultural commodity (FAOSTAT 2023b). The emission indicator is expressed in kilograms CO2e per kilogram of product. Further, a new estimate of emission intensities, expressed as farm-gate CO2e emissions per kilogram of protein in products with milk/egg and meat from cattle, buffaloes, goats and sheep, plus meat from swine, and meat and eggs from poultry, was derived by the authors. This was calculated by multiplying the protein content of animal products as reported in table 9.1 of the GLEAM v2.0 Documentation (FAO 2017) and as used by Chang et al. (2021), by the EI of those products (kilograms CO2e per kilogram animal product) to get an EI of kilogram of protein from each livestock product per kilogram CO2e, where the CO2e included all on-farm emissions associated with production, not just CH4 as was used by Chang et al. (2021). The summary statistics compiled in Table 2 focus on the SE Asian emission intensities of livestock categories for the period 2010 and 2020.
Product | 2010 | 2020 | |||||
---|---|---|---|---|---|---|---|
Emissions (Gg/year) | Product protein output (Gg/year) | Emission intensity of protein produced | Emissions (Gg/year) | Product protein output (Gg/year) | Emission intensity of protein produced | ||
Cattle meat | 79,493 | 225.0 | 353 | 90,547 | 269.4 | 336 | |
Buffalo meat | 29,742 | 57.7 | 515 | 27,006 | 46.2 | 584 | |
Sheep meat | 1649 | 7.3 | 226 | 2872 | 11.3 | 253 | |
Goat meat | 4623 | 24.0 | 193 | 6656 | 32.9 | 203 | |
Pig meat | 16,782 | 889 | 19 | 18,176 | 1017.2 | 18 | |
Cattle milk | 10,999 | 116.1 | 95 | 15,327 | 182.2 | 84 | |
Buffalo milk | 1198 | 15.7 | 76 | 1154 | 10.9 | 106 | |
Sheep milk | 912 | 4.0 | 228 | 1442 | 5.6 | 256 | |
Goats milk | 1731 | 11.6 | 149 | 2202 | 13.2 | 167 | |
Eggs | 2995 | 425.5 | 7 | 4128 | 1029.1 | 4 |
Based on FAOSTAT farm-gate multi-gas emission (AR5, 100-year time horizon) and product data and product protein contents of Chang et al. (2021).
Messages apparent from the production and EI data developed and displayed in Table 2 include the following: first, egg protein offers the lowest GHG-cost animal-sourced protein for the food basket (4 kg CO2e/kg protein). Eggs are also the animal-sourced protein, showing the greatest rate of production rise, more than doubling in the 2010–2020 decade. Second, of the ruminant proteins, milk protein has a far lower GHG cost than has beef or veal protein, consistent with the promotional effort to stimulate milk consumption in many SE Asian countries. While the proportional rise in SE Asian milk production over the decade is greater than that for meat from large ruminants, it comes from a small initial production, so is still a small industry, with countries such as Laos and Cambodia with very few dairies. Third, there was no consistent change in EI across the regional ruminant industries in the 2010–2020 decade. Although this may reflect the simplicity of Tier 1 GHG emission factors in FAOSTAT, it also suggests that individual animal productivity in the large ruminant industries did not rise markedly over the decade, or EI would have declined.
Management options to increase protein supply without raising GHG emissions
Changing human diets away from ruminant-sourced foods
While the protein transition describes the positive association between increased consumption of animal protein with increasing income that applies to much of SE Asia, there is a global drive to reduce the dietary inclusion of animal products as part of healthy diets from sustainable food systems (Willett et al. 2019; World Economic Forum 2023). These studies suggest that diets containing more plant-based foods also improved the health of consumers, consistent with previous studies linking red meat consumption with more deaths and a greater risk to the global burden of disease (Murray et al. 2020). If diet preferences changed to the EAT–Lancet target, cumulative GHG emissions could be reduced (Willett et al. 2019). Changing to a more plant-based diet resulted in positive environmental impact as vegetarian diets produce less GHG emissions (Zhang et al. 2022). This was associated with an emission reductions of approximately 41.9%, with the most significant GHG emission reductions observed in eastern Asia (54%), central Asia (72%) and the developed country regions (58%), but with little reduction in SE Asia.
Whereas reducing animal protein in diets is one component in sustainably feeding the future world population promoted by the EAT–Lancet Commission, there is a strong need to recognise the nutritional strengths of animal products over plant products for multiple minor nutrients as identified in the Dublin Declaration (Pethick et al. 2023). Much of the growth in animal protein supply into the food-basket of lower-middle-income countries in SE Asia will come from increased poultry production (with little addition from ruminants), and animal protein as a proportion of total protein in the diet will remain low, being 12%, in 2030 (Chang et al. 2021). This gives ample opportunity to manage the supply of animal proteins and ruminant proteins, in particular, by producing similar animal protein output with reduced GHG emissions in these countries.
Lowering the emission intensity of ruminant products by making animals more productive
Livestock management changes that will enable a country to both increase food supply and security while reducing GHG emissions are desirable, because they would support the poverty, hunger and environmental sustainable development goals. The foundation for increasing product output from ruminant systems is to achieve the highest feasible reproduction rate, because it eliminates retention of female livestock that produce GHGs for little or no saleable product (Hristov et al. 2013a).
All SE Asian countries fall within tropical or subtropical climates that lead to large seasonal variations in available feed and animal characteristics. The majority of ruminants are raised by smallholder farmers, who employ diverse feeding and husbandry practices (Tee et al. 2022; Bush 2024; Hilmiati et al. 2024). These practices include grazing on available forages around villages or under perennial crops, as well as using cut forages in sheds or feedlots, with or without supplementation from agricultural by-products. This mixed feeding system, often based on low-quality diets, contributes to the high GHG emissions and EI of beef in the Southeast Asian ruminant sector (Tee et al. 2022).
