Effects of micronised bamboo powder on growth performance, intestinal development, caecal chyme microflora and metabolic pathway of broilers aged 24–45 days
Fawen Dai
A College of Life Science, Leshan Normal University, No. 778, Binghe Road, Leshan, Sichuan 614000, China.
B Bamboo Diseases and Pests Control and Resources Development Key Laboratory of Sichuan Province, Leshan, Sichuan 614000, China.
C Guang’an Feed Industry Management Office, Guang’an, Sichuan 638000, China.
D College of Animal Science, South China Agricultural University, Guangzhou, Guangdong 510642, China.
Handling Editor: Natalie Morgan
Animal Production Science 63(12) 1196-1207 https://doi.org/10.1071/AN22363
Submitted: 28 September 2022 Accepted: 27 April 2023 Published: 29 May 2023
© 2023 The Author(s) (or their employer(s)). Published by CSIRO Publishing. This is an open access article distributed under the Creative Commons Attribution-NonCommercial-NoDerivatives 4.0 International License (CC BY-NC-ND)
Abstract
Context: Our previous study revealed that micronised bamboo powder (MBP) can promote the growth performance of broilers aged 1–22 days by improving oxidation resistance, balancing intestinal microflora and regulating metabolic pathways.
Aims: This study evaluates the effects of MBP on growth performance, intestinal development, caecal chyme microflora and metabolic pathways of broilers during the grower period, age 24–45 days.
Methods: Slow-growing spotted-brown broilers (n = 880, age 24 days) were randomly divided into two groups according to weight and sex and fed either a maize-based diet (control) or a diet with MBP at 1% replacing equivalent maize.
Key results: No significant difference was observed in growth performance between MBP and control groups. Broilers receiving MBP exhibited significantly higher (P < 0.05) caecal organ index, jejunum villus height and villi:crypt ratio. The relative abundance of Firmicutes was higher, and relative abundance of Bacteroidetes was lower in the MBP group. Addition of MBP also significantly (P < 0.05) upregulated abundance of p_Firmicutes, f_Alicyclobacillaceae, g_Acutalibacter, f_Peptococcaceae, f_Clostridiaceae, f_Bacillaceae, g_Enterococcus and f_Enterococcaceae, while downregulating abundance of p_Bacteroidetes, f_Bacteroidaceae, g_Bacteroides, o_Bacteroidales and c_Bacteroidia. For the metabolic pathways, 66 were observed to differ between dietary groups, including alanine, aspartic acid and glutamic acid metabolism, butyric acid metabolism, arginine synthesis, linoleic acid metabolism and β-alanine metabolism. Correlation analysis revealed that Firmicutes in caecal chyme were significantly positively correlated (P < 0.05) with some fatty acids including syringic, 3-methyl-2-oxovaleric, 3-(2-hydroxyphenyl) propanoic and butyric acids. Bacteroidetes were positively correlated (P < 0.05) with some amino acids including L-alanine, L-threonine, 3-methylthiopropionic acid and L-glutamic acid.
Conclusions: Replacing maize with MBP at 1% had no negative effect on growth performance of broilers. Feeding MBP improved intestinal development and increased the relative abundance of bacteria that promotes fatty acid metabolism and fibre degradation.
Implications: MBP provides an alternative to maize and is a beneficial source of fibre; further research is warranted to determine the optimum feeding level.
Keywords: broiler, correlation, growth performance, intestinal development, intestinal microflora, metabolic pathway, micronised bamboo powder (MBP), replacing corn.
Introduction
Supply shortages of maize, soybean and other feed raw materials have become a key factor restricting the sustainable and efficient development of the poultry industry. Feed manufacturers need to consider other unconventional feed resources (Makkar 2018). Since the ‘Prohibition of growth-promoting antibiotics’ policy was implemented in China, nutritional strategies such as probiotics and prebiotics to improve animal intestinal health and growth performance and promote feed utilisation efficiency have become some of the most effective measures for antibiotics replacement (Jiménez-Moreno et al. 2019).
Research on dietary fibre for broilers has focused on the effect of the fibre on growth performance (Kheravii et al. 2017). According to the conventional view, significantly increasing cellulose levels may dilute the nutrients available from the diet for broilers. Increasing the levels of dietary lignocellulose were shown to reduce apparent digestibility of organic matter and gross energy in slow-growing broilers (Röhe et al. 2020). However, some studies have proved that supplementation of insoluble dietary fibre (IDF) in a moderate way also exhibited several advantages for promoting the growth of young broilers, such as increasing gizzard weight and lowering gizzard pH (Jiménez-Moreno et al. 2019), balancing beneficial gut bacteria (Pourazadi et al. 2020), improving villus growth in jejunum (Sozcu 2019), and enhancing nutrient digestibility and utilisation (Nassar et al. 2019). These reports show that dietary fibre may be a kind of functional feed material for poultry.
Bamboo grows fast, with a high yield (He et al. 2014). Bamboo shoot shell fibre has been shown to have prebiotic potential and strong cholesterol-adsorption activity in vitro (Wu et al. 2020). Bamboo culm fibre is the most productive part of bamboo, and the total fibre value of young bamboo culm (>60%) is more than that of the powdered bamboo shoot (~24.44% crude fibre) (Mustafa et al. 2016; Felisberto et al. 2017). Compared with bamboo shoot, the powdered bamboo culm contains a growing amount of lignin (26–36%) with age (Zhu et al. 2020) and higher content of IDF, which might reduce its nutritional value. Owing to the richness of bamboo resources in China, and if the powdered bamboo culm can be used for poultry feed, it will help to alleviate the shortage of feed materials.
Micronisation of other plant powder such as olive pomace resulted in a redistribution of fibre fractions (from insoluble to soluble) and a decrease in lignin content (Speroni et al. 2020). Superfine processing could improve the functional properties of rice bran IDF, including greater water-holding capacity, swelling capacity and nitrite ion adsorption capacity than other IDF powders (Zhao et al. 2018). The particle size of IDF (cellulose, soyhulls) was shown to be an important factor in the modulation of nutrient digestibility, where smaller particles (100 μm) increased the nutrient digestibility of broilers compared with larger ones (600 μm) (Tejeda and Kim 2021). These reports show that micronised treatment can improve fibre properties and nutritional value.
Our previous study revealed that adding micronised bamboo powder (MBP) (neutral detergent fibre (NDF) 82.94%) from 5–6-year-old bamboo culm at a rate of 1% to replace equivalent maize improved the growth performance of weaned piglets, and the improvement effect of MBP was higher than that of general bamboo powder (Dai et al. 2021). In another study, we found that replacing maize with MBP at 1% in the diet of slow-growing broilers at age 1–22 days could promote growth performance by improving chyme microflora composition, increasing the relative abundance of phylum Firmicutes, and regulating the metabolism pathways responsible for intestinal fatty acids, amino acids and immunity, and that MBP tended to improve feed efficiency (Dai et al. 2022). The nutritional composition of MBP is different from that of maize, which is rich in starch. However, we found that MBP could partially replace maize, which might relate to its beneficial effects on intestinal health. As important indicators for intestinal structure integrity, the villus height and the crypt depth of the small intestine can, respectively, reflect the absorption capacity of the intestinal mucosa (Li et al. 2016) and the renewal rate of intestinal epithelial cells (Wang et al. 2021a).
The present study further evaluates whether MBP has similar growth-promoting and pro-biotic effects on broilers during the grower period (age 24–45 days). The study focuses on intestinal tissue development, microbiota balance and metabolic pathways, to provide insight into the mechanisms of dietary MBP effects in broiler chickens.
