Modelling of hydrogen gas generation from overmature organic matter in the Cooper Basin, Australia
Christopher J. Boreham

A Geoscience Australia, GPO Box 378, Canberra, ACT 2601, Australia.
B Murray Partners PPSA Pty. Ltd., Perth, Australia.
C GEOS4 GmbH, D‐14552 Michendorf, Germany.
The APPEA Journal 63 S351-S356 https://doi.org/10.1071/AJ22084
Accepted: 10 February 2023 Published: 11 May 2023
© 2023 The Author(s) (or their employer(s)). Published by CSIRO Publishing on behalf of APPEA. This is an open access article distributed under the Creative Commons Attribution 4.0 International License (CC BY)
Abstract
A significant portion of planned energy and mineral resource investment into Australia is now for hydrogen (H2). Whether from fossil fuels with carbon capture and storage or from electrolysis of water using renewable energy, there is a price premium for manufactured hydrogen. The production of H2 from geological sources (geologic H2) could be more cost-effective. The majority of sources for geologic H2 are abiotic and their resource potential is largely unknown. Biogenic (microbial and thermogenic) sources also exist. The focus for this study is on a thermogenic source where chemical kinetics of H2 generation from the thermal breakdown of land-plant-derived organic matter has been applied within a petroleum system modelling framework for the Cooper Basin. Modelling of mid-Patchawarra Formation coals and shales, the main source rocks for petroleum, indicate that free H2 is available at maturities >3.5% vitrinite reflectance and that a large volume of free H2 is predicted to occur in a ‘sweet spot’ deep within the Nappamerri Trough. In-situ free H2 concentrations deep within the Nappamerri Trough are predicted to be comparable to methane concentrations in productive unconventional shale gas plays. Nevertheless, exploration drilling within the Cooper Basin’s depocentre is sparse and a deep H2 system remains largely untested.
Keywords: chemical kinetics of hydrogen generation, Cooper Basin, geologic hydrogen, hydrogen system, natural hydrogen, coal and carbonaceous shale source rock, petroleum system modelling, thermogenic natural gas.
Introduction
Hydrogen gas (H2) is the most energy-rich gas weight-for-weight and is considered crucial for a sustainable clean energy future (International Energy Agency 2022). The production of H2 from geological sources (geologic H2) is increasingly recognised as a low-cost complement to manufactured H2 (Lapi et al. 2022). Although there are numerous sources of geologic H2 within sedimentary, metamorphic and igneous rocks (Boreham et al. 2021), the most studied and effectively modelled are those reactions associated with fluid–rock interactions e.g. ferrous to ferric iron oxidation accompanied by water reduction and the radiolysis of water to produce H2. Calculation of H2 production rates, coupled with a geological understanding, enables estimation of geologic H2 resource potential. The majority of sources for geologic H2 are abiotic and volumetric estimates of H2 generation are mostly qualitative. Biogenic (microbial and thermogenic) sources of H2 also exist. In this paper, the chemical kinetics of H2 generation from coals and carbonaceous shales containing land-plant-derived organic matter has been applied within a petroleum system modelling framework to the Cooper Basin to quantify the generation of H2 in a free state (free H2).
Cooper Basin petroleum generation
The Cooper Basin is a Pennsylvanian–Middle Triassic intracratonic basin containing predominantly terrigenous sediments, which covers an area of 127 000 km2 with sediment thicknesses typically being up to 2500 m, although the base of the section within the deepest trough (Nappamerri) reaches over 4400 m. The overlying Jurassic–Cretaceous Eromanga Basin extends beyond the preserved depositional edge of the Cooper Basin and is over 2500 m thick. The Palaeocene to Quaternary Lake Eyre Basin overlies the Eromanga Basin (Fig. 1a, b; Hall et al. 2019 and references therein) but it is thin (up to 300 m) across the Cooper Basin region.
