Ethyl-Iophenoxic acid as a serum marker for oral baiting of Tasmanian devils
Ruth Pye




A
B
C
Abstract
Oral baits containing vaccines, medicants or toxicants have been delivered to a broad range of wildlife species to protect against or treat disease, or suppress populations. Ethyl-Iophenoxic acid (Et-IPA) is used as a biomarker to determine oral bait consumption by wildlife species to assess and refine baiting strategies. Et-IPA is a persistent biomarker in many eutherian mammal species but not in the two marsupial species, swamp wallaby (Wallabia bicolor) and brushtail possum (Trichosurus vulpecula), tested to date. Et-IPA has not previously been evaluated in carnivorous marsupials. The Tasmanian devil (Sarcophilus harrisii), the largest extant carnivorous marsupial, is threatened by devil facial tumour disease (DFTD). Development of an oral DFTD vaccine bait is underway. In this study, eight devils were fed between 1 mg and 50 mg Et-IPA via oral baits to assess the effectiveness of Et-IPA as a serum biomarker for this species. Using liquid chromatography with tandem mass spectrometry, serum Et-IPA was detected in the devils up to 206 days after ingestion. This study demonstrates the utility of Et-IPA for estimating oral bait consumption by devils, the first carnivorous marsupial species to which this applies.
Keywords: biomarker, devil facial tumour disease, iophenoxic acid, oral bait vaccine, Tasmanian devil, vaccination, wildlife baits, wildlife disease.
Introduction
Vaccines, medications and toxicants have been delivered to vertebrate wildlife in baits for at least 50 years to prevent or treat disease and parasitic infections (Baer 1976; Qureshi et al. 1994), or to suppress populations of invasive or nuisance species (reviewed by Philip 2021). Central to understanding the effectiveness of initial baiting strategies for wildlife disease mitigation or population control is the ability to monitor bait consumption by free-ranging animals. A variety of biomarkers, in particular tetracycline, rhodamine B, and derivatives of iophenoxic acid, have been included in baits to identify bait consumption by target and non-target species.
Biomarkers should be non-toxic, palatable, easily incorporated into a bait, and detectable by a minimally invasive method and for a useful length of time after ingestion (Jacoblinnert et al. 2021). Tetracycline and its derivatives are antibiotics that accumulate in the teeth and bones of animals when ingested and have been identified for up to 150 days after ingestion (Van Brackle et al. 1994). Their use as biomarkers relies on invasive methods, i.e. tooth extraction or culling, for detection. Rhodamine B has been widely used as a bait biomarker in mammals and can be detected in whiskers and hair for between 28 and 196 days in different species (Fisher 1999). Limitations associated with rhodamine B include palatability and difficulty of use in large bait production facilities.
Ethyl-Iophenoxic acid (Et-IPA), a widely available derivative of IPA, has a long history as a useful bait biomarker in a wide variety of mammalian species. Serum Et-IPA can be detected indirectly by measuring levels of protein bound iodine, or directly using liquid chromatography-mass spectrometry (LC-MS), or liquid chromatography with tandem mass spectrometry (LC-MS/MS). These methods have detected Et-IPA in different species for up to 273 days (Ballesteros et al. 2013). Et-IPA has previously been tested in only two marsupial species, the swamp wallaby (Wallabia bicolor) (Fisher and Marks 1997) and the brushtail possum (Trichosurus vulpecula) (Eason et al. 1994). The separate studies used the indirect method of Et-IPA detection and both found plasma iodine levels had returned to baseline levels by day 7. The authors concluded that marsupials might have a physiological pathway that differs from eutherian mammals, resulting in faster excretion of Et-IPA (Fisher and Marks 1997). Both studies concluded that Et-IPA was not a useful biomarker in marsupials because of the short duration of serum detection. Wallabies and possums are from the order Diprotodontia, which are largely herbivorous. No Et-IPA trials have been conducted to date on species in the carnivorous marsupial order Dasyuromorphia.