Materials and methods
Preparation of MBP
Bamboo culm of Phyllostachys pubescens (from Sichuan province, China) aged 5–6 years was collected and processed into MBP using the previously reported method (Dai et al. 2022). Contents of moisture, crude protein (CP) and ash were tested according to AOAC methods (AOAC 2007), and were 7.83%, 1.51% and 1.01%, respectively. Contents of IDF, soluble dietary fibre (SDF) and total fibre (TF) were determined according to AOAC method 991.43, and were 82.33%, 3.30% and 85.63%, respectively. Contents of acid detergent fibre (ADF) and NDF were determined using the method of Van Soest et al. (1991), and were 74.80% and 82.84%, respectively.
Experimental animals and groups
Slow-growing spotted-brown broilers (Jinling, an improved local breed; WEN’S Group, China; n = 880) aged 22 days were all pre-fed with the same diet (a mixed diet with half each of treatment and control) for 2 days, and then randomly divided to two groups according to weight (495.3 ± 36.0 g) and sex. There were 16 pens, 55 birds per pen and eight pens in each treatment. The broilers in the control group were fed with a maize-based diet that was antibiotic-free. The broilers in the experimental group were supplemented with MBP at 1% to replace the equivalent maize of the control diet. The broilers were housed in floor pens littered with 8 cm of rice hull and offered ad libitum access to feed and water. Each replicate pen was 2 m long by 1.8 m wide, and stocking density was 15 birds/pen. Ambient temperature was lowered to 25°C using a cold-air fan. The experiment lasted for 21 days, corresponding to broiler age 24–45 days.
Diet ingredients and nutrition level
The ingredients and nutrition level of the diet in this study were prepared according to the standard ‘Nutritional Requirements of Yellow Feather Broilers’ (NY/T3645-2020, Ministry of Agriculture and Rural Affairs of China). The composition and nutrition level are shown in Table 1. In the diet of the MBP group, the same amount of MBP (1%) was used to replace the equivalent of maize. The value of metabolisable energy (ME) in the two groups was the same according to our previous study; we found that adding MBP at 1% to replace equivalent maize had no negative effect on the growth performance of broilers (Dai et al. 2022). It was assumed that the energy value of MBP was equivalent to that of maize under the conditions of appropriate addition. The formulated diet was mixed and made into pellets with steam-conditioning at 80°C.
Ingredients | CON (basal diet) | MBP |
---|---|---|
Maize (g/kg) | 678.4 | 668.4 |
Maize gluten meal (g/kg) | 20 | 20 |
Soybean oil (g/kg) | 16 | 16 |
Fermented soybean meal (g/kg) | 30 | 30 |
Soybean meal (CP 46%) (g/kg) | 169 | 169 |
MBP (g/kg) | 10 | |
Cottonseed meal (CP 46%) (g/kg) | 40 | 40 |
Premix (g/kg)A | 46.6 | 46.6 |
Total (g/kg) | 1000 | 1000 |
Calculated composition | ||
ME (MJ/kg) | 12.56 | 12.56 |
CP (%) | 17.73 | 17.67 |
Crude fibre (%) | 2.78 | 3.19 |
NDF (%) | 9.52 | 10.27 |
Lys (%) | 1.00 | 1.00 |
Met (%) | 0.45 | 0.45 |
Thr (%) | 0.73 | 0.73 |
Ca (%) | 0.95 | 0.95 |
Available P (%) | 0.44 | 0.44 |
ASuppling per kg diet: vitamin A (retinyl acetate), 10 000 IU; vitamin D3 (cholecalciferol), 2500 IU; vitamin E (DL-α-tocopheryl acetate), 40 IU; vitamin K3 (menadione sodium bisulfite), 2 mg; vitamin B1 (thiamine), 2 mg; vitamin B2 (riboflavin), 6 mg; vitamin B3 (niacin), 50 mg; vitamin B5 (calcium D-pantothenate), 12 mg; vitamin B6 (pyridoxine hydrochloride), 5 mg; vitamin B12 (cyanocobalamin), 0.02 mg; biotin, 0.12 mg; folic acid, 1.5 mg; choline chloride, 600 mg; Mn(MnSO4.H2O), 80 mg; Fe(FeSO4.7H2O), 80 mg; Zn (sulfate and oxide) 60 mg; Cu(CuSO4.5H2O), 8 mg; I (iodide), 1 mg; Se(Na2SeO3), 0.3 mg; Co (cobalt), 0.3 mg; NaCl, 3 g; antioxidant, 40 mg.
CON, control diet, antibiotic-free basal diet; MBP, basal diet with MBP added at 1% to replace equivalent maize.
Slaughtering and sampling
On Day 45, two randomly selected broilers (one male and one female) in each replicate were fasted for 12 h and sacrificed by cervical dislocation, after which the broilers were dissected for collection of internal organs including heart, liver, spleen, bursa of Fabricius, gizzard and proventriculus. The chyme in the caecum was put in a 1.5-mL sterile centrifuge tube, frozen with liquid nitrogen and stored at −80°C for further tests. Intestinal tissue lengths of 0.5 cm were collected from the duodenum, jejunum and ileum, from which chyme was washed with phosphate-buffered saline (PBS). These tissues were then fixed with 4% formalin for the following assays.
Indicators and methods of determination
At the beginning and end of the experiment, the average initial (IW) and final (FW) liveweights were determined per replicate. Feed intake (FI) was recorded per replicate daily for calculating the average daily FI (ADFI). Average daily gain (ADG) was calculated as difference between IW and FW, and the ratio of gain to feed consumption (G:FI) was calculated as ADG/ADFI.
The contents in the gizzard and proventriculus were removed. The tissues were rinsed with PBS and dried with filter paper to absorb the moisture. Other tissues and fat were removed for weighing. The duodenum, jejunum, ileum, colorectum and caecum were separated. The length of each intestinal tract was measured to the accuracy of 0.1 cm. Organ indexes were calculated as follows: organ index (%) = weight of organ/live broiler weight × 100; and intestinal organ index (cm/g) = length of intestine/live broiler weight.
The duodenum, jejunum and ileum of broilers were embedded in paraffin for preparation of sections. Samples were cut at a thickness of 5 μm and stained with hematoxylin and eosin. The values of villus height (VH) and crypt depth (CD) were obtained via optical microscopy (Leica Microsystems, Wetzlar, Germany). Twenty spots of villus and crypt from the intestinal samples were measured for calculation of the ratio VH:CD.
Caecal chyme microflora analysis was performed according to our previous report (Dai et al. 2022). Genomic DNA of caecal chyme microflora was extracted and the V3–V4 region of the 16S rRNA gene was amplified with PCR. The amplification products were purified and sequenced using the Illumina MiSeq platform (BioNovoGene, Suzhou, China). The raw reads were deposited in the European Nucleotide Archive database (Accession No.: ERP131138).
The raw sequence data generated from 16S rRNA MiSeq sequencing was processed according to the analysis flow of the QIIME 2 (ver. 2019.4, https://docs.qiime2.org/2019.4/tutorials/) DADA2 plugin method, and sequence denoising was performed. Low-quality sequences were filtered using the following criteria: length <150 bp, average Phred quality score <20, containing ambiguous bases, and containing mononucleotide repeats >8 bp. Operational taxonomic units (OTUs) were clustered with 97% similarity cutoff using Vsearch software (Rognes et al. 2016). Greengenes, SILVA, UNITE and nt databases were involved in the analysis. The microbial species were annotated with the QIIME 2 classify-sklearn algorithm and BROCC algorithm.
For 16S rRNA analysis, gene sequencing data were normalised by copy number. Phylum and genus at <1.0% relative abundance for both groups were excluded from all analyses. Alpha diversity analyses were performed using mothur software (ver. 1.35.1, https://github.com/mothur/mothur/releases/tag/v1.35.1). Community richness was identified using Chao1, observed species and Faith’s PD indexes. Community diversity was identified using the Shannon, Simpson and Pielou indexes. Coverage estimator was characterised by Good’s coverage.
