Effect of zinc supplementation on the quality of cooled, stored equine sperm
Patricio D. Palacios





A
B
C
D
Abstract
Collecting, cooling, and cryopreserving semen is essential for accessing genetically superior stallions. However, preserving stallion sperm presents unique challenges compared with other species.
This study aimed to investigate the effect of zinc (Zn) supplementation on the quality of equine sperm during cold storage.
Various factors contributing to sperm quality were assessed at 24 and 48 h after cooling, and after subjecting the sperm samples to a heat-resistance test. In Experiment 1, four experimental groups were examined, each with different concentrations of Zn sulfate, as follows: 0, 1, 2, and 3 mM. Subsequently, Experiment 2 involved testing a wider range of concentrations (0, 0.1, 0.2, 0.4, 0.8, 1.6, and 3.2 mM), including evaluation of samples after incubation for 240 min at 37°C (heat-resistance test).
The addition of different concentrations of Zn to the extender INRA96 did not yield substantial improvements in sperm-quality parameters for cooling stallion semen after 24 or 48 h. Moreover, no protective benefits were observed when samples underwent a heat-resistance test. Concentrations of Zn surpassing 3 mM had an adverse effect on sperm-quality parameters.
These findings have contributed to the understanding of Zn supplementation as a strategy for improving semen preservation in stallions.
Keywords: equine, fertility, heat-resistance test, horses, sperm quality, stallion, zinc supplementation, ZnSO4 .
Introduction
Assisted reproductive technologies have revolutionised animal genetic improvement and production efficiency, playing an important role in the field of animal breeding. These technologies encompass a range of innovative procedures such as artificial insemination, sperm cryopreservation, embryo transfer, in vitro fertilisation, cloning, stem-cell technologies, and gamete or embryo sexing (Squires 2005; Hansen 2014). Among these techniques, semen collection, cooling, and cryopreservation have been instrumental in accessing genetically superior stallions and preserving their valuable genetics indefinitely, eliminating the need for animal transport and preventing disease transmission (Yates and Whitacre 1988). However, stallion sperm preservation still poses challenges compared with other species, with overall lower sperm quality, higher rates of oxidative stress, and generally increased cell damage (Contreras et al. 2020; Yánez-Ortiz et al. 2022).
In horses, most refrigeration protocols include the replacement of the seminal plasma with commercial semen extenders aiming to improve sperm (Höfner et al. 2020; Len et al. 2020; Andrade et al. 2022). Intriguingly, seminal plasma boasts abundant levels of zinc (Zn), indicating a crucial involvement of this transition metal in enhancing the developmental competence of the sperm cells (Sørensen et al. 1999). Therefore, the incorporation of Zn during semen manipulation holds promising potential. Among other functions, Zn plays a vital role in regulating redox homeostasis (Atrian and Capdevila 2013), which is crucial for sperm survival, particularly for the stallion (Gibb and Aitken 2016; Peña et al. 2022). Previous research on the effects of Zn supplementation in equine semen extenders is limited (Ghallab et al. 2017), but a protective role of Zn in sperm samples has been described in species such as roosters (Khodaei-Motlagh et al. 2022), bulls (Gualtieri et al. 2014), humans (Isaac et al. 2017), ram (Soltani et al. 2022), and dog (Fayez et al. 2023) among others.
This study aims to optimise the concentration of Zn sulfate (ZnSO4) in an equine semen extender to improve sperm-quality parameters during cold storage for up to 48 h. Sperm-quality parameters include sperm motility, sperm morphology, plasma membrane integrity and acrosomal status, assessed 24 and 48 h after cooling and after a heat-resistance test (Schulze et al. 2021).
Materials and methods
Animals and environment
The present research study was performed at the Veterinary Teaching Hospital of the University of Córdoba, Spain. For all animals used in this study, guidelines from the National Research Council’s Guide for the care and use of laboratory animals have been followed. All animal procedures were also conducted in accordance with the Spanish ethical and legal requirements. Three stallions were used for the study, two Purebred Spanish and one Anglo-Arabic, aged 10–13 years old. The horses selected for the experiment were fertile and clinically healthy and three ejaculates were collected for each of the experiments. Throughout the study, all horses were provided with a well-balanced diet to support their nutritional needs. They were fed a daily ration of 1 kg of commercial concentrate for horses (Lot #0020944594, Fas Adulto G, Nanta, Tres Cantos, Spain), which was divided into three equal feedings. Additionally, the horses had unrestricted access to hay, salt lick, and water, ensuring their hydration and fibre intake. To maintain a controlled environment, the stallions were housed in the same corridor, separated from the mares.
Semen collection, processing, and cooling
Semen collection from each stallion was performed twice a week throughout the research period by using a Missouri-model artificial vagina (Minitüb, Tiefenbach, Germany) with a filter to separate the gel fraction, in the presence of a mare in oestrus. Semen samples were obtained from February to May at the University of Cordoba, Spain.
Immediately after collection, sperm concentration was measured using a SpermaCue photometer (Minitube, Tiefenbach, Germany). The total sperm number per ejaculate (×106 sperm) was calculated considering ejaculate gel-free volume. Sperm-quality assessment of fresh semen was conducted as described below in the section Sperm-quality assessment. Then, sperm was diluted 1:1 (v:v) with a skim milk-based extender (INRA96, IMV-Technologies, France), and centrifuged for 10 min at 600g at room temperature. The sperm pellets were resuspended to a final concentration of 50 × 106 sperm/mL with INRA96 supplemented with different concentrations of ZnSO4 (Z0251, Merk, USA) according to experimental groups. Before semen dilution, the pH of the ZnSO4-supplemented media was adjusted to pH 7 for all experimental groups. Afterwards, sperm refrigeration was performed in 15 mL tubes on a styrofoam shipping box for semen cooling (#17229/0002, Minitube, Tiefenbach, Germany) to keep samples at 5°C for up to 48 h.
Experimental design
To evaluate the effect of the addition of Zn to the extender INRA96, different ZnSO4 concentrations, refrigeration time (24 vs 48 h), and heat-resistance test (0 vs 240 min at 37°C), two experiments were conducted. For all experiments, sperm-quality parameters, including sperm morphology, sperm motility, kinetic parameters, membrane, and acrosomal status were recorded immediately after collection and at 24 h or 48 h of cooling (Figs 1, 2).
Visual representation of the experimental design. Freshly collected semen from three stallions was subjected to analysis and subsequent cooling for durations of 24 and 48 h, with varying concentrations of zinc sulfate. Furthermore, in Experiment 2, an assessment of sperm quality was conducted after heat-resistance testing, involving incubation at 37°C for a duration of 4 h.
