Possible relationships between the physical conditions of cattle and the occurrence of structural modifications of their coat hair
Yutaka Kawahara
A
B
C
D
E
Abstract
Concerning the epithelial tissue in the skin, including hair, vitamin A is required for the integrity of epithelial cells, strengthened by compounding keratin fibres. Further, the complex hormonal changes seen in pregnancy may contribute to the hair growth. Thus, the structure of the cattle coat hair may change depending on the conditions of vitamin A deficiency or pregnancy.
The effects of several diseases, i.e. vitamin A deficiency, pneumonia, chronic pneumonia, and chronic renal failure, or pregnancy, on the structure of the coat hair were investigated.
Physical properties of the coat hair, collected from Japanese black cattle (Bos Taurus) and Holstein cows (Bos taurus Taurus), were investigated to study the effects of vitamin A deficiency, other diseases, or pregnancy on the formation of hair.
When vitamin A concentration was lowered to about half of the minimum concentration for vitamin A adequacy of 20 μg/dL in plasma (i.e. deficiency level was high), we could perceive the interference in the self-assembly of the keratin fibrils. However, we also confirmed structural modifications of the keratin fibres obtained from the cattle suffering chronic renal failure. The coat hair collected from the pregnant cows tended to show superior tensile strengths to those of the hair from the non-pregnant ones when compared at the same diameter level, although pregnancy might have increased cortisol affecting the hair follicle function. Further, from the X-ray diffraction measurements, the non-pregnant cow coat hairs, having exhibited the inferior tensile properties, were found to be structurally modified.
The structure of the coat hair could be modified when the vitamin A concentration was severely reduced (vitamin A deficiency). The physiological conditions during pregnancy may be advantageous to serve the regular formation of the hair.
Hair has a function serving as a proxy of health status for a certain period of animal life.
Keywords: cow, higher order structure, pneumonia, pregnancy, renal failure, tensile strength, vitamin A deficiency, X-ray diffraction measurement.
Introduction
Particular attention has been paid to the changes in the composition of human hair, especially in the longitudinal direction, because the human hair may have a function serving as a clinical record for a certain period of human life. Recently, cortisol concentrations in hair have attracted medical attention from the viewpoint of analysing chronic-stress history applied to humans (Cieszynski et al. 2019; Kalliokoski et al. 2019; Liu and Doan 2019; Sugaya et al. 2020, 2021). Also, in the past, some specific human hairs attracted public attention, and the hairs have been retained to understand the cause of death. For example, the hairs taken from Beethoven’s head after his death have been retained for centuries, and, quite recently, they were re-examined using scanning electron microscopy (SEM) and laser-ablation inductively coupled plasma–mass spectroscopy (ICP-MS). It was shown that the periodically fluctuating strong peaks of lead in the hair medulla along the direction of the hair length were correlating with the treatment dates on which lead-containing drugs were administered to resolve the pneumonia (Reiter and Prohaska 2021), which means that the tissue in Beethoven’s growing hairs serves as a clinical record for a certain period (i.e. during anagen phase). It is likely that Beethoven suffered a massive liver failure; a strong interaction between the lead-containing compounds and the thiol group of cysteine (Cys) will interfere with the Cys-induced enzymatic reactions (Bal et al. 2017). The anagen phase of the human hair follicle, i.e. the growth period during which the hair grows approximately 1 cm per month (Ruszczak 2012), will normally last 3–5 years (Braun-Falco et al. 2000), during which the growing hair may work as a clinical record.
Furthermore, it is well known that the growth of hair can be affected by the administration of drugs (Merk 1990). In the case of veterinary care, the coat hairs of cattle suffering visceral diseases (often induced by the restriction of vitamin A) tend to exhibit inferior physical properties (Radostits et al. 2007).
Therefore, it is acceptable to assume that hair can be regarded as a fibrous clinical record.
Compared with human hair, the growth period of cattle coat hair is limited to less than several months due to shedding, i.e. the seasonal replacement of coat (Dowling and Nay 1960). The cattle hair follicles repeat the active phase, followed by resting twice per year. Thus, the shedding occurs in March and in September. However, such a short active period may be still enough to reflect the effects of some abnormal physiological conditions of cattle on the physical properties of their growing hair, such as, for example, lowering of the tensile properties and/or crystallinity by the abnormal self-assembly of the keratin fibrils.
It is well known that the hair cycle, as well as the structure of the hair follicle, are highly affected by various hormones (Grymowicz et al. 2020); for example, hair cortisol concentration has been reported to have an impact on correct hair growth (Xiang et al. 2017). Further, the presence of cortisol at high concentrations is strictly connected to a reduction in the synthesis and premature degradation of hyaluronans and proteoglycans, which interferes with the correct growth of hair. However, low cortisol concentrations can actually bring positive effects on hair growth by slowing down the degradation of these two skin components (Thom 2016).
However, it is certain that the nutrition management is important to secure the correct growth of hair. Concerning the micronutrients to maintain the human hair follicle cycle regularly, vitamins and minerals, such as vitamins A, B, C, D and E, and iron, selenium, and zinc, are considered to be major elements in the normal hair follicle cycle, playing a role in cellular turnover, preventing the occurrence of the non-scarring alopecia disease (Almohanna et al. 2019). Furthermore, among the vitamins, it is well known that vitamin A exhibits critical roles for the vision and the immune function, and plays essential roles for cellular growth and differentiation (McDowell 2000; Institute of Medicine 2001). Therefore, when providing daily veterinary medical care to suffering cattle, the level of vitamin A in plasma is one of the most important indices in ensuring an appropriate treatment.
When fattening cattle, the restriction of vitamin A content in the feed is effective for the production of beef with marbling adipose cellularity (Gorocica-Buenfil et al. 2007a, 2007b). However, too much restriction of vitamin A to promote marbling in the beef tissue might bring about serious visceral diseases to cattle. If vitamin A deficiency syndrome occurs, for example, it may cause pneumonia to the cattle because vitamin A is important for regulating immunity in the respiratory mucosa (McGill et al. 2019). Further, the physiological stress caused by deficiency in vitamin A will be applied to the liver because the liver is the main storage site for vitamin A. On the contrary, the liver is a target organ for vitamin A toxicity, and there is a strong causal association shown by human and animal data between excess vitamin A intake and liver abnormalities (Institute of Medicine 2001).
Concerning the epithelial tissue in the skin, including hair, vitamin A is also required for the integrity of epithelial cells strengthened by compounding keratin fibres (Gudas et al. 1994). Keratin fibres in the skin and hair are the resultant structures fabricated through the continuously and closely related biological reactions, sustaining the self-assembly of protein segments (e.g. the protofilaments predominantly consist of a coiled-coil structure of 45–50 nm in length, and their N- and C-terminal ends of ~3 nm in length are forming a non-helical globular structure) (Aebi et al. 1983; Alberts et al. 1989; Coulombe and Fuchs 1990; Katagata 1993; Popescu and Höcker 2007). Therefore, when a certain disorder occurs in those reactions, the resultant keratin fibre may contain structural defects in the crystal morphology, which may be detected by X-ray diffraction measurements.
Also, we sometimes perceive a slight difference in the appearance of hair of the pregnant women in our daily life. However, few reports have dealt with the effects of pregnancy on the growth of hair. The scalp hair data obtained from the pregnant women indicated that the hair diameter increases towards the scalp were found to start at the beginning of pregnancy (Nissimov and Elchalal 2003). In the case of cow coat hair, the mean hair follicle diameter of 108.8 ± 5.9 μm at 28 days before the parturition decreased to 95.5 ± 5.5 μm at 28 days after the parturition, and further it increased to 104.2 ± 5.3 μm at 56 days postpartum (Suzuki et al. 2018). Furthermore, it was confirmed that there was a significant correlation between the hair follicle width and the percentage of anagen follicles. That is, the percentage of anagen follicles changed during the peripartum period similarly as in the case of the hair follicle width (Suzuki et al. 2018). These phenomena may be related to the changes in blood oestrogen concentrations (Nissimov and Elchalal 2003; Shah et al. 2006; Sumiyoshi et al. 2014; Grymowicz et al. 2020).
However, there is another possibility that the decrease in the percentage of anagen follicles may be induced by the increase of prolactin (PRL, one of the prototypic pituitary hormones) concentration in plasma. It was confirmed that PRL could prematurely induce a catagen-like stage by using the organ-cultured human hair follicles, although the applied dose reached 400 ng/mL (Foitzik et al. 2006). PRL concentrations in normal pregnant women increased from 6 ng/mL during early pregnancy to 210 ng/mL near term to prepare the lactation after the parturition (Biswas and Rodeck 1976). Further, PRL concentrations in the women suffering hair loss and hyperprolactinemia were found to be below 50 ng/mL (Lutz 2012). Therefore, it is unlikely that PRL can induce a catagen-like stage independently in the hair follicles without any other hormone interactions in vivo. However, to our knowledge, there is no data dealing with the effects of pregnancy on the physical properties of hair.
In this paper, we first investigated the seasonal changes in the physical properties of healthy cattle coat hair, such as fibre diameter, tensile behaviour, and moisture-regaining property. Then, the effects of the several serious diseases, i.e. vitamin A deficiency, pneumonia, chronic pneumonia, and chronic renal failure, on the structural modifications of cattle coat hair were investigated, focusing on the concentrations of vitamin A in plasma. Furthermore, we also investigated the effects of physiological conditions during pregnancy on the physical properties of cow coat hair. We confirmed that the coat hair samples tended to show lower tensile properties when the concentrations of vitamin A in plasma were significantly reduced. On the contrary, the coat hair samples obtained from the pregnant cows tended to show tensile strengths superior to those of the hair samples from the non-pregnant ones when compared at the same diameter level.
Materials and methods
The study protocol and all procedures were conducted in accordance with the guidelines of the Ethics Committee for the Care and Use of Laboratory Animals for Research of Gunma University, Japan. The approval number was 21-059.
Material
The fresh hair sample of 150–250 mg was pulled out of the upper skin of the rib portion of Japanese black cattle (Bos Taurus) or Holstein (Bos taurus Taurus). To illustrate the phylogenetic relationship among Japanese black cattle, indicine cattle (Bos indicus) and Holstein, the numerical taxonomy was applied, and it was confirmed that the gene composition of Japanese black cattle was similar to that of Holstein (Namikawa 1972). The hair coat of the cattle is a double coat consisting of the underlayer coat and the top coat, similar to the other animal coats (Dowling and Nay 1960). The shedding of coat hair, observed between winter and spring, and between summer and autumn, are due to a replacement of the underlayer coat hair. Normally, the former change appears drastically, while the latter occurs imperceptibly, probably due to the short length of summer coat hair. On the contrary, the top coat hair follicles keep their biological activities independent of the seasonal changes, although the amount of the top coat hair follicles is smaller than the amount of the underlayer coat hair ones. Since it was difficult to separate the top coat hairs from the collected samples, it should be noted that the hair sample prepared in this study might contain a small amount of the top coat hair. The physical conditions of the cattle and cows, when the hair samples had been collected, are shown in Tables 1 and 2. The pregnant or non-pregnant periods for the cows at the time of hair sampling are also listed in Table 2.