A 100-mg sample was accurately weighed, and 0.6 mL 2-chlorophenylalanine in methanol (4 μL/L, −20°C) was added and mixed for 30 s. After adding glass beads (100 mg), the solution was ground for 90 s at 60 Hz in a tissue grinder, and then sonicated for 10 min at room temperature. After centrifuging at 10 656g for 10 min, 300 μL supernatant was filtered through a 0.22-μm membrane. The filtrate was stored for the following detection. A subsample (20 μL) of each sample was taken and mixed into a quality control (QC) sample. The remainder was used for liquid chromatography-mass spectrometry (LC-MS) detection. The LC was performed with an UltiMate 3000 (Thermo Fisher, Waltham, MA, USA) and ACQUITY UPLC HSS T3 1.8 μm (2.1 mm × 150 mm) column (Waters, Milford, MA, USA); and MS analysis was performed with a Q Exactive HF-X (Thermo Fisher), electrospray ion source (ESI), positive and negative ion ionisation modes. The detection conditions were set according to our previous report (Dai et al. 2022).
Raw data were collected and converted into mzXML format by ProteoWizard software (ver. 3.0.8789, https://proteowizard.sourceforge.io). The XCMS package of R (ver. 3.3.2, The R Foundation, Vienna, Austria) was applied for peak identification, peak filtration and peak alignment. Information including mass to charge ratio (m/z), retention time and peak intensity was obtained.
To find biomarkers, the relative standard deviation (RSD) of potential characteristic peaks in QC samples should be <30%. The characteristic peak with RSD <30% in this study was 77.1% in positive ion mode and 81.5% in negative ion mode, indicating that the data were good. The acquired data were processed with orthogonal partial least squares discriminant analysis (OPLS-DA) in the Mann–Whitney–Wilcoxon Test. Metabolites with variable importance value projection (VIP) >1 and P < 0.05 were considered as metabolites that could discriminate between the two groups.
Accurate molecular weight of the metabolite (error <30 μL/L) was obtained. Then, the fragment information obtained according to the MS/MS mode was retrieved in the Human Metabolome Database (HMDB), METLIN, MassBank, LIPID MAPS and mzCloud. The information was matched and annotated to obtain accurate information about metabolites. The impact of MBP on metabolic pathways and metabolite-set enrichment analysis were evaluated based on an online tool (www.metaboanalyst.ca).
Data processing and statistical analyses
SPSS 25.0 (IBM, Armonk, NY, USA) was applied for statistical analysis. Independent sample t-test was applied for analysing differences in growth performance, organ indexes, intestinal histology and microbiota diversity indexes. Data were reported as means ± s.e.m. Differences were considered significance at P ≤ 0.05, and a tendency was considered to exist at P-values >0.05 and <0.10.
The microbial differential species markers in caecal chyme were analysed with linear discriminant analysis (LDA) effect size (LEfSe), combined with nonparametric Kruskal–Wallis and Wilcoxon rank sum test. Spearman correlation analysis was applied to assess the correlations between microbiota at phylum level and metabolites in caecal chyme of broilers.
Ethics approval
All aspects of animal care and handling were approved by the Ethics Committee for Animal Experimentation, Science and Technology Department, Leshan Normal University, Sichuan, China. The protocols were supported by the regulations for animal experiments established by the Ministry of Science and Technology in China (2014).
Results
Effects of MBP on growth performance of broilers
Growth performance of broilers aged 24–45 days is shown in Table 2. No significant differences were observed in ADG, AFDI and G:FI between control and MBP groups.
CON | MBP | P-value | |
---|---|---|---|
Initial weight (IW) (g) | 483.6 ± 42.2 | 507.1 ± 36.1 | 0.429 |
Final weight (FW) (g) | 1333.3 ± 74.6 | 1377.3 ± 88.7 | 0.477 |
Average daily gain (ADG) (g/day) | 40.5 ± 2.5 | 41.4 ± 2.6 | 0.603 |
Average daily feed intake (ADFI) (g) | 94.1 ± 4.9 | 95.9 ± 3.5 | 0.562 |
Ratio of gain to feed consumption (G:FI) | 0.43 ± 0.02 | 0.43 ± 0.02 | 0.620 |
CON, control group, fed antibiotic-free basal diet; MBP, group fed basal diet with MBP added at 1% to replace equivalent maize.
Effects of MBP on organic indexes of broilers
No significant differences were found in organ indexes of the heart, liver, spleen, bursa of Fabricius, gizzard, proventriculus, duodenum, jejunum, ileum and colorectum between control and MBP groups (Fig. 1). The organ index of the caecum was significantly higher (P < 0.05) in the MBP than the control group, with an increase of ~15.79%.
Effects of dietary MBP on organ indexes of broilers: (a) organ index (%) = weight of organ/live broiler weight × 100; (b) intestinal organ index (cm/g) = length of intestine/live broiler weight. CON, control group, fed antibiotic-free basal diet; MBP, group fed basal diet with MBP added at 1% to replace equivalent maize. Data are presented as means ± s.e.m., n = 8. *P < 0.05.
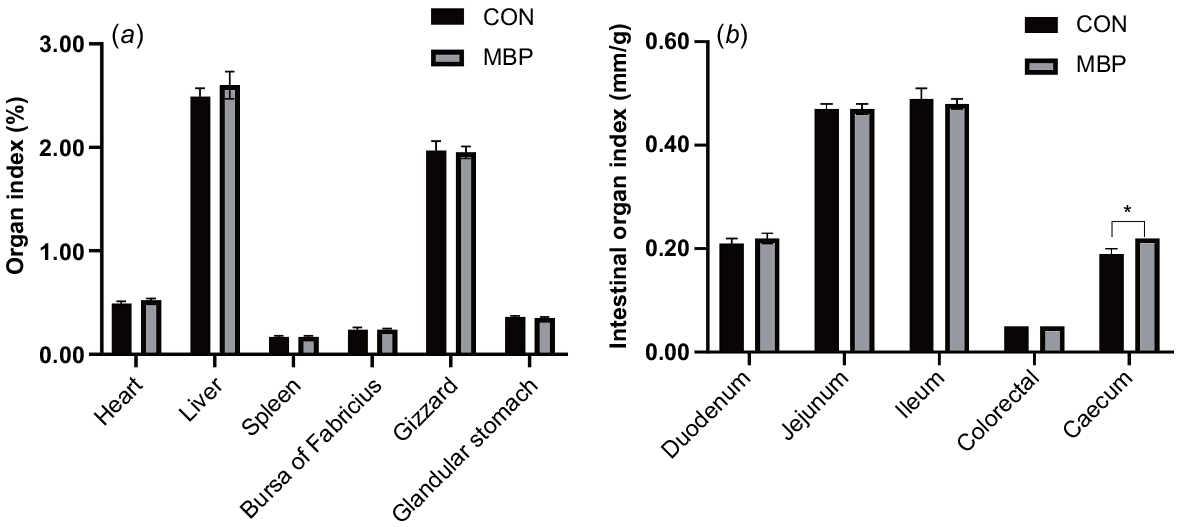
Effects of MBP on intestinal histology of broilers
With regard to intestinal histology, the broilers in the MBP group had significantly (P < 0.05) higher VH of the jejunum and ratio VH:CD than the control broilers, with increases of 29.66% and 28.77%, respectively (Fig. 2). No significant differences were found in VH, CD and ratio of VH:CD in the duodenum and ileum between treatment groups.
Effects of dietary MBP on intestinal histology of broilers: (a) villus height, (b) crypt depth, (c) villus height/crypt depth. CON, control group, fed antibiotic-free basal diet; MBP, group fed basal diet with MBP added at 1% to replace equivalent maize. Data are presented as means ± s.e.m., n = 8. *P < 0.05.