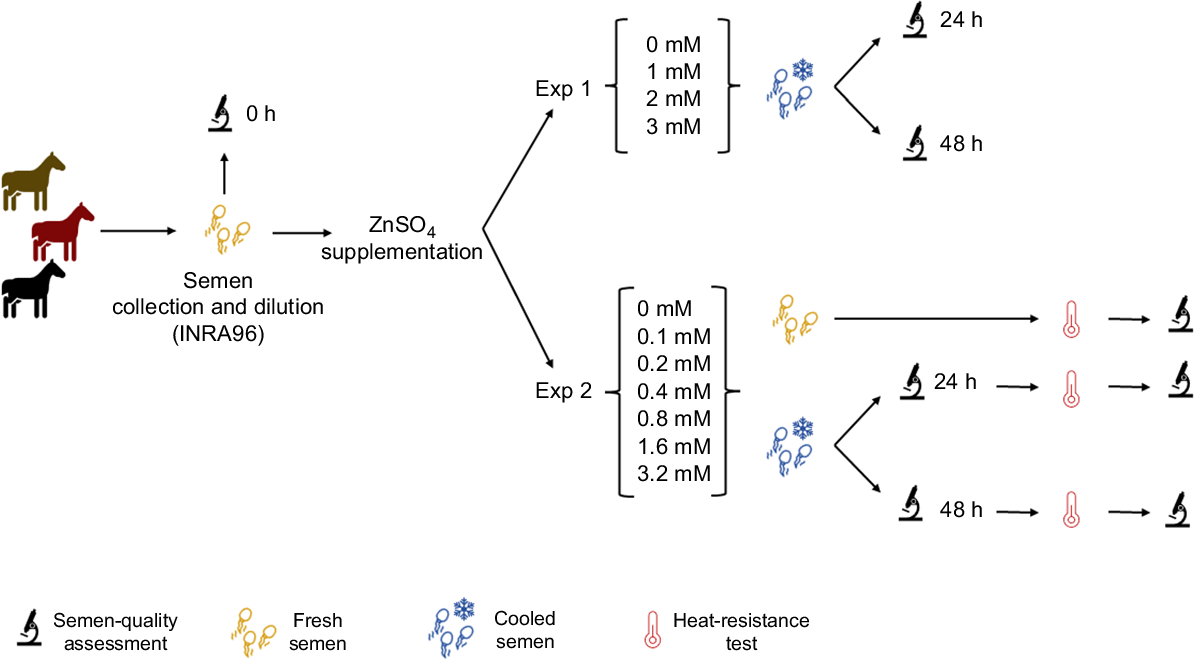
Sperm-quality parameters assessed for fresh and cooled sperm samples. N, normal morphology; AN, abnormal morphology; TM, total motility; PM, progressive motility; VCL, curvilinear velocity; VAP, average path velocity; VSL, straight-line velocity; STR, straightness; LIN, linearity; WOB, wobble, ALH, lateral amplitude of head displacement; BCF, beat cross-frequency; VNR, viable non-reacted; VR, viable reacted; NVNR, non-viable non-reacted; NVR, non-viable reacted.
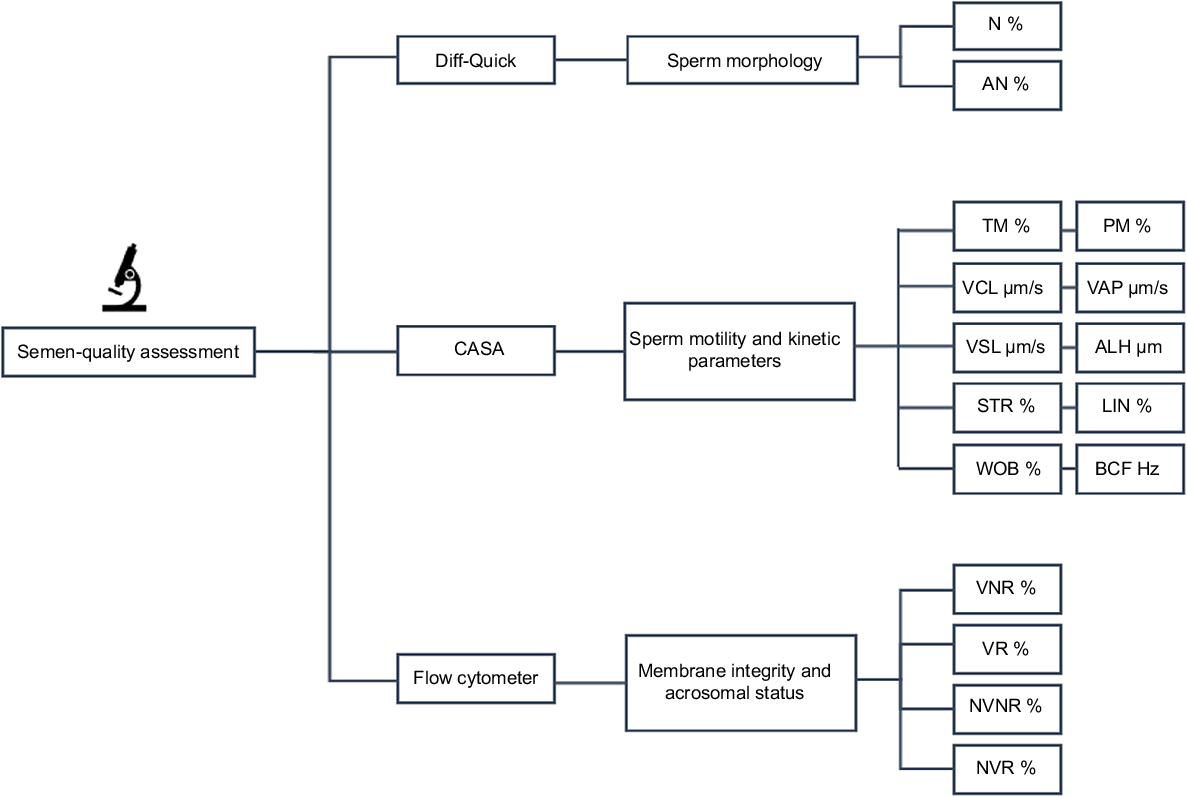
In this experiment, sperm samples supplemented with 0, 1, 2, and 3 mM ZnSO4 were analysed after 0, 24 and 48 h of cold storage, following the methodology described below.
In this experiment, sperm samples supplemented with 0, 0.1, 0.2, 0.4, 0.8, 1.6, and 3.2 mM ZnSO4 were analysed after 24 and 48 h of cold storage. Sperm-quality parameters were evaluated before and after heat-resistance test (240 min incubation at 37°C) (Schulze et al. 2021).
Sperm-quality assessment
Sperm assessment encompassed the analysis of fresh semen samples chilled for 24 and 48 h, as well as samples subjected to a heat-resistance test.
Sperm-morphology evaluation was performed by sperm staining with Diff-Quick® (Baxter DADE AG 3186, Düdingen, Switzerland), according to Veeramachaneni et al. (2006). In total, 200 sperm were carefully counted and observed for each treatment in all experiments, and any morphological abnormalities were documented. Subsequently, the percentage of spermatozoa exhibiting normal morphology (N, %) was calculated. The abnormalities recorded in our study encompassed various sperm morphological anomalies, including those related to the head (such as pyriform, microcephalic, knobbed acrosome, multiple heads, and absence of tail) as well as abnormalities in the mid-piece and tail (such as distal mid-piece reflex, proximal/distal droplets, duplication of the tail, and coiling of the mid-piece or the entire tail).