Animal number | Breed | Birthday | Sex | Condition | Sampling date | Tensile properties of coat hair | |||
---|---|---|---|---|---|---|---|---|---|
Diameter (μm) | Strength (MPa) | Elongation at break (%) | |||||||
6 | Bos Taurus | 25/5/2014 | Female | Healthy | 10/5/2015 | 93.5 ± 10.8 | 85.8 ± 24.2 | 54.2 ± 11.9 | |
7 | Bos Taurus | 30/9/2012 | Female | Vitamin A deficiency 27.0 μg/dL | 18/5/2015 | ||||
9 | Bos Taurus | 29/6/2013 | Female | Vitamin A deficiency 11.1 μg/dL NEFA 295 μmol/L | 19/5/2015 | 86.1 ± 22.0 | 128.5 ± 51.8 | 85.5 ± 13.7 | |
12 | Bos Taurus | 3/9/2012 | Female | Vitamin A deficiency 13.2 μg/dL NEFA 668 μmol/L | 27/5/2015 | 88.3 ± 17.7 | 147.9 ± 36.9 | 72.2 ± 10.5 | |
226 | Bos Taurus | 23/12/2018 | Castrated male | Pneumonia Vitamin A 23.4 μg/dL | 16/6/2020 | 90.3 ± 12.5 | 103.4 ± 15.7 | 40.9 ± 7.3 | |
344 | Bos Taurus | 1/4/2019 | Castrated male | Pneumonia Vitamin A 5.7 μg/dL | 16/6/2020 | 73.5 ± 12.3 | 102.3 ± 29.0 | 46.1 ± 6.5 | |
525 | Bos Taurus | 21/3/2019 | Female | Healthy | 25/2/2020 24/5/2020 20/8/2020 25/11/2020 | 71.9 ± 20.2 100.3 ± 8.8 101.7 ± 12.2 98.1 ± 7.8 | 134.9 ± 48.0 107.6 ± 11.5 124.9 ± 19.3 138.3 ± 19.9 | 53.7 ± 7.3 49.5 ± 5.2 40.5 ± 11.8 46.8 ± 9.4 | |
526 | Bos Taurus | 3/3/2019 | Female | Healthy | 25/2/2020 24/5/2020 27/8/2020 25/11/2020 | ||||
529 | Bos Taurus | 27/3/2019 | Female | Healthy | 25/2/2020 24/5/2020 20/8/2020 3/12/2020 | ||||
530 | Bos Taurus | 1/4/2019 | Female | Healthy | 25/2/2020 24/5/2020 19/8/2020 3/12/2020 | ||||
536 | Bos Taurus | 4/4/2019 | Female | Healthy | 25/2/2020 28/5/2020 27/8/2020 26/11/2020 | 93.1 ± 12.1 88.1 ± 17.4 107.2 ± 8.3 94.6 ± 8.0 | 135.3 ± 38.7 111.0 ± 35.8 111.5 ± 15.7 128.9 ± 16.1 | 41.2 ± 4.0 40.5 ± 8.4 35.3 ± 8.9 49.4 ± 7.0 | |
601 | Bos Taurus | 1/12/2015 | Female | Pneumonia Vitamin A 2.1 μg/dL | 16/10/2017 | ||||
1245 | Bos Taurus | 21/11/2015 | Female | Chronic pneumonia | 16/2/2017 | 67.9 ± 16.2 | 156.5 ± 48.7 | 57.6 ± 12.1 | |
1679 | Bos Taurus | 31/12/2016 | Female | Healthy | 27/10/2017 | 55.0 ± 17.6 | 212.7 ± 79.6 | 66.1 ± 4.3 | |
1831 | Bos Taurus | 20/7/2016 | Female | Chronic renal failure Vitamin A 41.0 μg/dL CRE 4.5 mg/dL | 22/5/2018 | 121.2 ± 15.3 | 81.4 ± 27.8 | 71.2 ± 16.5 | |
8430 | Bos Taurus | 4/12/2014 | Female | Pneumonia Vitamin A 9.0 μg/dL | 2/2/2017 |
NEFA, non-esterified fatty acid concentration in plasma; CRE, creatinine concentration in plasma.
Cow number | Breed | Birthday | Preg./non-preg. | Duration (days) | Sampling date | Tensile properties of coat hair | |||
---|---|---|---|---|---|---|---|---|---|
Diameter (μm) | Strength (MPa) | Elongation at break (%) | |||||||
455 | B. taurus Taurus | 6/11/2007 | Preg. | 55 | 5/4/2019 | ||||
1152 | B. taurus Taurus | 29/1/2015 | Non-preg. | 155 | 17/4/2019 | 72.1 ± 12.0 | 122.3 ± 42.2 | 74.8 ± 10.3 | |
4140 | B. taurus Taurus | 15/3/2013 | Preg. | 49 | 5/4/2019 | 101.9 ± 6.7 | 133.7 ± 19.8 | 54.8 ± 8.3 | |
7330 | B. taurus Taurus | 24/11/2014 | Preg. | 139 | 28/4/2021 | 95.0 ± 10.8 | 138.4 ± 36.0 | 65.8 ± 12.4 | |
7353 | B. taurus Taurus | 21/10/2015 | Non-preg. | 295 | 28/4/2021 | 94.6 ± 9.3 | 95.3 ± 13.4 | 57.4 ± 13.0 | |
7379 | B. taurus Taurus | 26/11/2015 | Non-preg. | 334 | 28/4/2021 | 97.6 ± 13.1 | 122.8 ± 19.8 | 56.8 ± 13.7 | |
7410 | B. taurus Taurus | 20/12/2017 | Preg. | 86 | 28/4/2021 | 109.4 ± 6.9 | 92.5 ± 10.2 | 56.9 ± 8.6 | |
7518 | B. taurus Taurus | 16/12/2016 | Preg. | 36 | 3/4/2019 | 106.1 ± 13.5 | 102.4 ± 17.8 | 58.8 ± 11.3 | |
7616 | B. taurus Taurus | 7/5/2013 | Non-preg. | 98 | 17/4/2019 | 67.7 ± 8.6 | 116.2 ± 26.9 | 43.0 ± 4.6 | |
8467 | B. taurus Taurus | 4/2/2010 | Preg. | 52 | 5/4/2019 | 105.8 ± 9.3 | 115.4 ± 18.5 | 84.1 ± 8.6 | |
8520 | B. taurus Taurus | 13/12/2010 | Non-preg. | 316 | 17/4/2019 | 88.7 ± 11.0 | 100.1 ± 23.3 | 51.7 ± 10.8 | |
8859 | B. taurus Taurus | 1/4/2006 | Preg. | 55 | 17/4/2019 | ||||
19 867 | B. taurus Taurus | 19/4/2014 | Non-preg. | 125 | 2/4/2019 | 101.8 ± 10.7 | 91.0 ± 16.7 | 72.9 ± 13.5 |
The collected hair samples were rinsed twice in a neutral detergent aqueous solution of 0.1% (NOIGEN®, DKS Co. Ltd, Kyoto, Japan) at room temperature, followed by mildly rinsing in water, then the samples were dried in the room air-conditioned at 20°C and 65%RH for several days. Then the rinsed hair samples were stored in the freezer set at −20°C, until further measurements.
Measurements
The dependence of the moisture-regain of coat hair on the ambient humidity was measured using a temperature and humidity control apparatus TH401HA (Kusumoto Chemicals, Ltd, Tokyo, Japan).
An amino acid analysis was performed using a HPLC system (JASCO Co., Hachioji, Japan). Prior to the analysis, the samples were hydrolysed via heating them in 6-N hydrochloric acid at 110°C for 24 h. During the hydrolysis treatment, tryptophan was destroyed. However, the destruction of tryptophan would hardly affect the amino acid composition of coat hair; in the case of Merino sheep hair, its tryptophan concentration was negligibly small enough to be ignored, i.e. 40 μmol/g (Popescu and Höcker 2007).
The wide-angle X-ray diffraction (WAXD) images were obtained using an R-Axis Rapid I X-ray diffractometer (Rigaku Denki Co., Akishima, Japan) operated at 40 kV and 30 mA and equipped with a cylindrical imaging plate camera. The monochromatised CuKα radiation beam, collimated by a 0.3 mm pinhole collimator, penetrated perpendicular to the fibre axis of the bundle of the hair sample inserted in a crystal capillary tube with an inner diameter of 0.5 mm. Thus, the volumes of the samples irradiated by the X-rays could be kept approximately constant. After the radiation, the background scattering by the tube was subtracted from the diffraction image. Each sample was radiated for 5 h to obtain its WAXD image. The hair sample gave a fibre diagram. In this case, the conversions to one dimensional equatorial WAXD intensity curves, I(2θ), were made by integrating the WAXD intensity I(ϕ) at 2θ along the azimuthal angle, ϕ, covering the specific diffraction peaks on the equator and repeating the similar integration stepwise with increments of Δ2θ = 0.2° in the range of 2θ = 1.8–42.0°.
Surface element analysis was performed on the cross-section of the cortex tissue of coat hair semi-quantitatively by energy-disperse X-ray spectroscopy (EDS) with a scanning electron microscope SU3500 (Hitachi High-Technologies Co., Tokyo, Japan) equipped with a prime EDS (AMETEK, Co., Ltd, Tokyo, Japan).
The coat hair filament was embedded in an ultraviolet (UV)-curable resin (LCR-D-800, Toagosei Co., Ltd, Tokyo, Japan) and subjected to the UV radiation for 5–10 min by using a LUXSPOT (Toagosei Co., Ltd), and then the sections of the hairs were obtained by cutting the hardened resin with steel knives on a HM-360 microtome (Carl Zeiss, Oberkochen, Germany). The sections were coated with Pt prior to the SEM observation.
The tensile properties were measured using a tensile tester STA-1150 (A&D Co., Ltd, Tokyo, Japan) with a cross-head speed set at 100% elongation per minute in the room air-conditioned at 20°C and 65%RH. Gauge length was set at 5–20 mm. Tensile strength was determined by averaging the maximum strength of 20 measurements. The blanks in the tensile data in Tables 1 and 2 mean that, in most cases, the sample hairs were too short to be measured correctly.
Results and discussion
Seasonal changes in the physical properties of cattle coat hair
It is well known that shedding of cattle coat hair occurs twice per year (Dowling and Nay 1960). Concerning the seasonally dependent cycles of coat replacements, the role of PRL in the hair-growth regulation has been comprehensively studied and has proved the fact that PRL can stimulate the hair growth, molting, and shedding in sheep and mink (Duncan and Goldman 1984; Martinet et al. 1984; Rougeot et al. 1984; Craven et al. 2001; Ignacak et al. 2012). However, there were contradictory data, indicating that the effects of PRL on hair shedding in geldings were minor, even though PRL concentrations were increased by sulpiride (Thompson and DePew 1997). The shedding seems to be regulated not only by PRL. Other hormone interactions should be considered to explain the shedding cycle.
This section deals with the seasonal changes in the physical properties of cattle coat hair by using the hair samples obtained from the healthy cattle. Fig. 1a, b shows the appearance of the surface of the coat hair (unwashed coat hair sample was used for the SEM observation). The whole length of the summer coat hair was not more than 30 mm, while that of the winter coat hair was a little longer, but did not exceed 50 mm. The adjacent cuticle cells appeared to be more densely overlapped in the summer coat hair. Fig. 1c, d shows the appearance of the tip of the coat hair. In most cases, the tip of the winter coat hair was covered with the lipid-like materials. On the contrary, as for the summer coat hair, in most cases, the fibrous cortex tissues were partially exposed to the air and, furthermore, the tip of summer coat hair was opened to the air like an exhaust port, which may be effective for discharging water vapour.
Appearance of typical side-surface. (a) Winter coat hair collected in February from animal Number 525, (b) summer coat hair collected in August from animal Number 526. The tip part for the coat hair collected from animal Number 525: (c) winter coat collected in February, and (d) summer coat collected in August.