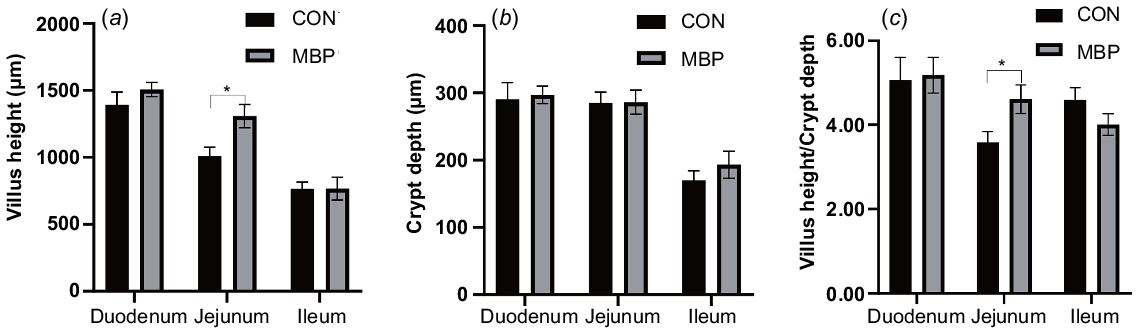
Effects of MBP on diversity and abundance of microflora in caecal chyme
There were 15 630 OTUs in the control group and 15 464 in the MBP group. The two groups shared 5100 OTUs, accounting for 19.62% of the total OTUs. The MBP group had 10 364 unique OTUs, accounting for 39.87% of the total OTUs found. Bacterial diversity estimators (Shannon, Simpson and Pielou), richness estimators (Chao1, observed species and Faith’s PD), and coverage estimator (Good’s coverage) are shown in Table 3. No significant difference was found in any of the indexes between the treatment groups.
Items | CON | MBP | P-Value |
---|---|---|---|
Chao1 | 3864 ± 771 | 3567 ± 800 | 0.491 |
Observed species | 3344 ± 776 | 3066 ± 755 | 0.509 |
Faith’s PD | 118.58 ± 25.20 | 112.99 ± 24.89 | 0.682 |
Shannon | 7.73 ± 1.54 | 7.47 ± 1.08 | 0.725 |
Simpson | 0.93 ± 0.06 | 0.93 ± 0.04 | 0.809 |
Pielou | 0.66 ± 0.11 | 0.65 ± 0.07 | 0.795 |
Good’s coverage | 0.984 ± 0.004 | 0.984 ± 0.003 | 0.793 |
CON, control group, fed antibiotic-free basal diet; MBP, group fed basal diet with MBP added at 1% to replace equivalent maize.
Combined with the results of data alignment in the databases and OTU species classification, the top 20 in caecal chyme microbiota composition were plotted in different groups at the levels of phylum and genus (Fig. 3). It can be seen that, in both MBP and control groups, the dominant microflora at the phylum level were Firmicutes, Bacteroidetes, Proteobacteria, etc. At the genus level, Bacteroides, Megamonas and Faecalibacterium were the dominant bacteria. However, the abundance of microflora was different between the two treatments groups. In the MBP group, the relative abundance of Firmicutes was higher than in the control group, whereas the relative abundance of Bacteroidetes was lower at the phylum level. MBP reduced the relative abundance of Bacteroides at the genus level.
Effects of dietary MBP on the caecal chyme microbial composition of broilers. Relative abundance of caecal chyme microbiota at (a) phylum level and (b) genus level. CON, control group, fed antibiotic-free basal diet; MBP, group fed basal diet with MBP added at 1% to replace equivalent maize. Data are presented as mean ± s.d. (n = 8).
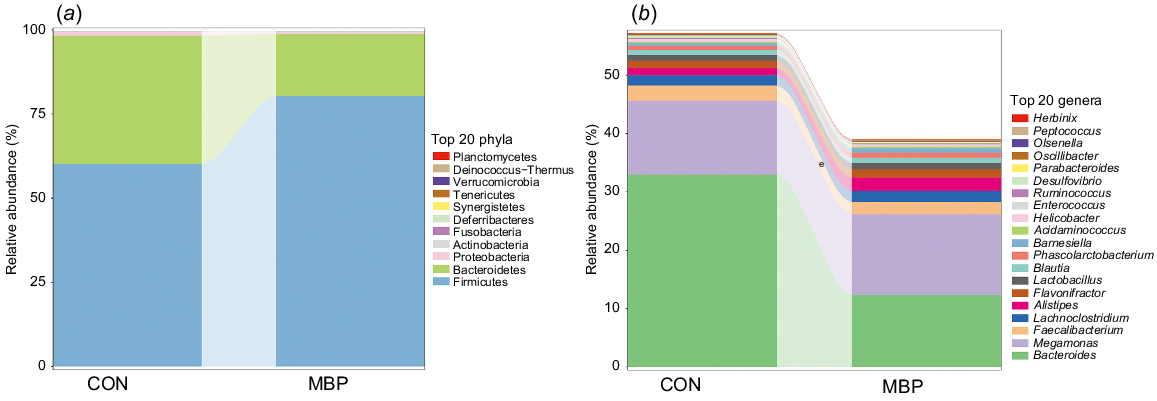
Results of the LEfSe algorithm module are presented in Fig. 4. Addition of MBP at 1% to the broiler diet significantly upregulated expression abundances of p_Firmicutes, f_Alicyclobacillaceae, g_Acutalibacter, f_Peptococcaceae, f_Clostridiaceae, f_Bacillaceae, g_Enterococcus and f_Enterococcaceae (P < 0.05), and significantly downregulated expression abundances of p_Bacteroidetes, f_Bacteroidaceae, g_Bacteroides, o_Bacteroidales and c_Bacteroidia in caecal chyme of broilers (P < 0.05).
Comparison of microbiota analysed with LEfSe. (a) Histogram of LDA effect values of marker species. Note: ordinate represents taxa with significant differences between groups (P < 0.05), and abscissa visually displays the logarithmic score of LDA analysis for each taxon in a bar chart. (b–f) Relative abundance distribution of marker species p_Firmicutes, f_Alicyclobacillaceae, g_Acutalibacter, f_Peptococcaceae and f_Clostridiaceae in the two treatment groups. CON, control group, fed antibiotic-free basal diet; MBP, group fed basal diet with MBP added at 1% to replace equivalent maize.