Sperm-motility parameters were evaluated using a computer-assisted sperm analysis (CASA, Sperm Class Analyser v.6.4, Microptic S.L., Barcelona, Spain) system. The CASA settings were based on the methodology described by Dorado et al. (2014). To assess sperm motility, a 5 μL volume of the sperm sample was placed under the microscope for analysis. A depth of 10 micron Makler counting chamber was used for analysis. Six microscopic fields were captured for each sample, ensuring a minimum observation of 200 sperm per sample. The following parameters were evaluated to characterise sperm movement: total motility (TM, %), progressive motility (PM, %), curvilinear velocity (VCL, μm/s), average path velocity (VAP, μm/s), straight-line velocity (VSL, μm/s), straightness (STR, calculated as VSL/VAP × 100, %), linearity (LIN, calculated as VSL/VCL × 100, %), wobble (WOB, calculated as VAP/VCL × 100, %), the lateral amplitude of head displacement (ALH, μm), and beat cross-frequency (BCF, Hz). Sperm were considered progressively motile if STR was >75%.
Sperm membrane and acrosomal integrity were assessed using fluorescence analysis on a flow cytometer, following the methodology described by Ortiz et al. (2021). Briefly, 40 μL of the selected sperm suspension was diluted in 133 μL of Dulbecco’s phosphate-buffered saline (DPBS, Thermofisher). Then, 10 μL of a 62.5 μg/mL solution of FITC-conjugated Pisum sativum agglutinin (FITC–PSA) in DPBS and 2 μL of a 2.4 mM solution of propidium iodide (PI) in distilled water were added, for final concentrations of 0.05 mg/mL PSA and 1.3 μM PI. The samples were then incubated at room temperature in the dark for 10 min. After this, 40 μL of the stained sample was added to 400 μL of DPBS and this suspension was placed in the feed of the flow cytometer (BD Accuri C6, BD Biosciences, USA). A run with a limit of 10,000 events per sample was used.
Subsequently, the following four distinct populations were identified: (1) viable non-reacted (VNR, %), representing viable spermatozoa that had an intact acrosome; (2) viable reacted (VR, %), denoting viable spermatozoa that had lost the acrosome; (3) non-viable non-reacted (NVNR, %), representing non-viable spermatozoa that had an intact acrosome; and (4) non-viable reacted (NVR, %), indicating non-viable spermatozoa that had lost the acrosome.
Heat-resistance test
This was performed only in Experiment 2 by incubating semen samples for 240 min at 37°C on the basis of the methods described by Schulze et al. 2021. Semen quality after the heat-resistance test was assessed in fresh samples and samples refrigerated for 24 and 48 h in all experimental groups of Experiment 2.
Statistical analyses
The results of the spermatozoa subjected to the different experiments were evaluated using a general linear mixed model (GLMM), considering time and Zn concentration/experimental group as fixed effects and the stallion and ejaculate as random effects. Differences between experiments were analysed using Duncan’s post hoc test. The normality of data distributions was assessed using the Kolmogorov–Smirnov test, while the homogeneity of variances was examined using the Cochran–Bartlett test. Results were expressed as mean ± standard deviation (s.d.). All the data analysis was performed with the statistical package SPSS v15.0 (SPSS®, http://www.spss.com). Significant differences were considered when P-values were <0.05.
Results
All ejaculates used for this study presented normal sperm parameters for the species (Colenbrander et al. 2003) (Supplementary Table S1).
In Experiment 1, we investigated the impact of various ZnSO4 concentrations on equine semen cooled for 24 and 48 h. These concentrations were chosen on the basis of relevant literature references (Ghallab et al. 2017). The supplementation of 1, 2, or 3 mM of ZnSO4 did not significantly alter most of the studied sperm-quality parameters after 24 h of cold storage. Compared with the control group, significant differences were observed only in beat cross-frequency (BCF) value (Table 1), with decreased values with 2 and 3 mM of ZnSO4. After 48 h of cold storage, several kinetic sperm parameters significantly differed among groups, with a significant decline in progressive motility (PM) in the group exposed to 3 mM concentration (Table 2), indicating a detrimental effect of Zn at this concentration.
Parameter | Experimental group | ||||
---|---|---|---|---|---|
Control | 1 mM | 2 mM | 3 mM | ||
TM (%) | 78.3 ± 9.39 | 82.42 ± 11.25 | 71.35 ± 7.81 | 71.2 ± 10.2 | |
PM (%) | 58.45 ± 11.84 | 54.26 ± 8.62 | 39.72 ± 12.43 | 41.6 ± 13.22 | |
VCL (μm/s) | 76.8 ± 10.6 | 70.66 ± 1.75 | 65.53 ± 11.95 | 64.96 ± 12.18 | |
VAP (μm/s) | 58.15 ± 5.97 | 54.57 ± 3.54 | 51.43 ± 8.15 | 51.05 ± 8.97 | |
VSL (μm/s) | 49.1 ± 4.18b | 41.84 ± 3.19ab | 38.84 ± 5.5a | 39.61 ± 6.47ab | |
LIN (%) | 65.4 ± 5.05 | 61.39 ± 2.47 | 61.13 ± 5.32 | 62.26 ± 8.37 | |
STR (%) | 82.67 ± 3.31 | 77.05 ± 1.93 | 76.17 ± 75.54 | 77.11 ± 7.39 | |
WOB (%) | 76.29 ± 3.49 | 76.46 ± 4.9 | 76.96 ± 3.2 | 77.33 ± 4.19 | |
ALH (μm) | 2.52 ± 0.41 | 2.31 ± 0.17 | 2.21 ± 0.44 | 2.18 ± 0.44 | |
BCF (Hz) | 9.02 ± 0.55b | 8.54 ± 0.21ab | 7.99 ± 0.43a | 7.93 ± 0.72a | |
N (%) | 82.67 ± 2.02 | 83.5 ± 3.28 | 80.33 ± 2.08 | 84.33 ± 3.21 | |
VNR (%) | 55.27 ± 9.4 | 62.47 ± 5.25 | 64 ± 5.88 | 64.43 ± 10.08 |
Means within a row followed by different letters are significantly different (at P = 0.05).
TM, total motility; PM, progressive motility; VCL, curvilinear velocity; VAP, average path velocity; VSL, straight-line velocity; LIN, linearity; STR, straightness; WOB, wobble; ALH, lateral amplitude of head displacement; BCF, beat cross-frequency; N, normal morphology; VNR, viable non-reacted.
Parameter | Experimental group | ||||
---|---|---|---|---|---|
Control | 1 mM | 2 mM | 3 mM | ||
TM (%) | 79.58 ± 9.45 | 61.83 ± 24.81 | 58.85 ± 13.11 | 43.86 ± 25.41 | |
PM (%) | 57.84 ± 7.57b | 37.92 ± 22.4ab | 31.62 ± 5.61ab | 22.3 ± 17.37a | |
VCL (μm/s) | 79.51 ± 1.4c | 68.79 ± 6.55b | 63.17 ± 4.93ab | 56.96 ± 6.75a | |
VAP (μm/s) | 61.21 ± 3.21b | 53.49 ± 6.77ab | 50.03 ± 3.69a | 43.38 ± 6.88a | |
VSL (μm/s) | 48.63 ± 2.8b | 38.94 ± 9.25ab | 37.62 ± 0.91ab | 31.6 ± 6.18a | |
LIN (%) | 60.78 ± 0.58 | 58.25 ± 6.95 | 59.67 ± 5.24 | 57.31 ± 4.43 | |
STR (%) | 77.21 ± 0.8 | 73.6 ± 5.58 | 75.54 ± 3.66 | 74.15 ± 3.11 | |
WOB (%) | 75.35 ± 1.25 | 75.84 ± 3.63 | 76.96 ± 3.2 | 73.14 ± 3.35 | |
ALH (μm) | 2.6 ± 0.1c | 2.28 ± 0.09b | 2.11 ± 0.19ab | 1.96 ± 0.19a | |
BCF (Hz) | 8.54 ± 0.67 | 7.97 ± 0.84 | 7.62 ± 0.89 | 7.59 ± 0.49 | |
N (%) | 85.5 ± 4.5 | 85.83 ± 5.25 | 82.33 ± 4.16 | 82.67 ± 2.02 | |
VNR (%) | 62.33 ± 6.66 | 63.87 ± 1.1 | 64.2 ± 3.55 | 68.63 ± 6.31 |
Means within a row followed by different letters are significantly different (at P = 0.05).
