Changes in parasite species distributions could be driven by host range expansions: the case of hybridisation between two Australian reptile ticks
Bridgette Barnden A , Amy L. Slender

A College of Science and Engineering, Flinders University, GPO Box 2100, Adelaide, SA 5001, Australia.
B Evolutionary Biology Unit, South Australian Museum, North Terrace, Adelaide, SA 5000, Australia.
Australian Journal of Zoology 70(5) 153-163 https://doi.org/10.1071/ZO23010
Submitted: 15 March 2023 Accepted: 24 April 2023 Published: 24 May 2023
© 2022 The Author(s) (or their employer(s)). Published by CSIRO Publishing. This is an open access article distributed under the Creative Commons Attribution-NonCommercial-NoDerivatives 4.0 International License (CC BY-NC-ND)
Abstract
Anthropogenic stressors such as climate change and diminishing habitats cause widespread disturbances in species distributions for a variety of taxa. Range shifts and hybridisation following secondary contact become of particular importance when it comes to parasites such as ticks, as they are dependent on their host distributions and can carry numerous harmful pathogens. We aimed to determine if two parapatric reptile tick species, Amblyomma albolimbatum and A. limbatum, hybridise at their parapatric boundary by comparing morphological and genomic variation using single nucleotide polymorphisms. Our results show that both tick species were genomically distinct, but hybridisation has occurred. We found a hybrid and an A. limbatum outside of their previously known range, suggesting there could have been a shift in tick distribution. What were thought to be hybrids due to intermediate morphological traits were not hybrids but morphological variants of the parental species. This information suggests more distinctive morphological features are needed for identifying these tick species and that novel environmental conditions and a broadening of tick niche due to hybridisation could increase host exposure to different pathogens.
Keywords: climate change, Egerniinae (Tiliquini), genomics, hybridisation, parapatry, SNPs, Squamata, ticks.
Introduction
Climate change has the potential to drastically alter how species are distributed across the globe (Engler et al. 2013). Although many species with specific niches may struggle to acclimatise to changing conditions – becoming locally extinct or facing a reduction of habitat availability (Ogston et al. 2016; Hermes et al. 2018) – others are able to persist (Brauer et al. 2023) or even expand their ranges (McQuillan and Rice 2015). Monitoring changes in species distributions can be useful for recognising cases where climate change has resulted in species becoming endangered or invasive (Beever et al. 2016; Carlson et al. 2017). Greater importance should be attributed to understanding the geographical ranges of particular species such as pathogen transmitting parasites (Dehhaghi et al. 2019).
Pathogen carrying parasites, such as ticks, pose a substantial economic risk (Lynen et al. 2008). These pathogens cause human illness, plus the loss of livestock and wildlife. Instances where tick prevalence has increased, or tick distribution has changed, resulted in tick-borne pathogens directly increasing occurrences of inflammatory illnesses, allergies, anaemia, and autoimmune diseases in humans (Estrada-Peña et al. 2012; Dehhaghi et al. 2019). As parts of the world succumb to longer and hotter summers, drier winters, or increased humidity, living conditions for some pathogen carrying parasites – including ticks – have become more favourable, allowing them to expand their ranges (Sungirai et al. 2015), introducing pathogens into novel environments (Dehhaghi et al. 2019).
After hatching from eggs, ticks go through three transformative life stages – larva, nymph and adult. Ticks first require a blood meal before they drop into the environment to moult into their next life stage. The need for ticks to quest for a new host means ticks are not only dependent on the host environment but are sensitive to abrupt changes in their environment. Many tick species have plastic morphological and life history traits that help them deal with short term changes in biotic (host contact, vegetation gradients) and abiotic (soil moisture, temperature, rainfall) environments (Alkishe et al. 2021). While this plasticity aids ticks with survival and endurance during short term environmental variability, changing host distributions and microhabitat fluctuations, along with climate changes have led to the expansion, contraction and change in distribution for a wide range of ixodid (hard ticks) species (Ostfeld and Brunner 2015; Sonenshine 2018). The contraction of parasite ranges under hotter climate conditions (Oliveira et al. 2017) could be directly beneficial for humans in instances where declines in pathogens, such as malaria, are predicted to follow (Léger et al. 2013). However, in parts of the world where conditions are becoming more favourable to parasites such as ticks, the prevalence of both the parasite and its pathogens have increased. For example, tick prevalence in the USA has increased, resulting in more cases of spotted fever and Lyme disease (Alkishe et al. 2021). The stark differences in possible changes to tick distributions and lack of large scale distributional data of ticks have made it difficult to understand the overall impacts of tick-borne pathogens on a global scale (Léger et al. 2013).
Shifts in tick distributions not only affect their hosts via the pathogens they carry but also affect other tick species that they encounter. Some tick species are known to have parapatric distributions, creating species boundaries that have abutting ranges with a small zone of overlap (Bull and Burzacott 2001). Parapatry is often thought to be maintained through factors like resource competition and reproductive incompatibilities (Andrews et al. 1982), but can also be influenced by other ecological features, such as ecotones, microhabitats, and interspecific competition (Bull 1991). Boundaries such as these can be highly sensitive to a changing environment with large climate events, like sudden rainfall. Environmental changes could favour one species over another, allowing them to flourish and their distribution to expand (Sutherst 1987). Monitoring parapatric species where climate events result in changes to species distribution, population growth and/or boundary movements is useful for understanding the effects of prolonged climate change (Harr and Price 2014; Taylor et al. 2015; Hunter et al. 2017).
Hybrid zones form under two conditions when interspecies reproductive incompatibility maintains parapatry (Sutherst 1987). First, environmental changes can cause shifts in the natural distribution of allopatric species that then make secondary contact (Harper and Hart 2007; Pfäffle et al. 2014). Second, species can diverge along a habitat gradient or cline (Barton and Hewitt 1985) until they become mostly segregated with a small contiguous overlap of ranges (Mayr 1970; Bull 1991). Parapatric boundaries are thought to be more common in natural systems than currently documented (Taylor et al. 2015). It can be difficult to observe abutting boundaries between morphologically similar species, as commonly seen between many bird species (Mayr 1963; Bull 1991). Hybridisation along abutting boundaries has been reported in ticks on several occasions (Oliver et al. 1972; Bournez et al. 2015; Sungirai et al. 2017). The potential for ticks to hybridise along a parapatric boundary can impact host health, as hybrids often carry novel and highly transmissible diseases (King et al. 2015).
Hybrid zones typically have three effects on species boundaries – the first being the erosion of traits that make a species unique and the boundary between the two species becoming blurred, eventually leading to complete introgression. Second, hybrids can persist within a particular location, potentially forming a population of their own, through phenomena such as hybrid swarms (Hasselman et al. 2014). These events would be particularly favoured if there were low levels of migration between the parental species and a hybrid population or if hybrids were less vulnerable to environmental changes such as climate (Brauer et al. 2023). Lastly, the hybrid zone may be strongly influenced by an unrelated ecological feature, such as an ecotone, and so the hybrids will have little to no effect on the boundary (Theodosopoulos et al. 2019). In all cases, hybridisation might influence the morphology of the parental species, and distinguishing between hybrid morphology and parental phenotypes can be difficult (Abbott et al. 2016). Identifying parental lineage is therefore made easier by next-generation sequencing and techniques such as ddRAD (Peterson et al. 2012) and DArTseq™ assays (Kilian et al. 2012).
