Cretaceous depositional environment interpretation of offshore Otway Basin cores and wireline logs; application to the generation of basin-scale gross depositional environment maps
Chris Cubitt A § * , Steve Abbott A , George Bernardel A , Merrie-Ellen Gunning A , Duy Nguyen A , Chris Nicholson A and Alan Stoate AA Geoscience Australia, Canberra, ACT, Australia.
The APPEA Journal 63 S215-S220 https://doi.org/10.1071/AJ22090
Accepted: 20 February 2023 Published: 11 May 2023
© 2023 The Author(s) (or their employer(s)). Published by CSIRO Publishing on behalf of APPEA. This is an open access article distributed under the Creative Commons Attribution 4.0 International License (CC BY).
Abstract
Gas production from the Inner Otway Basin commenced in the early 2000s but the deep-water part of this basin remains an exploration frontier. Historically, the understanding of plays in this region were largely model driven and therefore the ground-truthing of depositional environments (DE) and gross depositional environments (GDE) are critical. This aspect has been investigated for the Sherbrook Supersequence (SS) by the integration of legacy wireline and core data, with regional 2D seismic facies mapping of new and reprocessed data from Geoscience Australia’s 2020 Otway Basin seismic program. Core observations were matched to wireline logs and seismic facies with resulting well-based DE interpretations calibrated to seismic resolution Regional GDE intervals. Integration of well and seismic observations led to the compilation of a basin-wide Regional GDE map for the Sherbrook SS. This GDE map indicates the distribution of Sherbrook SS play elements such as source rock, seal and reservoir, especially across the Deep-water Otway Basin where well data is sparse.
Keywords: Australia, core, delta front, depositional environment, gross depositional environment, mouthbar, offshore Otway Basin, shelf, Sherbrook Supersequence, tidal, wireline log.
Introduction
The Late Cretaceous succession of the Otway Basin (Fig. 1a) includes some of the key hydrocarbon producing intervals in southern Australia. This study has focussed on the Upper Cretaceous Sherbrook Supersequence (SS) forming part of the ongoing interpretation of newly acquired regional 2D seismic and reprocessed data as part of the 2020 Otway Basin seismic program (Nicholson et al. 2022). This abstract investigates how the use of archival cores supports the formulation of the upper Sherbrook SS gross depositional environment (GDE) map (Fig. 1b).
(a) Otway Basin stratigraphic column excerpt from Krassay et al. (2004). (b) Map of the Otway Basin in southern Australia showing structural elements, wells and gas fields. The distribution of Sherbrook SS Regional GDEs (RGDEs) are from Abbott et al. (2023). Highlighted are wells with interpreted Sherbrook SS cores.
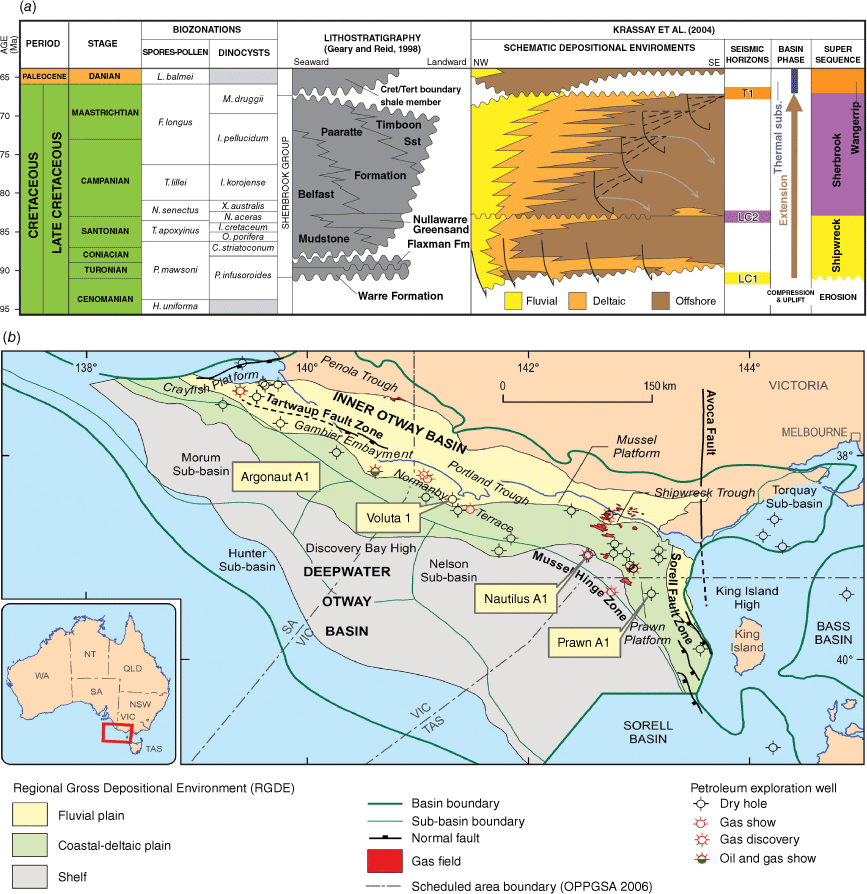