TM, total motility; PM, progressive motility; VCL, curvilinear velocity; VAP, average path velocity; VSL, straight-line velocity; LIN, linearity; STR, straightness; WOB, wobble; ALH, lateral amplitude of head displacement; BCF, beat cross-frequency; N, normal morphology; VNR, viable non-reacted.
Because the concentration of Zn tested in Experiment 1 did not show any benefit on motility parameters, we proceeded to explore in Experiment 2 a broader range of ZnSO4 concentrations (0, 0.1, 0.2, 0.4, 0.8, 1.6, and 3.2 mM, Tables 3 and 4). Consistent with our results in Experiment 1, the concentration of 3.2 mM ZnSO4 exhibited a detrimental effect on sperm, resulting in negative impacts on TM (total motility), STR (straightness), ALH (lateral amplitude of head displacement) and BCF (beat cross-frequency) parameters at 48 h of cold storage compared with the control group (Table 4).
Parameter | Experimental group | |||||||
---|---|---|---|---|---|---|---|---|
Control | 0.1 mM | 0.2 mM | 0.4 mM | 0.8 mM | 1.6 mM | 3.2 mM | ||
TM (%) | 78.17 ± 16.38 | 43.37 ± 33.2 | 72.04 ± 31.48 | 90.13 ± 4.54 | 50.32 ± 36.28 | 81.66 ± 8.13 | 42.23 ± 47.3 | |
PM (%) | 38.29 ± 29.87 | 11.44 ± 14.67 | 31.19 ± 29.63 | 54.26 ± 22.74 | 22.26 ± 34.43 | 26.02 ± 28.30 | 17.79 ± 30.04 | |
VCL (μm/s) | 49.49 ± 16.39 | 34.71 ± 9.17 | 44.42 ± 20.31 | 62.81 ± 17.1 | 43.09 ± 21.42 | 41.32 ± 15.72 | 31.48 ± 25.30 | |
VAP (μm/s) | 27.13 ± 12.78 | 17.02 ± 6.96 | 22.17 ± 12.25 | 36.18 ± 13.61 | 24.89 ± 17.82 | 22.04 ± 13.5 | 17.79 ± 16.96 | |
VSL (μm/s) | 23 ± 12.94 | 12.85 ± 6.32 | 17.2 ± 11.62 | 29.88 ± 12.26 | 20.25 ± 17.74 | 17.89 ± 13.97 | 14.64 ± 15.3 | |
LIN (%) | 44.46 ± 11.7 | 36.3 ± 13.23 | 36.03 ± 15.03 | 46.76 ± 9.67 | 41.64 ± 15.63 | 38.72 ± 14.34 | 35.73 ± 25.74 | |
STR (%) | 78.76 ± 8.72 | 68.87 ± 12.9 | 69.67 ± 19.21 | 79.96 ± 5.98 | 73.37 ± 11.73 | 72.88 ± 12 | 56.78 ± 38.44 | |
WOB (%) | 54.11 ± 8.79 | 48.77 ± 10.75 | 49.17 ± 8.57 | 56.65 ± 7.96 | 54.19 ± 11.96 | 51.02 ± 9.76 | 44.09 ± 30.33 | |
ALH (μm) | 2 ± 0.45 | 1.61 ± 0.33 | 1.97 ± 0.67 | 2.39 ± 0.46 | 1.92 ± 0.53 | 1.83 ± 0.32 | 1.41 ± 0.98 | |
BCF (Hz) | 10.29 ± 2.17ab | 7.52 ± 2.46ab | 8.69 ± 3.84ab | 11.1 ± 1.51b | 7.31 ± 4.36ab | 8.79 ± 2.47ab | 4.9 ± 5.57a | |
N (%) | 93.5 ± 3.32a | 92.67 ± 2.31a | 93.33 ± 1.53a | 93.75 ± 3.4a | 90.5 ± 1.29a | 93.5 ± 3.42a | 93.25 ± 1.71a | |
VNR (%) | 77.13 ± 7.84ab | 53.6 ± 23.08a | 82.73 ± 5.42b | 81.33 ± 6.42b | 54.4 ± 23.58a | 79.6 ± 3.61ab | 60.28 ± 22.23ab |
Means within a row followed by different letters are significantly different (at P = 0.05).
TM, total motility; PM, progressive motility; VCL, curvilinear velocity; VAP, average path velocity; VSL, straight-line velocity; LIN, linearity; STR, straightness; WOB, wobble; ALH, lateral amplitude of head displacement; BCF, beat cross-frequency; N, normal morphology; VNR, viable non-reacted.
Parameter | Experimental group | |||||||
---|---|---|---|---|---|---|---|---|
Control | 0.1 mM | 0.2 mM | 0.4 mM | 0.8 mM | 1.6 mM | 3.2 mM | ||
TM (%) | 60.68 ± 16.57bc | 24.93 ± 32.56ab | 63.68 ± 43.25bc | 81.57 ± 14.93c | 19.98 ± 26.63a | 65.91 ± 24.42bc | 6.56 ± 8a | |
PM (%) | 9.40 ± 9.60abc | 5.25 ± 8.01ab | 30.08 ± 27.99c | 26.18 ± 19.22bc | 0.5 ± 0.58a | 15.34 ± 17.21abc | 0.19 ± 0.39a | |
VCL (μm/s) | 33.02 ± 8.93ab | 34.17 ± 13.89ab | 47.09 ± 24.25b | 43.62 ± 15.06b | 15.71 ± 11.05a | 36.94 ± 19.19ab | 10.74 ± 13.24a | |
VAP (μm/s) | 15.83 ± 5.87ab | 17.7 ± 7.95ab | 24.24 ± 15.86b | 22.07 ± 8.53b | 8.01 ± 5.85ab | 19.97 ± 13.49ab | 4.78 ± 5.68a | |
VSL (μm/s) | 11.89 ± 5.52ab | 12.07 ± 6.56ab | 14.25 ± 9.15ab | 17.35 ± 6.59b | 5.65 ± 4.6ab | 14 ± 10.35ab | 3.13 ± 3.65a | |
LIN (%) | 35.55 ± 9.47ab | 37.93 ± 8.8ab | 35.46 ± 15.02ab | 40.14 ± 6.35b | 25.72 ± 20.06ab | 34.45 ± 11.88ab | 15.86 ± 18.47a | |
STR (%) | 69.86 ± 9.08b | 68.92 ± 11.33b | 68.15 ± 15.95b | 75.97 ± 3.38b | 47.54 ± 33.16ab | 65.15 ± 10.3b | 30.32 ± 35.21a | |
WOB (%) | 48.49 ± 6.64 | 51.78 ± 5.49 | 49.01 ± 10.91 | 51.08 ± 6.22 | 38.51 ± 26.78 | 49.83 ± 9.79 | 23.93 ± 27.66 | |
ALH (μm) | 1.58 ± 0.36bc | 1.61 ± 0.47bc | 2.03 ± 0.75c | 1.87 ± 0.56c | 0.82 ± 0.56ab | 1.64 ± 0.6bc | 0.57 ± 0.69a | |
BCF (Hz) | 7.78 ± 1.67bc | 7.06 ± 3.5bc | 8.25 ± 4.13bc | 9.31 ± 1.24c | 4.32 ± 3.19ab | 6.99 ± 2.5bc | 1.95 ± 2.28a | |
N (%) | 90 ± 3.92 | 90.33 ± 5.03 | 88.67 ± 7.09 | 87.75 ± 4.86 | 90.5 ± 4.51 | 93.75 ± 1.71 | 93 ± 3.16 | |
VNR (%) | 58.35 ± 17.6 | 40.53 ± 18.51 | 72.07 ± 7.38 | 72.35 ± 7.23 | 35.55 ± 26.46 | 71.95 ± 8.54 | 48.05 ± 31.21 |
Means within a row followed by different letters are significantly different (at P = 0.05).