High temperatures and humidity in SE Asia frequently cause heat stress, negatively affecting livestock production. Countries including Thailand, Malaysia, and Indonesia experience reduced reproductive rates in cattle, particularly in crossbred animals, as a result of heat stress (Fathoni et al. 2022) and associated health issues (Bush 2024). To address these challenges, several management strategies have been implemented to improve reproductive output. These include genetic-selection programs that focus on high-fertility and heat-tolerant breeds (Burrow 2015). The adoption of artificial insemination has provided access to superior genetics, optimizing breeding outcomes. Also, nutritional management, emphasizing balanced feed and mineral supplementation, has enhanced fertility and overall cattle health. Regular disease-prevention measures, such as vaccination and parasite control, have minimized reproductive losses (Horri et al. 2023). Advanced record-keeping systems enable better monitoring of reproductive cycles, facilitating timely interventions and heat-detection technologies that have also improved conception rates. Furthermore, enhanced forage quality, and improved housing infrastructure with better environmental management (such as providing shade and cooling sprinklers), have collectively reduced heat stress and supported reproductive performance for sustainable husbandry practices (Syarifuddin et al. 2024).
A study of Indonesian production systems also showed that reproductive performance (Mayberry et al. 2016) seriously constrained beef output, creating pressure to grow the slaughter stock more quickly through better management to maintain business viability, and this is also true in less extensive but equally seasonal environments in SE Asia (e.g. Indonesia, Poppi et al. 2017; Laos, LQBI 2019). Management changes that are desirable for animal performance are also desirable for reducing the EI of beef or animal products. For example, Arndt et al. (2021) assessed the feasibility of global livestock industries achieving the 11% mitigation identified as a target in the IPCC ‘Global Warming of 1.5°C’ report (IPCC 2018). It is of note that the three leading product-based reduction strategies were increasing feeding level, decreasing grass maturity and decreasing the dietary forage to concentrate ratio. These are approaches that primarily increase the productivity of the individual animal, by enabling it to increase its metabolisable energy intake, thus having an increased proportion of consumed energy available for productive purposes and a reduced proportion of consumed energy being invested in body maintenance. More recently, FAO (FAO 2023a) sought to identify low-emission livestock-production pathways, and increaseing animal productivity is a key strategy in this, anticipating that sustained productivity improvement could potentially reduce emissions by 24% compared with business as usual by 2050.
Over an enterprise, and often for each individual animal whose productivity is increased, these reductions in EI are associated with an increase in daily GHG emissions if animal numbers are unchanged (Gebbels et al. 2022). The question of whether the farmer or government should then reduce the number of animals to keep product output the same for a reduced GHG emission, or whether the country opts to maintain animal numbers for a greater total animal product but also more total GHG emissions, is a policy decision, it is not a scientific question.
Management decisions that increase animal productivity to reduce the EI of animal products primarily provide the livestock owner or the country with flexibility in managing GHG and food supply. Knowing that product output per animal can be increased proportionally more than total GHG output per animal through improving animal productivity, the following three options become available:
The producer or country can produce the same amount of food from fewer animals and with less absolute GHG emissions. By increasing animal product/unit of land used, this will release land currently under livestock to be used for other purposes (e.g. cropping, forestry, urban expansion).
The producer can keep animal numbers the same, greatly increasing animal product output and slightly increase absolute GHG output. In a country facing under-nourishment and with low emission penalties and mitigation ambitions, this is highly attractive.
An intermediate position is very possible. A situation where there is some reduction in livestock numbers and either a reduction or no change in absolute GHG emissions, but output of animal product is increased. The following section identifies nutritional management opportunities to give such productivity gain and flexibility for managing emissions versus food-supply goals.
Replacement of pastures with species offering greater nutritional value, biomass production or improved dry-season production is one management change that offers the flexibility described above. Expanded replacement of native grazing lands with improved forage (for grazing or cut and carry feeding) is seen as fundamental to future livestock development in Cambodia and Laos, with the Asia Development Bank about to implement 18,000 ha of improved-forage planting in Cambodia (https://www.adb.org/projects/53240-003/main#project-pds) and a smaller development in Laos. A simulation of the effects of replacing native pasture with improved pastures in Australia demonstrated the opportunity to differentially deliver on food and emission goals, subject to personal or policy priorities, and allowed the manager the three options described above (Alcock and Hegarty 2006, 2011).
The findings from the simulation were, that if the landowner commencing with 100 ha of unimproved grazing land wanted to pasture-improve and sustainably graze an area with the objective of maintaining the same profit as was obtained from the original area of unimproved pasture, they need to improve only 24% of the area for pasture, leaving over 75% of the land area to be used for other purposes. If the objective was to generate more animal output and more profit while not changing the absolute amount of livestock CH4 emissions, this was achievable by improving pasture on 43% of pasture, retiring 57% of the land area from grazing, while also resulting in an almost four-fold increase in profit without changing enteric CH4 output of the enterprise relative to grazing 100% of area as unimproved pasture. It follows that improving and sustainably stocking between 24% and 47% of the farm would give more profit and less absolute enteric CH4 output than does grazing 100% unimproved pasture. Recent experimental work evaluating effects of sustainable intensification of sheep production through pasture improvement confirmed these modelling data for animal productivity and also found a lower absolute emissions per head per day from sheep on improved pasture (McPhee et al. 2024).
Beyond pasture improvement, a suite of other readily applied management options to lower emissions and increase milk yield to provide similar flexibility have been identified for the Kenyan dairy sector (FAO and NZAGRC 2017). All can be considered ‘no-regrets’ strategies. These include, supplementing with legumes or concentrates, silages, local forages or molasses/urea blocks. Increasing feed digestibility and digestible feed intake is widely recommended to reduce CH4 intensity (Hristov et al. 2013b), but where concentrate is fed at low levels, such as by smallholders in SE Asia, total emissions per ruminant are unlikely to decline because of the modest net change in diet quality from starchy supplements (e.g. Duynisveld and Charmley 2018). Nutritional impacts can be further enhanced by also pursuing enhanced animal health in low-emission management pathways (FAO 2023a).
In contrast to supplementary concentrates, SE Asian practices such as feeding of Leucaena in the dry season to boost reproductive and growth performance, together with increased by-product use (Mayberry et al. 2016; Poppi et al. 2017), are consistent with substantially reducing the EI of the animal products and the total daily emissions of individual cattle, because of the direct mitigation action of these browse legumes. Browse and some forage legumes contain condensed tannins or polyphenol oxidase enzymes. These compounds have been shown to reduce CH4 emissions (Hegarty et al. 2021). Concentrated tannins can constitute up to 20% of the dry matter of tannin-rich legumes such as Leucaena leucocephala or Gliricidia spp. (Widiawati et al. 2022) and incorporating these legumes into grazing or cut-and-carry ruminant production systems in SE Asia can reduce CH4 yields by approximately 20%. These legumes also offer mitigation and production benefits in grazing systems (Harrison et al. 2015).