(a) Areal extent of the Cooper Basin showing the main depocentres, troughs and ridges and the distribution of oil and gas occurrences (note: ‘PSM well’ identifies the location of the well used in the petroleum system model of Hall et al. (2019)) and (b) cross-section showing the distribution of the sedimentary formations of the Cooper and Eromanga basins across the Nappamerri, Patchawarra and Tenappera troughs. Modified after Hall et al. (2019).
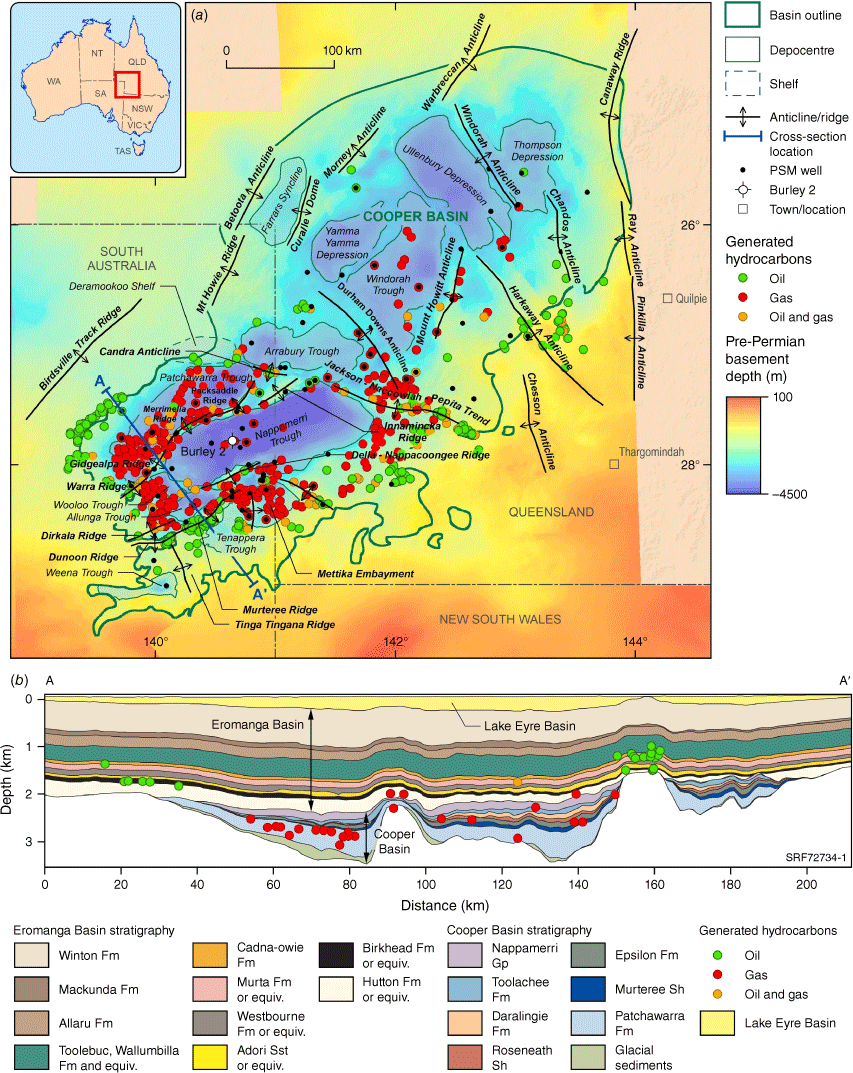
Hydrocarbon generating source rocks are dominated by land-plant-derived organic matter with the Toolachee and Patchawarra formations being considered prospective. Both Permian formations collectively host the main commercial reservoirs for gas in the Cooper Basin (Fig. 1b; Hall et al. 2019 and references therein). Petroleum system modelling indicated that the main phase of hydrocarbon generation began in the latest Permian in the Nappamerri Trough depocentre in South Australia while the mid-Cretaceous corresponded to a time when hydrocarbons were generated in the adjacent troughs, including the Patchawarra and Windorah troughs. Source rock maturity varies between depocentres, with large areas of the Nappamerri, Patchawarra and Windorah troughs being gas mature to overmature at present day (Hall et al. 2016).