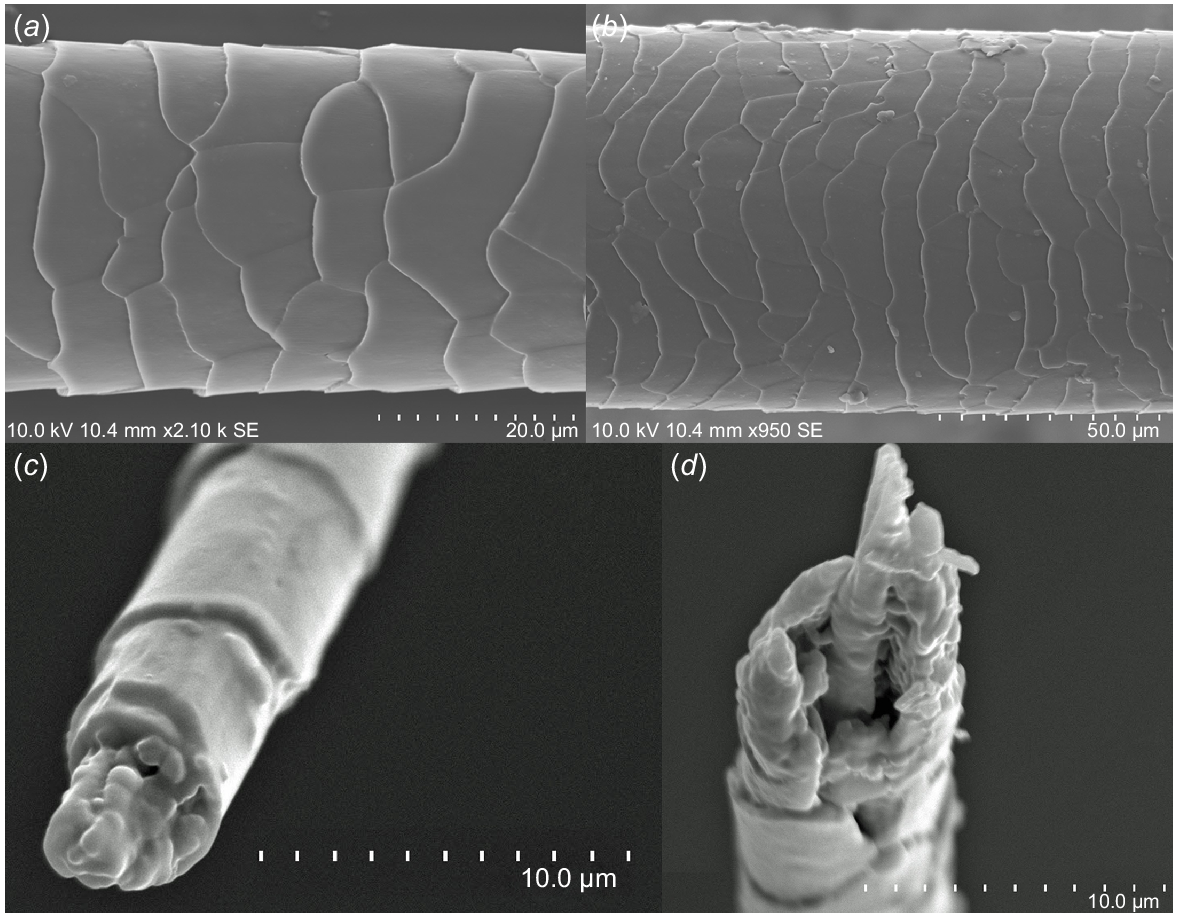
To control the vital warmth of cattle properly, especially in summer, the vaporisation of water from the skin is of great importance. The coat hair with larger specific surface area will be useful for the vaporisation of water effectively. To secure the vaporisation of water successfully from the surface of the coat hair, the constant migration of water throughout the hair tissue is necessary. For this purpose, in the case of wool or human hair, it was found that the central delta layer with a thickness of 15–18 nm of the continuously layer-structured cell-membrane complex (CMC), joining the whole adjacent cortex and cuticle cells, can work as a water channel, although the total content of CMC in the hair is no more than 3 wt% (Ohta et al. 2005; Inoue et al. 2007; Robbins 2009). The more dense overlapping of the cuticle cells in the case of the summer coat hair will increase the area of CMC (filling the interspace between the adjacent cuticle cells) exposed to the ambient air, which is effective for the vaporisation of water from the surface of hair. In addition to the function of the delta layer in CMC, a small duct on top of the summer coat hair is also probably related to the vaporisation of water (see Fig. 1d).
Fig. 2a shows the cross-section of the middle part (a little close to the hair bulb) of the typical winter coat hair. The central part of the hair shaft was medullated, and the medulla tissue was surrounded by the cortex cells. Fig. 2b shows the cross-section of the cortex tissue. The border of the cortex cell appeared to be a little complicated, although the border was partially not so clearly seen. The melanin pigment granule cells were embedded among the cortex cells. Fig. 2c shows the cuticle cell layer. It appears that two cuticle cell layers were covering the cortex tissue.
Cross-section of the winter coat hair collected in October from animal Number 1679: (a) whole shape, (b) cortex tissue portion (c) peripheral portion of the cross-section. M, melanin pigment granules; Me, medulla; Co, cortex tissue; Cu, cuticle layer. The borders of some cortex cells were traced by the broken white line.
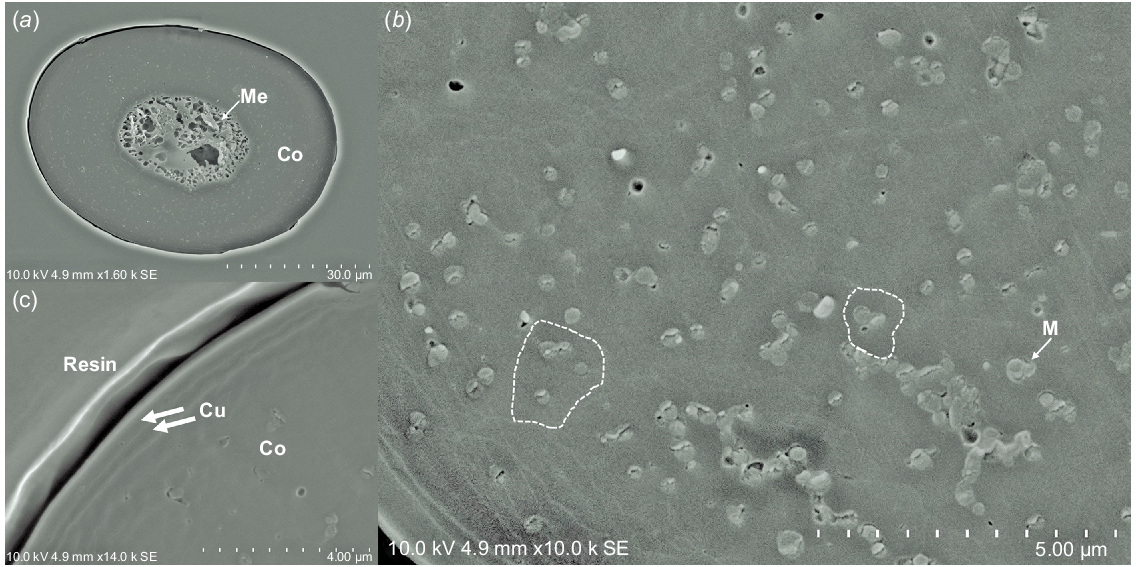
We tried to cut the summer coat hair to obtain its cross-section similarly, by using the microtome apparatus. However, we could not obtain a clear cross-section suitable for SEM observation without artifact, i.e. knife marks. Compared with the cortex tissue, the medulla tissue is much more porous and, therefore, the cross-section of the coat hair fibre would easily be collapsed by the cutting shear force if the strength of the outside cortex tissue were not strong enough (as discussed later), the winter coat hair tended to show superior tensile strengths to those of the summer coat hair, which was advantageous to prepare the cross-section from the winter coat hair). It should be noted that the amorphous medulla tissue was stabilised by the formation of isopeptide bonding rather than the cross-linking between Cys side chains (Swift 1977).
Fig. 3 shows the seasonal changes in the amount of sulfur content in the cortex tissue estimated by the surface-element analysis by using an EDS. A remarkable seasonal dependence was recognised between August and November/December, especially for animal Number 525 and Number 526 with significance (P < 0.05). This drastic change was definitely correlated with shedding. It should be noted that the major sulfur-containing amino acids in the hair are Cys and methionine (Met) (Popescu and Höcker 2007). In addition, the amino acid analysis data in Table 3 suggest that the Cys content changed, hardly depending on the seasons, but on the individual hair structure. Furthermore, Met content was almost unchanged (see Table 3). We suggest that the marked increase in the sulfur content in the summer coat hair in Fig. 3 did not merely imply the change in the sulfur-containing amino acid content. We should focus on the fact that the packing of the cortex cells will affect the penetration depth of the electron in the EDS measurement. A lower packing density of the cortex cells may allow deeper penetration of the electron, which results in a larger content of sulfur detected by the EDS measurement. Therefore, it was thought that the cortex cells of the summer coat hair were more loosely connected than were those of the winter coat hair.
Seasonal changes in the amount of sulfur (S) in the cortex tissue estimated by the EDS measurements. Asterisks indicate difference (P < 0.05) in S content between August and November/December.
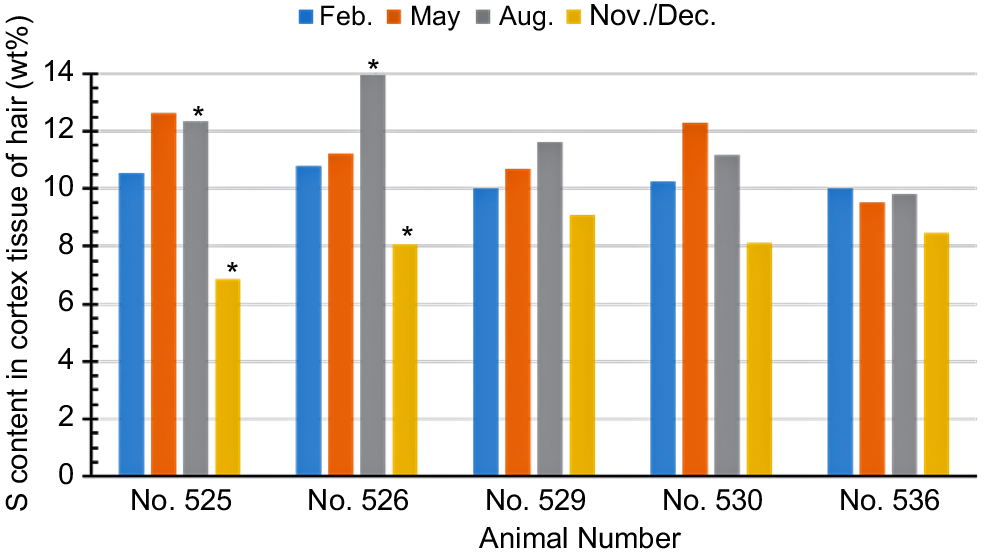
Animal Number and Sampling Date | 525 25 February 2020 | 525 20 August 2020 | 6 10 May 2015 | 9 19 May 2015 | 1831 22 May 2018 | |
---|---|---|---|---|---|---|
Condition | Healthy | Healthy | Healthy | Vit. A deficiency | Chronic renal failure | |
Amino acid | wt% | wt% | wt% | wt% | wt% | |
Asp | 5.4 | 5.8 | 5.2 | 4.9 | 5.4 | |
Thr | 4.9 | 5.5 | 4.9 | 5.2 | 4.3 | |
Ser | 8.0 | 7.8 | 6.8 | 7.0 | 8.0 | |
Glu | 11.5 | 13.7 | 13.6 | 11.2 | 11.9 | |
Pro | 5.8 | 5.9 | 5.6 | 6.0 | 5.7 | |
Gly | 4.3 | 3.7 | 3.5 | 3.8 | 4.1 | |
Ala | 3.3 | 3.3 | 2.6 | 2.6 | 2.4 | |
Cys | 6.8 | 7.8 | 7.7 | 9.7 | 6.3 | |
Val | 2.9 | 4.2 | 4.0 | 4.1 | 2.6 | |
Met | 0.3 | 0.4 | 0.4 | 0.4 | 0.4 | |
Ile | 1.7 | 2.7 | 2.6 | 2.5 | 1.2 | |
Leu | 5.9 | 6.9 | 6.1 | 5.5 | 5.3 | |
Tyr | 5.3 | 5.0 | 3.8 | 3.7 | 3.7 | |
Phe | 2.2 | 2.5 | 2.3 | 2.0 | 2.2 | |
His | 1.2 | 1.0 | 0.9 | 0.9 | 2.2 | |
Lys | 2.5 | 3.1 | 3.0 | 2.5 | 2.3 | |
Arg | 7.4 | 8.4 | 7.9 | 7.5 | 8.0 | |
Total | 79.4 | 87.7 | 80.9 | 79.5 | 76.0 |
Fig. 4 shows the seasonal changes in the tensile strength and the diameter of the coat hair. Compared with the summer coat hair, the winter coat hair exhibits superior tensile properties. Especially some thinner winter coat hairs exhibit excellent performances in strength. These results understandable considered the sulfur content data obtained from the EDS measurements, implying the densely packing of cortex cells of the winter coat hair. When the tensile data obtained in May were compared between animals Number 525 and Number 536, the data for the latter were more widely scattered, while the data for the former were concentrated in a certain area. It seems that the animal Number 525 had already finished shedding when the sample was collected in May.