Grazing management practices may have an impact on CH4 emissions from livestock, but results are not consistent. Rotational grazing is often considered as an improvement in pasture management relative to set-stocking pastures, claiming a more even distribution of grazing and manure piles, enhanced grazing capacity, efficiency, and forage uniformity, while preserving soil nutrient properties, and boosting productivity per unit area. In the study of DeRamus et al. (2003), rotational stocking of Paspalum notatum (Bahiagrass) and of Cynodon dactylon (Bermudagrass) caused an average increase in CH4 production of 17% and 14% respectively, and a lower CH4 emission intensity of 21% and 55% respectively for heifers. Zubieta et al. (2021) also found that cows rotationally grazed on Bermudagrass exhibited a 44% reduction in CH4 intensity, but no reduction in CH4 intensity occurred for cows grazed continuously on Bahiagrass pasture. This could be attributed to the fact that the stocking method did not affect the quantity and nutritional value of the herbage. Savian et al. (2014) also found that continuous grazing of Lolium multiflorum (Italian ryegrass), when managed at low and moderate herbage harvest intensities, increased individual animal liveweight gain and reduced CH4 intensity with regards to rotational stocking. Therefore, managing grazing to moderate the nutrient intake may improve animal performance and decrease CH4 intensity.
Confining ruminants for some or all of the day provides not only an opportunity to control the quality of feed consumed, but also the management of manure arising. An example of improving animal productivity is apparent in Cambodia. In Cambodia, a program to reduce burning of rice straw (RS) has been implemented across 25 provinces.
Currently, approximately 10 Tg of RS are being generated annually in Cambodia and approximately 3 Tg of this is burned (Dr Pramol Meak, GDAHP, pers. comm.). A desktop study was conducted to determine the possible impact of redirecting 1 Tg of RS from burning to the feeding of growing cattle in Cambodia (Table 3). National survey data (cattle numbers, liveweights and growth rates), recently collected as the basis for a Tier 2 GHG inventory for livestock, were used, together with published data on RS energy content and CH4 yield of burning RS. Key outcomes from the calculations were as follows: (1) Assuming that all maintenance and activity needs are already met for these cattle, because they are already growing, 1 Tg of RS would provide additional net energy for growth (NEg) equal to approximately 35% of the NEg already available to these cattle. Thus, for energy-limited growth, an increase in animal performance of 35% could be expected. (2) The CH4 release from enteric fermentation of 1 Tg of RS (19.5 t CH4) is higher than the CH4 avoided by not burning the RS (4.51 gG CH4). The net increase in CH4 emissions through the cattle ingesting an additional 1 Tg of RS is 17% of current enteric emission from these cattle. The methane emission intensity (tonnes CH4 per tonne liveweight gain) is 14% less than that in the current production system. So, although RS provides a more modest impact than does improving nutrition by pasture improvement, the principle of improving the nutritional status of ruminants to cause a proportional increase in productivity that exceeds the proportional increase in enteric emissions, is apparent. Like pasture improvement, this offers flexibility to favor increased animal production or reduce absolute emissions according to the balance of food and environmental priorities.
Parameter | Growing ♀ | Growing ♂ | Calf | Total | |
---|---|---|---|---|---|
Baseline | |||||
Animal attributes | |||||
Population | 538,449 | 293,726 | 788,052 | 1,620,227 | |
Average Liveweight | 245.3 | 279.5 | 112.4 | 637 | |
LWG (kg/head.day) | 0.556 | 0.257 | 0.532 | 1.344 | |
Total LWG (t/animal class.year) | 109,231 | 27,537 | 152,942 | 289,710 | |
Baseline CH4 emissions | |||||
Tier 1 enteric CH4/year (t) | 30,153 | 16,449 | 44,131 | 90,733 | |
Baseline CH4 intensity (t CH4/t LWG) | 0.28 | 0.60 | 0.29 | 0.31 | |
Baseline energetics | |||||
NEm (MJ/head.day) | 30.009 | 22.827 | 10.630 | 63 | |
NEa (MJ/head.day) | 0.30 | 0.23 | 0.11 | 1 | |
NEg (MJ/head.day) | 5.14 | 5.30 | 2.7 | 13.1 | |
National NEg (MJ/animal class.year) | 2.24E + 09 | 4.11E + 08 | 1.47E + 09 | 4.12E + 09 | |
When 1 Tg of additonal rice straw was consumed | |||||
NEg from 1 Tg additional Rice straw consumed (MJ) | 1.46E + 09 | ||||
% rise in NEg from 1 Tg Rice straw over current herd NEg | 35.48 | ||||
Potential increase in national growing cattle LWG from 1 TgRS (%) | 35.48 | ||||
CH4 spared by not burning 1 Tg of RS (t CH4/Mt RS) | 4510 | ||||
Additional enteric CH4 from consuming 1 Tg of RS (t) | 19.5 | ||||
Net CH4 output (enteric – spared from not burning; t CH4/year) | 105,720 | ||||
CH4 output as % of baseline scenario | 117% | ||||
CH4 intensity with 1 Tg of RS feeding (t CH4/t LWG) | 0.27 | ||||
CH4 intensity as % of baseline scenario (%) | 86.3 |
It is presumed all RS was fed to the three classes of growing cattle (growing male, growing female and calf).
Animal attributes, animal number, average liveweight (LW) and LWG of cattle are from 2024 national survey data collected for the Cambodian Tier 2 livestock GHG inventory development.
National NEg was calculated from LW and LWG (2024 Survey data) using NEm and NEa equations and IPCC default coefficients.
For CH4 spared by not burning 1 Tg of RS, the CH4 emission coefficient (4.51 g CH4/kg DM) of burning RS developed by Romasanta et al. (2017) was used.
Reduced manure emissions
Just as improving cattle productivity provides advantage by increasing food supply as well as by reducing emission intensity, so can improved manure management provide reduced emissions as well as a financial advantage for the ruminant or monogastric livestock owner. Increasingly, SE Asian ruminants are being managed in cut-and-carry feeding systems that enable manure to be collected and managed. Options such as composting are increasingly being used to value-add the manure for resale as fertiliser (McRoberts et al. 2018), whereas biogas production from manures is widely promoted in Cambodia and through SE Asia (e.g. Khalil et al. 2019). Considering that in some countries, manures contribute approximately one-quarter of on-farm CO2e emissions, these opportunities offer a real and economically desirable approach to lowering the EI of livestock products.