This research focuses on two species of Australian reptile ticks (Amblyomma albolimbatum and Amblyomma limbatum), which occur on the sleepy lizard (Tiliqua rugosa) host. The parapatric boundary between these two tick species, and a third (Bothriocroton hydrosauri) was first discovered over 40 years ago (Smyth 1973). Since then, one section of the parapatric boundary between A. limbatum and B. hydrosauri in central South Australia has been continuously studied, generating much of the knowledge we currently have on parapatric systems (Godfrey and Gardner 2017). Aiming to add to this knowledge, we focus on a different area of the parapatric boundary located on the north-eastern Eyre Peninsula in South Australia. Here we study the parapatry between A. albolimbatum and A. limbatum to determine if these two species form hybrids, which may provide insights into how their parapatric boundary is maintained. This boundary has received nominal research since its original survey published in 1973 (Smyth 1973); however, there have been reports of individual ticks in this area displaying intermediate characters between the two Amblyomma species (Sharrad 1979). Laboratory studies also confirm the attraction of A. albolimbatum and A. limbatum to non-conspecific pheromones (Andrews et al. 1982); however, this has not been observed in the field. We hypothesise that A. albolimbatum and A. limbatum hybridise along their parapatric boundary. We investigated the efficacy of both morphological and genomic data in detecting the potential hybrids.
Materials and methods
Study site and tick collection
Ticks were sampled from sleepy lizards of the north-eastern Eyre Peninsula (Fig. 1). Surveys were completed from October to December 2018 during the austral spring/summer when lizards are known to be active. Surveys were designed to catch ticks both adjacent and perpendicular to the previously predicted parapatric boundary between the two tick species (Smyth 1973) to determine the extent of the potential hybrid zone. Ticks were also sampled north (Port Augusta) and south (Cowell) of the predicted boundary, to ensure samples from unhybridised individuals were obtained. Sleepy lizards were opportunistically captured by random encounter on the roadside while driving or walking along the roads within the study area. Lizards were processed by marking individuals with unique toe clip combinations, and counting and removing their ticks. Ticks were stored in 95% ethanol.
Map of the study site on the north-eastern Eyre Peninsula, South Australia showing geographical locations where ticks were found. Coloured points represent samples used in this study. The symbol shape represents the species of tick determined from the genomic results – Amblyomma albolimbatum (yellow circles), Amblyomma limbatum (blue triangles) and the hybrids (red diamonds). Black points represent previously recorded locations of A. albolimbatum (circle) and A. limbatum (triangle) from Smyth (1973).
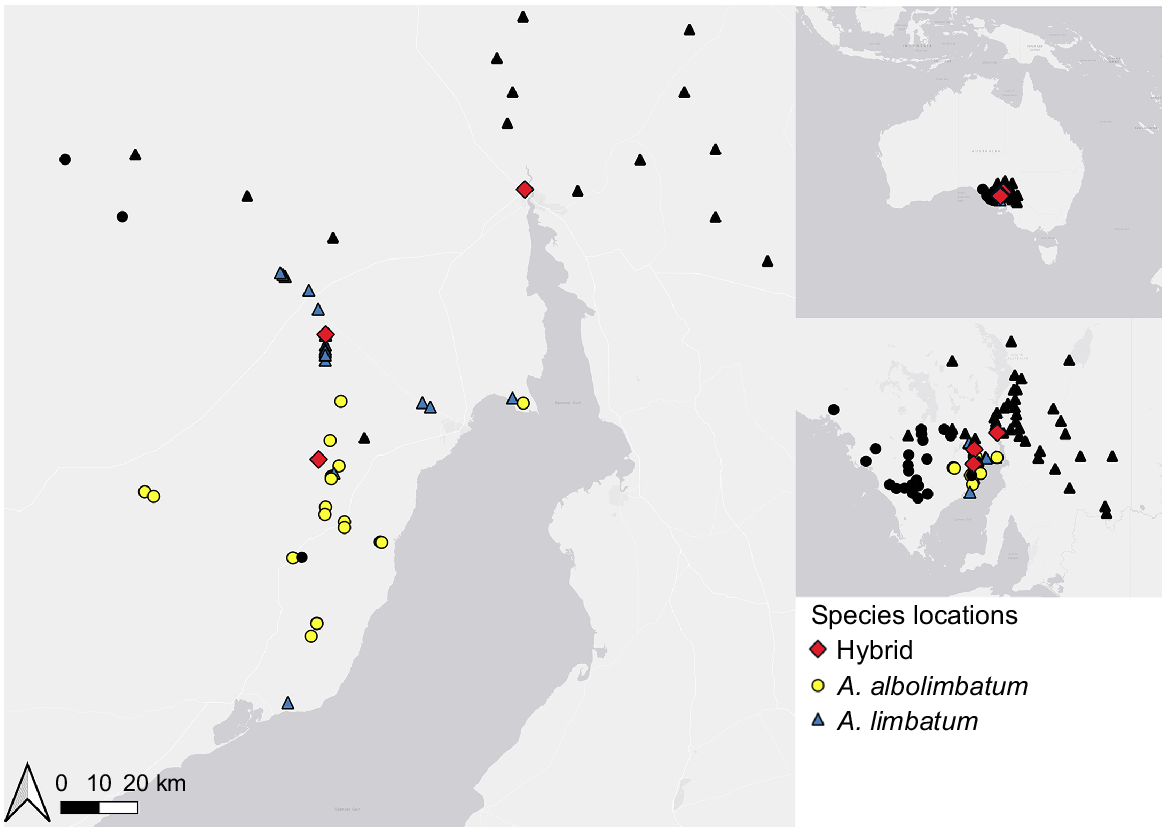
Morphological identification
Morphology was examined under a dissecting microscope to confirm species identification following Roberts (1970). Key characters used to distinguish adult A. albolimbatum and A. limbatum were: body shape; distance between porose areas on the base of the capitulum; and ornamentation and punctation marks on the scutum and the fourth coxa in males (Fig. 2). Larvae and nymphs do not display clear morphological differences, so were assigned to a species based on which adult tick species were present on the same lizard. No larvae or nymphs were observed on lizards without adult ticks or from those hosting both species of ticks. Individuals were identified as potential morphological hybrids when they had a combination of each species’ characters, and a clear species identification was not possible.
Key morphological characters used to differentiate A. limbatum from A. albolimbatum. Dorsal view of female A. limbatum (left, 65× magnification) – A: greater distance between the porose areas at the base of capitulum which are also smaller in size; B: the bilateral presence of two or more larger and deeper punctations forming a curved groove in the ocular region; C: ornamentation extending beyond the ocular regions towards the posterior part of the scutum; and D: anterolateral punctations are less frequent and shallower in depth. Dorsal view of male A. limbatum (middle, 65× magnification) – A: ovular body shape with round posterior and ornamentation extending along the lateral margins of the scutum; B: deep and well-defined lateral grooves; and C: punctation on posterior half of scutum. Ventral view of male A. albolimbatum (right, 125× magnification) – A: elongated fourth coxa.