Depositional environments (DEs) and gross depositional environments (GDEs) were interpreted from 18 Sherbrook SS cores from four widely separated offshore wells (Argonaut A1, Voluta 1, Nautilus A1 and Prawn A1 – Fig. 1b). Wireline log motifs were used to extend DE and GDE interpretation across the entire Cretaceous succession in each well. The integration of well-based DEs with basin-wide seismic facies interpretation has resulted in the generation of basin-scale GDE maps, such as that developed for the Sherbrook Supersequence (SS) (Fig. 1b) by Abbott et al. (2023).
Methods
Archival cores from offshore Otway Basin wells were accessed from Geoscience Australia’s Repository. The cores were in good condition with very little discolouration, oxidation or clay swelling (Fig. 2b–f) despite having been in storage for in excess of 50 years. Fig. 2 also shows the physical nature of the cores which are typically slabbed into 3D pieces. The good condition of most cores, along with a stratigraphic spread across the Sherbrook SS, gave confidence that the 80 m of coverage was sufficient to document wireline depositional signatures. The comparison of sedimentary features in Sherbrook SS core with the 407 m of Shipwreck SS core logged as part of a separate study yielded additional interpretation confidence.
Examples of a core log and examples of Sherbrook SS cores, all from Voluta 1. Note that all images display core depth. The red and blue stars indicate the location of core (c) and (e) images relative to the core log in (a). The vertical red line in (a) shows the extent of cores illustrated in (b). (a) Core log extract from the Sherbrook SS section in Voluta 1 [Core 9]. (b) Cores displaying 3D slabbing and good preservation. (c) Image of a sandy heterolithic core showing regular mudstone interbeds and a diverse (and moderately intense) bioturbation assemblage. (d) Sandy heterolithic core showing a diverse bioturbation assemblage including a large Ophiopmorpha burrow. (e) Transgressive surface consisting of a mud-supported conglomerate and associated exposure (?) colouration transitioning upwards into oxidised (layered) mudstone. (f) Muddy heterolithic core dominated by lenticular bedding with common mm-scale couplets, simple bioturbation and Thalassinoides burrows that have been passively infilled with green glauconitic sand.
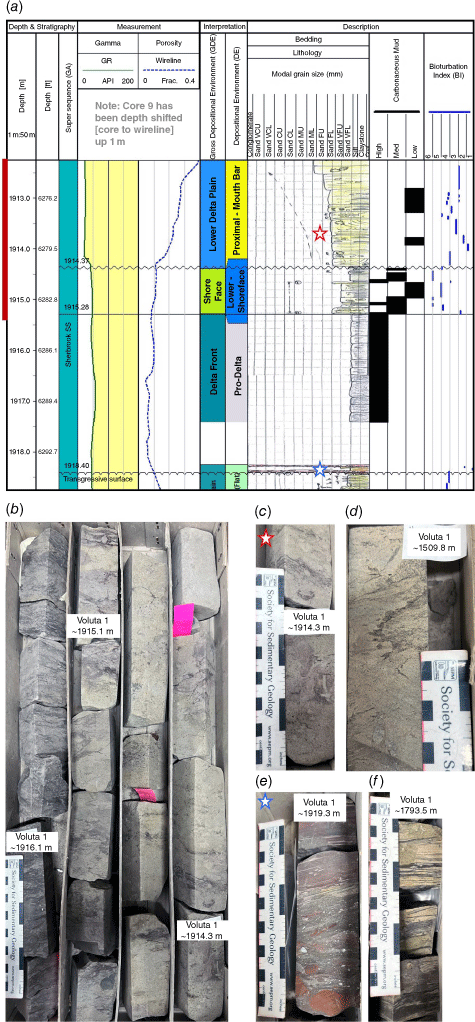
Central to core logging was grainsize and lithology determinations. Grainsize (Canadian stratigraphic services (Canstrat) 2000) was determined for individual bedsets using a hand lens and stereo-microscope. Sedimentary structures, bioturbation index, trace fossil diversity and clay content were also recorded (Fig. 2a). On the core logs grainsize coarsens to the left mimicking the gamma ray curve (Fig. 2a), linking wireline log motifs to lithology and DE/GDE interpretations. Depth matching of cores was achieved using the relationship between the gamma ray curve, core plug data, grain size and lithology. Wireline log motifs were applied to interpret DEs and GDEs for the non-cored Sherbrook SS in each well.