Mitigation through anti-methanogenic supplements
Evaluations and meta-analyses of nutritional supplements that may mitigate ruminant CH4 are being produced prolifically (e.g. Hristov et al. 2013a, 2022; Hegarty et al. 2021; Beauchemin et al. 2022; FAO 2023b); so, trying to review these options is out of scope here. However, it is noteworthy that a small survey of feed industry suppliers largely within SE Asia indicated that many had very poor understanding of the CH4-suppressing efficacy of feed additives (Passetti and Hegarty 2022). Specific CH4 inhibitors such as Bovaer 10 (not regionally available) and nitrate (no economic motivation) are not yet used in SE Asia, but could readily be adopted into cut-and-carry systems that predominate in the region. SE Asian scientists have pioneered a number of the technologies under consideration, such as biochar and shrub legumes (Kongthod et al. 2015; Schmidt et al. 2021). A number of technologies that are not being evaluated elsewhere, such as rice fermentation by-products (Yanti and Yayota 2017) and local seaweeds, are now being evaluated in the region.
Managing emission intensity in contrast to absolute livestock emissions in SE Asia
Each country would like to see a reduction in absolute livestock GHG emissions, because signatories to the NetZero by 2050 target have an obligation to reduce national net GHG emission by that date. Globally, approximately a third of responding countries have included livestock as a mitigation option in their nationally determined contribution (NDC) submissions (Rose et al. 2021; UNFCCC 2023), but often the emphasis is around manure management and the means to net zero are not yet specified. Even globally, there is no expectation that livestock emissions will reach absolute zero. Indeed, the IPCC expectation is that livestock CH4 emissions will continue to rise to 2050, even with efficiency changes. Although optimistically it has been shown that 100% adoption of practical technologies could bring about substantial reduction of absolute emissions (Arndt et al. 2022; FAO 2023a), such adoption is unrealistic and a more realistic anticipation of managing the intensity of, as well as the absolute emission from livestock, is needed.
Article 2 in the Paris Agreement says the agreement ‘aims to strengthen the global response to the threat of climate change, in the context of sustainable development and efforts to eradicate poverty’ and Section 2.1.b says this will be achieved by ‘Increasing the ability to adapt to the adverse impacts of climate change and foster climate resilience and low greenhouse gas emissions development, in a manner that does not threaten food production’. So, there are three key caveats embodied on the responsibility of a country to reduce its emission from food-producing livestock, being recognition of (1) the context of sustainable development, (2) eradication of poverty and (3) that it does not threaten food production.
Although individual GHG species targets were not specifically identified in the initial 1992 Rio Convention (DESA 1992; Mintzer and Leonard 1994), the recognition was made that not all countries can respond equally. In providing a basis for future global environmental management, the Rio Convention stated, in Principle 15, that ‘In order to protect the environment, the precautionary approach shall be widely applied by States according to their capabilities’. Hence, the administrative and legislative awareness of the need to manage GHG emissions in the context of food security is clear. Consequently, for SE Asia, where undernutrition remains a limit in some countries and where a growth in regional population is anticipated, looking to the livestock industries to manage both their food and GHG demands is most sensibly achieved by promoting reduced EI that would be consistent with the caveats in the Paris Agreement. The EI advantages of increasing animal productivity have been shown in Australia for sheep (Michalk et al. 2019), and for dairies in the EU and USA (Gerber et al. 2011; Capper and Cady 2020; Džermeikaitė et al. 2024). Targeting a reduction in EI may be the best option for national prosperity, equity and sustainability.
It is important that the voluntary carbon markets appear to be moving towards integration of EI into the suite of projects that can be given carbon credits of financial value. This is already apparent in Australia, where a nationally approved grazing beef methodology rewards the adoption of management practice that will reduce the EI, but not necessarily the absolute emission of CH4 (Hunt 2015). The major international schemes of Verra https://verra.org/methodologies/methodology-for-reducing-emissions-intensity-of-grassland-based-cattle-production/) and Gold Standard (https://www.goldstandard.org/our-work/innovations-consultations/reducing-methane-emissions-enteric-fermentation-beef-cattle) both have livestock methods under evaluation that in part, if not in full, can accommodate claims based on EI. The fact that the carbon industry is embracing emission intensity as a component for absolute global emission management creates possibilities for agriculture in the SE Asian region to participate in the voluntary carbon market as another motivator for change in industry practice to improve long-term sustainability.
Conclusions
With economic advancement, SE Asia is participating in the diet transition towards an increased animal-protein content in its people’s diet. This increased demand is largely met by importation of meat or animals, but increased local production is widely being pursued as a component of securing future national food security. Much of the additional food protein produced in the region in the past decade has, and will continue to, come from poultry, which have a low emission intensity and GHG footprint. The approximately seven-fold higher EI of beef (the biggest source of livestock emissions) from low-income countries relative to high-income countries identifies that lower EI for the region is possible, even when using ruminants. Further, this can be achieved by currently available nutritional and health management procedures available for cattle. An improvement in EI provides flexibility to manage absolute emissions from ruminants and/or increase ruminant production, subject to enterprise and national priorities. Managing the productivity of individual ruminants to reduce EI provides the opportunity to control the balance of protein production, animal GHG emissions and land-use allocation at both farm and national levels to meet policy goals. The increasing recognition of EI as an emission metric to describe and balance livestock GHG and animal protein supply offers important opportunities as the global voluntary carbon markets mature to recognise EI, not just absolute emissions.
Data availability
The data that support this study will be shared upon reasonable request to the corresponding author.
Declaration of funding
This paper was developed as an activity under the New Zealand government funded Climate Smart Agriculture Initiative to support the objectives of the Global Research Alliance on Agricultural Greenhouse Gases.
Acknowledgements
The financial contribution of the New Zealand Government and organisational and scientific input of the New Zealand Centre for Agricultural Greenhouse Gas Research and all participating organisations in Malaysia and Indonesia is acknowledged. The encouragement of the Organizing Committee of the International Conference on Sustainable Animal Agriculture for Developing Countries (SAADC 2023) is acknowledged. Figs 1, 2, 4, and 6 are adaptations of original works by the OECD/FAO. The opinions expressed and arguments employed in this adaptation are the sole responsibility of the author(s) of the adaptation and should not be reported as representing the official views of the OECD or of its member countries. Dr Pramol Meak, GDAHP, Cambodia is acknowledged for information on rice straw utilisation in Cambodia.