Modelling free H2 generation
Modelling of gas generation employed the Zetaware software suite (Kinex version 4.94, Genesis Version 5.68b and Trinity T3 version 6.61). Input parameters from Hall et al. (2019) were used, including basin history (burial, thermal and pressure), source characterisation (net thickness, richness and quality) and the primary methane, late methane and free H2 generation kinetics of Mahlstedt et al. (2022). The maturation history model is shown for Burley 2 (Fig. 2a) because it is a deep well located in the centre of the Nappamerri Trough (Fig. 1a) where the Patchawarra Formation source rocks are overmature (measured vitrinite reflectance (Ro) >2%) with predominantly dry gas generation. The modelled Ro is displayed in Fig. 2a, which also highlights the variation in the vitrinite reflectance kinetic models available in the literature and employed within the Zetaware software suite; for modelling vitrinite reflectance in Fig. 2a, b, this paper uses the ARCO vitrinite reflectance kinetic model (see Falvey and Middleton 1981). Additionally, well histories of Hall et al. (2019) were slightly modified for those wells where measured Ro was >2% to best honour the fit between the modelled and measured Ro profiles over this high-maturity region.
For Burley 2 well (a) modelled and measured vitrinite reflectance (Vitrinite Ro) versus depth, (b) transformation ratio for primary methane, late methane and free H2, and modelled vitrinite reflectance versus modelled temperature and (c) modelled temperature history versus age.
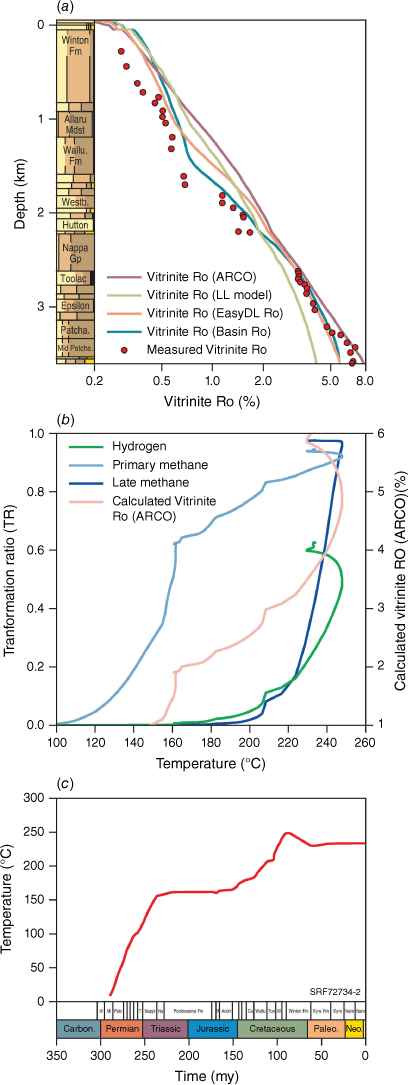
Utilising primary methane, late methane and free H2 generation kinetics (Mahlstedt et al. 2022), the cumulative transformation history (i.e. transformation ratio (TR) from 0 to 1) of a source rock positioned in the middle of the Patchawarra Formation in Burley 2 is modelled and displayed in Fig. 2b. Methane generation is exhausted at the hydrocarbon deadline where TR >0.9, which occurs at the time of maximum paleotemperature, in this case ~250°C and a modelled Ro of ~3.5%, in the Late Cretaceous just prior to the Winton Formation uplift and erosion (Fig. 2b, c). Importantly, at this same time, the transformation of the initial free H2 potential is only ~50% completed (TR ~0.5). Thus, where the Patchawarra Formation source rock experienced higher maturity and temperature, methane-free H2 would be generated.