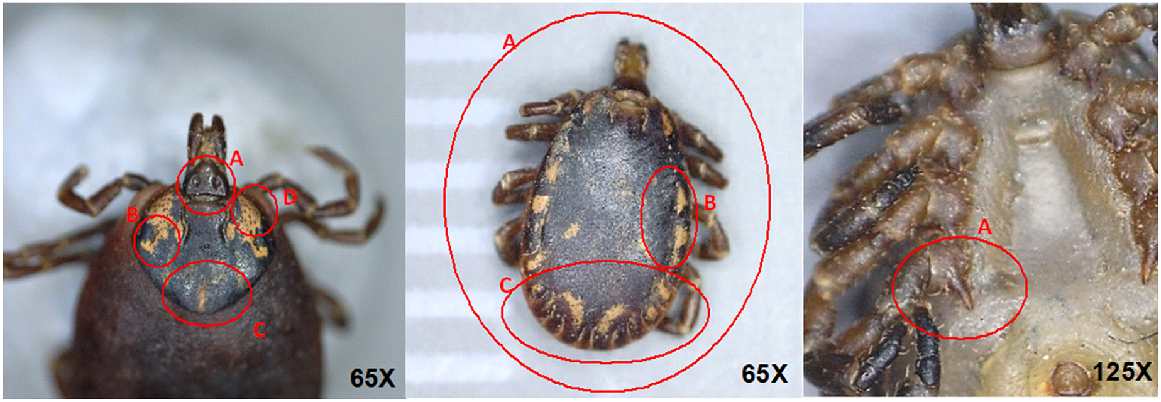
SNP genotyping and filtering
Tissue samples from 86 ticks were sent to Diversity Array Technology (DArT) in Canberra, Australia, for DNA extraction and a DArTseq™ assay using a low-density option (300 000 reads). DArT uses next-generation sequencing technologies and complexity reduction methods to assay single nucleotide polymorphisms (SNPs) (Sansaloni et al. 2011). Complexity reduction was achieved following Kilian et al. (2012), modified to use a combination of the restriction enzymes Pstl and Sphl with proprietary processing associated with SNP calling (Diversity Arrays Technology, Canberra). DNA from both legs and body of eight adult individuals (individual replicates) were sequenced to assess sequencing reproducibility between these two tissue types. Other adult ticks were sequenced from DNA extracted from legs only. DNA was extracted from whole bodies of ticks collected as nymphs or larvae as their legs were too small to obtain enough DNA (Table 1). We sequenced a maximum of three ticks from the same tick species per lizard per capture – to reduce the possibility of sampling siblings. In one case, five ticks from the same lizard and capture were sequenced because they included morphological hybrids and we suspected any of those ticks could have been a genomic hybrid. A range of life stages was chosen for sequencing when multiple ticks occurred on the same lizard in case hybrids had reduced fitness and did not survive till adulthood. DArTsoft14 DArT PL’s proprietary software filtered out potential viral and bacterial sequences during SNP calling. The blast alignment parameters used to determine the presence of viral and bacterial sequences were set to a minimum sequence percentage identity of 80% and a maximum E-value of 5e-7. The E-value represents the similarity between sequences of similar quality expected by chance where comparisons of sequences with smaller E-values have better homology.
Morphologically identified species and life stage | Genomically identified Amblyomma limbatum (n) | Genomically identified Amblyomma albolimbatum (n) | Genomically identified hybrid (n) | Total sequenced (n) | Total not sequenced (n) | Total morphologically identified (n) |
Amblyomma limbatum | ||||||
Adult female | 9 | 0 | 0 | 9 | 5 | 14 |
Adult male | 17 | 0 | 1 | 18 | 15 | 33 |
Nymph | 8 | 0 | 1 | 9 | 10 | 19 |
Larva | 0 | 0 | 0 | 0 | 0 | 0 |
Amblyomma albolimbatum | ||||||
Adult female | 0 | 10 | 1 | 11 | 15 | 26 |
Adult male | 3 | 20 | 1 | 24 | 52 | 76 |
Nymph | 0 | 3 | 0 | 3 | 6 | 9 |
Larva | 0 | 2 | 0 | 2 | 0 | 2 |
Suspected hybrids | ||||||
Adult female | 3 | 5 | 0 | 8 | 0 | 8 |
Adult male | 0 | 1 | 0 | 1 | 0 | 1 |
Nymph | 0 | 0 | 0 | 0 | 0 | 0 |
Larva | 0 | 0 | 0 | 0 | 0 | 0 |
Total ticks | 40 | 41 | 4 | 85 | 103 | 188 |
The number of ticks that were morphologically identified by life stage, species and sex for adults and genomically identified by sequencing of SNPs are shown.
We used the statistical environment R ver. 3.5.2 with the packages dartR (Gruber et al. 2018) and Adegenet (Jombart 2008; Jombart and Ahmed 2011) to assess the quality of the SNP dataset. Genotypes generated from adult tick bodies were removed, along with associated monomorphic loci, as these were duplicate samples. We performed a sensitivity analysis of the data with a Principal Coordinates Analysis (PCoA) to see whether any sources of potential bias may have influenced population structure. We did this by producing a PCoA after removing SNPs in the following categories: (1) SNPs with a reproducibility average (the proportion of genotypes that were the same across multiple sequencing runs), over all individuals (Gruber et al. 2018) less than one, (2) SNPs that contained alleles that were only found in whole body samples and not a leg sample (i.e. monomorphic in leg samples), (3) SNPs with any missing data, and (4) SNPs that appeared on the same sequence tag (keeping the SNP with the highest average Polymorphism Information Content). We also assessed whether the removal of individuals with high relatedness (r > 0.4) altered the PCoA results (supplementary material).
Genomic analysis and identifying hybrids
Genomic similarities of individuals were visualised by a PCoA and the overall level of differentiation between populations was ascertained with an FST value generated with Adegenet (Jombart 2008; Jombart and Ahmed 2011). We used the Bayesian clustering based software STRUCTURE ver. 2.3.4 (Pritchard et al. 2000) to assign individuals into populations of each species (A. albolimbatum or A. limbatum) and identify hybrids. STRUCTURE categorises populations by determining the number of groupings that minimise deviation from Hardy–Weinberg equilibrium. The software tested the likelihood of models from K = 1–4 with a burn-in of 10 000 Markov chain Monte Carlo (MCMC) iterations followed by a further 100 000 data-producing MCMC iterations. Each measure of K was replicated five times and was run using the admixture model. No other priors were used. We used the web-based program STRUCTURE HARVESTER (Earl and vonHoldt 2012) to identify the best fit for K. We determined K, where K ≠ 1 for L(K) (mean ± s.e.), using the Evanno method (Earl and vonHoldt 2012). Replicate models were used to determine the variance of L(K). Then, the software CLUMPP ver. 1.1.2 (Jakobsson and Rosenberg 2007) was used to merge the replicates from the output of the most likely model. Q values of the most likely K were visualised using the software DISTRUCT ver. 1.1 (Rosenberg 2004).
Individuals were allocated to specific hybrid classes (e.g. F1, F2, backcrosses, etc.) using the program NEWHYBRIDS ver. 1.1 (Anderson and Thompson 2002). NEWHYBRIDS uses patterns of gene inheritance within each locus as opposed to the proportions of gene inheritance within each individual to categorise individuals into hybrid classes (Wringe et al. 2017). Due to memory constraints, NEWHYBRIDS could only use ~200 loci per run. The dartr function gl.nhybrids – used in the program R STUDIO ver. 3.5.2 – was used to randomly select 200 loci from the filtered dataset. This dartr function was then executed 10 times for each dataset, meaning 20 datasets were created for the NEWHYBRIDS analysis. The NEWHYBRIDS analysis was performed with a burn-in of 10 000 iterations followed by 100 000 data-producing MCMC iterations. The output containing posterior probabilities was averaged across runs to determine which hybrid category each tick belonged to. The results from STRUCTURE and NEWHYBRIDS were combined to map the current distribution of the two tick species and their hybrids using QGIS (QGIS.org 2022).
Results
Sample collection and morphological identification
A total of 47 sleepy lizards (one was recaptured on three separate occasions) resulted in the collection of 188 ticks (Table 1). Of the 188 ticks collected, 66 were morphologically identified as A. limbatum (37 were sent for sequencing) and 113 were morphologically identified as A. albolimbatum (40 were sent for sequencing). There were nine individuals that displayed intermediate morphological characters between A. albolimbatum and A. limbatum; these were designated suspected hybrids and all were sent for sequencing (Fig. 3).
Suspected hybrid female tick (from morphology) displaying ocular region punctations as seen in A. limbatum (left, 125× magnification) as well as a smaller distance between the porose areas at the base of capitulum, which are also larger in size as seen in A. albolimbatum (right, 125× magnification).