References
Alcock D, Hegarty RS (2006) Effects of pasture improvement on productivity, gross margin and methane emissions of a grazing sheep enterprise. International Congress Series 1293, 103-106.
| Crossref | Google Scholar |
Alcock DJ, Hegarty RS (2011) Potential effects of animal management and genetic improvement on enteric methane emissions, emissions intensity and productivity of sheep enterprises at Cowra, Australia. Animal Feed Science and Technology 166-167, 749-760.
| Crossref | Google Scholar |
Álvarez ER, Castiblanco JS, Montoya MM (2024) Sustainable intensification of palm oil production through cattle integration: a review. Agroecology and Sustainable Food Systems 48, 313-331.
| Crossref | Google Scholar |
Arndt C, Hristov AN, Price WJ, McClelland SC, Pelaez AM, Cueva SF, Oh J, Bannink A, Bayat AR, Crompton LA, Dijkstra J, Eugène MA, Kebreab E, Kreuzer M, McGee M, Martin C, Newbold CJ, Reynolds CK, Schwarm A, Shingfield KJ, Veneman JB, Yáñez-Ruiz DR, Yu Z-T (2021) Strategies to mitigate enteric methane emissions by ruminants: a way to approach the 2.0° C target. CABI AgriRxiv. 10.31220/agriRxiv.2021.00040
Arndt C, Hristov AN, Price WJ, McClelland SC, Pelaez AM, Cueva SF, Oh J, Dijkstra J, Bannink A, Bayat AR, Crompton LA, Crompton LA, Eugène MA, Enahoro D, Kebreab E, Kreuzer M, McGee M, Martin C, Newbold CJ, Reynolds CK, Schwarm A, Shingfield KJ, Veneman JB, Yáñez-Ruiz DR, Yu Z (2022) Full adoption of the most effective strategies to mitigate methane emissions by ruminants can help meet the 1.5°C target by 2030 but not 2050. Proceedings of the National Academy of Sciences 119(20), e2111294119.
| Crossref | Google Scholar |
ASEANstats (2023) ASEANStats data portal, Indicators, IMTS, Trade in Goods, 2023, Commodity 02. Available at http://data.aseanstats.org [Accessed 12 December 2024]
Bateki CA, Wassie SE, Wilkes A (2023) The contribution of livestock to climate change mitigation: a perspective from a low-income country. Carbon Management 14, 1-16.
| Crossref | Google Scholar |
Beauchemin KA, Ungerfeld EM, Abdalla AL, Alvarez C, Arndt C, Becquet P, Benchaar C, Berndt A, Mauricio RM, McAllister TA, Oyhantçabal W, Salami SA, Shalloo L, Sun Y, Tricarico J, Uwizeye A, De Camillis C, Bernoux M, Robinson T, Kebreab E (2022) Invited review: current enteric methane mitigation options. Journal of Dairy Science 105, 9297-9326.
| Crossref | Google Scholar | PubMed |
Besar NA, Suardi H, Phua M-H, James D, Mokhtar MB, Ahmed MF (2020) Carbon stock and sequestration potential of an agroforestry system in Sabah, Malaysia. Forests 11, 210.
| Crossref | Google Scholar |
Bush RD (2024) Advances in smallholder large ruminant production and profitability in Southeast Asia over the past decade – lessons from the Mekong region: a review. Animal Production Science 64, AN23406.
| Crossref | Google Scholar |
Capper JL, Cady RA (2020) The effects of improved performance in the U.S. dairy cattle industry on environmental impacts between 2007 and 2017. Journal of Animal Science 98, skz291.
| Crossref | Google Scholar |
Chang J, Peng S, Yin Y, Ciais P, Havlik P, Herrero M (2021) The key role of production efficiency changes in livestock methane emission mitigation. AGU Advances 2, e2021AV000391.
| Crossref | Google Scholar |
da Silva GDP, Hesselberg T (2020) A review of the use of black soldier fly larvae, Hermetia illucens (Diptera: Stratiomyidae), to compost organic waste in tropical regions. Neotropical Entomology 49, 151-162.
| Crossref | Google Scholar | PubMed |
DeRamus HA, Clement TC, Giampola DD, Dickison PC (2003) Methane emissions of beef cattle on forages: efficiency of grazing management systems. Journal of Environmental Quality 32, 269-277.
| Crossref | Google Scholar | PubMed |
DESA (1992) Report of the United Nations conference on environment and development, (Rio de Janeiro, 3–14 June 1992) Annex 1, Rio declaration on environment and development. Available at https://www.un.org/en/development/desa/population/migration/generalassembly/docs/globalcompact/A_CONF.151_26_Vol.I_Declaration.pdf [accessed 29 May 2024]
Drewnowski AP, Poulain J-P (2018) What lies behind the transition from plant-based to animal protein? AMA Journal of Ethics 20, E987-993.
| Crossref | Google Scholar | PubMed |
Duynisveld JL, Charmley E (2018) Potato processing waste in beef finishing diets; effects on performance, carcass and meat quality. Animal Production Science 58, 546-552.
| Crossref | Google Scholar |
Džermeikaitė K, Krištolaitytė J, Antanaitis R (2024) Relationship between dairy cow health and intensity of greenhouse gas emissions. Animals 14, 829.