Employing a 2D surface of a mid-Patchawarra Formation source rock (coal and carbonaceous shale), the cumulative gas generation (m3 gas/m2 rock) for methane (primary + late) and free H2 is mapped in Fig. 3a, b, respectively. Methane generation is prevalent across much of the Cooper Basin, while free H2 generation is predominantly restricted to a ‘sweet spot’ in the central Nappamerri Trough. Interestingly, both methane and free H2 have similar maximum ‘m3 gas/m2 rock’ generation rates. With consideration of rock thickness and a conservative H2 expulsion efficiency of 90%, Mahlstedt et al. (2022) estimated 86 Tcf free H2 gas is still retained, much more must have expelled from the overmature Patchawarra Formation source rocks.
Areal distribution of modelled cumulative gas generation volumes m3/km2 from a mid-Patchawarra Formation source rock for (a) methane (primary + late) and (b) free H2 (the enclosed black line marks the 50 km boundary from the edge of the H2 ‘sweet spot’. Note: initial primary methane potential = 40 mg/g TOC (total organic content), initial late methane potential = 50 mg/g TOC, initial free H2 potential = 20 mg/g TOC (Mahlstedt et al. 2022)) and (c) well location with reported maximum H2 mol% where H2 >0.1 mol% (Supplementary Table S1 lists data from WCRs and five well gases in Boreham et al. (2021). Note: well names for maximum H2 ≥0.8 mol%; Burley 3 with a maximum H2 = 0.82 mol% is for pre-well-fire gas whereas post-well-fire gas has a maximum H2 = 40 mol%). Note: Apply structural elements in Fig. 1 to Fig. 3.
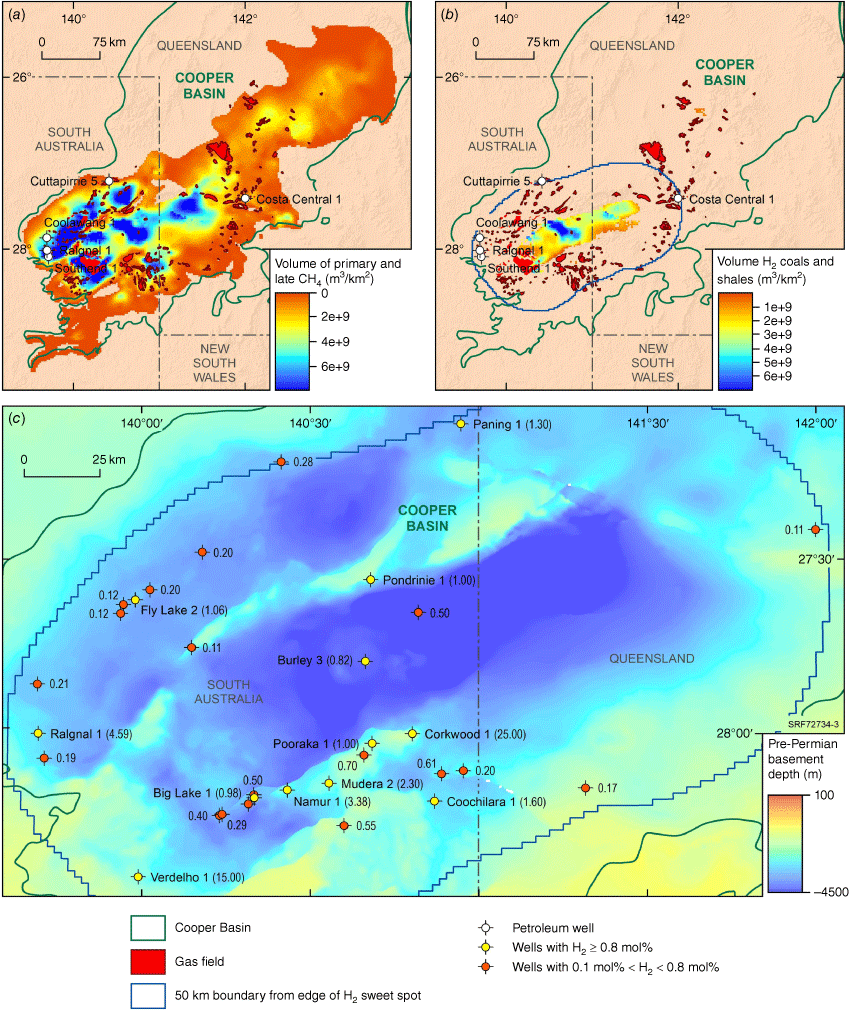
The H2 system
The concentration of H2 in Australian natural gas is typically low with ~80% of the dataset having H2 <0.1 mol%. Nevertheless, natural gases with H2 concentration >1 mol% are found in all Australian states and the Northern Territory (Boreham et al. 2021). In the Cooper Basin, the areal extent of those gases reported in Boreham et al. (2021) shows that the five gases with H2 >0.1 mol% (Table S1) fall either on or within a 50 km boundary extended from the outline of the H2 ‘sweet spot’ in the Nappamerri Trough (Fig. 3b). While the migration and fate of H2 gas is beyond the scope of this paper, if the source of H2 is from the thermal decomposition of organic matter then this may define the extent of the H2 system, consistent with the critical elements of a petroleum system defined by Magoon and Dow (1994).