The Tasmanian devil (Sarcophilus harrisii) is a carnivorous marsupial threatened by the transmissible cancers that cause devil facial tumour disease (DFTD). The prevalence of DFTD has not decreased over time (Lazenby et al. 2018). Local devil densities have declined by 82% and the total devil population by an estimated 68% as a consequence of the disease (Cunningham et al. 2021). Research is underway to develop a preventative DFTD vaccine, and an oral bait on which to deliver it (Flies et al. 2020; Dempsey et al. 2022). The proposed DFTD oral bait vaccine shares many aspects in common with oral rabies bait vaccine programs targeting mesocarnivore wildlife reservoirs (reviewed in Muller et al. 2015). Initial field trials to assess DFTD vaccine effectiveness will require both a bait marker and serology tests that can independently identify animals that consume baits and animals that mount an immune response to the vaccine. Detection of the bait marker but no immune response indicates either a lack of seroconversion by the individual animal, or incomplete bait consumption. The latter relates to how the vaccine and biomarker are incorporated into the bait. For example, the vaccine component of the fishmeal polymer RABORAL V-RG® oral rabies vaccine bait is packaged inside the edible bait, and a biomarker (tetracycline), when used, has been included in the external bait matrix. This has resulted in some discrepancies between tetracycline marking and serological results (Maki et al. 2017).
Recent and ongoing research has reported on the effectiveness and duration of serum IPA as a vaccine bait biomarker for the small Indian mongoose (Urva auropunctata), using sensitive analytical detection methods (Berentsen et al. 2019). In this study we tested the suitability of Et-IPA as a bait marker in Tasmanian devils, using LC-MS/MS to directly measure serum Et-IPA. The LC-MS/MS method described here is similar to previously reported methods (Berentsen et al. 2019, 2020).
Methods
Animals, Et-IPA administration and blood sample collection
Eight captive Tasmanian devils (TD1 to TD8), housed individually or in pairs in outdoor enclosures and complying with the guidelines of Natural Resources and Environment Tasmania (NRET), were used for this study. The devils’ sex, age and weight are summarised in Table 1. Their diet consisted predominantly of possum (Trichosurus vulpecula) and wallaby (Macropus rufogriseus) meat, and water was provided ad libitum. All animal procedures were approved by the University of Tasmania Animal Ethics Committee under 23220 and A0017550.
Devil ID | Sex | Age (years) | Body weight (kg) | Housing | Et-IPA dose mg/devil (mg/kg body weight) | Volume of Et-IPA/corn oil mixture administered and bait used | |
---|---|---|---|---|---|---|---|
TD1 | Male | 4 | 11 | Individual | 50 (4.5) | 1 mL; chick A | |
TD2 | Female | 5 | 7 | Individual | 50 (7.1) | 1 mL; chick A | |
TD3 | Female | 4 | 6.8 | Individual | 50 (7.3) | 1 mL; chick A | |
TD4 | Male | 4 | 9 | Individual | 20 (2.2) | 0.4 mL; chick A | |
TD5 | Female | 4 | 6.2 | Co-housed with TD7 | 5 (0.8) | 0.1 mL; mince ball B | |
TD6 | Male | 3 | 10 | Co-housed with TD8 | 5 (0.5) | 0.1 mL; placebo bait C | |
TD7 | Female | 4 | 7.4 | Co-housed with TD5 | 1 (0.1) | 0.1 mL; mince ball B | |
TD8 | Male | 3 | 9.2 | Co-housed with TD6 | 1 (0.1) | 0.1 mL; placebo bait C |
Et-IPA (α-Ethyl-3-hydroxy-2,4,6-triiodohydrocinnamic acid, CAS 96-84-4, Sigma Aldrich) was dissolved in edible corn oil to make up concentrations of 10 mg/mL and 50 mg/mL. Following previous protocols (Ballesteros et al. 2013), the Et-IPA and corn oil mixture was heated to 90°C to aid dissolution, then returned to room temperature.
Doses of the Et-IPA–corn oil mixture that equated to 50 mg, 20 mg, 5 mg or 1 mg of Et-IPA were incorporated into oral baits. Oral baits consisted of either a day-old chicken carcass, minced wallaby meat or a commercially prepared fish-based bait (Table 1). Each devil received a single dose on day 0. Table 1 details the Et-IPA dose given to each of the devils, and the bait type used. The Et-IPA dose range administered was between 0.1 and 7.3 mg/kg of devil body weight.
TD1 to TD4 had baseline (pretreatment) blood samples collected prior to the Et-IPA oral bait administration. These pretreatment samples were used to rule out sample matrix interference in the analyses. Blood samples were collected from two devils (TD1, TD2) on days 1, 14, 26, 56, 98 and 180 after baiting; from another two devils (TD3, TD4) on days 1, 14, 56 and 198 or 206 after baiting. The remaining four devils (TD5 to TD8) had blood collected on days 1, 14, 28, 56 and 180. Blood collection was carried out under general anaesthesia following NRET protocols. A 3 mL sample of whole blood was collected at each time-point from the jugular vein into clot activating tubes. Samples were centrifuged at 1700g for 5 min and serum pipetted into 1.5 mL cryotubes and stored at −80°C. Serum from an additional captive devil housed under conditions described above, and from eight wild devils (details in Supplementary Table S1) were used for the external calibration step of the Et-IPA detection method described below. Serum samples from the untreated devils were collected opportunistically prior to this trial and stored at −80°C.