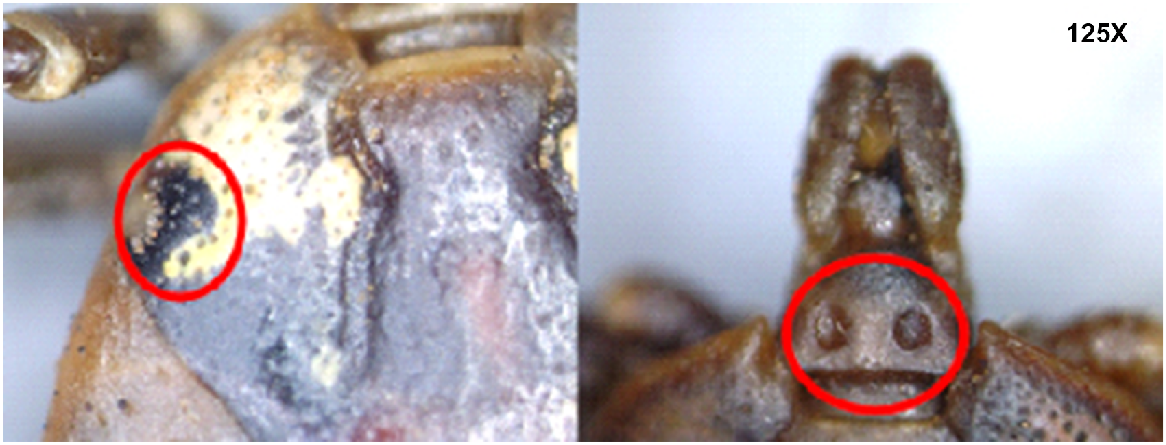
SNP genotyping
The NCBI blast conducted by DArT found a high level of bacterial contamination across the dataset. The blast aligned the contaminated sequences with two sequences from their databases, Bacterias_NCBI and Fungi_NCBI20170606. The removal of all bacterial and fungal sequences prior to SNP calling resulted in one individual being excluded from the data as it contained only bacterial sequences.
Following SNP calling, DArT produced an initial dataset containing 77 904 SNPs (33.45% missing data). The working dataset contained 6361 SNPs (with 0% missing data). A PCoA generated with the working dataset separated A. albolimbatum from A. limbatum with component 1, which contained 93.7% of the variation (Fig. 4). Four intermediate individuals (hybrids) were present in the PCoA plots based on component 1. We compared PCoA plots generated with the full filtering method (Fig. S1), and the reduced filtering steps (working dataset), as well as the working dataset with no relatedness >0.4 (Fig. S2) and found there to be no differences in patterns of population structure. A large proportion of variation (0.937, component 1) separated the two genomic clusters that represent A. limbatum and A. albolimbatum. This variation can be explained by the distribution of distinct alleles between the species; 46% of alleles were fixed between genomic A. limbatum and A. albolimbatum, 62.6% of alleles were private to genomic A. albolimbatum and 76% of alleles were private to genomic A. limbatum.
Plot of principal coordinate analysis. The group symbol specifies the tick species determined by morphological identification (adults) and the species of other adults present on the same lizard (nymphs and larvae).
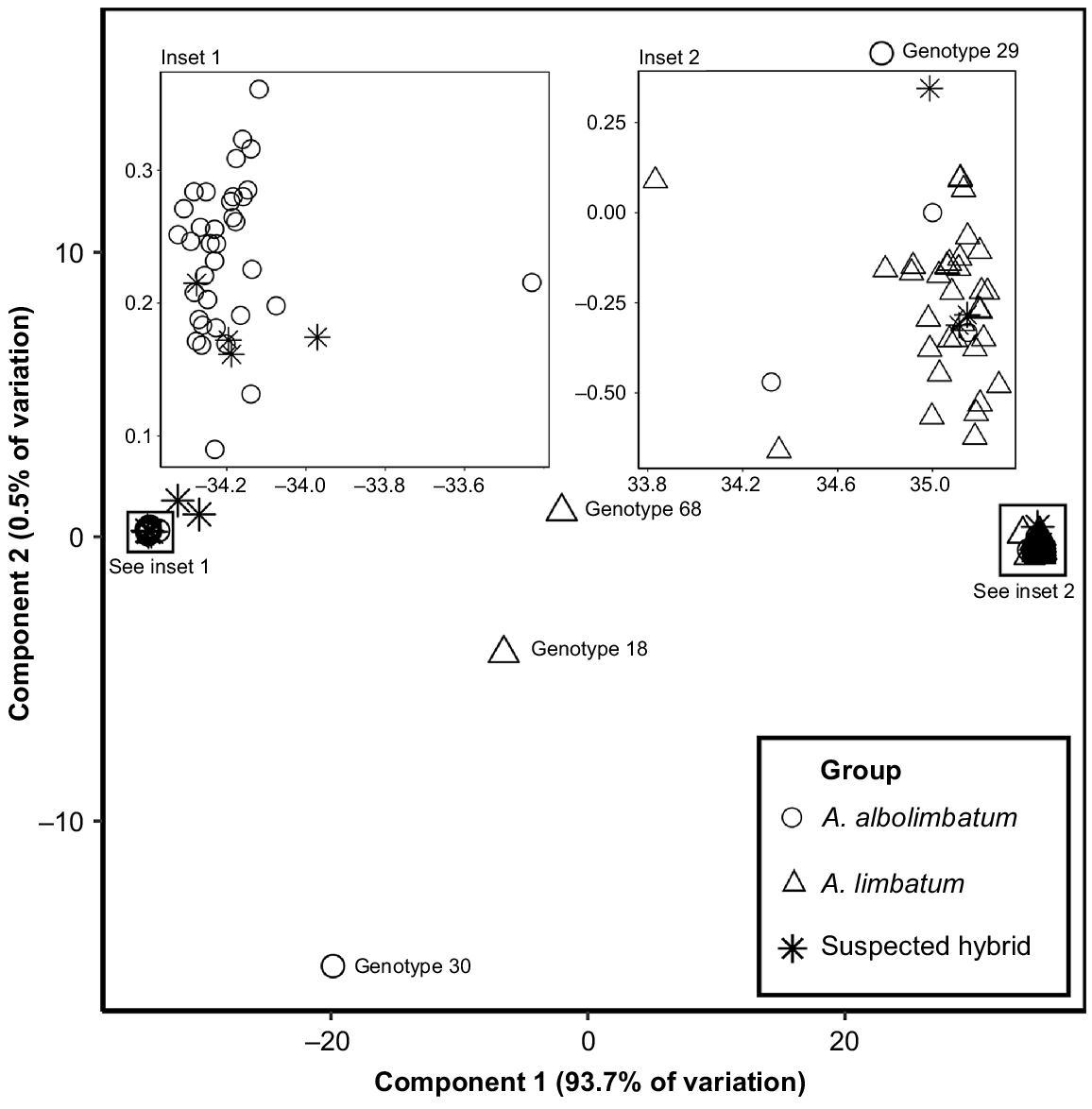
We investigated other potential sources of sequencing error and found that only 3% of loci within the initial unfiltered dataset contained alleles that only appeared in one or more of the 13 body samples sequenced. The average error rate between duplicate samples (leg and body) was 2.2% total error. Most of this error occurred between calls that were homozygous in one sample and heterozygous in the other (on average 97.4% of the total error). Possible sources of this error may be low read number for some alleles or tick body specific sequences that may originate from non-tick DNA, e.g. from a tick’s gut content. We checked whether low locus reproducibility or unique alleles within the hybrid individuals contributed to the 100 loci with the greatest loading on PC2 (generated with the working dataset). We found that these characteristics did not explain the low proportion of variation for PC2.