In order to further constrain and have confidence in the areal extent of an inferred H2 system, well completion reports (WCRs) were consulted for petroleum wells drilled in the Cooper and Eromanga basins. WCRs from 3346 wells were examined with a low proportion (557 wells) containing gas data and a limited number (35 wells and 108 gas analyses) containing H2 mol% values. Of these, 26 wells have gases with H2 >0.1 mol% and 9 of these wells having gases with H2 >1.0 mol% (Fig. 3c) (Table S1). The south-west margin of the Nappamerri Trough hosts H2-rich (>10 mol% H2) natural gases in South Australia where migration of H2 may be up to ~40 km from the edge of the thermogenic H2 generation ‘sweet spot’ (kitchen) (Fig. 3b, c). Natural gases with much lower H2 contents typify the northern extent over the Patchawarra Trough in South Australia where source rock maturities are lower. The lack of wells both fewer in number and without H2 mol% data lends to an uncertain and unconstrained H2 system in Queensland (Fig. 3c).
Summary and conclusions
The need for a reliable and volumetrically significant supply of H2 at the right price is a challenge for the future low-carbon economy. Experience gained over more than a century by petroleum and mineral explorationists guarantee the right tools are now available for the exploration for geologic hydrogen. Geologic hydrogen gas has the advantage over petroleum since it has around 10 times more sub-surface sources and hence greater opportunities, compared to sources of petroleum. Thermogenic hydrogen is seen as a viable candidate in the hydrogen ‘mix’ because an organic-rich, highly overmature rock can be modelled to determine where geologic hydrogen is generated, in this case the central Nappamerri Trough in the Cooper Basin, and hence influence future exploration opportunities and activities.
Acknowledgements
Marita Bradshaw and Tehani Palu are thanked for their reviews of a pre-submission version of the manuscript. Chris Evenden and Silvio Mezzomo provided assistance in drafting the figures. We would like to acknowledge Minerals, Energy and Groundwater Information Services for data compilation. C. J. B., D. S. E. and A. F. publish with the permission of the CEO, Geoscience Australia.