TM, total motility; PM, progressive motility; VCL, curvilinear velocity; VAP, average path velocity; VSL, straight-line velocity; LIN, linearity; STR, straightness; WOB, wobble; ALH, lateral amplitude of head displacement; BCF, beat cross-frequency; N, normal morphology; VNR, viable non-reacted.
Because no significant differences were observed in overall sperm quality among experimental groups of ZnSO4 supplementation lower than 3.2 mM following cooling, we hypothesised that the protective role of Zn might be shown under certain conditions where, for example, sperm cells were forced to exhibit higher metabolic activity, generating more reactive oxygen species. To test this hypothesis, we subjected the sperm samples to a 240 min incubation at 37°C (heat-resistance test) and, afterwards, re-evaluated sperm-quality parameters across all experimental groups in fresh and chilled samples.
After the heat-resistance test conducted on fresh semen, we observed that sperm exposed to concentrations of 0.4 and 1.6 mM ZnSO4 exhibited significantly higher survival rates (viable non-reacted, VNR) than did the control group (Table 5). However, when 24 h-chilled samples underwent the heat-resistance test, no obvious protective effects of Zn supplementation were observed. In fact, concentrations of Zn of 3.2 mM had a negative impact on TM, PM and BCF sperm values (Table 6). Likewise, no protective effects of Zn supplementation were observed after the heat-resistance test for semen chilled for up to 48 h (Table 7). Thus, consistent with our observations for cooled semen, high concentrations of Zn resulted in significantly reduced sperm quality.
Parameter | Experimental group | |||||||
---|---|---|---|---|---|---|---|---|
Control | 0.1 mM | 0.2 mM | 0.4 mM | 0.8 mM | 1.6 mM | 3.2 mM | ||
TM (%) | 61.8 ± 40.26ab | 47.93 ± 39.88ab | 57.68 ± 46.44ab | 74.88 ± 36.04b | 22.08 ± 44.16ab | 42.14 ± 37.69ab | 1.25 ± 1.98a | |
PM (%) | 25.04 ± 27.13 | 9.33 ± 8.09 | 19.29 ± 18.71 | 28.61 ± 26.75 | 10.71 ± 21.42 | 10.46 ± 17.45 | 0 ± 0 | |
VCL (μm/s) | 42.65 ± 20.72b | 34.46 ± 5.88ab | 37.48 ± 10.49ab | 45.81 ± 21.68b | 14.12 ± 28.25ab | 24.84 ± 22.82ab | 8.29 ± 9.57a | |
VAP (μm/s) | 24.56 ± 12.36 | 19.11 ± 3.66 | 19.37 ± 6.32 | 26.04 ± 15.14 | 10.48 ± 20.96 | 14.37 ± 16.2 | 4.08 ± 4.75 | |
VSL (μm/s) | 19.38 ± 11.48 | 14.51 ± 3 | 15.17 ± 6.31 | 20.42 ± 13.01 | 9.01 ± 18.03 | 10.61 ± 13.69 | 2.22 ± 2.6 | |
LIN (%) | 41.92 ± 10.54ab | 45.31 ± 1.4b | 39.85 ± 7.21ab | 40.66 ± 11.49ab | 14.5 ± 29ab | 24.97 ± 21.4a | 12.79 ± 15.07a | |
STR (%) | 59.04 ± 38.22abc | 75.34 ± 2.71c | 73.47 ± 10.03bc | 72.62 ± 9.71bc | 20.53 ± 41.05a | 46.65 ± 34.19abc | 24.86 ± 28.74ab | |
WOB (%) | 55.82 ± 4.55b | 59.38 ± 2.9b | 53.71 ± 2.15b | 53.88 ± 8.25b | 17.08 ± 34.16a | 38.38 ± 26.95ab | 24.29 ± 28.26ab | |
ALH (μm) | 1.78 ± 0.58b | 1.71 ± 0.03b | 1.77 ± 0.22b | 1.93 ± 0.59b | 0.52 ± 1.04a | 1.17 ± 0.91ab | 0.55 ± 0.63a | |
BCF (Hz) | 8.09 ± 3.36bc | 6.31 ± 2.86abc | 7.64 ± 3.75bc | 8.64 ± 2.84c | 2.3 ± 4.6ab | 5.02 ± 4.01abc | 1.1 ± 1.35a | |
N (%) | 92.5 ± 1.29b | 90.33 ± 0.58ab | 87 ± 4.36a | 92.75 ± 2.22b | 93 ± 3.74b | 92.5 ± 2.65b | 94.25 ± 3.3b | |
VNR (%) | 56.85 ± 28.8a | 38.83 ± 15.64a | 63.3 ± 27.48ab | 79.93 ± 4.66b | 34.5 ± 33.46a | 80.58 ± 0.26b | 44.33 ± 27.53a |
Means within a row followed by different letters are significantly different (at P = 0.05).
TM, total motility; PM, progressive motility; VCL, curvilinear velocity; VAP, average path velocity; VSL, straight-line velocity; LIN, linearity; STR, straightness; WOB, wobble; ALH, lateral amplitude of head displacement; BCF, beat cross-frequency; N, normal morphology; VNR, viable non-reacted.