DEs are defined as packages of sediment that have formed in specific geological conditions (i.e. a mouthbar or a delta front). These packages of sediment have been defined according to a set of criteria which include grain size trends, lithology assemblages, sedimentary features, bioturbation, as well as depositional package juxtaposition and regional context (Table 1). Genetically related DEs were grouped into GDEs (Fig. 2a). For example, a lower delta plain GDE may include flood plain, crevasse splay, distributary channel and mouth bar DEs.
Depositional environments (DEs) | DE interpretation criteria | Gross depositional environments (GDEs) |
Proximal and distal delta front DEs | Proximal delta fronts (PDF): sandstone-dominated intervals associated with simple trace fossil assemblages, trace fossil kill events and evidence of terrestrial influence (i.e. organic chaff, woody material). | Delta front GDE |
Distal delta front deposits: claystone/heterolithic-dominated intervals with common lenticular bedding, diverse bioturbation and suspension deposition. | ||
Inner and outer shelf DEs | Inner shelf: mudstone-dominated with weak and massive bedding. Grain flows may be present and simple low-intensity bioturbation is typical. | Shelf GDE |
Outer shelf: Mudstone, commonly massive, with rare laminations and trace fossils. | ||
Proximal mouth bar DE | Proximal mouth bar: coarsening upwards, sand-dominated intervals with massive, planar and horizontal bedding common. If an abandonment section exists, then bioturbation is a common feature. | Lower delta plain GDE |
GDEs are a collection of genetically related DEs.
Results and discussion
Heterolithic and to a lesser extent sandstone and mudstone cores display a diverse set of sedimentary features (Fig. 2b–f). Common to most Sherbrook SS cores were sedimentary features such as sand-mud couplets (Fig. 2f), syneresis cracks, rip up clasts (Fig. 2b) and micro-faulting. Although observed in Prawn A1, Nautilus A1 and Voluta 1, shell hash is rare. Bedding styles observed in all four wells include horizontal bedding and planar cross-bedding in sandstones (Fig. 2b), whilst massive and laminated bedding were typical in mudstones (Fig. 2e). Ripple (wave and current), flaser and lenticular bedding were commonly noted in heterolithic intervals (Fig. 2f).
DEs and GDEs were interpreted in Sherbrook SS core intervals (Table 1). The most common of the 18 DEs recognised are proximal delta front, distal delta front, proximal mouth bar, inner shelf and outer shelf. Grouping of genetically related DE resulted in seven GDEs of which lower delta plain, delta front and shelf are the most common. Fig. 1b reveals that the upper Sherbrook SS intervals at Argonaut A1, Voluta 1 and Prawn A1 are situated at the transition between fluvial and coastal/delta plain Regional GDEs (or RGDEs as defined in Abbott et al. 2023), whilst the section at Nautilus A1 is firmly placed in a shelfal location (Fig. 1b). In all four wells, good agreement was observed between core, wireline and seismic facies observations which gives confidence that this map reasonably reflects the depositional nature of the upper Sherbrook SS.
The 80 m of Sherbrook SS cores constitute a representative dataset despite the modest meterage. This is because multiple regularly spaced cores exist in all four wells. Voluta 1, for example, has seven regularly spaced Sherbrook SS cores (Fig. 3a). This core spacing reduces uncertainty in the assignment of DE interpretations to wireline log responses. Once depth-calibrated with the core, the wireline dataset was interpreted with respect to DE and GDE. For example, the wireline log signature in Voluta 1 over the (tidal) lower delta plain core interval (Fig. 3c) was defined and propagated to other intervals with a similar log signature. This same approach is illustrated in the Nautilus A1 section (Fig. 3b, d), whereby the shelf mudstone log signature was assigned to similar intervals in the absence of core control. This approach was used to interpret wireline responses in non-cored intervals of the upper Sherbrook SS across the basin.
