Drone surveys cause less disturbance than ground-based surveys in endangered spectacled flying-foxes (Pteropus conspicillatus)
Emmeline Bernadette Barrett Norris
A
Abstract
Robust population estimates are critical for detecting biodiversity declines. Thermal drones offer a promising alternative to invasive, imprecise ground-based techniques for monitoring endangered spectacled flying-foxes (Pteropus conspicillatus). This study evaluated spectacled flying-fox behavioural responses to drones to address concerns that they will disturb roosting colonies. At two studied roosts, drones elicited minimal disturbance, whereas ground-based surveys triggered alarm and escape responses, particularly among unhabituated flying-foxes. These findings highlight thermal drones as a non-invasive tool for monitoring spectacled flying-foxes. Further research is needed to evaluate their accuracy and precision compared with ground counts.
Keywords: animal behaviour, Chiroptera, conservation, Old World fruit bats, Pteropodidae, RPAS, thermal infrared, UAV, wildlife monitoring.
Introduction
Robust population estimates are critical for detecting biodiversity declines and evaluating conservation interventions (Lindenmayer et al. 2012). Monitoring techniques must avoid altering natural behaviours because stress may negatively affect reproductive success (Carey 2009; Edwards et al. 2019). For highly mobile, colonial species such as flying-foxes and waterbirds, ground-based breeding colony censuses can induce widespread disturbance, roost abandonment, and potential miscounts (Borrelle and Fletcher 2017). Minimising disturbance while maximising detectability is critical to effective population monitoring (Lindenmayer et al. 2020).
Drones have emerged as a promising tool for monitoring colonial-nesting fauna. Compared with ground counts, they offer greater area coverage, access to remote sites, and reduced observer bias (Lyons et al. 2019; Lachman et al. 2020; Hayes et al. 2021; Attard et al. 2024). Drone-mounted thermal cameras (thermal drones) further enhance detection for camouflaged or partially obscured animals by distinguishing their heat signatures from background environments (Witt et al. 2020; Virtue et al. 2023). Although many species do not react to drones (Arona et al. 2018; Geldart et al. 2022; Norris and Larson 2025), some taxa exhibit strong disturbance responses (Weimerskirch et al. 2018; Giles et al. 2021; Aubin et al. 2023; Demmer et al. 2024). Assessing target species’ responses to drone- and ground-based surveys is crucial for selecting a suitable monitoring approach.
Flying-foxes (Pteropodidae) are well-suited to thermal drone monitoring because their heat signatures are readily detected while roosting in forest canopies (McCarthy et al. 2021; Seegobin et al. 2024). Thermal drones have been successfully used to count grey-headed flying-foxes (Pteropus poliocephalus), providing more accurate and precise colony estimates than do ground counts by human observers (McCarthy et al. 2021, 2022). Currently, 38 of 54 assessed Pteropus spp. are threatened and six are extinct (Kingston et al. 2023; IUCN 2025). As long-distance pollinators and seed dispersers (Fujita and Tuttle 1991; McConkey and Drake 2006), flying-foxes require robust population assessments to detect declines that could negatively affect forest biodiversity. Yet, established ground-based census methods, such as fly-out or walk-through counts, are hindered by roost inaccessibility, human-induced disturbance, and observer bias, limiting their ability to detect shifting population trends (Westcott and McKeown 2004; Westcott et al. 2012). Thermal drones could enhance statistical power to detect trends by enabling objective, whole-roost surveys and reducing disturbance-induced movement that could skew counts (McCarthy et al. 2022).
Endangered spectacled flying-foxes (Pteropus conspicillatus), endemic to tropical north-eastern Queensland (Roberts et al. 2020), have declined by approximately 75% over 14 years as a result of deforestation, cyclones, and an extreme heat event that killed nearly a third of the population (Westcott et al. 2018; Lopes et al. 2024). Timely detection of ongoing trends in the diminished population requires more precise local abundance estimates to improve monitoring power (Westcott et al. 2012). Flying-fox intolerance to humans often prevents ground counters from entering roosts, necessitating extrapolation at larger roosts (Westcott et al. 2011, 2012). Given these constraints, thermal drones are being trialled as an alternative for improving count accuracy and precision (E. B. B. Norris, unpubl. data). This study compares spectacled flying-fox behavioural responses to drone- and ground-based surveys, evaluating disturbance levels and establishing the minimum flight height to prevent disturbance.