Seasonal changes in the tensile strength and the corresponding diameter of the coat hairs: top, animal Number 525; bottom, animal Number 536.
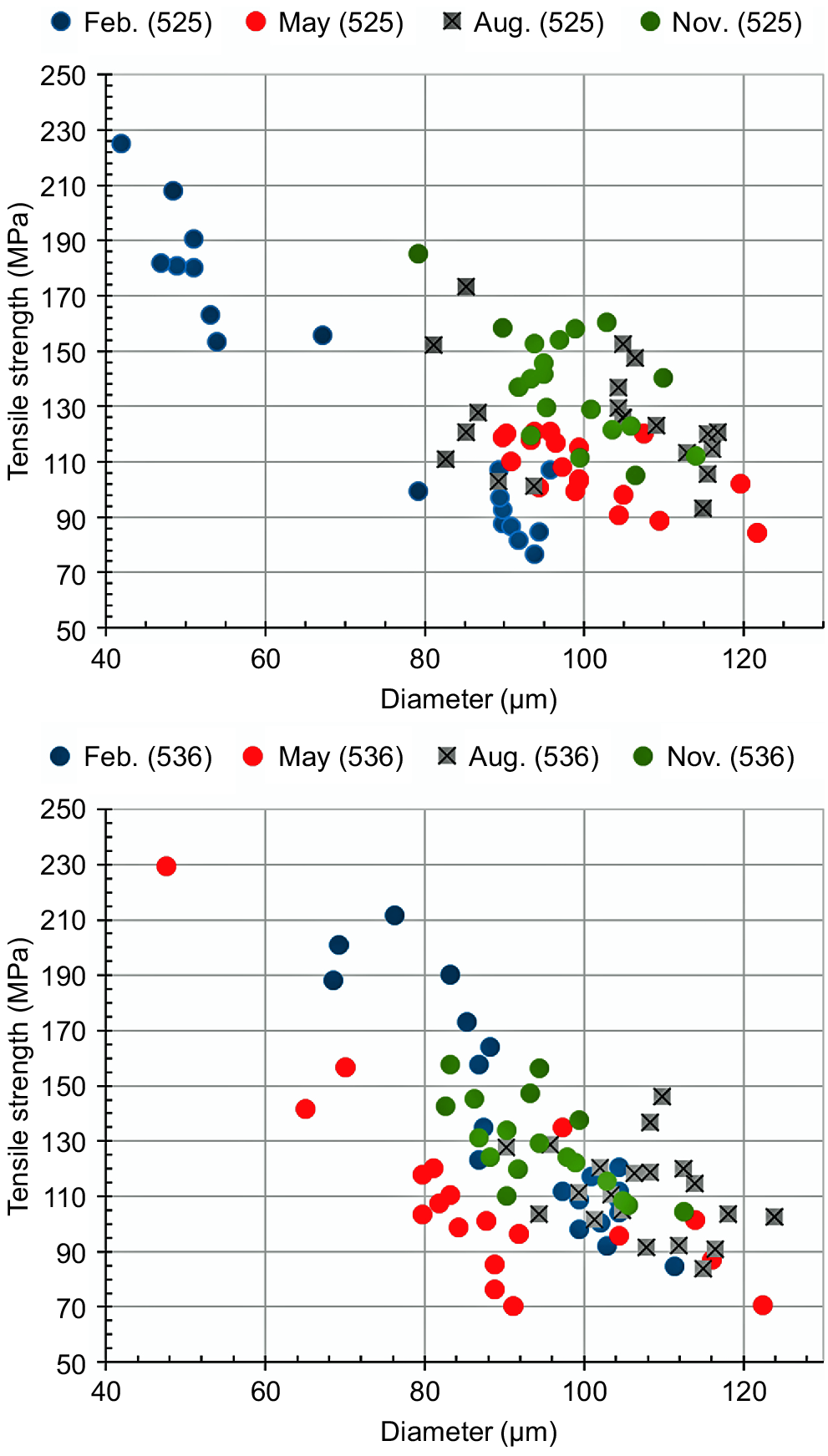
Fig. 5 shows the WAXD patterns and the corresponding equatorial WAXD intensity curves for the coat hair. The coat hair showed similar WAXD patterns with two equatorial peaks at 2θ = 3° and 2θ = 9°, and a halo of diffuse scattering around 2θ = 20°, although they were collected in different seasons. A peak at 2θ = 3° is attributable to the ordered structure of the proto-filament comprising the two coiled-coils of the dimers with the thickness of 3 nm (Aebi et al. 1983; Alberts et al. 1989; Katagata 1993). A peak at 2θ = 9° is attributable to the interspace distance of 0.98 nm between the chains constructing the dimer having the stranded left-handed coiled-coil structure (Popescu and Höcker 2007). A diffuse halo around 2θ = 20° is attributable to the X-ray scattering from the non-crystalline portions of the hair, such as the matrix portion of the cortex cell, cuticle layer, medulla, and CMC. The WAXD measurements demonstrated the ordered arrangement in the coiled-coil structure of the proto-filament embedded in the cortex tissue, regardless of the seasonal changes. It was likely that the keratinisation process in each coat hair follicle was fully regulated even after the shedding cycle, as long as the cattle were healthy.
WAXD patterns (hair shaft axis is vertical) and the corresponding equatorial WAXD intensity curves for the coat hair collected in February and August from animal Number 536.
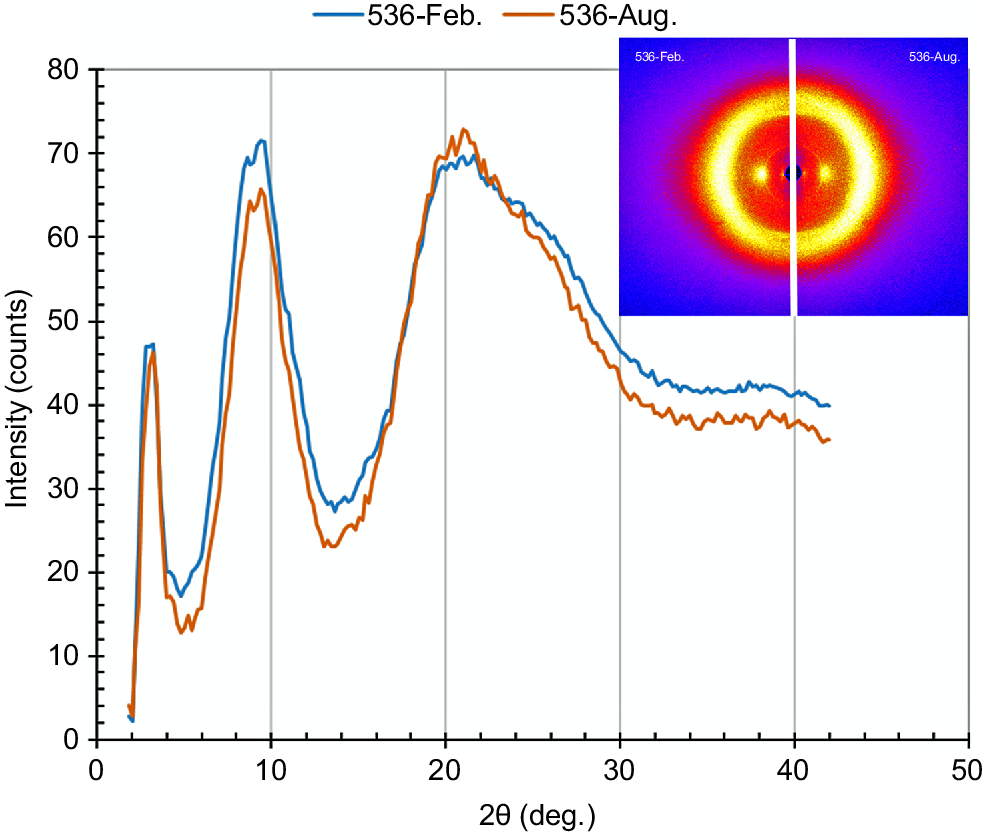
An alternative factor, affecting the tensile behaviour of the coat hair, is the occurrence of cross-linking between the adjacent molecular chains. In addition to the hydrophilicity of the tissue, the moisture-regaining property of the tissue will depend on the density of effective cross-links between the adjacent molecular chains, suppressing the volume increase of the cortex tissue with adsorption of water (Arai et al. 1996; Naito et al. 1996). Fig. 6 shows the moisture-regaining properties of the coat hair samples collected from the healthy cattle (Numbers 525, 526, 529, 530, 536). So as to characterise the slight difference in the individual cortex tissue structures, the abscissa axis represents the sulfur content on the cross-sectional surface of each hair sample detected by the EDS measurements; the side chains of cysteine tends to be cross-linked with each other, which will affect the stiffness of the resultant individual cortex tissues. Therefore, electron beam excitation behaviour of sulfur in the tissue may differ depending on the stiffness of the individual cortex tissues. With an increasing ambient air humidity, the difference in the moisture-regain between the coat hair samples collected in August and February/May became significantly (P < 0.01) larger (most of the cattle in May were probably still wearing the winter coat hair when the hair sample had been collected). The larger moisture-regain of the summer coat hair samples may imply the poor cross-linking between the adjacent molecular chains.
Dependence of moisture-regain of the coat hair collected in February/May or August on the ambient air humidity. To characterise the slight difference in the individual cortex tissue structures, the abscissa axis represents the sulfur content of each hair sample estimated by the EDS measurements. Asterisks indicate a significant (P < 0.01) difference in moisture-regain between February/May and August
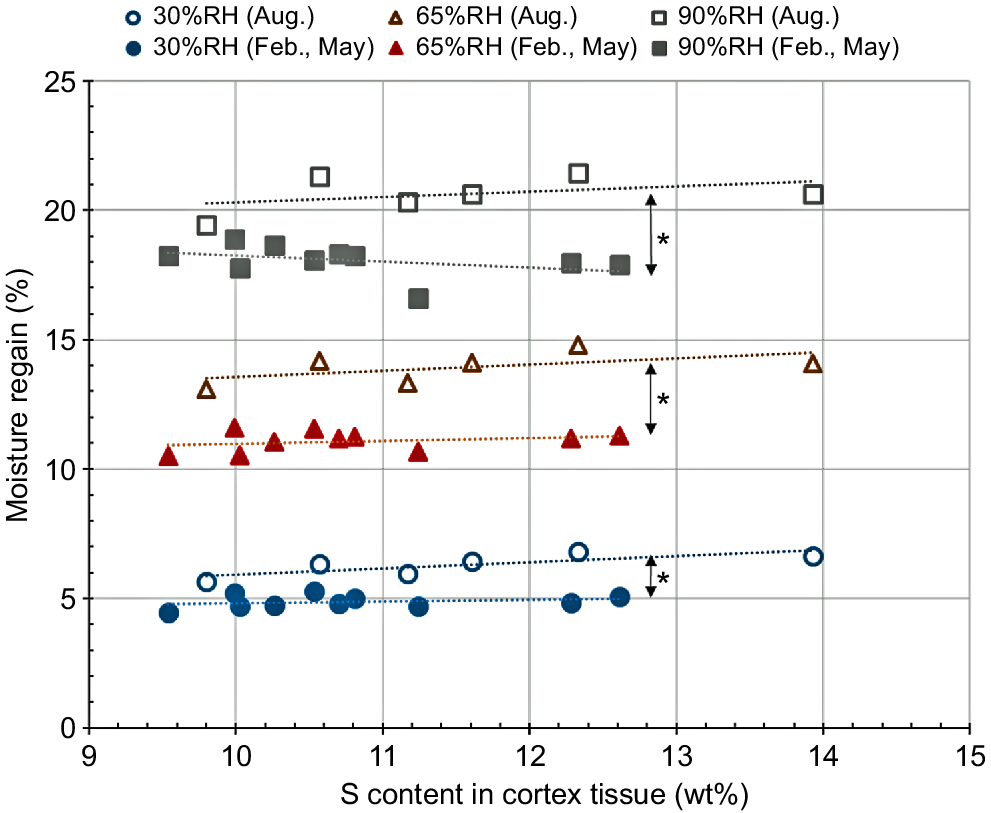
We investigated the changes in the amino acid composition, focusing on the contents of cross-linking Cys and Met (see Table 3). The acid-soluble proteins, composed of a low-sulfur protein fraction, are usually dealt with in the amino acid analysis. The solutions prepared from low-sulfur proteins are called α-keratoses (Kidd 1977). The low solubility against the acid, often observed in the hydrolysis of the high-sulfur protein fraction, is ascribed to the densely formed covalent bonds between Cys side chains. In the case of human hair, Cys content in the cuticle tissue is about 1.4 times larger than that in the cortex tissue (Popescu and Höcker 2007). Thus, the large amount of insoluble residues in Table 3, sometimes exceeding 20 wt%, was mainly due to the hydrolysate of the cuticle tissue.