Integrated Sherbrook SS core and wireline log interpretations from selected wells. (a) Voluta 1 core-controlled wireline DE/GDE interpretation. (b) Nautilus A1 core-controlled wireline DE/GDE interpretation. (c) Voluta 1 core showing a sandy heterolithic interval with tidal features such as mud drapes and mm-scale couplet laminae. (d) Nautilus A1 core showing massive mudstone. Note: both images display core depth and arrows indicate their respective well locations. Note: all core labels are in drillers depth.
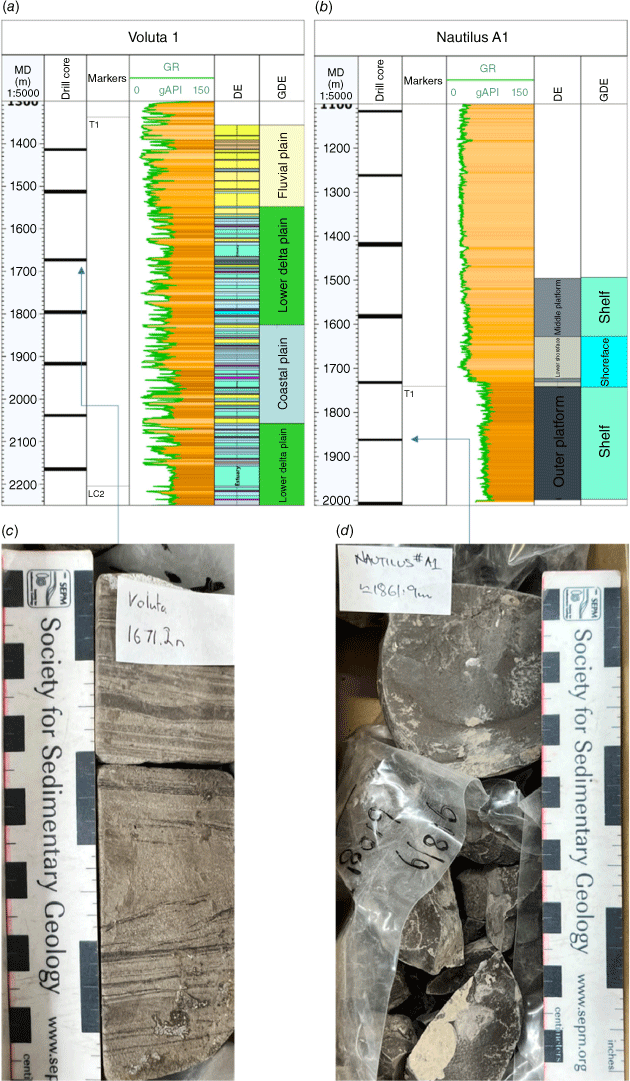
GDE interpretations from core and wireline logs were integrated with seismic facies at the basin scale (Abbott et al. 2023). As the number of regionally mappable seismic facies was less than the number of GDEs, genetically related GDE were grouped to formulate Regional GDE (RGDE). The integration of seismic facies and well-based GDE interpretation yielded a basin-scale GDE map of the upper Sherbrook SS (Fig. 1b).
Conclusions
Investigation of archival Sherbrook SS cores from four wells across the Otway Basin revealed 18 DEs and 7 GDEs. The most common DEs include proximal delta front, outer shelf, distal delta front, proximal mouth bar and inner shelf. Sherbrook SS GDE intervals were mostly deposited in coastal plain, lower delta plain and shelfal settings. DE/GDE wireline log signatures derived from core interpretations were applied to non-cored intervals across the basin. Integration of well-based interpretations with seismic facies led to the development of an upper Sherbrook SS RGDE map. The work presented here represents a step forward in the understanding of plays in the offshore Otway Basin. For example, the presence of thick intervals of marine mudstone associated with the Shelf GDE across the Deep-water Otway Basin indicates the potential for productive source rocks and effective cross-fault seal in these areas.