| Crossref | Google Scholar |
ESCAP (2023) ESCAP-World Bank trade cost databank. Available at https://databank.worldbank.org/source/escap-world-bank-international-trade-costs. [accessed 21 May 2024]
FAO (2017) Global livestock Environmental Assessment Model: Model Description Version 2.0. Available at https://www.fao.org/fileadmin/user_upload/gleam/docs/GLEAM_2.0_Model_description.pdf [accessed 19 November 2024]
FAO (2018) World livestock: transforming the livestock sector through the sustainable development goals. Food and Agriculture Organization of the United Nations, Rome, Italy. Available at http://www.fao.org/3/CA1201EN/ca1201en.pdf [accessed 21 November 2024]
FAO (2023a) Pathways towards lower emissions: a global assessment of the greenhouse gas emissions and mitigation options from livestock agrifood systems. Food and Agriculture Organization of the United Nations, Rome, Italy. Available at https://doi.org/10.4060/cc9029en [accessed 21 May 2024]
FAO (2023b) Methane emission in livestock and rice systems. Available at https://www.fao.org/documents/card/en?details=cc7607en [accessed 21 September 2023]
FAOSTAT (2023a) Food and agriculture data. Available at http://www.fao.org/faostat/en/#home [accessed 12 September 2023]
FAOSTAT (2023b) FAO domain Emission intensities. Methodological note, release October 2023. Available at https://files-faostat.fao.org/production/EI/EI_e.pdf [accessed 12 December 2024]
FAOSTAT (2024) Suite of food security indicators. Available at https://www.fao.org/faostat/en/#data/FS [accessed 21 November 2024]
FAO and NZAGRC (2017) Options for low emission development in the kenya dairy sector: reducing enteric methane for food security and livelihoods. Food and Agriculture Organization of the United Nations, Rome, Italy. Available at https://openknowledge.fao.org/handle/20.500.14283/i7669en [accessed 21 May 2024]
Fathoni A, Boonkum W, Chankitisakul V, Duangjinda M (2022) An appropriate genetic approach for improving reproductive traits in crossbred Thai–Holstein cattle under heat stress conditions. Veterinary Sciences 9(4), 163.
| Crossref | Google Scholar |
Gebbels JN, Kragt ME, Thomas DT, Vercoe PE (2022) Improving productivity reduces methane intensity but increases the net emissions of sheepmeat and wool enterprises. Animal 16, 100490.
| Crossref | Google Scholar |
Gerber P, Vellinga T, Opio C, Steinfeld H (2011) Productivity gains and greenhouse gas emissions intensity in dairy systems. Livestock Science 139, 100-108.
| Crossref | Google Scholar |
GFSI-UN (2023) Economic impact: global food security index report. Corteva Agriscience. Available at https://impact.economist.com/sustainability/project/food-security-index/reports/Economist_Impact_GFSI_2022_Global_Report_Sep_2022.pdf [accessed 21 November 2024]
Hamilton-Hart N (2019) Indonesia’s quest for food self-sufficiency: a new agricultural political economy? Journal of Contemporary Asia 49, 734-758.
| Crossref | Google Scholar |
Harrison MT, McSweeney C, Tomkins NW, Eckard RJ (2015) Improving greenhouse gas emissions intensities of subtropical and tropical beef farming systems using Leucaena leucocephala. Agricultural Systems 136, 138-146.
| Crossref | Google Scholar |
Hegarty RS, Cortez Passetti RA, Dittmer KM, Wang Y, Shelton S, Emmet-Booth J, Wollenberg E, McAllister T, Leahy S, Beauchemin K, Gurwick N (2021) An evaluation of emerging feed additives to reduce methane emissions from livestock. Edn 1. A report coordinated by Climate Change, Agriculture and Food Security (CCAFS) and the New Zealand Agricultural Greenhouse Gas Research Centre (NZAGRC) initiative of the Global Research Alliance (GRA). Available at https://hdl.handle.net/10568/116489
Henson IE, Ruiz RR, Romero HM (2012) The greenhouse gas balance of the oil palm industry in Colombia: a preliminary analysis. I. Carbon sequestration and carbon offsets. Agronomía Colombiana 30(3), 359-369.
| Google Scholar |
Hilmiati N, Ilham N, Nulik J, Rohaeni ES, deRosari B, Basuki T, Hau DK, Ngongo Y, Lase JA, Fitriawaty F, Surya S, Qomariyah N, Hadiatry MC, Ahmad SN, Qomariah R, Suyatno S, Munir IM, Hayanti SY, Panjaitan T, Yusriani Y (2024) Smallholder cattle development in Indonesia: learning from the past for an outcome-oriented development model. International Journal of Design & Nature and Ecodynamics 19(1), 169-184.
| Crossref | Google Scholar |
Horri M, Suyanto , Jusnita RAE (2023) Disease control management strategy in Bali cattle. Influence International Journal of Science Review 5, 333-342.
| Crossref | Google Scholar |
Hristov AN, Ott T, Tricarico J, Rotz A, Waghorn G, Adesogan A, Dijkstra J, Montes F, Oh J, Kebreab E, Oosting SJ, Gerber PJ, Henderson B, Makkar HPS, Firkins JL (2013a) Special topics – mitigation of methane and nitrous oxide emissions from animal operations: III. A review of animal management mitigation options. Journal of Animal Science 91, 5095-5113.
| Crossref | Google Scholar | PubMed |
Hristov AN, Oh J, Firkins JL, Dijkstra J, Kebreab E, Waghorn G, Makkar HPS, Adesogan AT, Yang W, Lee C, Gerber PJ, Henderson B, Tricarico JM (2013b) Special topics – mitigation of methane and nitrous oxide emissions from animal operations: I. A review of enteric methane mitigation options. Journal of Animal Science 91, 5045-5069.
| Crossref | Google Scholar | PubMed |
Hristov AN, Melgar A, Wasson D, Arndt C (2022) Symposium review: effective nutritional strategies to mitigate enteric methane in dairy cattle. Journal of Dairy Science 105, 8543-8557.
| Crossref | Google Scholar | PubMed |
Hunt G (2015) Carbon credits (Carbon Farming Initiative – Beef Cattle Herd Management) Methodology Determination 2015. Available at https://www.legislation.gov.au/Details/F2015L01434/Html/Text#_Toc426038013 [accessed 23 March 2023]
IPCC (2018) Global Warming of 1.5°C. An IPCC Special Report on the impacts of global warming of 1.5°C above pre-industrial levels and related global greenhouse gas emission pathways, in the context of strengthening the global response to the threat of climate change, sustainable development, and efforts to eradicate poverty (Eds V Masson-Delmotte, P Zhai, HO Pörtner, D Roberts, J Skea, PR Shukla, A Pirani, W Moufouma-Okia, C Péan, R Pidcock, S Connors, JBR Matthews, Y Chen, X Zhou, MI Gomis, E Lonnoy, T Maycock, M Tignor, T Waterfield). Intergovernmental Panel on Climate Change.