Methods
The study formed part of a pilot study testing thermal drones for monitoring spectacled flying-foxes. The research was approved by James Cook University Animal Research Authority No. A2969.
Behavioural assessments occurred at two spectacled flying-fox roosts in north-eastern Queensland, Australia, during September 2024 (Fig. 1). The El Arish roost (–17.801560, 146.006416) is an open eucalypt forest and the Tolga Scrub roost (–17.230005, 145.479608) is a complex notophyll vine forest. Roosts were selected on the basis of accessibility, occupancy, and unrestricted airspace for drone flights.
Maps of (a, b) the two study sites at El Arish and Tolga Scrub in north-eastern Queensland, Australia, and (c, d) aerial images showing drone launch sites adjacent to spectacled flying-fox (Pteropus conspicillatus) roost trees at (c) El Arish and (d) Tolga Scrub.
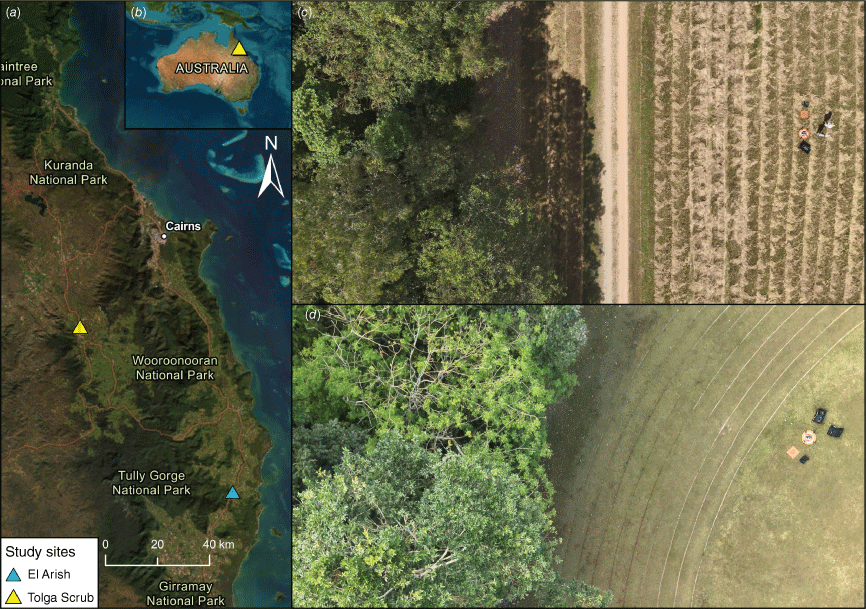
Two drone models were tested, namely, a DJI Matrice 350 RTK (M350) with a DJI Zenmuse H30T payload (7.39 kg), and a DJI Mavic 3T Enterprise (M3T) with a built-in thermal camera (0.92 kg; Supplementary Table S1) (DJI Technology Co., Shenzhen, China). Six drone flights were conducted at El Arish over 3 days and four at Tolga Scrub in 1 day, all between sunrise and midday in partly cloudy conditions.
To determine the minimum disturbance-free flight height, the M350 was flown to 50 m above canopy height (ACH), then gradually lowered to 5 m ACH (Supplementary Video S1). The conservative initial flight height, which exceeded the 23 m flight height used for grey-headed flying-foxes (McCarthy et al. 2022), was chosen in case spectacled flying-foxes reacted differently to the drone, as has been observed among congeneric waterbird species (Marchowski 2021). No reaction was observed to the drone flying >7 m ACH; however, a minimum flight altitude of 15 m ACH was implemented to improve orthomosaic processing. Subsequent automated flights were conducted at this height with speeds of 2–3 m s−1, a nadir-oriented camera, and following parallel transects with 90% image overlap.
To compare disturbance responses, drone flights preceded a single ground-based survey. The smaller M3T drone, which produces less noise and was expected to cause less disturbance, was deployed first on each survey occasion, to assess flying-fox tolerance before using the M350. Ground surveys were conducted last, as flying-foxes may abandon roosts when approached by humans (Westcott et al. 2011). During all surveys, disturbance behaviours were classified using an ethogram (Table 1) based on personal observations and established drone-response behaviours in primates (Rahman et al. 2021). Alarm calls were distinguished from social vocalisations, and wing flapping as an alarm response was distinguished from thermoregulatory wing fanning.