Genomic and hybrid analysis
STRUCTURE analysis indicated that the most likely model for the number of populations was K = 2. This model showed that most individuals were full members of only one particular species (A. albolimbatum or A. limbatum) and only a few individuals had very small amounts of mixed ancestry (Fig. 5, Table S1). The genomic differences between A. albolimbatum and A. limbatum produced an FST of 0.445. The STRUCTURE results resembled the PCoA plots as four intermediate individuals were identified as hybrids. The four hybrid individuals in the STRUCTURE results were genotype 29, genotype 68, genotype 18 and genotype 30. These were the same hybrid individuals shown in the PCoA and the NEWHYBRIDS result. Three of these hybrids were reported in a location where both parental species occurred; however, one hybrid was located ~80 km north of the suspected species boundary (genotype 18). These hybrid ticks included one fully engorged adult female, one nymph, and two adult males (Table S1). Two of these ticks (the engorged female and one of the adult males) were found on the same lizard. Genomically, none of the nine morphologically intermediate ticks (suspected hybrids) were found to be hybrids; three were classified as A. albolimbatum and six were classified as A. limbatum. There were three morphological A. albolimbatum individuals within the genomic A. limbatum cluster, (genotype 19, genotype 88 and genotype 92). There were no morphological A. limbatum individuals within the genomic A. albolimbatum cluster.
STRUCTURE results showing Bayesian assignment of individuals for K = 2. Each column shows an individual’s posterior probability of membership to each cluster with blue representing A. albolimbatum and orange representing A. limbatum. The hybrids are in the following order: genotype 18, genotype 29, genotype 30 and genotype 68.
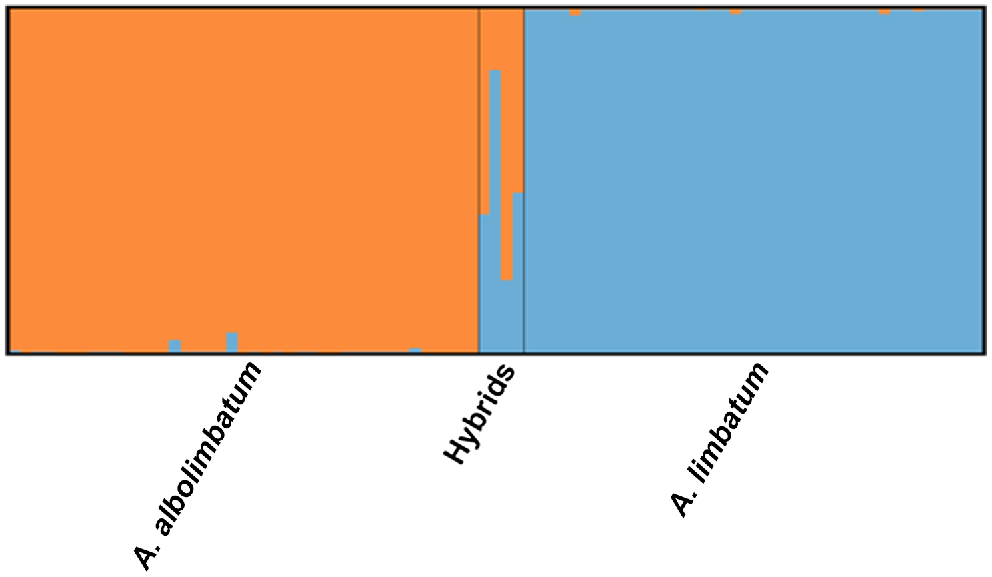
Following analysis using the NEWHYBRIDS software, one genomically identified hybrid (nymph) was found to be from an F1 generation and the other three were backcrosses (Table 2). Two of the backcrosses (two males) had a closer genomic makeup to A. albolimbatum while the other backcross (female) was more closely related to A. limbatum. All runs of NEWHYBRIDS were in agreeance with each other regarding the species or hybrid category in which each individual was found.
Hybrid category | F1 | F2 | A. albolimbatum backcross | A. limbatum backcross |
Genotype 18 | 0 | 0.000903 | 0.583015 | 0.416082 |
Genotype 29 | 0 | 0 | 0.416667 | 0.583333 |
Genotype 30 | 0 | 0 | 0.583333 | 0.416667 |
Genotype 68 | 0.999984 | 0 | 0.000015 | 0 |
Posterior probabilities of each hybrid individual belonging to each hybrid class (F1, F2, A. albolimbatum backcross and A. limbatum backcross).
Distributions
The genomic data confirmed that A. albolimbatum and A. limbatum have a parapatric boundary where admixture is occurring (Fig. 1). The parapatric boundary is located on the northern part of the Eyre Peninsula and proceeds from the coast of the Spencer Gulf to the west for roughly 80 km. Four individuals, three A. limbatum and one hybrid, were found outside of their previously known range. The three A. limbatum individuals (genotype 20, genotype 21 and genotype 25) occurred south of the parapatric boundary. The hybrid (genotype 18) was found north of the known boundary within what was previously thought to be only A. limbatum territory but was from an A. albolimbatum backcross. The three remaining hybrids were found within the previously known parapatric region.
Discussion
Studying natural overlap zones between closely related species can provide insights into what may occur as species ranges come into contact under climate change – this is particularly relevant for pathogen transmitting parasites. This study examined the parapatric boundary of A. albolimbatum and A. limbatum resulting in three critical findings. First, A. albolimbatum and A. limbatum are confirmed as distinct species. Second, that the two species can hybridise. Third, that individuals displaying intermediate morphological traits were not identified as genomic hybrids.
This is the first report of naturally occurring hybridisation between the two tick species A. albolimbatum and A. limbatum. The identification, frequency and location of hybrid ticks helps our understanding of the impact of climate change on tick distributions and mechanisms behind the maintenance of parapatric regions. The discovery of hybrid ticks suggests there may be a risk that novel genomic combinations could lead to transmission of locally novel pathogens. We found that the geographic location of the parapatric boundary between A. albolimbatum and A. limbatum was similar to what was previously described (Smyth 1973); however, the extent of the parapatric region was much larger than expected. One A. albolimbatum backcross hybrid was found ~80 km north of the previously described parapatric boundary (within expected A. limbatum territory), suggesting that the two parental lineages may be co-located in this area. Both tick and sleepy lizard dispersal is known to be slow (Bull 1991) as ticks heavily rely on host species to disperse, and sleepy lizards do not have large home ranges (Bull and Freake 1999). Additionally, three A. limbatum individuals were found ~100 km south of the parapatric boundary within expected A. albolimbatum territory. We sequenced less than half of the number of ticks that were collected, and we were unable to identify hybrids morphologically using the typical morphological traits that distinguish these tick species so it is highly likely that hybrids may be more frequent than what this study shows. Our findings indicate that A. albolimbatum and A. limbatum are sexually compatible and that hybrids between the two species are reproductively viable. Future research should focus on mapping the extent of the parapatric boundary, identifying morphological traits that could be used to identify hybrids as well as assessing the pathogenic composition of hybrid ticks and their impact on host health.
Changes in climate can affect species distributions so that expanding ranges could increase the occurrence of hybridisation. Finding A. limbatum so far south could be a consequential factor of the drought that occurred during the survey period; however, southern Australia is experiencing increasing heat and dryness in response to climate change (Siebentritt et al. 2014). A. limbatum is a more arid adapted species than A. albolimbatum, which may allow A. limbatum to migrate south into areas that usually have a cooler climate. Species introgression is also known to impact hybrid zone movement particularly when gene flow is unidirectional. The occurrence of a hybrid so far north of the parapatric zone could indicate unidirectional geneflow where A. albolimbatum is the advancing species. An example where unidirectional geneflow has caused hybrid zone movement has previously been described in crested newt species (genus Triturus) by Wielstra et al. (2017). Further research is required to consolidate the parapatric boundary location and whether changes in temperature, rainfall and other climatic factors are instigating species boundary shifts or whether patterns of introgression across the hybrid zone are causing movements of the hybrids or the hybrid zone (Barton and Hewitt 1985; Currat et al. 2008; Wielstra et al. 2017).