References
Boreham CJ, Edwards DS, Czado K, Rollet N, Wang L, van der Wielen S, Champion D, Blewett R, Feitz A, Henson PA (2021) Hydrogen in Australian natural gas: occurrences, sources and resources. The APPEA Journal 61, 163–191.| Hydrogen in Australian natural gas: occurrences, sources and resources.Crossref | GoogleScholarGoogle Scholar |
Falvey DA, Middleton MF (1981) Passive continental margins: evidence for a prebreakup deep crustal metamorphic subsidence mechanism. In ‘Oceanologica Acta’, 1981. pp. 103–114. Special Issue open access version. Available at https://archimer.ifremer.fr/doc/00246/35699
Hall LS, Palu TJ, Murray AP Boreham CJ, Edwards DS, Hill AJ, Troup A (2016) ‘Cooper Basin Petroleum Systems Modelling: Regional Hydrocarbon Prospectivity of the Cooper Basin: Part 3’. Record 2016/029. (Geoscience Australia: Canberra)
| Crossref |
Hall LS, Palu TJ, Murray AP, Boreham CJ, Edwards DS, Hill AJ, Troup A (2019) Hydrocarbon prospectivity of the Cooper Basin, Australia. AAPG Bulletin 103, 31–63.
| Hydrocarbon prospectivity of the Cooper Basin, Australia.Crossref | GoogleScholarGoogle Scholar |
International Energy Agency (2022) Hydrogen. Available at https://www.iea.org/fuels-and-technologies/hydrogen
Lapi T, Chatzimpiros P, Raineau L, Prinzhofer A (2022) System approach to natural versus manufactured hydrogen: an interdisciplinary perspective on a new primary energy source. International Journal of Hydrogen Energy 47, 21701–21712.
| System approach to natural versus manufactured hydrogen: an interdisciplinary perspective on a new primary energy source.Crossref | GoogleScholarGoogle Scholar |
Magoon LB, Dow WG (1994) ‘The Petroleum System—From Source to Trap’. AAPG Memoir 60. (The American Association of Petroleum Geologists: Tulsa, OK, USA)
| Crossref |
Mahlstedt N, Horsfield B, Weniger P, Misch D, Shi X, Noah M, Boreham C (2022) Molecular hydrogen from organic sources in geological systems. Journal of Natural Gas Science and Engineering 105, 104704
| Molecular hydrogen from organic sources in geological systems.Crossref | GoogleScholarGoogle Scholar |
![]() Christopher J. Boreham is a Principal Organic Geochemist at Geoscience Australia working in the Minerals, Energy and Groundwater Division. He obtained a Ph.D. in Chemistry at the Australian National University. Chris applies his skills to understand the evolution of petroleum and abiogenic gas in Australian basins. Chris is a member of PESA and AAPG. |
![]() Dianne S. Edwards is a Principal Organic Geochemist at Geoscience Australia working in the Minerals, Energy and Groundwater Division. She received her Ph.D. from the University of Adelaide in 1996. Dianne’s focus is on building databases and web services to release geochemical information that underpins Australia’s petroleum systems. Dianne is a member of PESA. |
![]() Andrew J. Feitz is an Environmental Engineer and Director of Low Carbon Geoscience and Advice at Geoscience Australia. He holds a Ph.D. from UNSW and worked as a senior researcher at The University of New South Wales (UNSW) and the Karlsruhe Institute of Technology (Germany). Andrew leads GA’s efforts supporting implementation of the National Hydrogen Strategy. |
![]() Andrew Murray is a director of Murray Partners PPSA and provides training and consulting in petroleum system analysis. He holds M.Sc. and Ph.D. degrees in Petroleum Geochemistry and was head of the discipline at Woodside Energy for 18 years. |
![]() Nicolaj Mahlstedt is a Petroleum System Analyst at GEOS4 GmbH and holds a Ph.D. in Organic Geochemistry from the Technical University (TU) of Berlin. He has 10+ years’ experience in upstream research and development. His major interests include petroleum system analysis in conventional as well as unconventional settings, and compositional kinetic modelling. |
![]() Brian Horsfield is CEO and Managing Director of GEOS4, a geochemistry research and service provider for the upstream energy sector. He is an elected member of the German Academy of Science and Technology, and is Emeritus Professor of Organic Geochemistry and Hydrocarbon Systems at TU Berlin. |