Behaviour | Description | |
---|---|---|
Resting | Inactive, sleeping, grooming, playing, or mating | |
Vigilant | Lateral head movements and eyes open | |
Alert | Visually tracking disturbance source | |
Alarmed | Alarm calling, flapping wings, or moving short distances between branches | |
Escape | Moving away from disturbance source |
Drones were launched ~20 m from roosts to minimise pre-flight disturbance (Fig. 1c, d) and flown individually over the forest edge where flying-foxes beneath the drone were visible to ground-based observers. Reactions were observed by a ground observer by using binoculars and the drone pilot monitoring real-time thermal video (Table 2). Flights were to be terminated immediately if disturbance was observed.
Date | Site | Start time | Flight duration (min) | Drone model | SFF response (drone) | SFF response (ground) | |
---|---|---|---|---|---|---|---|
2/9/2024 | El Arish | 10:30 am | 2 | M3T | Resting | Alert, alarmed, escape | |
2/9/2024 | El Arish | 10:52 am | 9 | M350 | Resting | ||
6/9/2024 | El Arish | 9:48 am | 25 | M350 | Resting | Alert, alarmed | |
18/9/2024 | El Arish | 7:36 am | 10 | M3T | Resting | Alert, alarmed, escape | |
18/9/2024 | El Arish | 7:49 am | 12 | M350 | Resting | ||
18/9/2024 | El Arish | 8:18 am | 4 | M3T | Resting | ||
19/9/2024 | Tolga Scrub | 8:33 am | 5 | M3T | Resting | Alert | |
19/9/2024 | Tolga Scrub | 8:41 am | 6 | M350 | Resting | ||
19/9/2024 | Tolga Scrub | 12:17 am | 10 | M350 | Vigilant | Alert, alarmed | |
19/9/2024 | Tolga Scrub | 12:31 am | 5 | M3T | Vigilant |
Each ground survey was conducted once per set of drone flights to minimise colony stress. Behavioural response categories are defined in Table 1. M3T and M350 refer to the DJI Mavic Enterprise 3T and DJI Matrice 350 RTK drones respectively.
For ground surveys, one observer recorded flying-fox responses as they approached the roost from ~20 m away and slowly entered the understorey. A second observer recorded responses from a fixed position 20 m away by using binoculars (Table 2). The approaching observer retreated if more than 20 flying-foxes initiated flight, following established disturbance thresholds for grey-headed flying-foxes (McCarthy et al. 2022).
Results and discussion
Spectacled flying-foxes reacted minimally to drone surveys, with no alarm or escape responses being observed (Table 2). Despite its greater size and noise output, the M350 drone did not elicit stronger responses than did the M3T. When the M350 descended to 7 m ACH, some individuals briefly looked up, indicating increased alertness (Table 1), before resuming their activity (Supplementary Video S1). Hence, a minimum flight height of 7 m ACH was initially considered adequate to avoid disturbance. However, lower altitudes reduced the camera’s field of view, increasing the number of images required for orthomosaic processing and impeding image alignment. To balance these factors, a minimum flight height of 15 m ACH was established to maintain sufficient image quality and enable orthomosaic processing.
Disturbance responses to drones varied with time of day. During early morning, most individuals remained asleep and unresponsive to drone presence. Social activity increased after ~9 am and, by midday, individuals were more vigilant towards the drones (Table 2). Although afternoon vigilance responses were mild (Table 1), early morning drone surveys are preferable not only to minimise disturbance but also to take advantage of improved thermal contrast and detectability in cooler ambient temperatures with indirect sunlight (Burke et al. 2019).
Spectacled flying-foxes showed strong disturbance responses to on-ground human observers. At El Arish, individuals alarm-called, flapped their wings, or climbed higher when approached, with entry into the understorey triggering flight (Table 2; Supplementary Video S2). At Tolga Scrub, responses were more subdued, with few individuals initiating flight (Table 2). This difference is likely to reflect human habituation, because Tolga Scrub is occupied year-round and borders a frequently used sportsground (Fig. 1) (Parsons et al. 2011). In contrast, the El Arish roost is occupied periodically (E. B. B. Norris, pers. obs.), and no human activity was observed during surveys, despite its proximity to a sportsground (Fig. 1). Drone habituation was unlikely, because neither site had prior drone exposure. Although some individuals may have encountered drones elsewhere, the rarity of past drone surveys suggests that the observed responses reflect natural behaviour, supporting the broader applicability of these findings.