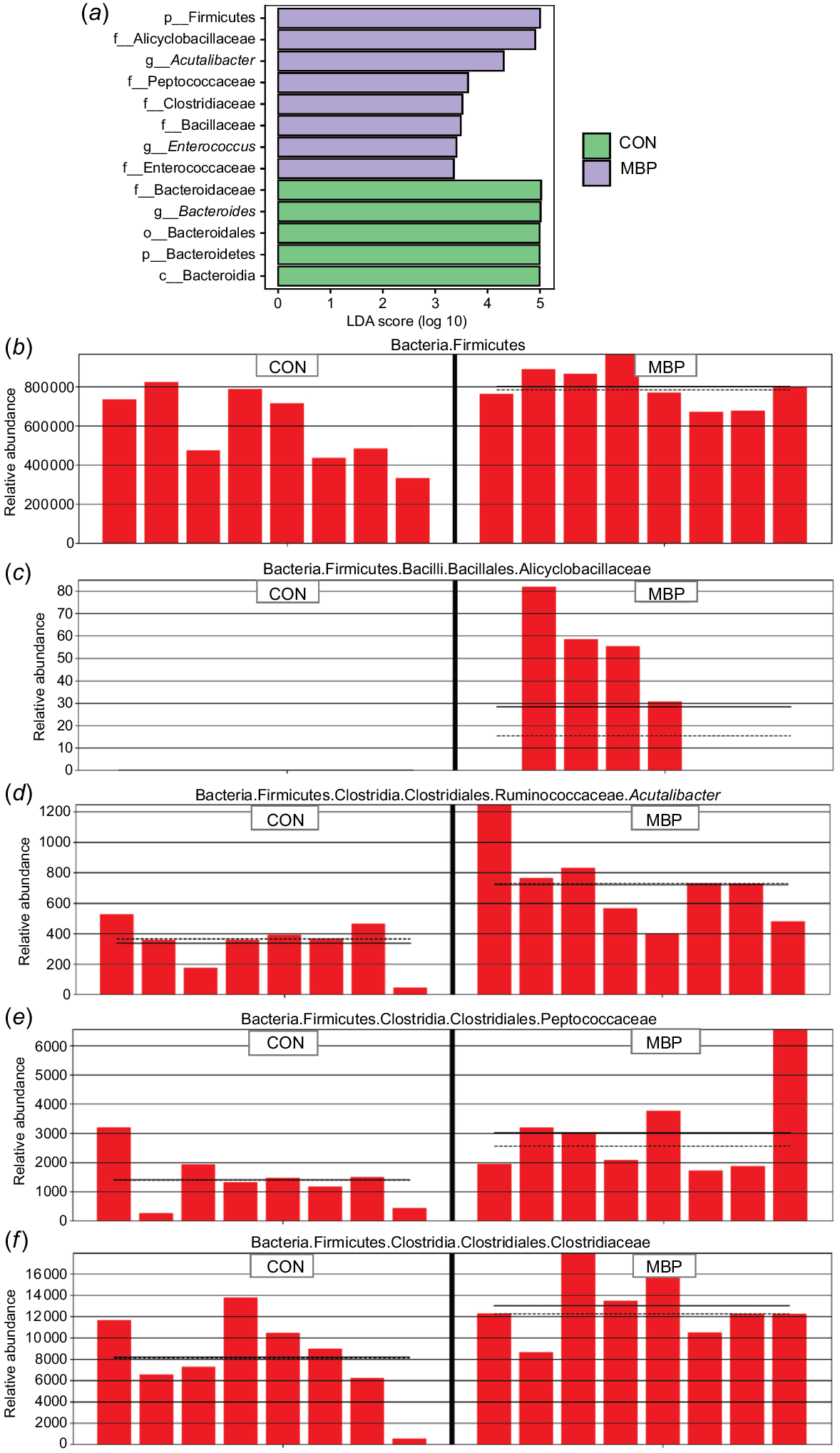
Effects of MBP on chyme metabolic pathways in broilers
Typical metabolite chromatograms are presented in Fig. 5. PLS-DA and OPLS-DA models showed an obvious separation in metabolites between treatment groups, suggesting that the fecal metabolic profiles have been greatly changed by addition of MBP.
Effects of dietary MBP on chyme metabolites in broilers: (a) chromatogram of basal peaks of typical caecal chyme samples of broilers in the two treatment groups; (b) PLS-DA and OPLS-DA scores of caecal chyme of broilers in the two groups. CON, control group, fed antibiotic-free basal diet; MBP, group fed basal diet with MBP added at 1% to replace equivalent maize.
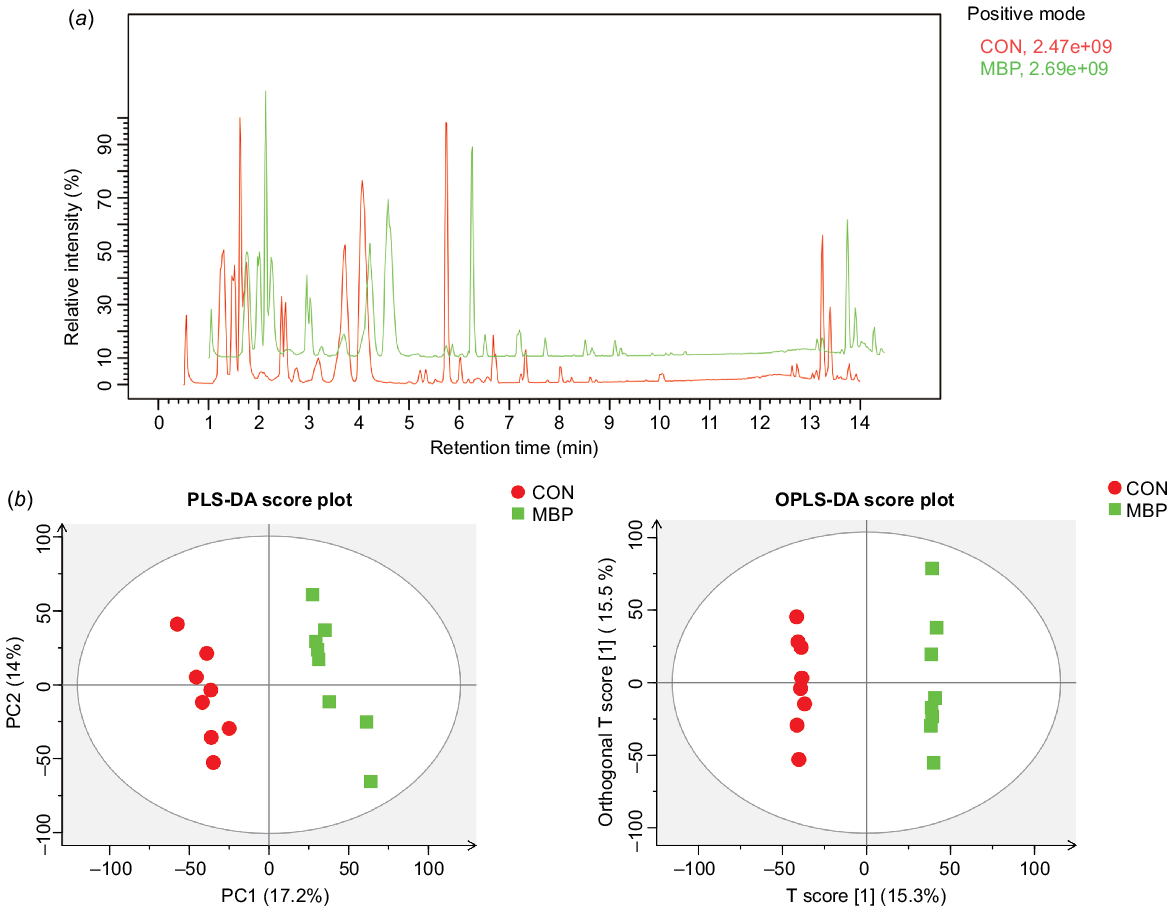
The metabolic pathways of different metabolites are presented in Fig. 6. Compared with the control group, there were 1635 upregulated and 837 downregulated primary metabolites in the MBP group. In total, 66 different metabolic pathways were identified between treatment groups, mainly including alanine, aspartic acid and glutamic acid metabolism, butyric acid metabolism, arginine synthesis, linoleic acid metabolism, and β-alanine metabolism.
Effects of dietary MBP on chyme metabolic pathways in broilers: (a) histogram of differential metabolites; (b) metabolome view map of the differential metabolites (VIP >1, P < 0.05) identified in caecal chyme of young broilers between the MBP and control (CON) groups. The node colour is based on its P-value, and the node radius is determined based on the pathway impact values. Larger sizes and darker colours represent higher pathway enrichment and impact values, respectively.