Parameter | Experimental group | |||||||
---|---|---|---|---|---|---|---|---|
Control | 0.1 mM | 0.2 mM | 0.4 mM | 0.8 mM | 1.6 mM | 3.2 mM | ||
TM (%) | 41.92 ± 21.67b | 14.85 ± 25.73ab | 40.22 ± 35.67b | 36.48 ± 22.64ab | 7.93 ± 13.74ab | 14.41 ± 16.21ab | 1.67 ± 2.64a | |
PM (%) | 9.74 ± 8.22b | 1.81 ± 3.14ab | 6.69 ± 6.41ab | 4.66 ± 3.77ab | 1.88 ± 3.26ab | 2.17 ± 3.24ab | 0.03 ± 0.06a | |
VCL (μm/s) | 33.27 ± 8.03ab | 10.4 ± 18.01a | 38.49 ± 11.24b | 28.36 ± 8.39ab | 13.14 ± 22.76a | 20.77 ± 17.31ab | 8.95 ± 10.61a | |
VAP (μm/s) | 17.94 ± 5.41 | 5.37 ± 9.31 | 18.8 ± 5.26 | 15.08 ± 7.53 | 8.93 ± 15.47 | 11.38 ± 10.84 | 4.61 ± 5.47 | |
VSL (μm/s) | 14.6 ± 5.61 | 4.13 ± 7.15 | 11.41 ± 1.65 | 11.17 ± 6.81 | 7.36 ± 12.75 | 8.07 ± 8.92 | 2.92 ± 3.4 | |
LIN (%) | 40.43 ± 6.52 | 13.43 ± 23.27 | 32.54 ± 9.1 | 33.36 ± 9.32 | 15.28 ± 26.46 | 24.38 ± 17.44 | 16.9 ± 19.69 | |
STR (%) | 73.39 ± 7.96 | 24.49 ± 42.41 | 65.01 ± 14.35 | 65.39 ± 8.21 | 24.48 ± 42.4 | 44.65 ± 30.68 | 29.6 ± 34.19 | |
WOB (%) | 52.9 ± 4.28 | 17.76 ± 30.76 | 48.83 ± 2.29 | 49.29 ± 7.59 | 19.77 ± 34.24 | 39.39 ± 26.35 | 26.01 ± 30.18 | |
ALH (μm) | 1.55 ± 0.22ab | 0.49 ± 0.85a | 2.17 ± 1.14b | 1.42 ± 0.22ab | 0.54 ± 0.94a | 1.07 ± 0.78ab | 0.5 ± 0.59a | |
BCF (Hz) | 7.77 ± 2.17c | 2.46 ± 4.26ab | 6.83 ± 2.08bc | 6.05 ± 1.37abc | 2.37 ± 4.1ab | 3.69 ± 2.84abc | 1.32 ± 1.87a | |
N (%) | 92 ± 3.16ab | 91.33 ± 2.52a | 95.67 ± 0.58bc | 92.5 ± 2.89ab | 92.75 ± 1.26ab | 92.5 ± 1.73ab | 96.88 ± 1.93c | |
VNR (%) | 33.55 ± 16.31ab | 21.97 ± 13.76ab | 44.37 ± 34.53ab | 53.58 ± 25.53b | 12.88 ± 12.94a | 54.4 ± 13.94b | 14.23 ± 14.55a |
Means within a row followed by different letters are significantly different (at P = 0.05).
TM, total motility; PM, progressive motility; VCL, curvilinear velocity; VAP, average path velocity; VSL, straight-line velocity; LIN, linearity; STR, straightness; WOB, wobble; ALH, lateral amplitude of head displacement; BCF, beat cross-frequency; N, normal morphology; VNR, viable non-reacted.
Parameter | Experimental group | |||||||
---|---|---|---|---|---|---|---|---|
Control | 0.1 mM | 0.2 mM | 0.4 mM | 0.8 mM | 1.6 mM | 3.2 mM | ||
TM (%) | 36.78 ± 26.31b | 6.42 ± 8.76a | 38.66 ± 37.78b | 23.14 ± 11.50ab | 10.9 ± 14.85ab | 4.61 ± 5.35a | 0.71 ± 1.04a | |
PM (%) | 7.24 ± 6.06b | 0.63 ± 1.09a | 4.83 ± 5.58ab | 0.55 ± 0.52a | 1 ± 2.01a | 0.22 ± 0.44a | 0 ± 0a | |
VCL (μm/s) | 27.96 ± 10.2 | 17.77 ± 15.42 | 24.59 ± 10.04 | 19.62 ± 2.86 | 23.72 ± 16.38 | 10.68 ± 12.62 | 14.92 ± 19.68 | |
VAP (μm/s) | 15.45 ± 5.86 | 10.16 ± 9.28 | 12.42 ± 5.6 | 9.16 ± 1.57 | 11.96 ± 8.73 | 5.53 ± 6.46 | 8.02 ± 10.14 | |
VSL (μm/s) | 11.97 ± 5.79b | 5.94 ± 5.18ab | 8.96 ± 4.56ab | 5.51 ± 1.38ab | 6.67 ± 5.7ab | 3.64 ± 4.26a | 3.53 ± 4.79a | |
LIN (%) | 38.71 ± 5.41b | 24.3 ± 21.82ab | 34.41 ± 3.59ab | 25.81 ± 5.1ab | 20.15 ± 15.82ab | 15.75 ± 18.27ab | 12.98 ± 15.58a | |
STR (%) | 68.74 ± 8.19b | 40.52 ± 35.36ab | 66.92 ± 4.14b | 55.06 ± 4.80ab | 39.57 ± 28.18ab | 29.09 ± 33.59ab | 22.7 ± 2654a | |
WOB (%) | 54.16 ± 5.05 | 38.91 ± 36.06 | 50.65 ± 1.28 | 46.17 ± 5.94 | 36.58 ± 25.41 | 25.69 ± 29.72 | 27.02 ± 31.2 | |
ALH (μm) | 1.33 ± 0.33 | 1.11 ± 0.98 | 1.24 ± 0.32 | 1.12 ± 0.88 | 1.37 ± 1.03 | 0.64 ± 0,.74 | 0.86 ± 1.1 | |
BCF (Hz) | 6.46 ± 2.67b | 2.48 ± 2.71ab | 5.78 ± 2.41bc | 4.32 ± 0.85abc | 3.16 ± 2.69abc | 1.71 ± 2.05a | 1.48 ± 1.71a | |
N (%) | 93.75 ± 2.75a | 90.33 ± 4.16a | 90.17 ± 4.19a | 93 ± 5.23a | 92.5 ± 2.38a | 91.75 ± 1.71a | 91.25 ± 3.3a |
Means within a row followed by different letters are significantly different (at P = 0.05).
TM, total motility; PM, progressive motility; VCL, curvilinear velocity; VAP, average path velocity; VSL, straight-line velocity; LIN, linearity; STR, straightness; WOB, wobble; ALH, lateral amplitude of head displacement; BCF, beat cross-frequency; N, normal morphology; VNR, viable non-reacted.
Discussion
This study has thoroughly examined the effects of varying concentrations of Zn on sperm-quality parameters during the cold storage of stallion semen. While certain differences in sperm motility and membrane integrity were observed in specific experimental groups, overall, no discernible benefits of increasing the availability of Zn molecules in the commercial semen extender INRA96 were detected for sperm cooling.