Reproductive stage may influence behavioural responses to drones and ground-based human observers. During the mating season (January to May), polygynous males exhibit heightened defensive behaviours, potentially increasing energetic costs as males defend harems from perceived threats (Fox 2006; Welbergen 2006). Meanwhile, females may limit flight responses during gestation and parturition to conserve energy and protect young. The surveys described in this study were conducted in September, during gestation but before parturition and copulation. At this time, heightened male defensive behaviours were not expected, but gravid females may have limited their flight responses to conserve energy. Whereas further research is needed to assess seasonal variation in behavioural responses, subsequent whole-roost drone surveys during parturition (November) and early mating (January), conducted since this study, have also not elicited obvious disturbance (E. B. B. Norris, pers. obs.).
Spectacled flying-foxes are vulnerable to raptor predation (Koel 2023), but show minimal response to overhead raptors while roosting (R. Hopkinson, pers. comm.). This lack of reaction, as with drones, may be an anti-predator strategy, because initiating flight could increase predation risk (Welbergen 2006). Instead, flying-foxes may rely on concealment to avoid detection. Although no visible distress was observed, and individuals displayed natural behaviours (e.g. grooming, socialising) during drone flights, future research incorporating physiological stress metrics (e.g. heart rate, glucocorticoids) could better assess potential disturbance effects of drone exposure (Reeder et al. 2006; Geldart et al. 2022).
The present findings support thermal drones as a minimally invasive tool for censusing spectacled flying-foxes. Compared with ground surveys, drones reduce disturbance-induced movement, enabling whole-roost surveys and potentially reducing miscounts (Vallecillo et al. 2021). However, dense vegetation and higher ambient temperatures in tropical environments may affect detection rates, making it essential to assess whether drone-based methods yield similarly robust results, as those reported for grey-headed flying-foxes in temperate open forests (McCarthy et al. 2022). Ongoing research will evaluate these trade-offs, comparing the accuracy and precision of thermal drone and ground-based counts for spectacled flying-foxes.
Data availability
This research note is based on incidental observations. All relevant data have been included in the paper and supplementary material. Additional raw observation records, images, and metadata can be made available upon reasonable request to the corresponding author.
Conflicts of interest
The authors declare that there are no conflicts of interest regarding the publication of this paper.
Declaration of funding
This research was funded by the National Environmental Science Program (NESP), Skyrail Rainforest Foundation, the Linnean Society of New South Wales, and the Australian Wildlife Society. The funding bodies had no role in the study design, data collection, analysis, or interpretation, nor in the decision to publish this paper.
Acknowledgements
I am grateful to R. Hopkinson and N. Preece for their assistance in the field and feedback on drafts, A. Dennis and Terrain NRM for their collaboration on the project, and Cassowary Coast Regional Council for facilitating fieldwork. I also thank S. Laurance, W. Edwards, and A. Krockenberger for their supervision, feedback, and support. I appreciate the reviewer’s constructive comments, which contributed to improving this paper.
References
Arona, L., Dale, J., Heaslip, S. G., Hammill, M. O., and Johnston, D. W. (2018). Assessing the disturbance potential of small unoccupied aircraft systems (UAS) on gray seals (Halichoerus grypus) at breeding colonies in Nova Scotia, Canada. PeerJ 6, e4467.
| Crossref | Google Scholar | PubMed |
Attard, M. R. G., Phillips, R. A., Bowler, E., Clarke, P. J., Cubaynes, H., Johnston, D. W., and Fretwell, P. T. (2024). Review of satellite remote sensing and unoccupied aircraft systems for counting wildlife on land. Remote Sensing 16(4), 627.
| Crossref | Google Scholar |
Aubin, J. A., Mikus, M.-A., Michaud, R., Mennill, D., and Vergara, V. (2023). Fly with care: Belugas show evasive responses to low altitude drone flights. Marine Mammal Science 39(3), 718-739.
| Crossref | Google Scholar |
Borrelle, S. B., and Fletcher, A. T. (2017). Will drones reduce investigator disturbance to surface-nesting seabirds? Marine Ornithology 45, 89-94.
| Google Scholar |
Burke, C., Rashman, M., Wich, S., Symons, A., Theron, C., and Longmore, S. (2019). Optimizing observing strategies for monitoring animals using drone-mounted thermal infrared cameras. International Journal of Remote Sensing 40(2), 439-467.
| Crossref | Google Scholar |
Carey, M. J. (2009). The effects of investigator disturbance on procellariiform seabirds: a review. New Zealand Journal of Zoology 36(3), 367-377.