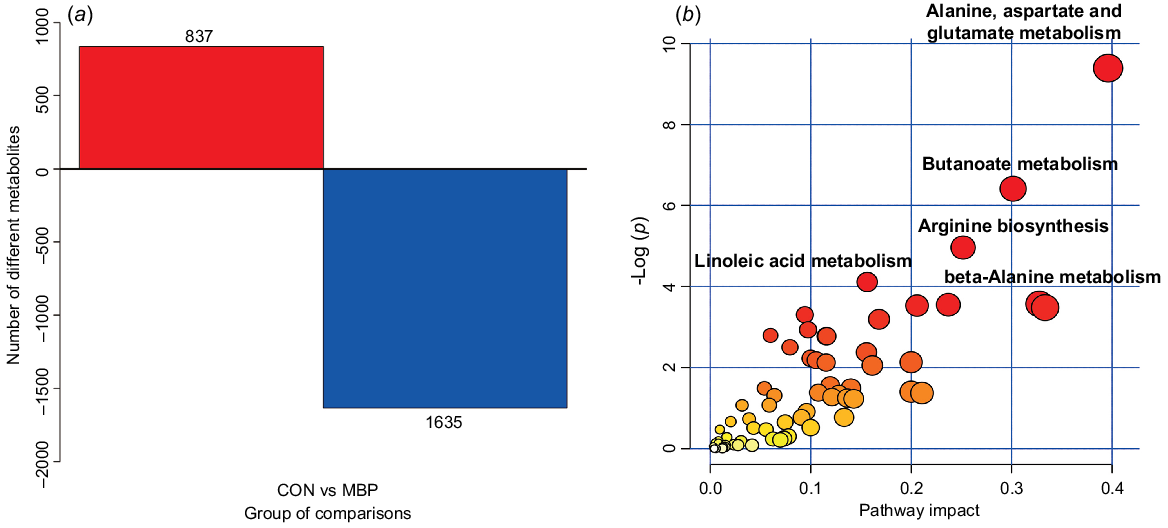
Correlation between caecal microflora at phylum level and metabolites in broilers
The correlation between microflora at phylum level and metabolites in caecal chyme of broilers was analysed (Fig. 7). Firmicutes were positively correlated (P < 0.05) with syringic acid, 3-methyl-2-oxovaleric acid, 3-(2-hydroxyphenyl) propanoic acid and butyric acid, and negatively correlated (P < 0.05) with 3-(methylthio)propionic acid, L-alanine, γ-aminobutyric acid, L-glutamic acid and ornithine. Bacteroidetes were positively correlated (P < 0.05) with L-alanine, L-threonine, 3-(methylthio)propionic acid and L-glutamic acid, etc., and negatively correlated with butyric acid, 3-methyl-2-oxovaleric acid, 3-(2-hydroxyphenyl) propanoic acid, syringic acid, etc.
Discussion
Effects of MBP on growth performance
Previous studies with broilers proved that supplementation with insoluble fibre can promote growth of broilers (Jiménez-Moreno et al. 2016, 2019; Nassar et al. 2019). The effects of fibre on the growth performance of broilers is dependent on several factors including fibre resources (Pourazadi et al. 2020; Tejeda and Kim 2020), addition level (Tejeda and Kim 2021), particle size (Tejeda and Kim 2021) and feeding stage (Berrocoso et al. 2020). We found that the regulatory effect of MBP on the growth performance of broilers aged 24–45 days in this study was greater than its effect on growth performance of young broilers aged 1–22 days (Dai et al. 2022). There was no significant effect on ADG, ADFI or G:FI, which may be related to the application stage of MBP. However, adding MBP at 1% to replace an equivalent amount of maize had no negative effect on the performance of broilers in the middle growth stage.
Effects of MBP on the development of the gastrointestinal tract
Several studies have reported that adding insoluble fibres to the diet can promote the gastrointestinal development of broilers, with improvement in digestive organ development and intestinal histology, and then improve the digestion and absorption of nutrients, as well as the ability to repair intestinal damage. Processed lignocellulose added to feed at 0.5–2 kg/t can promote the development of jejunum villi in broilers (Sozcu 2019). Supplementation with IDF (Arbocel RC) at 1% can improve digestive organ weights and enzyme activities in pullets, which may contribute to an improvement in feed utilisation (Yokhana et al. 2016). Adding soybean hull at 4% to the maize–soybean meal diet can increase villus height in the duodenum and jejunum of broilers, thus improving amino acid digestibility (Tejeda and Kim 2020). Supplementing wheat bran at 3% in the diet of broilers was shown to be beneficial to gizzard development, intestinal digestive enzyme activities and morphology of broilers (Shang et al. 2020). The fibre composition of lignocellulose, Arbocel RC, soybean hull and wheat bran in the above studies is similar to that of MBP, which is mainly composed of insoluble fibres. Similarly, bamboo leaf fibre, which is rich in IDF, could increase intestinal length of broilers (Bersalona 2015). Bamboo charcoal containing 1.0% bamboo vinegar could also significantly improve the structure of villi in laying hens (Rattanawut 2014).
Bamboo powder is rich in insoluble fibre, with a content of ~62.54–89.79% (Felisberto et al. 2017). MBP is a type of concentrated fibre raw material, and its IDF content reached 82.33% in the present study. Similar to previous research, our study has found that adding MBP at only 1% to the diet significantly increased the VH of jejunum, the ratio VH:CD, and the index of digestive organs (caecum). This may be related to the physicochemical properties of IDF, such as water-holding capacity, oil-holding capability, swelling capacity and cholesterol absorption capability. These physicochemical properties have a good correlation with the function of the IDF of tea; tea IDF significantly enhanced the production of short chain fatty acids during in vitro fermentation (Bai et al. 2022). MBP may similarly improve the digestion and absorption ability of broilers by promoting intestinal development, which may be one of the reasons why the growth performance was not reduced after replacing maize with equivalent MBP (1%).
Effects of MBP on caecal chyme microflora
Our study found that the composition of the caecal chyme microbiota was essentially stable in broilers of the two groups at the phylum level and genus level. At the phylum level, the main bacteria were Firmicutes, followed by Bacteroidetes and Proteobacteria, whereas at the genus level, the dominant bacteria were Bacteroides, followed by Megamonas and Faecalibacterium. The results are consistent with a previous study, which reported that the Firmicutes ranked first among the dominant microorganisms in the caecum of broilers, followed by Proteobacteria and Bacteroidetes (Sergeant et al. 2014). This indicates that MBP at 1% might not interfere with the natural composition of caecal microflora of broilers; instead, it is more likely to regulate the relative abundance of caecal microbiota.
Firmicutes are specialists in fibre degradation (Sun et al. 2021a); their cellulase and cellulosomes can degrade dietary fibres into monosaccharides that could be used as an energy source (Artzi et al. 2017). The present study revealed that adding MBP at 1% had no significant effect on the diversity of caecal microorganisms, but the abundance proportion of Firmicutes in caecal chyme was increased, leading to the lower abundance proportion of other bacteria such as Bacteroidetes. Specifically, MBP can significantly upregulate the abundance of p_Firmicutes, f_Alicyclobacillaceae, g_Acutalibacter, f_Peptococcaceae, f_Clostridiaceae, f_Bacillaceae, g_Enterococcus and f_Enterococcaceae, all of which are key degraders of dietary fibre (Suen et al. 2011; Leth et al. 2018). Another study also found that adding lignocellulose increased the relative abundance of cellulose-degrading Prevotellaceae UCG-001 and Alloprevotella, without improving the diversity of microorganisms (Sun et al. 2021b). Another study of various IDFs and SDFs showed broader effects with more and different types of gut microbial species, which may be due to the type and dose of fibre (Swanson et al. 2020). It indicated that the addition of MBP may improve fibre metabolism by improving the microflora composition, and MBP was expected to be a high-quality raw material for providing prebiotic lignocellulose.