In Table 3, except for the glutamic acid (Glu) content, the contents of the other amino acids were almost similar regardless of the seasonal effects. They were slightly different depending on the individual cortex structures. However, the decrease in the Glu content, observed in the winter coat hair, may imply the occurrence of the cross-linking induced by the transglutaminase (TGase). It is well known that TGase can interact with the side chain of glutamine (Gln) with its thiol group, and the TGase-interacted Gln will react with the side chain of lysine (Lys) or with NH2-terminus to form the covalent bonding (Glu content in Table 3 was the sum of Glu and Gln contents). The content of Lys in Table 3 was almost unchanged. Thus, the latter-type cross-linking reactions might occur in the keratinisation process in the hair follicles. Among TGases, TGase-3 was found to work in the hair follicles (Steinert et al. 1996; Kalinin et al. 2002; Hitomi et al. 2003; Hitomi 2005). However, unfortunately, the coat hair tissues were so densely connected that we failed in extracting TGase directly. Nevertheless, the data (i.e. the higher tensile strengths, the lower moisture regain, and the smaller Glu content) obtained from the winter coat hair suggest a possibility that the TGase-induced cross-linking might occur preferentially in the formation of the winter coat hair.
Effects of vitamin A deficiency and other diseases
Vitamin A is essential for the regeneration of the visual purple necessary for dim-light vision, for normal bone growth and for maintenance of normal epithelial tissues (covering all body surfaces, lining body cavities and hollow organ surfaces) (Radostits et al. 2007). Therefore, vitamin A deficiency will induce many clinical diseases, not only in the skin, including hair, but also in visceral organs of cattle, such as, for example, pneumonia (see Table 1). A rough, dry coat with a shaggy appearance and heavy deposits of bran-like scales on the hair and skin are the typical features for the cattle suffering vitamin A deficiency (Radostits et al. 2007). The hair sample of animal Number 9 in Table 1 showed such appearance. Animal Number 12 showed a vitamin A concentration similar to that of animal Number 9. However, the appearance of its coat hair was not so shaggy. Further, both animals Number 9 and Number 12 showed high non-esterified fatty acid (NEFA) concentrations (plasma concentrations of ~200 μmol/L are the normal concentration for NEFA). Animal Number 7 was at an initial stage of vitamin A deficiency (recovered after the administration of vitamin A at 250 kU). Animal Number 1831 showed some peculiar features; i.e. a normal vitamin A concentration was detected, whereas a high creatinine (CRE) value was recorded, reflecting chronic renal failure. Plasma concentrations of 20 μg/dL are the minimal concentration for vitamin A adequacy (Radostits et al. 2007). Vitamin A concentrations for the cattle suffering pneumonia decreased to less than 10 μg/dL in plasma, except for animal Number 226 treated with administrating vitamin A preparation until about 1 month before the blood test. Animal Number 1245 had been suffering pneumonia for 125 days, and had gotten the treatment 30 times, including the administration of vitamin preparations, before the collection of its hair sample. Thus, unfortunately, the exact vitamin A concentration was masked in the case of animal Number 1245.
Fig. 7a shows the surface of the coat hair collected from animal Number 12. Adhesion of small scale-like fragments was still recognised on the surface, even after the sample was washed twice before the SEM observation. Deposits of bran-like scales on the hair are a typical feature for the cattle suffering vitamin A deficiency (Radostits et al. 2007). It should be noted such adhesion was hardly observed in the case of the healthy cattle hair (see Fig. 1). Further, the central medulla tissue had been broken at several positions (indicated by arrows in Fig. 7b). These breakages may be artifacts generated by the pulling stress applied when the sample hair was collected. It was likely that the amorphous medulla tissue of animal Number 12 hair shaft was too weak to withstand the pulling stress. Fig. 7c shows the cross-section of the cortex tissue prepared from animal Number 1831, which was suffering chronic renal failure. Lots of pits were observed in the cross-section. Those pits were considered to be generated by falling down of the melanin pigment granule cells through the stress applied by the microtome knife edge at the preparation of the cross-section sample, which may also suggest the weakened connections between the cortex cells and melanin pigment cells. Thus, we suggest that the interference in the normal connections between the cortex cells and melanin pigment cells during the keratinisation process in the hair follicles was caused by the long-lasting depressed physical conditions, i.e. chronic renal failure.
Surface morphology and optical microscopic image of the coat hair collected from animal Number 12: (a) small scale-like fragments observed in the encircled area of the cuticle surface, (b) breakage of the medulla tissue indicated by arrows. (c) Cross-section of the cortex tissue of the coat hair collected from animal Number 1831, suffering chronic renal failure.
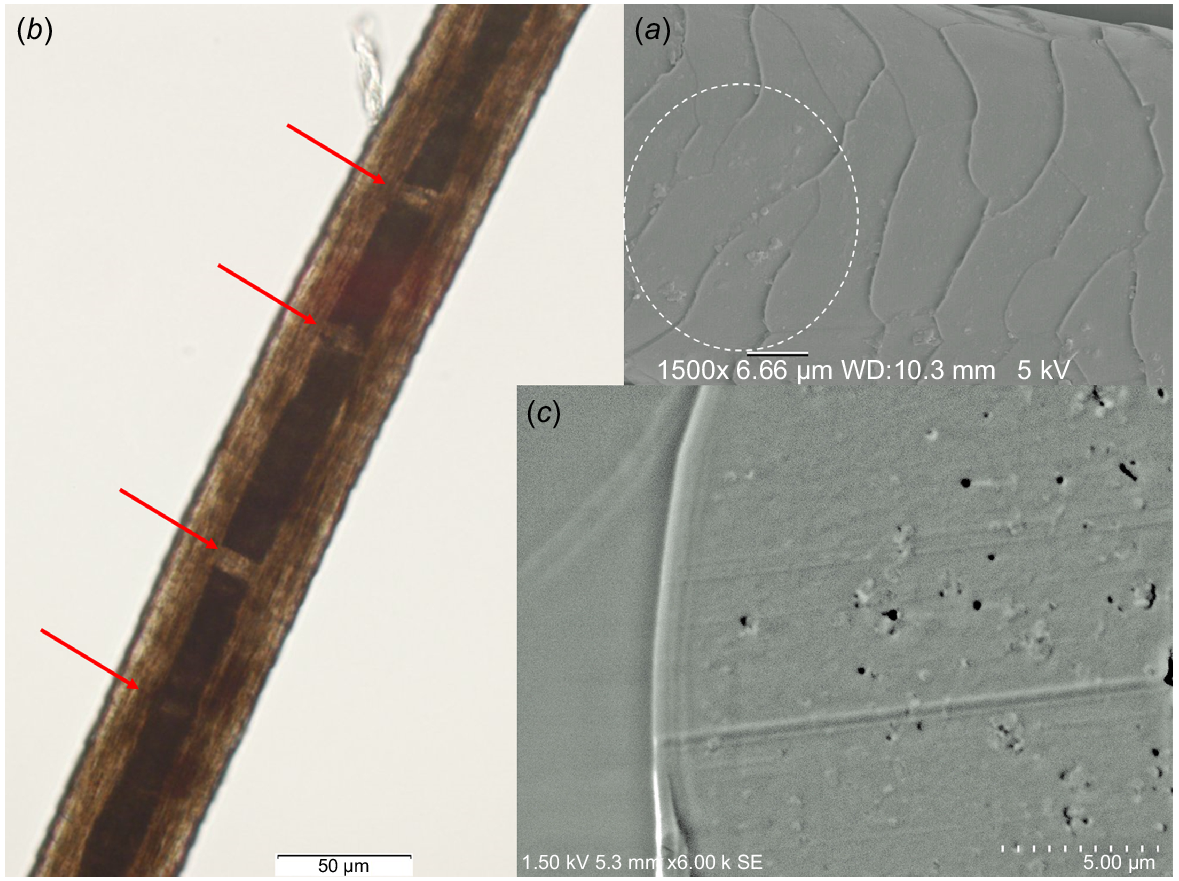
In Table 3, the amino acid compositions of the coat hair samples collected from animal Number 9 and Number 1831, suffering vitamin A deficiency and chronic renal failure respectively, showed features similar to those of the winter coat hair of animal Number 525, although those hair samples were collected late in May. The onset of shedding might have been delayed due to the effects of the respective diseases.
Fig. 8 shows the averaged stress-strain (S-S, n = 16–18) curves for the hair samples collected from animals Number 1245 and Number 1831 suffering chronic pneumonia and chronic renal failure respectively, along with the averaged S-S curve obtained from a healthy animal Number 1679. All of the hair samples used in Fig. 8 were considered to be classified into winter coat on the basis of the amino acid composition analyses and each collected date (see Tables 1 and 3). It is well known that the S-S curve of Merino sheep hair shows three stages, i.e. Hookean region, yield region, and past-yield region, when the hair is stretched in water; the hydrogen bonds in the hair tend to be dissociated by hydration (Wortmann and Zahn 1994). Further, while stretching in water, the border between the yield and the past-yield regions was found to be existing at the elongation percentage of about 30%. In this paper, the tensile measurements were made in air. However, the S-S curves showed almost similar features, although the yield region was not so flat and the border between the yield and the past-yield regions was unclear. It was likely that the free water in the hair served as a plasticiser to allow the deformation of the hair. The longer elongation with the higher stress observed in the tensile behaviour of animal Number 1679 hair implies the integrity of the cortex cells, fully connected with each other, constructing the hair shaft. The brittle property observed in animal Number 1245 hair was probably ascribed to the insufficient connections between the cortex cells induced by the serious deficiency in vitamin A. The inferior tensile properties of animal Number 1831 hair were also due to the weakened connections between the cortex cells (see Fig. 7c).
Averaged stress-strain curves (n = 16–18) for the cattle hair collected from the healthy animal (Number 1679), the animal (Number 1245) suffering chronic pneumonia, and the animal (Number 1831) suffering chronic renal failure.