| Crossref | Google Scholar |
Demmer, C. R., Demmer, S., and McIntyre, T. (2024). Drones as a tool to study and monitor endangered Grey Crowned Cranes (Balearica regulorum): behavioural responses and recommended guidelines. Ecology and Evolution 14(2), e10990.
| Crossref | Google Scholar |
Fujita, M. S., and Tuttle, M. D. (1991). Flying foxes (Chiroptera: Pteropodidae): threatened animals of key ecological and economic importance. Conservation Biology 5(4), 455-463.
| Crossref | Google Scholar |
Geldart, E. A., Barnas, A. F., Semeniuk, C. A. D., Gilchrist, H. G., Harris, C. M., and Love, O. P. (2022). A colonial-nesting seabird shows no heart-rate response to drone-based population surveys. Scientific Reports 12(1), 18804.
| Crossref | Google Scholar |
Giles, A. B., Butcher, P. A., Colefax, A. P., Pagendam, D. E., Mayjor, M., and Kelaher, B. P. (2021). Responses of bottlenose dolphins (Tursiops spp.) to small drones. Aquatic Conservation: Marine and Freshwater Ecosystems 31(3), 677-684.
| Crossref | Google Scholar |
Hayes, M. C., Gray, P. C., Harris, G., Sedgwick, W. C., Crawford, V. D., Chazal, N., Crofts, S., and Johnston, D. W. (2021). Drones and deep learning produce accurate and efficient monitoring of large-scale seabird colonies. Ornithological Applications 123(3), duab022.
| Crossref | Google Scholar |
IUCN (2025). Pteropodidae (spatial data). The IUCN Red List of Threatened Species 2024(2). Available at https://www.iucnredlist.org [accessed 17 February 2025].
Kingston, T., Florens, F. B. V., and Vincenot, C. E. (2023). Large Old World fruit bats on the brink of extinction: causes and consequences. Annual Review of Ecology, Evolution, and Systematics 54, 237-257.
| Crossref | Google Scholar |
Lachman, D., Conway, C., Vierling, K., and Matthews, T. (2020). Drones provide a better method to find nests and estimate nest survival for colonial waterbirds: a demonstration with Western Grebes. Wetlands Ecology and Management 28(5), 837-845.
| Crossref | Google Scholar |
Lindenmayer, D. B., Gibbons, P., Bourke, M., Burgman, M., Dickman, C. R., Ferrier, S., Fitzsimons, J., Freudenberger, D., Garnett, S. T., Groves, C., Hobbs, R. J., Kingsford, R. T., Krebs, C., Legge, S., Lowe, A. J., Mclean, R., Montambault, J., Possingham, H., Radford, J., Robinson, D., Smallbone, L., Thomas, D., Varcoe, T., Vardon, M., Wardle, G., Woinarski, J., and Zerger, A. (2012). Improving biodiversity monitoring. Austral Ecology 37(3), 285-294.
| Crossref | Google Scholar |
Lindenmayer, D., Woinarski, J., Legge, S., Southwell, D., Lavery, T., Robinson, N., Scheele, B., and Wintle, B. (2020). A checklist of attributes for effective monitoring of threatened species and threatened ecosystems. Journal of Environmental Management 262, 110312.
| Crossref | Google Scholar |
Lopes, C., Firth, C., and Laurance, S. G. W. (2024). Occupancy of urban roosts by spectacled flying-foxes (Pteropus conspicillatus) is not affected by diurnal microclimate. Austral Ecology 49(2), e13487.
| Crossref | Google Scholar |
Lyons, M. B., Brandis, K. J., Murray, N. J., Wilshire, J. H., McCann, J. A., Kingsford, R. T., and Callaghan, C. T. (2019). Monitoring large and complex wildlife aggregations with drones. Methods in Ecology and Evolution 10(7), 1024-1035.
| Crossref | Google Scholar |
Marchowski, D. (2021). Drones, automatic counting tools, and artificial neural networks in wildlife population censusing. Ecology and Evolution 11(22), 16214-16227.
| Crossref | Google Scholar |
McCarthy, E. D., Martin, J. M., Boer, M. M., and Welbergen, J. A. (2021). Drone-based thermal remote sensing provides an effective new tool for monitoring the abundance of roosting fruit bats. Remote Sensing in Ecology and Conservation 7(3), 461-474.
| Crossref | Google Scholar |
McCarthy, E. D., Martin, J. M., Boer, M. M., and Welbergen, J. A. (2022). Ground-based counting methods underestimate true numbers of a threatened colonial mammal: an evaluation using drone-based thermal surveys as a reference. Wildlife Research 50(6), 484-493.