Effects of MBP on metabolic pathways of caecal chyme
Given the significant differences in chyme microbiota, we next investigated the alterations of chyme metabolic pathways. Through metabolic pathway enrichment analysis, this study found alanine, aspartic acid and glutamic acid metabolism, butyric acid metabolism, arginine synthesis, linoleic acid metabolism and β-alanine metabolism to be considerably affected by MBP. The results show that MBP might have the potential to regulate the metabolism of amino acids and fatty acids in broilers. An alteration in the levels of caecal acetate and iso-butyrate was more pronounced for young broilers with inulin in the diet than with wheat bran (Li et al. 2018). Another study found that dietary mixed fibre (4% insoluble fibre/4% soluble fibre) promoted an improvement in gut microbiota and short-chain fatty acid (SCFA) profile, and the multiplicity of gut microbiota was useful in sustaining colonic integrity by producing SCFAs to some extent (Zhai et al. 2018). Rice bran from specific rice cultivars resulted in significant changes including decreased Firmicutes abundance and an increased number of metabolites associated with fatty acid metabolism (Rubinelli et al. 2017). Another study found that increasing the levels of dietary lignocellulose significantly lowered caecal counts of Escherichia/Hafnia/Shigella and reduced the total concentration of SCFAs (Röhe et al. 2020). The above reports indicated that addition of dietary fibre mainly with IDF may regulate the chyme microbiota and SCFA profile, and is associated with fibre source and addition level. Similarly, MBP is rich in IDF and its addition at 1% increased the relative abundance of Firmicutes in the caecal chyme of broilers. Meanwhile, Firmicutes were positively correlated with syringic acid, 3-methyl-2-oxovalenic acid, 3-(2-hydroxyphenyl) propanoic acid and butyric acid. This indicates that MBP might improve the metabolism of fatty acids by improving relative abundance of specific microbiota, and then promote the development of the caecum and intestinal health.
The gut microbiota perform a crucial function in facilitating the regulation of the amino acid pool and profile. Gut microbiota such as bacteria of the genera Fusobacterium, Bacteroides and Veillonella can synthesise several nutritionally essential amino acids de novo, a potential regulatory factor in amino acid homeostasis (Lin et al. 2017). Thus, the metabolism of amino acids might be regulated by specific microbiota. As a dietary fibre source with 26.4% crude fibre, California-type almond hulls at 9% in the diet could increase the population of certain bacteria in the genera Clostridium and Oscillospira and decrease ileal dry matter and ileal nitrogen digestibility of broilers (Wang et al. 2021b). Another study revealed that a high-fibre diet increased the relative abundance of Clostridium and Coprococcus and reduced apparent total tract digestibility of CP and 18 amino acids in finishing pigs (Hu et al. 2021). The above research showed that there was a strong link between dietary fibre, gut microbiota, and amino acid metabolism. The present study analysed the differential metabolites and found that MBP was involved in amino acid metabolism in caecal chyme of broilers aged 24–45 days. Specifically, adding MBP at 1% significantly reduced the abundance of Bacteroides in chyme, and Bacteroidetes were positively correlated with the production of L-alanine, L-threonine, 3-(methylthio)propionic acid and L-glutamic acid.
Conclusions
This study demonstrated that replacing maize with MBP at 1% in the diet of broilers aged 24–45 days had no significant effect on growth performance. However, the organ index of the caecum, the height of jejunal villus, and the abundance of cellulolytic bacteria in caecal chyme was significantly improved in birds fed MBP. Addition of MBP significantly affected the metabolite profile of caecal chyme and improved the metabolism of fatty acids with increasing the relative abundance of Firmicutes. The results indicate that MBP may be an efficacious prebiotic for broilers. Additional research is warranted into the optimal amount of maize that can be replaced with MBP.
Data availability
The data that support this study will be shared upon reasonable request to the corresponding author. A pre-print of the paper is available at https://doi.org/10.21203/rs.3.rs-1949857/v3.
Conflicts of interest
We declare that we have no financial and personal relationships with other people or organisations that can inappropriately influence our work. All results were strictly in accordance with the experimental data.
Declaration of funding
This work was supported by the Key Research Program (No. 2021YFN0106) and the Educational Reform Project (JG2021-1250) of Sichuan Province, and the Research Start-up Foundation of Leshan Normal University (No. RC202004).
Author contributions
Fawen Dai initiated the idea and designed the experiment. Fawen Dai, Tao Lin, Xia Huang and Yaojun Yang conducted the animal trial and laboratory index determination. Tao Lin and Fawen Dai prepared the initial manuscript in English. Xiang Nong, Jianjun Zuo and Dingyuan Feng made the final revision. All authors read and approved the final manuscript.
References
Artzi L, Bayer EA, Moraïs S (2017) Cellulosomes: bacterial nanomachines for dismantling plant polysaccharides. Nature Reviews Microbiology 15(2), 83-95.
| Crossref | Google Scholar |
Bai X, He Y, Quan B, Xia T, Zhang X, Wang Y, Zheng Y, Wang M (2022) Physicochemical properties, structure, and ameliorative effects of insoluble dietary fiber from tea on slow transit constipation. Food Chemistry: X 14, 100340.
| Crossref | Google Scholar |
Berrocoso JD, García-Ruiz A, Page G, Jaworski NW (2020) The effect of added oat hulls or sugar beet pulp to diets containing rapidly or slowly digestible protein sources on broiler growth performance from 0 to 36 days of age. Poultry Science 99(12), 6859-6866.
| Crossref | Google Scholar |
Dai FW, Lin T, Su BY, Yao HM, Gu DC, Yang YJ (2021) Effects of feeding bamboo powder on growth performance, serum biochemical indexes and fecal microorganism of weaned piglets. Chinese Journal of Animal Nutrition 33(12), 6709-6720 [In Chinese].
| Google Scholar |
Dai F, Lin T, Cheng L, Wang J, Zuo J, Feng D (2022) Effects of micronized bamboo powder on growth performance, serum biochemical indexes, cecal chyme microflora and metabolism of broilers aged 1-22 days. Tropical Animal Health and Production 54(3), 166.
| Crossref | Google Scholar |
Felisberto MHF, Miyake PSE, Beraldo AL, Clerici MTPS (2017) Young bamboo culm: potential food as source of fiber and starch. Food Research International 101, 96-102.
| Crossref | Google Scholar |
He M-X, Wang J-L, Qin H, Shui Z-X, Zhu Q-L, Wu B, Tan F-R, Pan K, Hu Q-C, Dai L-C, Wang W-G, Tang X-Y, Hu GQ (2014) Bamboo: a new source of carbohydrate for biorefinery. Carbohydrate Polymers 111, 645-654.
| Crossref | Google Scholar |
Hu P, Wang L, Hu Z, Jiang L, Hu H, Rao Z, Wu L, Tang Z (2021) Effects of multi-bacteria solid-state fermented diets with different crude fiber levels on growth performance, nutrient digestibility, and microbial flora of finishing pigs. Animals (Basel) 11(11), 3079.
| Crossref | Google Scholar |
Jiménez-Moreno E, de Coca-Sinova A, González-Alvarado JM, Mateos GG (2016) Inclusion of insoluble fiber sources in mash or pellet diets for young broilers. 1. Effects on growth performance and water intake. Poultry Science 95(1), 41-52.
| Crossref | Google Scholar |
Jiménez-Moreno E, González-Alvarado JM, de Coca-Sinova A, Lázaro RP, Cámara L, Mateos GG (2019) Insoluble fiber sources in mash or pellets diets for young broilers. 2. Effects on gastrointestinal tract development and nutrient digestibility. Poultry Science 98(6), 2531-2547.
| Crossref | Google Scholar |
Kheravii SK, Swick RA, Choct M, Wu S-B (2017) Coarse particle inclusion and lignocellulose-rich fiber addition in feed benefit performance and health of broiler chickens. Poultry Science 96(9), 3272-3281.
| Crossref | Google Scholar |
Leth ML, Ejby M, Workman C, Ewald DA, Pedersen SS, Sternberg C, Bahl MI, Licht TR, Aachmann FL, Westereng B, Abou Hachem M (2018) Differential bacterial capture and transport preferences facilitate co-growth on dietary xylan in the human gut. Nature Microbiology 3(5), 570-580.
| Crossref | Google Scholar |
Li M, Li C, Song S, Zhao F, Xu X, Zhou G (2016) Meat proteins had different effects on oligopeptide transporter PEPT1 in the small intestine of young rats. International Journal of Food Sciences and Nutrition 67(8), 995-1004.