The discovery of intermediate morphotypes is often the first indicator of species hybridisation which could impact how we manage and classify biodiversity. Detecting hybrids morphologically can be problematic as morphological variations are often attributed to environmental factors such as food availability and temperature (Lopes et al. 2018). Ticks especially have cryptic morphology and are difficult to describe taxonomically (Dantas-Torres 2018). In this study, we found morphologically intermediate ticks that were either A. limbatum or A. albolimbatum (not hybrids), and morphologically cryptic A. limbatum individuals which were misidentified as A. albolimatum. This suggests there is morphological variability within these species that has previously gone undetected. It is not unusual for nymphs or engorged females to be misidentified as their form may be highly variable. In this study, the morphologically intermediate ticks were all adult females but the three misidentified A. limbatum individuals were all male. Therefore, even male A. limbatum are problematic to identify morphologically. Surprisingly, the genomically identified hybrid ticks in this study were not described as hybrids following the usual morphological attributes. This finding can be explained for one of the backcrossed hybrid ticks (female) as their morphotype corresponded to the species that represented a greater proportion of their genomic background. The other two backcrossed hybrids (both males), however, were morphologically more like the alternate species and not the species represented by their genomic background. For ticks, the sex and species of the hybrid parents can play a role in which morphotype is displayed by the hybrid offspring, as is exemplified by two species of Rhipicephalus ticks, R. appendiculatus and R. zambeziensis (Zivkovic et al. 1986). Cryptic species and morphological variation associated with various levels of hybridisation are also commonly reported among a wide range of tick families (Kovalev et al. 2016; Dantas-Torres 2018). Detecting hybrid ticks in the future may depend on genomic interrogation of individual ticks.
The presence of morphologically undetectable hybrids and the variability of tick morphotypes impacts past, current, and future work. In this system, no other study has used genomic data to identify species, which questions the reliability of morphological identifications from the past. Our study has highlighted the need for a revision of our current morphological assessments which should be paired with genomic confirmation. We would also suggest that the keys and descriptions used to distinguish morphological differences between A. albolimbatum and A. limbatum (Roberts 1970) are not fully reliable. To ensure higher accuracy in species identifications, the use of genomic approaches is recommended to identify phenotypic traits that more accurately distinguish among species and hybrids.
Recent anthropogenically caused environmental degradation and climatic change are bringing species together that may not have ordinarily come into contact. Over the globe we are seeing both expanding and contracting ranges of different species as they try to adjust to the growing anthropogenic pressures and diminishing habitat availability (Taylor et al. 2015). As a result, species that may not have ordinarily encountered one another are now being pushed into abutting ranges, or sometimes even sympatry (Pfäffle et al. 2014). Parasites like ticks have greatly impacted both human and animal health. Hybridisation between two closely related species can further diversify a parasite’s home range, host species and pathogens, leaving new host species susceptible to higher parasitic infection and risk of disease than the hosts that coevolved alongside the parasite (Jones et al. 2018). Increased temperatures can also increase pathogen development, survival rates, disease transmission and host susceptibility (Harvell et al. 2002). The hybridisation of A. albolimbatum and A. limbatum provides an opportunity to examine what may happen when two closely related species’ distributional boundaries abut under a changing climate.
Acknowledgements
All procedures from this study were approved by the Flinders University Animal Welfare Committee (permit no. E45417). The research was conducted under permits from the South Australian Department for Environment and Water (permit no. A23436-26). Lastly, we thank Dr Gerrut Norval for help with manuscript corrections, and all the volunteers who assisted with the data collection.
References
Abbott, RJ, Barton, NH, and Good, JM (2016). Genomics of hybridization and its evolutionary consequences. Molecular Ecology 25, 2325–2332.| Genomics of hybridization and its evolutionary consequences.Crossref | GoogleScholarGoogle Scholar |
Alkishe, A, Raghavan, RK, and Peterson, AT (2021). Likely geographic distributional shifts among medically important tick species and tick-associated diseases under climate change in North America: a review. Insects 12, 225.
| Likely geographic distributional shifts among medically important tick species and tick-associated diseases under climate change in North America: a review.Crossref | GoogleScholarGoogle Scholar |
Anderson, EC, and Thompson, EA (2002). A model-based method for identifying species hybrids using multilocus genetic data. Genetics 160, 1217–1229.
| A model-based method for identifying species hybrids using multilocus genetic data.Crossref | GoogleScholarGoogle Scholar |
Andrews, RH, Petney, TN, and Bull, CM (1982). Reproductive interference between three parapatric species of reptile tick. Oecologia 52, 281–286.
| Reproductive interference between three parapatric species of reptile tick.Crossref | GoogleScholarGoogle Scholar |
Barton, NH, and Hewitt, GM (1985). Analysis of hybrid zones. Annual Review of Ecology and Systematics 16, 113–148.
| Analysis of hybrid zones.Crossref | GoogleScholarGoogle Scholar |
Beever, EA, O’Leary, J, Mengelt, C, West, JM, Julius, S, Green, N, Magness, D, Petes, L, Stein, B, Nicotra, AB, Hellmann, JJ, Robertson, AL, Staudinger, MD, Rosenberg, AA, Babij, E, Brennan, J, Schuurman, GW, and Hofmann, GE (2016). Improving conservation outcomes with a new paradigm for understanding species’ fundamental and realized adaptive capacity. Conservation Letters 9, 131–137.
| Improving conservation outcomes with a new paradigm for understanding species’ fundamental and realized adaptive capacity.Crossref | GoogleScholarGoogle Scholar |
Bournez, L, Cangi, N, Lancelot, R, Pleydell, DRJ, Stachurski, F, Bouyer, J, Martinez, D, Lefrancois, T, Neves, L, and Pradel, J (2015). Parapatric distribution and sexual competition between two tick species, Amblyomma variegatum and A. hebraeum (Acari, Ixodidae), in Mozambique. Parasites & Vectors 8, 504.
| Parapatric distribution and sexual competition between two tick species, Amblyomma variegatum and A. hebraeum (Acari, Ixodidae), in Mozambique.Crossref | GoogleScholarGoogle Scholar |
Brauer, CJ, Sandoval-Castillo, J, Gates, K, Hammer, MP, Unmack, PJ, Bernatchez, L, and Beheregaray, LB (2023). Natural hybridization reduces vulnerability to climate change. Nature Climate Change 13, 282–289.
| Natural hybridization reduces vulnerability to climate change.Crossref | GoogleScholarGoogle Scholar |
Bull, CM (1991). Ecology of parapatric distributions. Annual Review of Ecology and Systematics 22, 19–36.
| Ecology of parapatric distributions.Crossref | GoogleScholarGoogle Scholar |
Bull, CM, and Burzacott, D (2001). Temporal and spatial dynamics of a parapatric boundary between two Australian reptile ticks. Molecular Ecology 10, 639–648.
| Temporal and spatial dynamics of a parapatric boundary between two Australian reptile ticks.Crossref | GoogleScholarGoogle Scholar |
Bull, CM, and Freake, MJ (1999). Home-range fidelity in the Australian sleepy lizard, Tiliqua rugosa. Australian Journal of Zoology 47, 125–132.
| Home-range fidelity in the Australian sleepy lizard, Tiliqua rugosa.Crossref | GoogleScholarGoogle Scholar |
Carlson, CJ, Burgio, KR, Dougherty, ER, Phillips, AJ, Bueno, VM, Clements, CF, Castaldo, G, Dallas, TA, Cizauskas, CA, Cumming, GS, Doña, J, Harris, NC, Jovani, R, Mironov, S, Muellerklein, OC, Proctor, HC, and Getz, WM (2017). Parasite biodiversity faces extinction and redistribution in a changing climate. Science Advances 3, e1602422.
| Parasite biodiversity faces extinction and redistribution in a changing climate.Crossref | GoogleScholarGoogle Scholar |
Currat, M, Ruedi, M, Petit, RJ, and Excoffier, L (2008). The hidden side of invasions: massive introgression by local genes. Evolution 62, 1908–1920.
| The hidden side of invasions: massive introgression by local genes.Crossref | GoogleScholarGoogle Scholar |
Dantas-Torres, F (2018). Species concepts: what about ticks? Trends in Parasitology 34, 1017–1026.