The inclusion of molecules in commercial semen extenders, aimed at enhancing the longevity of cooled sperm, could potentially have a significant impact on the equine breeding industry. This impact might allow for longer transportation times and, as a result, a broader dissemination of male genetics through artificial insemination. Previous reports in other species suggest that supplementing an appropriate concentration of Zn can effectively enhance sperm quality (Fallah et al. 2018; Sutovsky et al. 2019), potentially leading to improved fertility (Allouche-Fitoussi and Breitbart 2020). These effects are mainly attributed to the ability of Zn to stabilise the sperm membrane (Zhao et al. 2016), protect the mitochondrial potential of spermatozoa (Kotdawala et al. 2012) and aid in the detoxification of reactive oxygen species through sperm proteins (Henkel et al. 1999). Furthermore, higher plasma concentrations of Zn have been associated with better sperm quality in stallions (Kandiel and El Khawagah 2018). However, limited research has been conducted on the effect of Zn supplementation on stallion sperm. For instance, one study demonstrated that adding 0.2 mM ZnSO4 to semen extender significantly improved motility, viability, plasma membrane integrity, and sperm acrosomal status of Arabian stallion spermatozoa after cooling at 5°C or after freezing and thawing (Ghallab et al. 2017). In contrast, our study was unable to support these findings, because no significant differences were observed among the experimental groups, possibly owing to the use of a different semen diluent media and refrigeration time. It is worth noting that Zn can bind with proteins and receptors within the diluent, potentially leading to variations in outcomes (Allouche-Fitoussi and Breitbart 2020). Such variations could explain the disparities observed in sperm quality following Zn supplementation across different research studies.
Our study showed that concentrations higher than 3 mM of ZnSO4 had a detrimental effect on sperm quality. The normal concentration of Zn in stallion seminal plasma is approximately 0.0132 mM (Pesch et al. 2006). Therefore, the concentration used in our study was approximately 240 times higher than the normal concentration found in seminal plasma. Similar findings have been reported in water buffalo sperm, where high concentrations of ZnSO4 were found to have a toxic action, resulting in alterations in sperm motility, viability, and membrane integrity (Dorostkar et al. 2014). The negative effects of Zn excess can be attributed to its pro-oxidant properties when imbalanced, leading to mitochondrial oxidative stress (Lee 2018). Our findings further support the notion that a delicate balance in Zn concentrations is needed to maintain sperm function. Interestingly, the only protective role of Zn supplementation in our study was evident when fresh sperm was subjected to a heat-resistance test. The addition of 0.4 mM of ZnSO4 showed a reduction in the rate of sperm membrane damage. This concentration also exhibited higher numerical values for several sperm parameters, although these were not statistically significant. Thus, it is possible that, if any, the optimal range of Zn supplementation is close to 0.4 mM in horses using the semen extender employed in our study.
Zn is also recognised for its role in sperm capacitation (Sutovsky et al. 2019; Zigo et al. 2022). However, our understanding of this process remains limited in horses, because in vitro capacitation of horse sperm has been shown to be challenging, as discussed in previous studies (Leemans et al. 2016; Maitan et al. 2022). In our study, despite the addition of various concentrations of Zn to the extender media, no significant effects were observed on the number of sperm exhibiting acrosome reactions. These findings indicated that simply increasing the number of Zn molecules in the semen extender is not sufficient to induce the necessary changes in the sperm membrane for triggering this event.
In conclusion, our study has provided evidence that supplementing the extender INRA 96 with various concentrations of ZnSO4 does not significantly improve sperm-quality parameters in chilled stallion semen after 24 or 48 h. Furthermore, no protective effects were observed when chilled sperm samples were subjected to the heat-resistance test. Importantly, concentrations of ZnSO4 exceeding 3 mM had a negative impact on sperm-quality parameters, indicating a toxic effect. These findings contribute to the understanding of Zn supplementation as a strategy for improving semen preservation in stallions and highlight the importance of maintaining a delicate balance in Zn concentrations to ensure optimal sperm function. Further research is warranted to explore potential combinations of Zn with other molecules that may have a role in sperm quality.
Data availability
The data that support this study are available in the article and accompanying online supplementary material.
Declaration of funding
This research was partially supported by grants: AGL-2013-42726-R (‘Ministerio de Economía Industria y Competitividad’) and UCO-FEDER-20 REF 1381332-R (Universidad de Córdoba-Junta de Andalucía).
Author contributions
P. D. Palacios: acquisition of data, analysis and interpretation of the results, data curation, visualisation, writing, conclusions, review and editing of the paper. I. Ortiz: acquisition of data, performing the investigation, formal analysis, review and editing of the paper, supervision. J. R. García-Díaz: design of the study, methodology. M. Hidalgo: conceptualisation, design of the study, methodology, review and editing of the paper, project administration and funding acquisition. J. Dorado: conceptualisation, design of the study, methodology, writing, review and editing of the paper, project administration and funding acquisition. A. Gambini: acquisition of data, conceptualisation, design of the study, methodology, writing, review and editing of the paper, supervision.
Acknowledgements
The authors are extremely thankful to the Ministry of Defense, especially to the equine breeding services of the Spanish Army located in Ecija, Spain, for providing animals to develop the experiments.
References
Allouche-Fitoussi D, Breitbart H (2020) The role of zinc in male fertility. International Journal of Molecular Sciences 21(20), 7796.
| Crossref | Google Scholar | PubMed |
Andrade AFC, Knox RV, Torres MA, Pavaneli APP (2022) What is the relevance of seminal plasma from a functional and preservation perspective? Animal Reproduction Science 246, 106946.
| Crossref | Google Scholar |
Atrian S, Capdevila M (2013) Metallothionein–protein interactions. Biomolecular Concepts 4(2), 143-160.
| Crossref | Google Scholar | PubMed |
Colenbrander B, Gadella BM, Stout TAE (2003) The predictive value of semen analysis in the evaluation of stallion fertility. Reproduction in Domestic Animals 38(4), 305-311.
| Crossref | Google Scholar | PubMed |
Contreras MJ, Treulen F, Arias ME, Silva M, Fuentes F, Cabrera P, Felmer R (2020) Cryopreservation of stallion semen: effect of adding antioxidants to the freezing medium on sperm physiology. Reproduction in Domestic Animals 55(2), 229-239.
| Crossref | Google Scholar | PubMed |
Dorado J, Acha D, Ortiz I, Gálvez MJ, Carrasco JJ, Gómez-Arrones V, Calero-Carretero R, Hidalgo M (2014) Effect of extender and amino acid supplementation on sperm quality of cooled-preserved Andalusian donkey (Equus asinus) spermatozoa. Animal Reproduction Science 146(1–2), 79-88.
| Crossref | Google Scholar | PubMed |
Dorostkar K, Shoushtari SMA, Khaki A (2014) Effects of in vitro zinc sulphate additive to the semen extender on water buffalo (bubalusbubalis) spermatozoa before and after freezing. International Journal of Fertility & Sterility 8(3), 325.
| Google Scholar | PubMed |
Fallah A, Mohammad-Hasani A, Colagar AH (2018) Zinc is an essential element for male fertility: a review of zn roles in men’s health, germination, sperm quality, and fertilization. Journal of Reproduction & Infertility 19(2), 69.
| Google Scholar | PubMed |
Fayez E, El Sayed MAI, Rawash ZM, Salama A (2023) Influence of the addition of zinc oxide nanoparticles to cryopreservation medium for dog epididymal spermatozoa. Topics in Companion Animal Medicine 52, 100736.
| Crossref | Google Scholar | PubMed |
Ghallab ARM, Shahat AM, Fadl AM, Ayoub MM, Moawad AR (2017) Impact of supplementation of semen extender with antioxidants on the quality of chilled or cryopreserved Arabian stallion spermatozoa. Cryobiology 79, 14-20.