Khalil M, Berawi MA, Heryanto R, Rizalie A (2019) Waste to energy technology: the potential of sustainable biogas production from animal waste in Indonesia. Renewable and Sustainable Energy Reviews 105, 323-331.
| Crossref | Google Scholar |
Kongthod T, Thanachit S, Anusontpornperm S, Wiriyakitnateekul W (2015) Effects of biochars and other organic soil amendments on plant nutrient availability in an ustoxic quartzipsamment. Pedosphere 25, 790-798.
| Crossref | Google Scholar |
Liu J, Wang M, Yang L, Rahman S, Sriboonchitta S (2020) Agricultural productivity growth and its determinants in south and Southeast Asian countries. Sustainability 12, 4981.
| Crossref | Google Scholar |
LQBI (2019) Enhancing rural livelihoods in Laos by farmer training. Available at https://mekonglivestock.wordpress.com/2019/01/28/enhancing-rural-livelihoods-in-laos-by-farmer-training-cross-visits/ [accessed 21 May 2024]
MAFI (2021) Malaysia National Agrofood Policy 2021-2030 (NAP 2.0). Available at https://faolex.fao.org/docs/pdf/mal211654.pdf
Mayberry D, Cowley F, Cramb R, Poppi D, Quigley S, McCosker K, Priyanti A (2016) Project improving the reproductive performance of cows and performance of fattening cattle in low input systems of Indonesia and northern Australia. Available at https://espace.library.uq.edu.au/view/UQ:bce11d4 [accessed 21 November 2024]
Mbow C, Rosenzweig CE, Barioni LG, Benton TG, Herrero M, Krishnapillai M, Ruane AC, Liwenga E, Pradhan P, Rivera-Ferre MG, Sapkota T, et al. (2019) Food Security (No. GSFC-E-DAA-TN78913). Available at https://www.ipcc.ch/site/assets/uploads/sites/4/2019/11/08_Chapter-5.pdf [accessed 21 May 2024]
McPhee MJ, Edwards C, Harden S, Naylor T, Phillips FA, Guppy C, Hegarty RS (2024) GrassGroTM simulation of pasture, animal performance and greenhouse emissions on low and high sheep productivity grazing systems: 1-year validation and 25-year analysis. Animal 18, 101088.
| Crossref | Google Scholar |
McRoberts KC, Nicholson CF, Parsons D, Nam LV, Ba NX, Ketterings QM, Cherney DJR (2018) Structure and impact of cattle manure trade in crop–livestock systems of Vietnam. Renewable Agriculture and Food Systems 33, 86-101.
| Crossref | Google Scholar |
Michalk DL, Kemp DR, Badgery WB, Wu J, Zhang Y, Thomassin PJ (2019) Sustainability and future food security – a global perspective for livestock production. Land Degradation & Development 30, 561-573.
| Crossref | Google Scholar |
Mota-Rojas D, Braghieri A, Álvarez-Macías A, Serrapica F, Ramírez-Bribiesca E, Cruz-Monterrosa R, Masucci F, Mora-Medina P, Napolitano F (2021) The use of draught animals in rural labour. Animals 11, 2683-2700.
| Crossref | Google Scholar | PubMed |
Murray CJL, Aravkin AY, Zheng P, Abbafati C, Abbas KM, Abbasi-Kangevari M, Abd-Allah F, Abdelalim A, Abdollahi M, Abdollahpour I, Abegaz KH, et al. (2020) Global burden of 87 risk factors in 204 countries and territories, 1990–2019: a systematic analysis for the Global Burden of Disease Study 2019. The Lancet 396, 1223-1249.
| Crossref | Google Scholar |
Nasution SS, Girsang BM, Hariati H (2021) Evaluation of the effect of sociocultural factors on the children stature in langkat regency, Indonesia. Open Access Macedonian Journal of Medical Sciences 9, 461-466.
| Crossref | Google Scholar |
OECD/FAO (2022) OECD–FAO Agricultural Outlook 2023–2032. OECD Publishing, Paris, France. Available at https://www.oecd-ilibrary.org/agriculture-and-food/oecd-fao-agricultural-outlook-2022-2031_f1b0b29c-en [accessed 21 November 2024]
OECD/FAO (2023) OECD–FAO Agricultural Outlook 2023–2032. OECD Publishing, Paris, France. Available at https://www.oecd-ilibrary.org/agriculture-and-food/oecd-fao-agricultural-outlook-2023-2032_08801ab7-en [accessed 21 May 2025]
Pethick DW, Bryden WL, Mann NJ, Masters DG, Lean IJ (2023) The societal role of meat: the Dublin Declaration with an Australian perspective. Animal Production Science 63, 1805-1826.
| Crossref | Google Scholar |
Pham-Thanh L, Magnusson U, Can-Xuan M, Nguyen-Viet H, Lundkvist Å, Lindahl J (2020) Livestock development in Hanoi City, Vietnam: challenges and policies. Frontiers in Veterinary Science 7, 566.
| Crossref | Google Scholar |
Poppi DP, Quigley SP, Mayberry DE, Cowley F, Harper KJ, McLennan SR (2017) Bridging the gap: strategies to increase live weight gain and profitability in beef cattle in Indonesia. Improving Livestock Productivity, Quality and Safety to Respond to the Increasing Demand from Upper and Middle-Class Consumers. Available at https://hdl.handle.net/1959.11/29654 [accessed 21 May 2024]
Romasanta RR, Sander BO, Gaihre YK, Alberto MC, Gummert M, Quilty J, Nguyen VH, Castalon AG, Balingbing C, Sandro J, Correa T, Jr, Wassmann R (2017) How does burning of rice straw affect CH4 and N2O emissions? A comparative experiment of different on-field straw management practices. Agriculture, Ecosystems & Environment 239, 143-153.
| Crossref | Google Scholar |
Rose S, Khatri-Chhetri A, Dittmer K, Stie, M, Wilkes A, Shelton SW, Arndt C, Wollenberg EK (2021) Livestock management ambition in the new and updated nationally determined contributions: 2020–2021: analysis of agricultural sub-sectors in national climate change strategies. Available at https://hdl.handle.net/10568/115885 [accessed 21 May 2024]
Rustinsyah R (2019) The significance of social relations in rural development: a case study of a beef-cattle farmer group in Indonesia. Journal of Co-operative Organization and Management 7, 100088.