| Species concepts: what about ticks?Crossref | GoogleScholarGoogle Scholar |
Dehhaghi, M, Kazemi Shariat Panahi, H, Holmes, EC, Hudson, BJ, Schloeffel, R, and Guillemin, GJ (2019). Human tick-borne diseases in Australia. Frontiers in Cellular and Infection Microbiology 9, 3.
| Human tick-borne diseases in Australia.Crossref | GoogleScholarGoogle Scholar |
Earl, DA, and vonHoldt, BM (2012). STRUCTURE HARVESTER: a website and program for visualizing STRUCTURE output and implementing the Evanno method. Conservation Genetics Resources 4, 359–361.
| STRUCTURE HARVESTER: a website and program for visualizing STRUCTURE output and implementing the Evanno method.Crossref | GoogleScholarGoogle Scholar |
Engler, JO, Rödder, D, Elle, O, Hochkirch, A, and Secondi, J (2013). Species distribution models contribute to determine the effect of climate and interspecific interactions in moving hybrid zones. Journal of Evolutionary Biology 26, 2487–2496.
| Species distribution models contribute to determine the effect of climate and interspecific interactions in moving hybrid zones.Crossref | GoogleScholarGoogle Scholar |
Estrada-Peña, A, Ayllón, N, and De La Fuente, J (2012). Impact of climate trends on tick-borne pathogen transmission. Frontiers in Physiology 3, 64.
Godfrey, SS, and Gardner, MG (2017). Lizards, ticks and contributions to Australian parasitology: C. Michael Bull (1947–2016). International Journal for Parasitology: Parasites and Wildlife 6, 295–298.
Gruber, B, Unmack, PJ, Berry, OF, and Georges, A (2018). dartr: an r package to facilitate analysis of SNP data generated from reduced representation genome sequencing. Molecular Ecology Resources 18, 691–699.
| dartr: an r package to facilitate analysis of SNP data generated from reduced representation genome sequencing.Crossref | GoogleScholarGoogle Scholar |
Harper, FM, and Hart, MW (2007). Morphological and phylogenetic evidence for hybridization and introgression in a sea star secondary contact zone. Invertebrate Biology 126, 373–384.
| Morphological and phylogenetic evidence for hybridization and introgression in a sea star secondary contact zone.Crossref | GoogleScholarGoogle Scholar |
Harr, B, and Price, T (2014). Climate change: a hybrid zone moves north. Current Biology 24, R230–R232.
| Climate change: a hybrid zone moves north.Crossref | GoogleScholarGoogle Scholar |
Harvell, CD, Mitchell, CE, Ward, JR, Altizer, S, Dobson, AP, Ostfeld, RS, and Samuel, MD (2002). Climate warming and disease risks for terrestrial and marine biota. Science 296, 2158–2162.
| Climate warming and disease risks for terrestrial and marine biota.Crossref | GoogleScholarGoogle Scholar |
Hasselman, DJ, Argo, EE, McBride, MC, Bentzen, P, Schultz, TF, Perez-Umphrey, AA, and Palkovacs, EP (2014). Human disturbance causes the formation of a hybrid swarm between two naturally sympatric fish species. Molecular Ecology 23, 1137–1152.
| Human disturbance causes the formation of a hybrid swarm between two naturally sympatric fish species.Crossref | GoogleScholarGoogle Scholar |
Hermes, C, Keller, K, Nicholas, RE, Segelbacher, G, and Schaefer, HM (2018). Projected impacts of climate change on habitat availability for an endangered parakeet. PLoS ONE 13, e0191773.
| Projected impacts of climate change on habitat availability for an endangered parakeet.Crossref | GoogleScholarGoogle Scholar |
Hunter, EA, Matocq, MD, Murphy, PJ, and Shoemaker, KT (2017). Differential effects of climate on survival rates drive hybrid zone movement. Current Biology 27, 3898–3903.e4.
| Differential effects of climate on survival rates drive hybrid zone movement.Crossref | GoogleScholarGoogle Scholar |
Jakobsson, M, and Rosenberg, NA (2007). CLUMPP: a cluster matching and permutation program for dealing with label switching and multimodality in analysis of population structure. Bioinformatics 23, 1801–1806.
| CLUMPP: a cluster matching and permutation program for dealing with label switching and multimodality in analysis of population structure.Crossref | GoogleScholarGoogle Scholar |
Jombart, T (2008). adegenet: a R package for the multivariate analysis of genetic markers. Bioinformatics 24, 1403–1405.
| adegenet: a R package for the multivariate analysis of genetic markers.Crossref | GoogleScholarGoogle Scholar |
Jombart, T, and Ahmed, I (2011). adegenet 1.3-1: new tools for the analysis of genome-wide SNP data. Bioinformatics 27, 3070–3071.
| adegenet 1.3-1: new tools for the analysis of genome-wide SNP data.Crossref | GoogleScholarGoogle Scholar |
Jones, W, Kulma, K, Bensch, S, Cichoń, M, Kerimov, A, Krist, M, Laaksonen, T, Moreno, J, Munclinger, P, Slater, FM, Szöllősi, E, Visser, ME, and Qvarnström, A (2018). Interspecific transfer of parasites following a range-shift in Ficedula flycatchers. Ecology and Evolution 8, 12183–12192.
| Interspecific transfer of parasites following a range-shift in Ficedula flycatchers.Crossref | GoogleScholarGoogle Scholar |
Kilian A, Wenzl P, Huttner E, Carling J, Xia L, Blois H, Caig V, Heller-Uszynska K, Jaccoud D, Hopper C, Aschenbrenner-Kilian M, Evers M, Peng K, Cayla C, Hok P, Uszynski G (2012) Diversity arrays technology: a generic genome profiling technology on open platforms. In ‘Data production and analysis in population genomics’. (Eds F Pompanon, A Bonin) pp. 67–89. (Humana Press)
King, KC, Stelkens, RB, Webster, JP, Smith, DF, and Brockhurst, MA (2015). Hybridization in parasites: consequences for adaptive evolution, pathogenesis, and public health in a changing world. PLoS Pathogens 11, e1005098.
| Hybridization in parasites: consequences for adaptive evolution, pathogenesis, and public health in a changing world.Crossref | GoogleScholarGoogle Scholar |
Kovalev, SY, Golovljova, IV, and Mukhacheva, TA (2016). Natural hybridization between Ixodes ricinus and Ixodes persulcatus ticks evidenced by molecular genetics methods. Ticks and Tick-borne Diseases 7, 113–118.
| Natural hybridization between Ixodes ricinus and Ixodes persulcatus ticks evidenced by molecular genetics methods.Crossref | GoogleScholarGoogle Scholar |
Léger, E, Vourc’h, G, Vial, L, Chevillon, C, and McCoy, KD (2013). Changing distributions of ticks: causes and consequences. Experimental and Applied Acarology 59, 219–244.
| Changing distributions of ticks: causes and consequences.Crossref | GoogleScholarGoogle Scholar |
Lopes, PC, Kanno, RH, Sourassou, NF, and Moraes, GJ (2018). Effect of temperature and diet on the morphology of Euseius concordis (Acari: Phytoseiidae). Systematic and Applied Acarology 23, 1322–1332.
| Effect of temperature and diet on the morphology of Euseius concordis (Acari: Phytoseiidae).Crossref | GoogleScholarGoogle Scholar |
Lynen, G, Zeman, P, Bakuname, C, Di Giulio, G, Mtui, P, Sanka, P, and Jongejan, F (2008). Shifts in the distributional ranges of Boophilus ticks in Tanzania: evidence that a parapatric boundary between Boophilus microplus and B. decoloratus follows climate gradients. Experimental and Applied Acarology 44, 147–164.