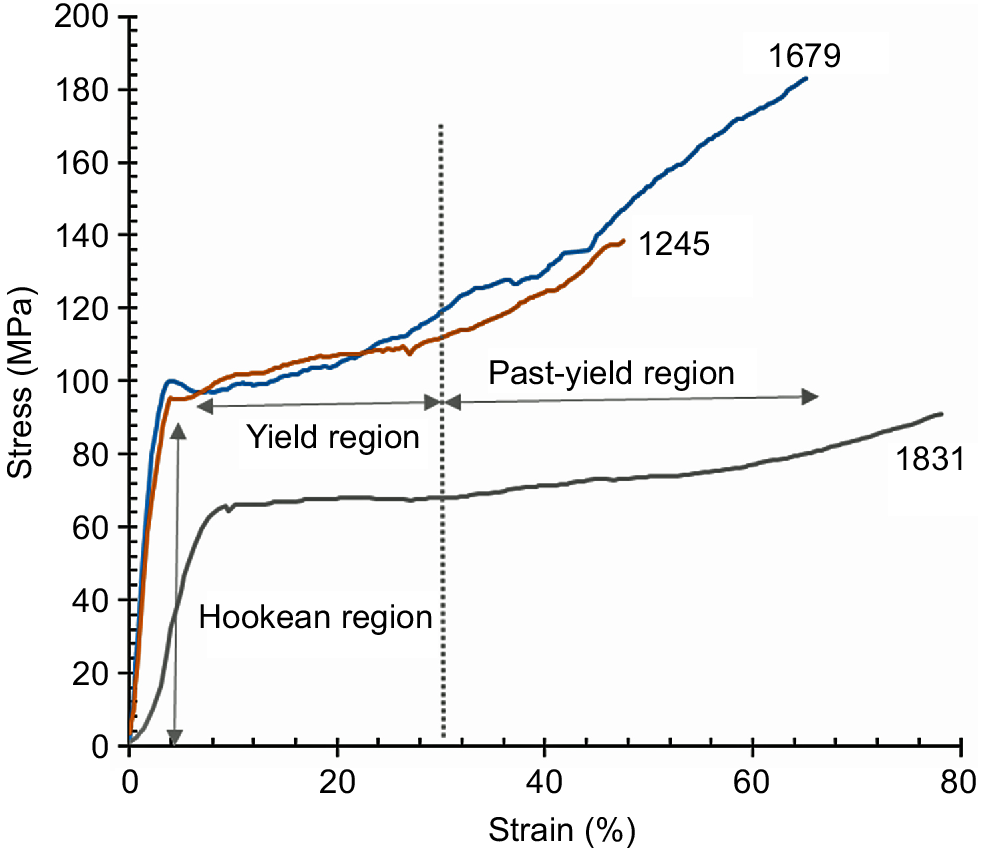
Fig. 9 shows the equatorial WAXD intensity curves for the coat hair samples collected from the cattle suffering vitamin A deficiency or other serious diseases, along with that for the hair sample of the healthy animal Number 6. Concerning the effects of vitamin A deficiency on the modification of WAXD intensity curve, a peak at 2θ = 9° was significantly weakened in the WAXD curve of animal Number 9 hair (vitamin A concentration of 11.1 μg/dL in plasma), although the hair samples collected from the different cattle, suffering vitamin A deficiency, showed WAXD curves similar to that of healthy animal Number 6 (see Fig. 9a). The decrease in the peak intensity may reflect the occurrence of disorder in the interspace distance between the adjacent keratin chains, and such disordering will be incorporated in the resultant stranded left-handed coiled-coil structure (Popescu and Höcker 2007).
Equatorial WAXD intensity curves for the cattle coat hair. The coat hair collected from the healthy animal (Number 6) was used as a standard: (a) the intensity curves for the hair collected from the cattle suffering vitamin A deficiency (Numbers 7, 9, 12); (b) the intensity curves for the hair from the cattle suffering pneumonia (Numbers 601, 1245, 8430), and the animal (Number 1831) suffering chronic renal failure.
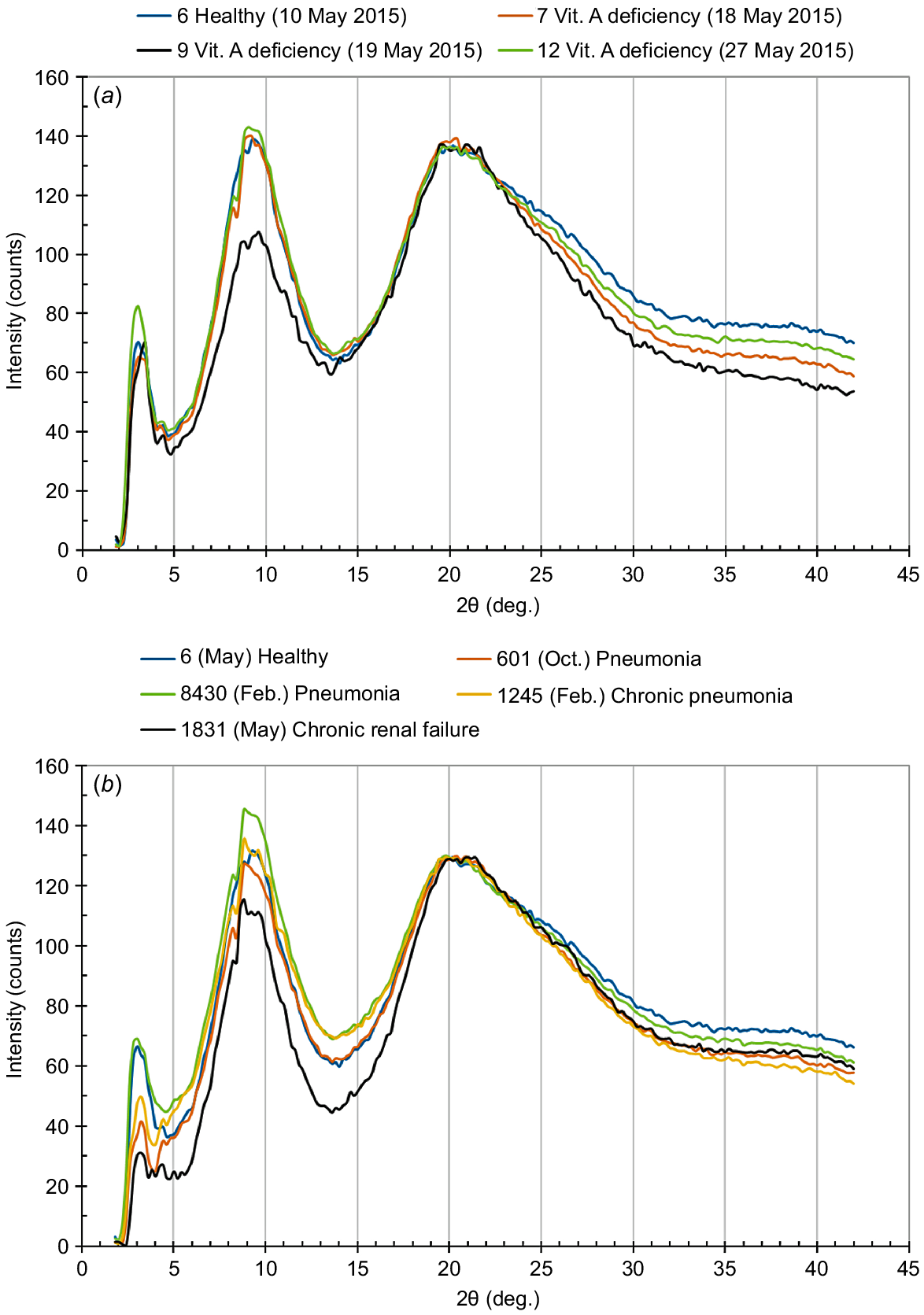
On the contrary, concerning the effects of pneumonia on the modification of WAXD intensity curve, a marked decrease was observed for the peak at 2θ = 3°, except for animal Number 8430 that showed a relatively high vitamin A concentration of 9.0 μg/dL in plasma (see Fig. 9b). The peak at 2θ = 3° corresponds to the proto-filament thickness of 3 nm (Aebi et al. 1983; Alberts et al. 1989; Katagata 1993). When we focus on the vitamin A concentration in plasma, we suggest that there may be a threshold value at ~10 μg/dL, concerning the occurrence of disordering in the formation of keratin fibrils. However, other effects should be taken into account because the WAXD intensity curve of the cattle hair collected from animal Number 1831, suffering chronic renal failure (vitamin A concentration of 41.0 μg/dL in plasma), was also modified. In this case, both peaks at 2θ = 3° and 2θ = 9° were significantly weakened.
Effects of pregnancy
Fig. 10 shows the optical microscopic images of the hair bulbs of the pregnant or non-pregnant cows obtained by carefully pulling the hair shaft without breaking its hair bulb. All of them exhibited club-like shapes partially covered with the follicle cells. The club-like shapes imply that the obtained hairs were at a telogen stage (Dowling and Nay 1960; Higgins et al. 2009; Nonogaki and Ueki 2020). The effects of pregnancy on the morphology of the hair bulb were hardly recognisable.
Optical microscopic images of the hair bulbs collected from the pregnant and non-pregnant cows.
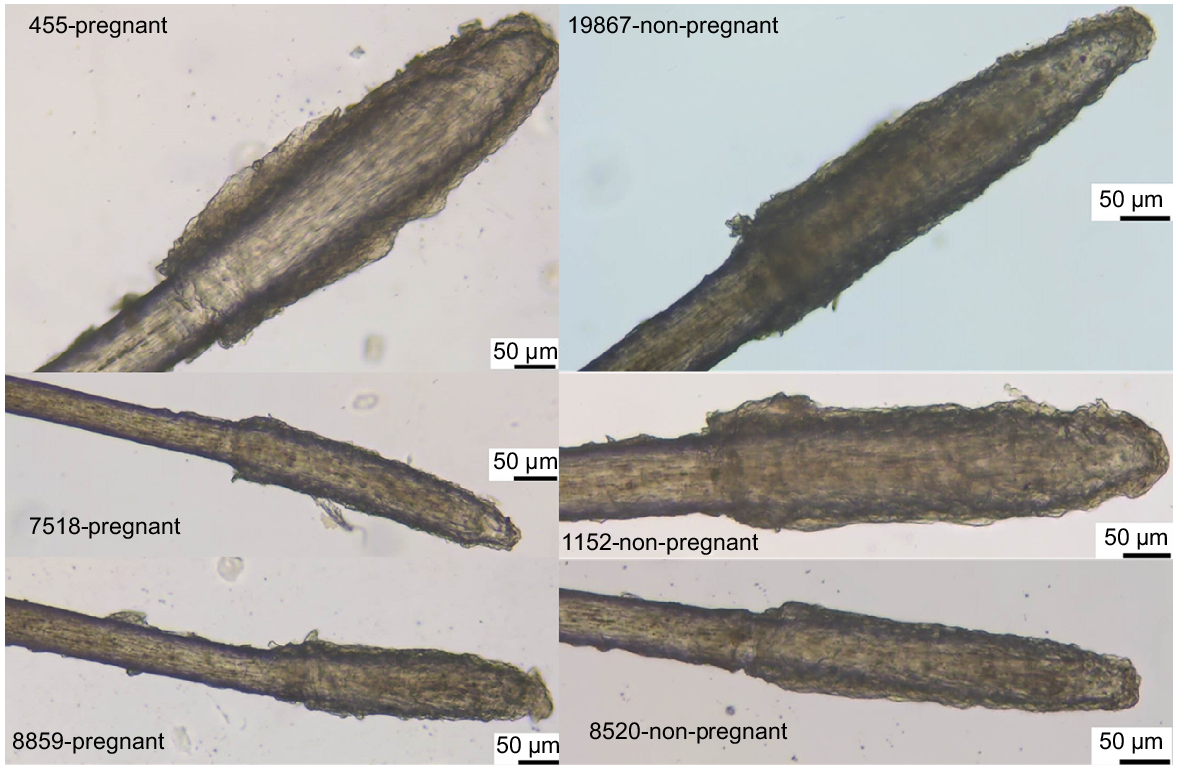
Fig. 11 shows the moisture-regaining properties of the hair samples collected from the pregnant or non-pregnant cows, measured by changing the ambient air humidity. As shown in Fig. 6, so as to characterise the slight difference in the individual cortex tissue structures, the abscissa axis represents the sulfur content of each hair sample estimated by the EDS measurements. Each moisture-regain increased monotonously with an increasing ambient air humidity, regardless of the effects of pregnancy and the structural differences in each cortex tissue. We suggest that both the hydrophilicity of the cow hair and its external and internal structure to allow the adsorption of water molecules would not be modified by the physiological effects induced by pregnancy.
Dependence of moisture-regain of the cow coat hair on the ambient air humidity. To characterise the slight difference in the individual cortex tissue structures, the abscissa axis represents the sulfur content of each hair sample estimated by the EDS measurements.