Data availability
Well data were derived from well completion reports accessible via the NOPIMS portal, and Nguyen et al. (2022). Core log data including grainsize, lithology, net-to-gross, bioturbation intensity, sedimentary features and comments along with DE/GDE interval data will be made available via an upcoming Geoscience Australia data release.
Acknowledgements
We thank Geoscience Australia’s Repository for core handling and management of data. We thank Barry Bradshaw and Adam Bailey for providing constructive reviews. This paper is published with the permission of the CEO, Geoscience Australia.
References
Abbott S, Cubitt C, Bernardel G, Nicholson C, Nguyen D (2023) Towards a regional understanding of Sherbrook Supersequence Gross Depositional Environments, offshore Otway Basin. In ‘4th AEGC Conference’, 13–18 March 2023, Brisbane, Australia. eCat #147395. (Geoscience Australia)Canadian stratigraphic services (Canstrat) (2000) Grain Size Cards. Available at https://www.canstrat.com
Krassay A, Cathro D, Ryan D (2004) A regional tectonostratigraphic framework for the Otway Basin. In ‘Eastern Australasian Basins Symposium II’, 19–22 September 2004. (Eds PJ Boult, DR Johns, SC Lang) pp. 97–116. (Petroleum Exploration Society of Australia: Adelaide)
Nguyen D, Edwards DS, Gunning M-E, Bernardel G (2022) The Northwest Offshore Otway Basin Well Folio. The APPEA Journal 62, S461–S466.
| The Northwest Offshore Otway Basin Well Folio.Crossref | GoogleScholarGoogle Scholar |
Nicholson C, Abbott S, Bernardel G, Gunning M-E (2022) Stratigraphic framework and structural architecture of the Upper Cretaceous in the Deep-water Otway Basin – implications for frontier hydrocarbon prospectivity. The APPEA Journal 62, S467–S473.
| Stratigraphic framework and structural architecture of the Upper Cretaceous in the Deep-water Otway Basin – implications for frontier hydrocarbon prospectivity.Crossref | GoogleScholarGoogle Scholar |
§ Chris Cubitt is now Deputy Director at Exploration Geoscience, Department for Energy and Mining, South Australia.
![]() Chris Cubitt graduated from the University of Adelaide with a PhD in Diagenesis and Sedimentology. He has over 20 years of industry experience in reservoir and regional geology working basins worldwide. He joined Geoscience Australia as a Basin Analyst in 2021 and recently joined South Australia’s Department for Energy and Mining (DEM) as a Deputy Director. |
![]() Steve Abbott joined Geoscience Australia in 2013 where he works as a Basin Analyst on prospectivity studies of Australia’s offshore basins. He earned his qualifications in sedimentary geology from Flinders (Hons, 1987) and James Cook Universities (PhD 1994). |
![]() George Bernardel is a Basin Analyst at Geoscience Australia. He gained his BSc (Honours) in Geophysics from the University of Sydney in 1986. He joined Geoscience Australia in 1995 where he works on prospectivity studies of Australia’s offshore basins. |
![]() Merrie-Ellen Gunning has over 20 years of experience in the oil and gas industry where she has performed a diverse range of roles from strategic planning, business development and operations management, to technical roles specialising in geophysics and exploration. She has an MBA from Melbourne Business School and a Bachelor of Applied Science in Geology. Merrie-Ellen joined Geoscience Australia in 2015 where she works on prospectivity studies of Australia’s offshore basins. She is currently Director of Offshore Energy Systems. |
![]() Duy (Victor) Nguyen holds BE and ME (Petroleum Engineering) degrees from the University of New South Wales. Duy has 5 years of oil and gas industrial experience. Since 2009, Duy has worked as a Petroleum Engineer at Geoscience Australia assessing the CO2 storage potential and petroleum prospectivity of Australia’s offshore basins. |
![]() Chris Nicholson joined Geoscience Australia in 2004 as a Basin Analyst working on prospectivity studies of Australia’s offshore basins. Chris graduated with a BSc (Hons) in Geology from the Australian National University in 2000. |
![]() Alan Stoate received a BSc (Geology and Geophysics)/BEng (Civil & Structural) as well as a Bachelor of Computer Science from the University of Adelaide in 2018. He joined Geoscience Australia in 2021 where he works on prospectivity studies of Australia’s offshore basins. |