| Crossref | Google Scholar |
Sarmin S, Liang JB (1992) Economic comparisons between draught buffalo use and mechanized in-field transportation of fruit bunches in the palm oil industry in Malaysia. In ‘Proceedings of the sixth AAAP animal science congress Vol. III’, Kasetsart University, Bangkok, Thailand. p. 297. (Faculty of Agriculture. Department of Animal Science. The Animal Husbandry Association of Thailand: Bangkok Thailand)
Savian JV, Neto AB, de David DB, Bremm C, Schons RMT, Genro TCM, do Amaral GA, Gere J, McManus CM, Bayer C, de Faccio Carvalho PC (2014) Grazing intensity and stocking methods on animal production and methane emission by grazing sheep: implications for integrated crop–livestock system. Agriculture, Ecosystems & Environment 190, 112-119.
| Crossref | Google Scholar |
Schmidt MI (1992) The relationship between cattle and savings: a cattle-owner perspective. Development Southern Africa 9, 433-444.
| Crossref | Google Scholar |
Schmidt H-P, Kammann C, Hagemann N, Leifeld J, Bucheli TD, Sánchez Monedero MA, Cayuela ML (2021) Biochar in agriculture: a systematic review of 26 global meta-analyses. GCB Bioenergy 13, 1708-1730.
| Crossref | Google Scholar |
Syarifuddin H, Afdal M, Yurleni Y, Hamidah A, Devitriano D, Poy TT (2024) Sustainability analysis and decision-making strategy for swamp buffalo (Bubalus bubalis carabauesis) conservation in Jambi Province, Indonesia. Open Agriculture 9, 20220293.
| Crossref | Google Scholar |
Tee TP, Goh YM, Zainudin MHM, Candyrine SCL, Sommart K, Kongphitee K, Sumamal W, Phaowphaisal I, Namsilee R, Angthong W, Sunato S, Keaokliang O, Maeda K, Thu NV, Trung TT, Dong NTK, Purnomoadi A, Kurihara M, Jayanegara A, Higuchi K, Kobayashi Y, Ohtani F, Abe H, Terada F, Kumagai H, Matsuyama H, Nonaka I, Takusari N, Shiba N, Hosoda K, Suzuki T, Kamiya Y, Nishida T, Hayasaka K, Shibata M, Wang M, Tan ZL, Wang R, Kebreab E, van Lingen HJ, Hristov AN, Liang JB (2022) Enteric methane emission models for diverse beef cattle feeding systems in South-east Asia: a meta-analysis. Animal Feed Science and Technology 294, 115474.
| Crossref | Google Scholar |
Tenrisanna V, Kasim SN (2020) Trends and forecasting of meat production and consumption in Indonesia: livestock development strategies. IOP conference series: Earth and Environmental Science 492, 012156.
| Crossref | Google Scholar |
Tiemann T, Douxchamps S (2023) Opportunities and challenges for integrated smallholder farming systems to improve soil nutrient management in Southeast Asia. World Development Sustainability 3, 100080.
| Crossref | Google Scholar |
Torres CMME, Jacovine LAG, Nolasco de Olivera Neto S, Fraisse CW, Soares CPB, de Castro Neto F, Ferreira LR, Zanuncio JC, Lemes PG (2017) Greenhouse gas emissions and carbon sequestration by agroforestry systems in southeastern Brazil. Scientific Reports 7, 16738.
| Crossref | Google Scholar |
Tubiello FN, Karl K, Flammini A, Gütschow J, Obli-Laryea G, Conchedda G, Pan X, Qi SY, Heiðarsdóttir HH, Wanner N, Quadrelli R, Rocha Souza L, Benoit P, Hayek M, Sandalow D, Mencos Contreras E, Rosenzweig C, Rosero Moncayo J, Conforti P, Torero M (2022) Pre- and post-production processes increasingly dominate greenhouse gas emissions from agri-food systems. Earth System Science Data Discussions 14, 1795-1809.
| Crossref | Google Scholar |
UNDESA (2021) Circular agriculture for sustainable rural development. Policy Brief No. 105. Available at https://www.un.org/development/desa/dpad/wp-content/uploads/sites/45/publication/PB_105.pdf [accessed 01 October 2024]
UNDESA (2022) World population prospects 2022: summary of results. UN DESA/POP/2022/TR/NO. 3. United Nations Department of Economic and Social Affairs, Population Division [UNDESA]. Available at https://population.un.org/wpp/Graphs/Probabilistic/POP/TOT/920
UNFCCC (2023) Nationally determined contributions under the Paris Agreement: synthesis report by the secretariate. Available at https://unfccc.int/documents/632334 [accessed 21 May 2024]
Widarjono A, Rucbha SM (2016) Household food demand in Indonesia: a two-stage budgeting approach. Journal of Indonesian Economy and Business 31, 163-177.
| Crossref | Google Scholar |
Willett W, Rockström J, Loken B, Springmann M, Lang T, Vermeulen S, Garnett T, Tilman D, DeClerck F, Wood A, Jonell M, Clark M, Gordon LJ, Fanzo J, Hawkes C, Zurayk R, Rivera JA, De Vries W, Majele Sibanda L, Afshin A, Chaudhary A, Herrero M, Agustina R, Branca F, Lartey A, Fan S, Crona B, Fox E, Bignet V, Troell M, Lindahl T, Singh S, Cornell SE, Srinath Reddy K, Narain S, Nishtar S, Murray CJL (2019) Food in the Anthropocene: the EAT–Lancet Commission on healthy diets from sustainable food systems. The Lancet 393, 447-492.
| Crossref | Google Scholar |
World Bank (2023) GDP per capita. Available at http://data.worldbank.org/indicator/NY.GDP.PCAP.CD [accessed 10 March2023]
World Economic Forum (2023) What is the future of sustainable protein in Asia-Pacific? Three expert leaders share their views. Available at https://www.weforum.org/agenda/2023/06/protein-transition-asia-pacific/ [accessed 21 May 2023]
Yanti Y, Yayota M (2017) Agricultural by-products as feed for ruminants in tropical area: nutritive value and mitigating methane emission. Reviews in Agricultural Science 5, 65-76.
| Crossref | Google Scholar |
Zhang M, Feng J-C, Sun L, Li P, Huang Y, Zhang S, Yang Z (2022) Individual dietary structure changes promote greenhouse gas emission reduction. Journal of Cleaner Production 366, 132787.
| Crossref | Google Scholar |
Zubieta ÁS, Savian JV, de Souza Filho W, Wallau MO, Gómez AM, Bindelle J, Bonnet OJF, de Faccio Carvalho PC (2021) Does grazing management provide opportunities to mitigate methane emissions by ruminants in pastoral ecosystems? Science of The Total Environment 754, 142029.
| Crossref | Google Scholar |