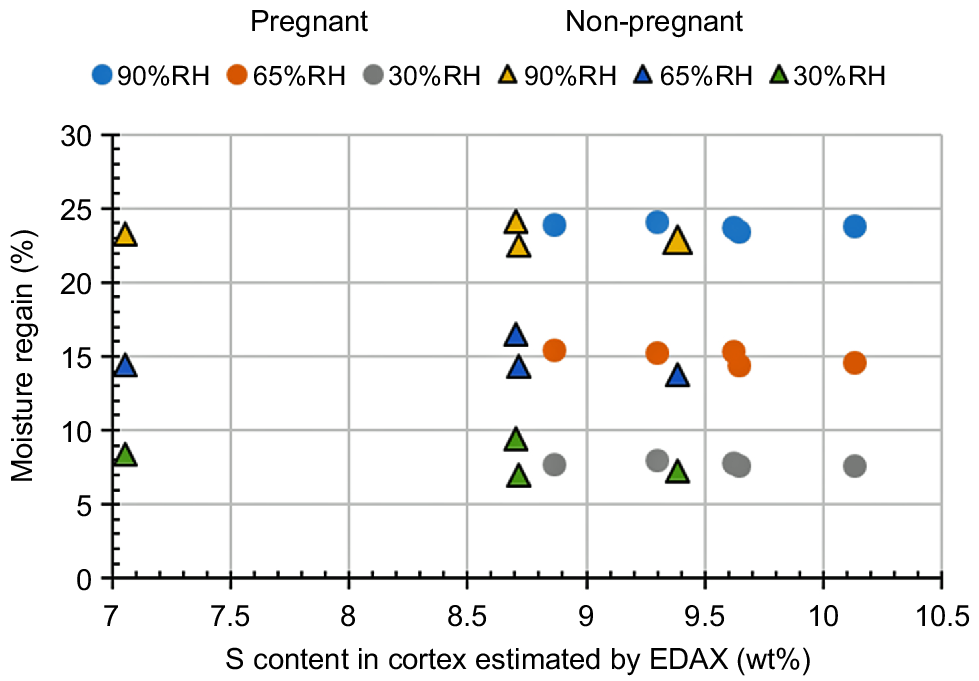
Fig. 12 shows the relationship between the diameters of hair and the tensile strengths, measured for the middle portion of the coat hair samples collected from the pregnant or non-pregnant cows. The mean values of the tensile data are listed in Table 2. Especially in the range of the diameter of <100 μm, the tensile strengths of hair samples collected from the pregnant cows showed somewhat superior values to those from the non-pregnant cows when the strengths were compared at the same diameter level. Thus, the WAXD intensity curves for the non-pregnant cow hair samples, having exhibited the inferior tensile properties (i.e. Numbers 1152, 7616), were measured to investigate the structural disorder in the filaments embedded in the cortex cells. The WAXD intensity curves for the hair samples obtained from the pregnant cows (i.e. Numbers 4140, 7330) were also measured for comparison (see Fig. 13). The WAXD peak at 2θ = 3° (d-spacing = 3 nm), corresponding to the thickness of the proto-filament (Aebi et al. 1983; Alberts et al. 1989; Katagata 1993), was weakened in the WAXD curve of the hair of cow Number 1152. Furthermore, in addition to the weakening of the peak at 2θ = 3°, the peak at 2θ = 9°, corresponding to the interspace distance between the adjacent keratin chains (Popescu and Höcker 2007), was significantly weakened in the WAXD curve of the hair of cow Number 7616.
Tensile strength and the corresponding diameter of the coat hairs collected from the pregnant or non-pregnant cows. The solid circle and triangle symbols indicate the data from the pregnant cow group (Numbers 4140, 7330, 7410, 7518, 8467) and non-pregnant cow group (Numbers 1152, 7379, 7353, 7616, 8520, 19 867) respectively.
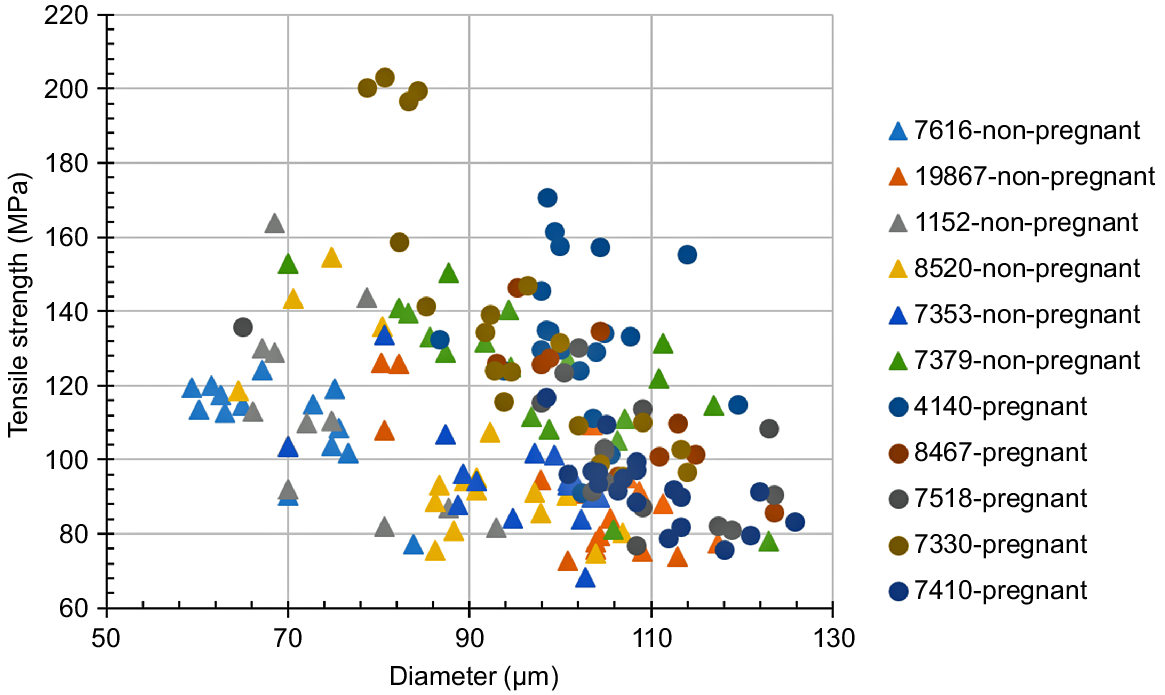
Equatorial WAXD intensity curves for the cow coat hair collected from the pregnant cows (Numbers 4140, 7330) or the non-pregnant cows (Numbers 1152, 7616).
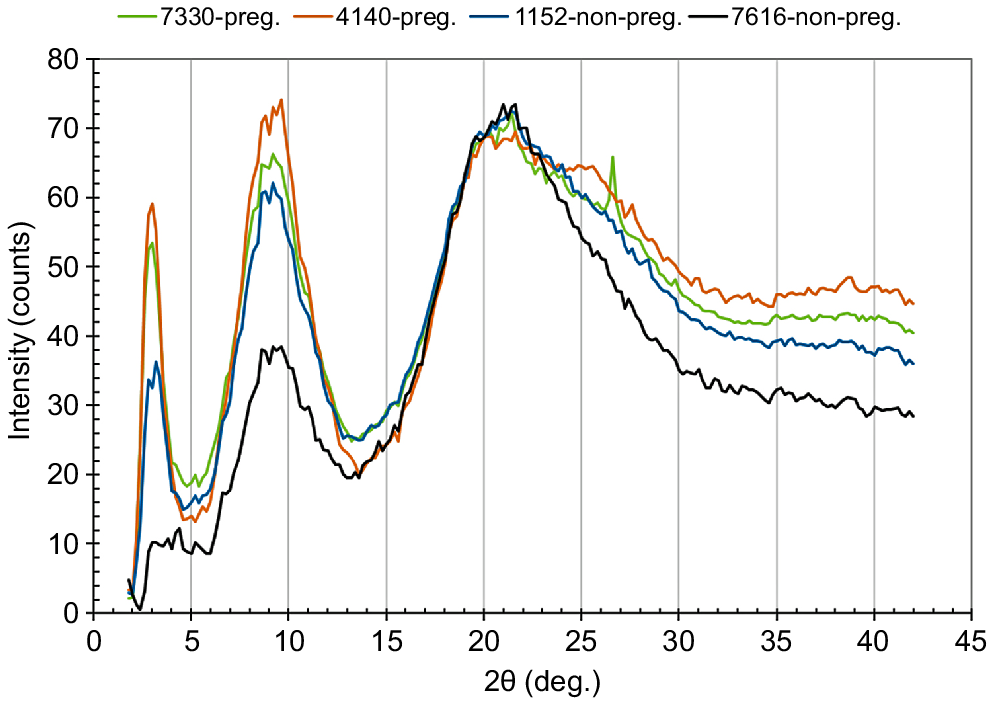
During pregnancy, it was reported that the teloptosis phase was delayed and the number of shedding hairs was reduced. Moreover, the diameter of scalp hair increased during pregnancy (Nissimov and Elchalal 2003). These phenomena were attributed to the effects of high concentrations of oestrogen during gestation (Nissimov and Elchalal 2003). However, the complex hormonal changes seen in pregnancy may well contribute to the increase in the rate of hair growth, in the hair diameter, and in the anagen:telogen ratio observed in pregnant women (Mirmirani 2011; Yoon et al. 2014; Mirallas and Grimalt 2016). Further, if the cortisol concentrations in plasma of the pregnant cows used in this study stayed low enough during pregnancy, it would be likely that the hair samples from the pregnant cows would show superior tensile properties to those of the non-pregnant ones; low cortisol concentrations can actually bring positive effects on hair growth (Thom 2016). However, in the actual veterinary care, we concentrated on the nutrition management. Thus, unfortunately, we have no data about the cortisol concentrations in plasma for the pregnant cows used in this study.
Conclusions
The microscopic observation of the summer- and winter-coat hair collected from the healthy cattle showed the seasonal differences in both the thickness of hair and the overlapping behaviour of cuticles. Their tensile properties and the moisture-regaining behaviour also changed seasonally. Furthermore, the data for the amino acid composition of hair suggested a possibility that the TGase-induced crosslinking might occur preferentially in the formation of the winter-coat hair.
On the basis of the hair data obtained from the healthy cattle, we observed some tendencies in the relationship between the physical condition of cattle and the physical features of their coat hair. It is well known that vitamin A is essential for the regeneration of normal epithelial tissue. Therefore, when the vitamin A concentration was extremely low, we could perceive the occurrence of the structural modification in the cattle coat hair via the WAXD measurements, reflecting the interference in the self-assembly of the keratin fibrils. We propose that there may be a threshold value at ~10 μg/dL of vitamin A in plasma, concerning a possible interference. However, not only did vitamin A deficiency cause structural modification of the coat hair, but also the coat hair from cattle suffering chronic renal failure was structurally modified.
Furthermore, so as to investigate the effects of pregnancy on the keratinisation process in the coat-hair follicles, the higher-order structure of the coat hair collected from the pregnant or non-pregnant cows was analysed. It was confirmed that both the amino acid composition and the internal structure were hardly modified by the physiological effects of pregnancy. However, the physiological conditions during pregnancy may be advantageous to the integrity of coat hair; the hair samples collected from the pregnant cows showed superior tensile strengths to those from the non-pregnant cows when compared at the same diameter level. In addition, the WAXD measurements showed the occurrence of the structural modification in the non-pregnant cow coat hair samples that had showed inferior tensile strengths.
We will continue further investigations on the structure of hair to show that hair has a function serving as a proxy for health status for a certain period.
Data availability
The data used to support the findings of this study are available on request to the corresponding author.