| Crossref | Google Scholar |
Li B, Leblois J, Taminiau B, Schroyen M, Beckers Y, Bindelle J, Everaert N (2018) The effect of inulin and wheat bran on intestinal health and microbiota in the early life of broiler chickens. Poultry Science 97(9), 3156-3165.
| Crossref | Google Scholar |
Lin R, Liu W, Piao M, Zhu H (2017) A review of the relationship between the gut microbiota and amino acid metabolism. Amino Acids 49(12), 2083-2090.
| Crossref | Google Scholar |
Makkar HPS (2018) Review: Feed demand landscape and implications of food-not feed strategy for food security and climate change. Animal 12(8), 1744-1754.
| Crossref | Google Scholar |
Mustafa U, Naeem N, Masood S, Farooq Z (2016) Effect of bamboo powder supplementation on physicochemical and organoleptic characteristics of fortified cookies. Food Science and Technology 4(1), 7-13.
| Crossref | Google Scholar |
Nassar MK, Lyu S, Zentek J, Brockmann GA (2019) Dietary fiber content affects growth, body composition, and feed intake and their associations with a major growth locus in growing male chickens of an advanced intercross population. Livestock Science 227, 135-142.
| Crossref | Google Scholar |
Pourazadi Z, Salari S, Tabandeh MR, Abdollahi MR (2020) Effect of particle size of insoluble fibre on growth performance, apparent ileal digestibility and caecal microbial population in broiler chickens fed barley-containing diets. British Poultry Science 61(6), 734-745.
| Crossref | Google Scholar |
Rattanawut J (2014) Effects of dietary bamboo charcoal powder including bamboo vinegar liquid supplementation on growth performance, fecal microflora population and intestinal morphology in betong chickens. The Journal of Poultry Science 51(2), 165-171.
| Crossref | Google Scholar |
Rognes T, Flouri T, Nichols B, Quince C, Mahé F (2016) VSEARCH: a versatile open source tool for metagenomics. PeerJ 4, e2584.
| Crossref | Google Scholar |
Röhe I, Metzger F, Vahjen W, Brockmann GA, Zentek J (2020) Effect of feeding different levels of lignocellulose on performance, nutrient digestibility, excreta dry matter, and intestinal microbiota in slow growing broilers. Poultry Science 99(10), 5018-5026.
| Crossref | Google Scholar |
Rubinelli PM, Kim SA, Park SH, Roto SM, Nealon NJ, Ryan EP, Ricke SC (2017) Differential effects of rice bran cultivars to limit Salmonella Typhimurium in chicken cecal in vitro incubations and impact on the cecal microbiome and metabolome. PLoS ONE 12(9), e0185002.
| Crossref | Google Scholar |
Sergeant MJ, Constantinidou C, Cogan TA, Bedford MR, Penn CW, Pallen MJ (2014) Extensive microbial and functional diversity within the chicken cecal microbiome. PLoS ONE 9(3), e91941.
| Crossref | Google Scholar |
Shang Q, Wu D, Liu H, Mahfuz S, Piao X (2020) The impact of wheat bran on the morphology and physiology of the gastrointestinal tract in broiler chickens. Animals (Basel) 10(10), 1831.
| Crossref | Google Scholar |
Sozcu A (2019) Growth performance, pH value of gizzard, hepatic enzyme activity, immunologic indicators, intestinal histomorphology, and cecal microflora of broilers fed diets supplemented with processed lignocellulose. Poultry Science 98(12), 6880-6887.
| Crossref | Google Scholar |
Speroni CS, Bender ABB, Stiebe J, Ballus CA, Ávila PF, Goldbeck R, Morisso FDP, Silva LPd, Emanuelli T (2020) Granulometric fractionation and micronization: a process for increasing soluble dietary fiber content and improving technological and functional properties of olive pomace. LWT - Food Science and Technology 130, 109526.
| Crossref | Google Scholar |
Suen G, Weimer PJ, Stevenson DM, Aylward FO, Boyum J, Deneke J, Drinkwater C, Ivanova NN, Mikhailova N, Chertkov O, Goodwin LA, Currie CR, Mead D, Brumm PJ (2011) The complete genome sequence of Fibrobacter succinogenes S85 reveals a cellulolytic and metabolic specialist. PLoS ONE 6(4), e18814.
| Crossref | Google Scholar |
Sun B, Hou L, Yang Y (2021a) The development of the gut microbiota and short-chain fatty acids of layer chickens in different growth periods. Frontiers in Veterinary Science 8, 666535.
| Crossref | Google Scholar |
Sun B, Hou L, Yang Y (2021b) Effects of adding eubiotic lignocellulose on the growth performance, laying performance, gut microbiota, and short-chain fatty acids of two breeds of hens. Frontiers in Veterinary Science 8, 668003.
| Crossref | Google Scholar |
Swanson KS, de Vos WM, Martens EC, Gilbert JA, Menon RS, Soto-Vaca A, Hautvast J, Meyer PD, Borewicz K, Vaughan EE, Slavin JL (2020) Effect of fructans, prebiotics and fibres on the human gut microbiome assessed by 16S rRNA-based approaches: a review. Beneficial Microbes 11(2), 101-129.
| Crossref | Google Scholar |
Tejeda OJ, Kim WK (2020) The effects of cellulose and soybean hulls as sources of dietary fiber on the growth performance, organ growth, gut histomorphology, and nutrient digestibility of broiler chickens. Poultry Science 99(12), 6828-6836.
| Crossref | Google Scholar |
Tejeda OJ, Kim WK (2021) Effects of fiber type, particle size, and inclusion level on the growth performance, digestive organ growth, intestinal morphology, intestinal viscosity, and gene expression of broilers. Poultry Science 100(10), 101397.
| Crossref | Google Scholar |
Van Soest PJ, Robertson JB, Lewis BA (1991) Methods for dietary fiber, neutral detergent fiber, and nonstarch polysaccharides in relation to animal nutrition. Journal of Dairy Science 74(10), 3583-3597.
| Crossref | Google Scholar |
Wang L, Tan X, Wang H, Wang Q, Huang P, Li Y, Li J, Huang J, Yang H, Yin Y (2021a) Effects of varying dietary folic acid during weaning stress of piglets. Animal Nutrition 7(1), 101-110.
| Crossref | Google Scholar |
Wang J, Singh AK, Kong F, Kim WK (2021b) Effect of almond hulls as an alternative ingredient on broiler performance, nutrient digestibility, and cecal microbiota diversity. Poultry Science 100(3), 100853.
| Crossref | Google Scholar |
Wu W, Hu J, Gao H, Chen H, Fang X, Mu H, Han Y, Liu R (2020) The potential cholesterol-lowering and prebiotic effects of bamboo shoot dietary fibers and their structural characteristics. Food Chemistry 332, 127372.
| Crossref | Google Scholar |
Yokhana JS, Parkinson G, Frankel TL (2016) Effect of insoluble fiber supplementation applied at different ages on digestive organ weight and digestive enzymes of layer-strain poultry. Poultry Science 95(3), 550-559.
| Crossref | Google Scholar |
Zhai X, Lin D, Zhao Y, Li W, Yang X (2018) Effects of dietary fiber supplementation on fatty acid metabolism and intestinal microbiota diversity in C57BL/6J mice fed with a high-fat diet. Journal of Agricultural and Food Chemistry 66(48), 12706-12718.
| Crossref | Google Scholar |
Zhao G, Zhang R, Dong L, Huang F, Tang X, Wei Z, Zhang M (2018) Particle size of insoluble dietary fiber from rice bran affects its phenolic profile, bioaccessibility and functional properties. LWT - Food Science and Technology 87, 450-456.
| Crossref | Google Scholar |
Zhu Y, Huang J, Wang K, Wang B, Sun S, Lin X, Song L, Wu A, Li H (2020) Characterization of lignin structures in Phyllostachys edulis (Moso Bamboo) at different ages. Polymers (Basel) 12(1), 187.
| Crossref | Google Scholar |