| Crossref | Google Scholar |
McConkey, K. R., and Drake, D. R. (2006). Flying foxes cease to function as seed dispersers long before they become rare. Ecology 87(2), 271-276.
| Crossref | Google Scholar |
Norris, E. B. B., and Larson, J. (2025). Thermal drones are highly effective for detecting elusive Bennett’s tree kangaroos (Dendrolagus bennettianus) in Australia’s tropical rainforests. Australian Mammalogy 47(1), AM24053.
| Crossref | Google Scholar |
Parsons, J. G., Robson, S. K. A., and Shilton, L. A. (2011). Roost fidelity in spectacled flying-foxes Pteropus conspicillatus: implications for conservation and management. In ‘Biology and Conservation of Australasian Bats. Vol. 35. (Eds L. Bradley, E. Peggy, L. Daniel, L. Lindy.) pp. 66–71. (Royal Zoological Society of New South Wales: Sydney, NSW, Australia)
Rahman, D. A., Setiawan, Y., Rahman, A. A. A. F., and Martiyani, T. R. (2021). Javan langur responses to the repeated exposure of ground survey and novel stimulus, unmanned aerial vehicles. IOP Conference Series: Earth and Environmental Science 948, 012006.
| Crossref | Google Scholar |
Reeder, D. M., Kosteczko, N. S., Kunz, T. H., and Widmaier, E. P. (2006). The hormonal and behavioral response to group formation, seasonal changes, and restraint stress in the highly social Malayan Flying Fox (Pteropus vampyrus) and the less social Little Golden-mantled Flying Fox (Pteropus pumilus) (Chiroptera: Pteropodidae). Hormones and Behavior 49(4), 484-500.
| Crossref | Google Scholar |
Roberts, B., Eby, P., and Westcott, D. (2020). Pteropus conspicillatus. The IUCN Red List of Threatened Species 2020: e.T18721A22080456. https://dx.doi.org/10.2305/IUCN.UK.2020-3.RLTS.T18721A22080456.en
Seegobin, V. O., Oleksy, R. Z., and Florens, F. B. V. (2024). Foraging habitat quality of an Endangered mass-culled flying fox is reduced by alien plant invasion and improved by alien plant control. Journal for Nature Conservation 78, 126569.
| Crossref | Google Scholar |
Vallecillo, D., Gauthier-Clerc, M., Guillemain, M., Vittecoq, M., Vandewalle, P., Roche, B., and Champagnon, J. (2021). Reliability of animal counts and implications for the interpretation of trends. Ecology and Evolution 11(5), 2249-2260.
| Crossref | Google Scholar |
Virtue, J., Turner, D., Williams, G., Zeliadt, S., Walshaw, H., and Lucieer, A. (2023). Burrow-nesting seabird survey using UAV-mounted thermal sensor and count automation. Drones 7(11), 674.
| Crossref | Google Scholar |
Weimerskirch, H., Prudor, A., and Schull, Q. (2018). Flights of drones over sub-Antarctic seabirds show species- and status-specific behavioural and physiological responses. Polar Biology 41(2), 259-266.
| Crossref | Google Scholar |
Welbergen, J. A. (2006). Timing of the evening emergence from day roosts of the grey-headed flying fox, Pteropus poliocephalus: The effects of predation risk, foraging needs, and social context. Behavioral Ecology and Sociobiology 60(3), 311-322.
| Crossref | Google Scholar |
Westcott, D., and McKeown, A. (2004). Observer error in exit counts of flying-foxes (Pteropus spp.). Wildlife Research 31(5), 551-558.
| Crossref | Google Scholar |
Westcott, D. A., Fletcher, C. S., McKeown, A., and Murphy, H. T. (2012). Assessment of monitoring power for highly mobile vertebrates. Ecological Applications 22(1), 374-383.
| Crossref | Google Scholar |
Westcott, D. A., Caley, P., Heersink, D. K., and McKeown, A. (2018). A state-space modelling approach to wildlife monitoring with application to flying-fox abundance. Scientific Reports 8, 4038.
| Crossref | Google Scholar |
Witt, R. R., Beranek, C. T., Howell, L. G., Ryan, S. A., Clulow, J., Jordan, N. R., Denholm, B., and Roff, A. (2020). Real-time drone derived thermal imagery outperforms traditional survey methods for an arboreal forest mammal. PLoS One 15, e0242204.
| Crossref | Google Scholar |