References
Aebi U, Fowler WE, Rew P, Sun T-T (1983) The fibrillar substructure of keratin filaments unraveled. The Journal of Cell Biology 97, 1131-1143.
| Crossref | Google Scholar | PubMed |
Almohanna HM, Ahmed AA, Tsatalis JP, Tosti A (2019) The role of vitamins and minerals in hair loss: a review. Dermatology and Therapy 9, 51-70.
| Crossref | Google Scholar | PubMed |
Arai K, Naito S, Dang VB, Nagasawa N, Hirano M (1996) Crosslinking structure of keratin. VI. Number, type, and location of disulfide crosslinkages in low-sulfur protein of wool fiber and their relation to permanent set. Journal of Applied Polymer Science 60, 169-179.
| Crossref | Google Scholar |
Bal C, Ağış ER, Gündüzöz M, Büyükşekerci M, Alışık M, Şen O, Tutkun E, Yılmaz ÖH (2017) Dynamic disulfide/thiol homeostasis in lead exposure denoted by a novel method. Toxicology and Industrial Health 33, 426-430.
| Crossref | Google Scholar | PubMed |
Biswas S, Rodeck CH (1976) Plasma prolactin levels during pregnancy. BJOG: An International Journal of Obstetrics and Gynaecology 83, 683-687.
| Crossref | Google Scholar | PubMed |
Braun-Falco O, Plewig G, Wolff HH, Burgdorf WHC (2000) Diseases of hair. In ‘Dermatology’. 2nd, completely revised edn. (Eds O Braun-Falco, G Plewig, HH Wolff, WHC Burgdorf) p. 1101. (Springer: New York, NY, USA) doi:10.1007/978-3-642-97931-6
Cieszynski L, Jendrzejewski J, Wisniewski P, Owczarzak A, Sworczak K (2019) Hair cortisol concentration in a population without hypothalamic–pituitary–adrenal axis disorders. Advances in Clinical and Experimental Medicine 28, 369-373.
| Crossref | Google Scholar | PubMed |
Coulombe PA, Fuchs E (1990) Elucidating the early stages of keratin filament assembly. The Journal of Cell Biology 111, 153-169.
| Crossref | Google Scholar |
Craven AJ, Ormandy CJ, Robertson FG, Wilkins RJ, Kelly PA, Nixon AJ, Pearson AJ (2001) Prolactin signaling influences the timing mechanism of the hair follicle: analysis of hair growth cycles in prolactin receptor knockout mice. Endocrinology 142, 2533-2539.
| Crossref | Google Scholar | PubMed |
Dowling DF, Nay T (1960) Cyclic changes in the follicles and hair coat in cattle. Australian Journal of Agricultural Research 11, 1064-1071.
| Crossref | Google Scholar |
Duncan MJ, Goldman BD (1984) Hormonal regulation of the annual pelage color cycle in the Djungarian hamster, Phodopus sungorus. II. Role of prolactin. Journal of Experimental Zoology 230, 97-103.
| Crossref | Google Scholar | PubMed |
Foitzik K, Krause K, Conrad F, Nakamura M, Funk W, Paus R (2006) Human scalp hair follicles are both a target and a source of prolactin, which serves as an autocrine and/or paracrine promoter of apoptosis-driven hair follicle regression. The American Journal of Pathology 168, 748-756.
| Crossref | Google Scholar | PubMed |
Gorocica-Buenfil MA, Fluharty FL, Reynolds CK, Loerch SC (2007a) Effect of dietary vitamin A concentration and roasted soybean inclusion on marbling, adipose cellularity, and fatty acid composition of beef. Journal of Animal Science 85, 2230-2242.
| Crossref | Google Scholar | PubMed |
Gorocica-Buenfil MA, Fluharty FL, Bohn T, Schwartz SJ, Loerch SC (2007b) Effect of low vitamin A diets with high-moisture or dry corn on marbling and adipose tissue fatty acid composition of beef steers. Journal of Animal Science 85, 3355-3366.
| Crossref | Google Scholar | PubMed |
Grymowicz M, Rudnicka E, Podfigurna A, Napierala P, Smolarczyk R, Smolarczyk K, Meczekalski B (2020) Hormonal effects on hair follicles. International Journal of Molecular Sciences 21(15), 5342.
| Crossref | Google Scholar | PubMed |
Higgins CA, Westgate GE, Jahoda CAB (2009) From telogen to exogen: mechanisms underlying formation and subsequent loss of the hair club fiber. Journal of Investigative Dermatology 129, 2100-2108.
| Crossref | Google Scholar | PubMed |
Hitomi K (2005) Transglutaminases in skin epidermis. European Journal of Dermatology 15, 313-319.
| Google Scholar | PubMed |
Hitomi K, Presland RB, Nakayama T, Fleckman P, Dale BA, Maki M (2003) Analysis of epidermal-type transglutaminase (transglutaminase 3) in human stratified epithelia and cultured keratinocytes using monoclonal antibodies. Journal of Dermatological Science 32, 95-103.
| Crossref | Google Scholar | PubMed |
Ignacak A, Kasztelnik M, Sliwa T, Korbut RA, Rajda K, Guzik TJ (2012) Prolactin – not only lactotrophin. A ‘new’ view of the ‘old’ hormone. Journal of Physiology and Pharmacol 63, 435-443.
| Google Scholar | PubMed |
Inoue T, Iwamoto Y, Ohta N, Inoue K, Yagi N (2007) Structural analysis of the cell membrane complex in the human hair cuticle using microbeam X-ray diffraction: relationship with the effects of hair dyeing. Journal of Cosmetic Science 58, 11-17.
| Google Scholar | PubMed |
Institute of Medicine (2001) Panel on micronutrients. In ‘Dietary reference intakes for vitamin A, vitamin K, arsenic, boron, chromium, copper, iodine, iron, manganese, molybdenum, nickel, silicon, vanadium, and zinc’. (Eds V Young, C Garza) pp. 82–161. (National Academies Press: Washington, DC, USA) doi:10.17226/10026. pubmed id:25057538
Kalinin AE, Kajava AV, Steinert PM (2002) Epithelial barrier function: assembly and structural features of the cornified cell envelope. BioEssays 24, 789-800.
| Crossref | Google Scholar | PubMed |
Kalliokoski O, Jellestad FK, Murison R (2019) A systematic review of studies utilizing hair glucocorticoids as a measure of stress suggests the marker is more appropriate for quantifying short-term stressors. Scientific Reports 9, 11997.
| Crossref | Google Scholar | PubMed |
Katagata Y (1993) Biochemistry of keratin proteins. Protein, Nucleic Acid and Enzyme 38, 2711-2722.
| Google Scholar |
Liu CH, Doan SN (2019) Innovations in biological assessments of chronic stress through hair and nail cortisol: conceptual, developmental, and methodological issues. Developmental Psychobiology 61(3), 465-476.
| Crossref | Google Scholar | PubMed |
Lutz G (2012) Hair loss and hyperprolactinemia in women. Dermato-Endocrinology 4, 65-71.
| Crossref | Google Scholar | PubMed |
Martinet L, Allain D, Weiner C (1984) Role of prolactin in the photoperiodic control of moulting in the mink (Mustela vison). Journal of Endocrinology 103, 9-15.
| Crossref | Google Scholar | PubMed |
McGill JL, Kelly SM, Guerra-Maupome M, Winkley E, Henningson J, Narasimhan B, Sacco RE (2019) Vitamin A deficiency impairs the immune response to intranasal vaccination and RSV infection in neonatal calves. Scientific Reports 9, 15157.
| Crossref | Google Scholar | PubMed |
Merk HF (1990) Drugs affecting hair growth. In ‘Hair and hair diseases’. (Eds CE Orfanos, R Happle) pp. 601–609. (Springer: New York, NY, USA) doi:10.1007/978-3-642-74612-3
Mirallas O, Grimalt R (2016) The postpartum telogen effluvium fallacy. Skin Appendage Disorders 1, 198-201.
| Crossref | Google Scholar | PubMed |
Mirmirani P (2011) Hormonal changes in menopause: do they contribute to a ’midlife hair crisis’ in women? British Journal of Dermatology 165(Suppl. 3), 7-11.
| Crossref | Google Scholar |
Naito S, Arai K, Hirano M, Nagasawa N, Sakamoto M (1996) Crosslinking structure of keratin. V. Number and type of crosslinks in microstructures of untreated and potassium cyanide treated human hair. Journal of Applied Polymer Science 61, 1913-1925.
| Crossref | Google Scholar |
Namikawa T (1972) Genetic similarities among seven cattle populations of eastern asia and holstein. breed. SABRAO Newsletter 4, 17-25.
| Google Scholar |
Nissimov J, Elchalal U (2003) Scalp hair diameter increases during pregnancy. Clinical and Experimental Dermatology 28, 525-530.
| Crossref | Google Scholar | PubMed |
Nonogaki K, Ueki R (2020) A case of telogen effluvium associated with drug-induced hyperprolactinemia. Japanese Society of Clinical Dermatology Journal 37, 75-79.
| Crossref | Google Scholar |
Ohta N, Oka T, Inoue K, Yagi N, Kato S, Hatta I (2005) Structural analysis of cell membrane complex of a hair fibre by micro-beam X-ray diffraction. Journal of Applied Crystallography 38, 274-279.
| Crossref | Google Scholar |
Popescu C, Höcker H (2007) Hair: the most sophisticated biological composite material. Chemical Society Reviews 36, 1282-1291.
| Crossref | Google Scholar | PubMed |
Radostits OM, Gay CC, Hinchcliff KW, Constable PD (2007) Diseases associated with nutritional deficiencies. In ‘Veterinary medicine, a textbook of the diseases of cattle, horses, sheep, pigs and goats’. 10th edn. (Eds OM Radostits, CC Gay, KW Hinchcliff, PD Constable) pp. 1771–1777. (Saunders/Elsevier: Philadelphia, PA, USA)
Reiter C, Prohaska T (2021) Beethoven’s death: the result of medical malpractice? Wiener Medizinische Wochenschrift 171, 356-362.
| Crossref | Google Scholar | PubMed |
Robbins C (2009) The cell membrane complex: three related but different cellular cohesion components of mammalian hair fibers. Journal of Cosmetic Science 60, 437-465.
| Crossref | Google Scholar | PubMed |
Rougeot J, Allain D, Martinet L (1984) Photoperiodic and hormonal control of seasonal coat changes in mammals with special reference to sheep and mink. Acta Zoologica Fennica 171, 13-18.
| Google Scholar |
Ruszczak Z (2012) Hair disorders and alopecia. In ‘Textbook of clinical pediatrics’. (Eds AY Elzouki, HA Harfi, HM Nazer, FB Stapleton, W Oh, RJ Whitley) pp. 1489–1508. (Springer: New York, NY, USA) doi:10.1007/978-3-642-02202-9_146
Shah KD, Nakao T, Kubota H (2006) Plasma estrone sulphate (E1S) and estradiol-17β (E2β) profiles during pregnancy and their relationship with the relaxation of sacrosciatic ligament, and prediction of calving time in Holstein-Friesian cattle. Animal Reproduction Science 95, 38-53 Epub 2005 Oct 7.
| Crossref | Google Scholar | PubMed |
Steinert PM, Kim S-Y, Chung S-I, Marekov LN (1996) The transglutaminase 1 enzyme is variably acylated by myristate and palmitate during differentiation in epidermal keratinocytes. Journal of Biological Chemistry 271, 26242-26250.
| Crossref | Google Scholar | PubMed |
Sugaya N, Izawa S, Ogawa N, Shirotsuki K, Nomura S (2020) Association between hair cortisol and diurnal basal cortisol levels: a 30-day validation study. Psychoneuroendocrinology 116, 104650.
| Crossref | Google Scholar | PubMed |
Sugaya N, Izawa S, Nomura S (2021) Validation of hair and nail cortisol evaluation as a new stress assessment approach. Japanese Journal of Psychosomatic Medicine 61, 496-505.
| Crossref | Google Scholar |
Sumiyoshi T, Tanaka T, Kamomae H (2014) Relationships between the appearances and changes of estrous signs and the estradiol-17β peak, luteinizing hormone surge and ovulation during the periovulatory period in lactating dairy cows kept in tie-stalls. Journal of Reproduction and Development 60, 106-114.
| Crossref | Google Scholar | PubMed |
Suzuki B, Sugiyama T, Yoshida C, Nakao T (2018) Short communication: temporal changes in the skin morphology of dairy cows during the periparturient period. Journal of Dairy Science 101, 6616-6621.
| Crossref | Google Scholar | PubMed |
Thom E (2016) Stress and the hair growth cycle: cortisol-induced hair growth disruption. Journal of Drugs in Dermatology 15(8), 1001-1004.
| Google Scholar | PubMed |
Thompson DL, DePew CL (1997) Prolactin, gonadotropin, and hair shedding responses to daily sulpiride administration in geldings in winter. Journal of Animal Science 75, 1087-1091.
| Crossref | Google Scholar |
Wortmann F-J, Zahn H (1994) The stress/strain curve of α-keratin fibers and the structure of the intermediate filament. Textile Research Journal 64, 737-743.
| Crossref | Google Scholar |
Xiang L, Sunesara I, Rehm KE, Marshall GD (2017) Hair cortisol concentrations are associated with hair growth rate. Neuroimmunomodulation 23, 287-294.
| Crossref | Google Scholar | PubMed |
Yoon S-Y, Yoon J-S, Jo SJ, Shin CY, Shin J-Y, Kim J-I, Kwon O, Kim KH (2014) A role of placental growth factor in hair growth. Journal of Dermatological Science 74, 125-134.
| Crossref | Google Scholar | PubMed |