| Shifts in the distributional ranges of Boophilus ticks in Tanzania: evidence that a parapatric boundary between Boophilus microplus and B. decoloratus follows climate gradients.Crossref | GoogleScholarGoogle Scholar |
Mayr E (1963) ‘Animals, species and evolution.’ (Harvard University Press: Cambridge, Massachusetts)
Mayr E (1970) ‘Populations, species, and evolution: an abridgment of animal species and evolution.’ (Belknap Press of Harvard University Press)
McQuillan, MA, and Rice, AM (2015). Differential effects of climate and species interactions on range limits at a hybrid zone: potential direct and indirect impacts of climate change. Ecology and Evolution 5, 5120–5137.
| Differential effects of climate and species interactions on range limits at a hybrid zone: potential direct and indirect impacts of climate change.Crossref | GoogleScholarGoogle Scholar |
Ogston, G, Beatty, SJ, Morgan, DL, Pusey, BJ, and Lymbery, AJ (2016). Living on burrowed time: aestivating fishes in south-western Australia face extinction due to climate change. Biological Conservation 195, 235–244.
| Living on burrowed time: aestivating fishes in south-western Australia face extinction due to climate change.Crossref | GoogleScholarGoogle Scholar |
Oliveira, SVd, Romero-Alvarez, D, Martins, TF, Santos, JPd, Labruna, MB, Gazeta, GS, Escobar, LE, and Gurgel-Gonçalves, R (2017). Amblyomma ticks and future climate: range contraction due to climate warming. Acta Tropica 176, 340–348.
| Amblyomma ticks and future climate: range contraction due to climate warming.Crossref | GoogleScholarGoogle Scholar |
Oliver, JH, Wilkinson, PR, and Kohls, GM (1972). Observations on hybridization of three species of North American dermacentor ticks. The Journal of Parasitology 58, 380–384.
| Observations on hybridization of three species of North American dermacentor ticks.Crossref | GoogleScholarGoogle Scholar |
Ostfeld, RS, and Brunner, JL (2015). Climate change and Ixodes tick-borne diseases of humans. Philosophical Transactions of the Royal Society B: Biological Sciences 370, 20140051.
| Climate change and Ixodes tick-borne diseases of humans.Crossref | GoogleScholarGoogle Scholar |
Peterson, BK, Weber, JN, Kay, EH, Fisher, HS, and Hoekstra, HE (2012). Double digest RADseq: an inexpensive method for de novo SNP discovery and genotyping in model and non-model species. PLoS ONE 7, e37135.
| Double digest RADseq: an inexpensive method for de novo SNP discovery and genotyping in model and non-model species.Crossref | GoogleScholarGoogle Scholar |
Pfäffle, M, Černá Bolfíková, B, Hulva, P, and Petney, T (2014). Different parasite faunas in sympatric populations of sister hedgehog species in a secondary contact zone. PLoS ONE 9, e114030.
| Different parasite faunas in sympatric populations of sister hedgehog species in a secondary contact zone.Crossref | GoogleScholarGoogle Scholar |
Pritchard, JK, Stephens, M, and Donnelly, P (2000). Inference of population structure using multilocus genotype data. Genetics 155, 945–959.
| Inference of population structure using multilocus genotype data.Crossref | GoogleScholarGoogle Scholar |
Roberts FHS (1970) Australian ticks. CSIRO Publishing, Melbourne.
Rosenberg, NA (2004). DISTRUCT: a program for the graphical display of population structure. Molecular Ecology Notes 4, 137–138.
| DISTRUCT: a program for the graphical display of population structure.Crossref | GoogleScholarGoogle Scholar |
Sansaloni, C, Petroli, C, Jaccoud, D, Carling, J, Detering, F, Grattapaglia, D, and Kilian, A (2011). Diversity Arrays Technology (DArT) and next-generation sequencing combined: genome-wide, high throughput, highly informative genotyping for molecular breeding of Eucalyptus. BMC Proceedings 5, P54.
| Diversity Arrays Technology (DArT) and next-generation sequencing combined: genome-wide, high throughput, highly informative genotyping for molecular breeding of Eucalyptus.Crossref | GoogleScholarGoogle Scholar |
Sharrad RD (1979) Studies of factors which determine the distributions of three species of South Australian reptile ticks. PhD thesis, University of Adelaide, Adelaide.
Siebentritt M, Halsey N, Stafford-Smith M (2014) Regional climate change adaptation plan for the Eyre Peninsula. Prepared for the Eyre Peninsula Integrated Climate Change Agreement Committee. Seed Consulting Services.
Smyth, M (1973). The distribution of three species of reptile ticks, Aponomma hydrosauri (Denny), Amblyomma albolimbatum Neumann, and Amb. limbatum Neumann I. Distribution and hosts. Australian Journal of Zoology 21, 91–101.
| The distribution of three species of reptile ticks, Aponomma hydrosauri (Denny), Amblyomma albolimbatum Neumann, and Amb. limbatum Neumann I. Distribution and hosts.Crossref | GoogleScholarGoogle Scholar |
Sonenshine, DE (2018). Range expansion of tick disease vectors in North America: implications for spread of tick-borne disease. International Journal of Environmental Research and Public Health 15, 478.
| Range expansion of tick disease vectors in North America: implications for spread of tick-borne disease.Crossref | GoogleScholarGoogle Scholar |
Sungirai, M, Madder, M, Moyo, DZ, De Clercq, P, and Abatih, EN (2015). An update on the ecological distribution of the Ixodidae ticks in Zimbabwe. Experimental and Applied Acarology 66, 269–280.
| An update on the ecological distribution of the Ixodidae ticks in Zimbabwe.Crossref | GoogleScholarGoogle Scholar |
Sungirai, M, Abatih, EN, Moyo, DZ, Clercq, PD, and Madder, M (2017). Shifts in the distribution of ixodid ticks parasitizing cattle in Zimbabwe. Medical and Veterinary Entomology 31, 78–87.
| Shifts in the distribution of ixodid ticks parasitizing cattle in Zimbabwe.Crossref | GoogleScholarGoogle Scholar |
Sutherst, RW (1987). The dynamics of hybrid zones between tick (Acari) species. International Journal for Parasitology 17, 921–926.
| The dynamics of hybrid zones between tick (Acari) species.Crossref | GoogleScholarGoogle Scholar |
Taylor, SA, Larson, EL, and Harrison, RG (2015). Hybrid zones: windows on climate change. Trends in Ecology & Evolution 30, 398–406.
| Hybrid zones: windows on climate change.Crossref | GoogleScholarGoogle Scholar |
Theodosopoulos, AN, Hund, AK, and Taylor, SA (2019). Parasites and host species barriers in animal hybrid zones. Trends in Ecology & Evolution 34, 19–30.
| Parasites and host species barriers in animal hybrid zones.Crossref | GoogleScholarGoogle Scholar |
Wielstra, B, Burke, T, Butlin, RK, Avcı, A, Üzüm, N, Bozkurt, E, Olgun, K, and Arntzen, JW (2017). A genomic footprint of hybrid zone movement in crested newts. Evolution Letters 1, 93–101.
| A genomic footprint of hybrid zone movement in crested newts.Crossref | GoogleScholarGoogle Scholar |
Wringe, BF, Stanley, RRE, Jeffery, NW, Anderson, EC, and Bradbury, IR (2017). parallelnewhybrid: an R package for the parallelization of hybrid detection using newhybrids. Molecular Ecology Resources 17, 91–95.
| parallelnewhybrid: an R package for the parallelization of hybrid detection using newhybrids.Crossref | GoogleScholarGoogle Scholar |
Zivkovic, D, Pegram, RG, Jongejan, F, and Mwase, ET (1986). Biology of Rhipicephalus appendiculatus and R. zambeziensis and production of a fertile hybrid under laboratory conditions. Experimental & Applied Acarology 2, 285–298.
| Biology of Rhipicephalus appendiculatus and R. zambeziensis and production of a fertile hybrid under laboratory conditions.Crossref | GoogleScholarGoogle Scholar |