| Crossref | Google Scholar | PubMed |
Gibb Z, Aitken RJ (2016) The impact of sperm metabolism during in vitro storage: the stallion as a model. BioMed Research International 2016, 9380609.
| Crossref | Google Scholar |
Gualtieri R, Barbato V, Fiorentino I, Braun S, Rizos D, Longobardi S, Talevi R (2014) Treatment with zinc, d-aspartate, and coenzyme Q10 protects bull sperm against damage and improves their ability to support embryo development. Theriogenology 82(4), 592-598.
| Crossref | Google Scholar | PubMed |
Henkel R, Bittner J, Weber R, Hüther F, Miska W (1999) Relevance of zinc in human sperm flagella and its relation to motility. Fertility and Sterility 71(6), 1138-1143.
| Crossref | Google Scholar | PubMed |
Höfner L, Luther A-M, Waberski D (2020) The role of seminal plasma in the liquid storage of spermatozoa. Animal Reproduction Science 220, 106290.
| Crossref | Google Scholar | PubMed |
Isaac AV, Kumari S, Nair R, Urs DR, Salian SR, Kalthur G, Adiga SK, Manikkath J, Mutalik S, Sachdev D, Pasricha R (2017) Supplementing zinc oxide nanoparticles to cryopreservation medium minimizes the freeze-thaw-induced damage to spermatozoa. Biochemical and Biophysical Research Communications 494(3–4), 656-662.
| Crossref | Google Scholar | PubMed |
Kandiel MMM, El Khawagah ARM (2018) Evaluation of semen characteristics, oxidative stress, and biochemical indices in Arabian horses of different ages during the hot summer season. Iranian Journal of Veterinary Research 19(4), 270-275.
| Google Scholar | PubMed |
Khodaei-Motlagh M, Masoudi R, Karimi-Sabet MJ, Hatefi A (2022) Supplementation of sperm cooling medium with zinc and zinc oxide nanoparticles preserves rooster sperm quality and fertility potential. Theriogenology 183, 36-40.
| Crossref | Google Scholar | PubMed |
Kotdawala AP, Kumar S, Salian SR, Thankachan P, Govindraj K, Kumar P, Kalthur G, Adiga SK (2012) Addition of zinc to human ejaculate prior to cryopreservation prevents freeze-thaw-induced DNA damage and preserves sperm function. Journal of Assisted Reproduction and Genetics 29, 1447-1453.
| Crossref | Google Scholar | PubMed |
Lee SR (2018) Critical role of zinc as either an antioxidant or a prooxidant in cellular systems. Oxidative Medicine and Cellular Longevity 2018, 9156285.
| Crossref | Google Scholar |
Leemans B, Gadella BM, Stout TAE, De Schauwer C, Nelis H, Hoogewijs M, Van Soom A (2016) Why doesn’t conventional IVF work in the horse? The equine oviduct as a microenvironment for capacitation/fertilization. Reproduction 152(6), R233-R245.
| Crossref | Google Scholar | PubMed |
Len J, Beehan D, Eilts B, Ebrahimie E, Lyle S (2020) Stallion sperm integrity after centrifugation to reduce seminal plasma concentration and cool storage for 4 days. Journal of Equine Veterinary Science 85, 102819.
| Crossref | Google Scholar | PubMed |
Maitan P, Bromfield EG, Stout TAE, Gadella BM, Leemans B (2022) A stallion spermatozoon’s journey through the mare’s genital tract: in vivo and in vitro aspects of sperm capacitation. Animal Reproduction Science 246, 106848.
| Crossref | Google Scholar | PubMed |
Ortiz I, Felix M, Resende H, Ramírez-Agámez L, Love CC, Hinrichs K (2021) Flow-cytometric analysis of membrane integrity of stallion sperm in the face of agglutination: the ‘zombie sperm’ dilemma. Journal of Assisted Reproduction and Genetics 38(9), 2465-2480.
| Crossref | Google Scholar | PubMed |
Peña FJ, Ortiz-Rodríguez JM, Gaitskell-Phillips GL, Gil MC, Ortega-Ferrusola C, Martín-Cano FE (2022) An integrated overview on the regulation of sperm metabolism (glycolysis–Krebs cycle–oxidative phosphorylation). Animal Reproduction Science 246, 106805.
| Crossref | Google Scholar | PubMed |
Pesch S, Bergmann M, Bostedt H (2006) Determination of some enzymes and macro- and microelements in stallion seminal plasma and their correlations to semen quality. Theriogenology 66(2), 307-313.
| Crossref | Google Scholar | PubMed |
Schulze M, Mohammadpour F, Schröter F, Jakop U, Hönicke H, Hasenfuss T, Henne H, Schön J, Müller K (2021) Suitability of semen stress tests for predicting fertilizing capacity of boar ejaculates. Theriogenology 176, 73-81.
| Crossref | Google Scholar | PubMed |
Soltani L, Samereh S, Mohammadi T (2022) Effects of different concentrations of zinc oxide nanoparticles on the quality of ram cauda epididymal spermatozoa during storage at 4°C. Reproduction in Domestic Animals 57(8), 864-875.
| Crossref | Google Scholar | PubMed |
Sørensen MB, Stoltenberg M, Danscher G, Ernst E (1999) Chelation of intracellular zinc ions affects human sperm cell motility. Molecular Human Reproduction 5(4), 338-341.
| Crossref | Google Scholar | PubMed |
Squires EL (2005) Integration of future biotechnologies into the equine industry. Animal Reproduction Science 89(1–4), 187-198.
| Crossref | Google Scholar | PubMed |
Sutovsky P, Kerns K, Zigo M, Zuidema D (2019) Boar semen improvement through sperm capacitation management, with emphasis on zinc ion homeostasis. Theriogenology 137, 50-55.
| Crossref | Google Scholar | PubMed |
Veeramachaneni DNR, Moeller CL, Sawyer HR (2006) Sperm morphology in stallions: ultrastructure as a functional and diagnostic tool. Veterinary Clinics of North America: Equine Practice 22(3), 683-692.
| Google Scholar | PubMed |
Yánez-Ortiz I, Catalán J, Rodríguez-Gil JE, Miró J, Yeste M (2022) Advances in sperm cryopreservation in farm animals: cattle, horse, pig and sheep. Animal Reproduction Science 246, 106904.
| Crossref | Google Scholar | PubMed |
Yates DJ, Whitacre MD (1988) Equine artificial insemination. Veterinary Clinics of North America: Equine Practice 4(2), 291-304.
| Google Scholar | PubMed |
Zhao J, Dong X, Hu X, Long Z, Wang L, Liu Q, Sun B, Wang Q, Wu Q, Li L (2016) Zinc levels in seminal plasma and their correlation with male infertility: a systematic review and meta-analysis. Scientific Reports 6(1), 22386.
| Crossref | Google Scholar |
Zigo M, Kerns K, Sen S, Essien C, Oko R, Xu D, Sutovsky P (2022) Zinc is a master-regulator of sperm function associated with binding, motility, and metabolic modulation during porcine sperm capacitation. Communications Biology 5(1), 538.
| Crossref | Google Scholar | PubMed |