Sample preparation
Serum samples were extracted based on previously described protocols (Bruce et al. 2009) where 200 μL of serum was taken and extracted with 800 μL of Methanol:Acetone (1:1, v/v). The mixture was vortexed for 30 s then placed at −20°C for 30 min to aid in protein precipitation. The solution was then centrifuged at 21,130g for 12 min at 4°C, and the supernatant transferred to an ultraperformance liquid chromatography (UPLC) vial for analysis.
To validate and determine the accuracy of our Et-IPA quantification method, control samples were prepared. A spiked recovery sample was produced from a pretreatment serum sample where 50 μL of extraction solution was substituted with a 200 ng/mL working standard of Et-IPA. No-serum extraction samples comprised extraction solution (Methanol:Acetone) alone. These control samples were repeated with each analytical group of experimental samples.
For quantitation of Et-IPA in devil serum samples, matrix matched external calibrations were used. An individual external calibration was constructed from two serum aliquots collected pretreatment for TD1 to TD4, or two serum aliquots from untreated devils for TD5 to TD8. One serum sample was extracted as described above as a blank sample matrix (Blank). The second serum sample was extracted where 300 μL of the extraction solution was substituted with a 2000 ng/mL working standard of Et-IPA (High Spike). Proportions of the Blank and High Spike serum extracts were then combined in various ratios to produce matrix matched external calibration standards over the concentration range of 0.5–5000 ng/mL Et-IPA in serum. Where measured serum concentrations in sample extracts exceeded the external calibration range, sample serum extracts were diluted with the Blank serum extract and reanalysed. Estimation of the Limit of Quantitation (LOQ) for the method was achieved by measurement of the signal to noise ratio (S/N) obtained from the lowest calibration standard (average, n = 4). The LOQ was estimated as the extrapolated concentration that would yield a S/N value of 10.
Sample analysis
Sample analysis was undertaken using a Waters Acquity® H-class UPLC system (Waters Corporation, Milford, MA). Chromatography was performed using a Waters Acquity VanGuard C18 precolumn (5 × 2.1 mm) coupled to a Waters Acquity C18 analytical column (100 × 2.1 mm × 1.7 μm particles). The UPLC was operated with a mobile phase consisting of 1.0% (v/v) Acetic acid in water (Solvent A) and Acetonitrile (Solvent B). Elution utilised a gradient program beginning with 50% Solvent B held for 0.5 min, moving to 95% B at 4.0 min, held for 1.0 min. At 5.5 min, composition was returned to 50% B and the column equilibrated for a further 3 min. The flow rate was 0.35 mL/min and the column was held at 35°C. Injection volume was 2 μL. Typical retention time for Et-IPA was 3.0 min.
The UPLC was coupled to a Waters Xevo TQ triple quadrupole mass spectrometer (Waters Corporation). Analyses were undertaken using simultaneous single ion monitoring (SIM) and multiple reaction monitoring (MRM) in negative electrospray ionisation mode (refer to Supplementary Material).
Results
All of the devils readily consumed the Et-IPA-laden baits either immediately (TD2, TD3, TD5, TD6, TD7, TD8) or within 30 min of offering (TD1, TD4).
The LC-MS/MS analytical method was successfully validated for use with devil serum. No-serum extractions together with serum extractions from untreated and pretreatment devils confirmed there were no sample matrix interferences affecting the analyses (Fig. 1a). Recovery of Et-IPA from the spiked recovery samples was achieved at 70 ± 3% (n = 3) over the course of three independent (interday) analyses and LOQ for the method was estimated at 0.05 ng/mL. External, matrix matched calibration was successfully demonstrated over the concentration range 0.5–5000 ng/mL of Et-IPA in devil serum. A linear correlation coefficient of 0.9974 ± 0.0013 (n = 3) was achieved, with an example shown in Fig. S1.
Chromatograms of serum extract analysis from TD1 samples: pretreatment (panel a), and 1 day after ingestion of Et-IPA (panel b). For both panels, upper chromatogram represents MRM1 [M-H]− precursor (m/z) 570.7 to product (m/z) 442.8. Middle chromatogram represents MRM2 [M-H]− (m/z) 570.7 to (m/z) 126.8. Lower chromatogram represents SIM [M-H]− (m/z) 570.7. For all chromatograms, time in minutes is shown on the x axis, and intensity as a percentage is shown on the y axis.
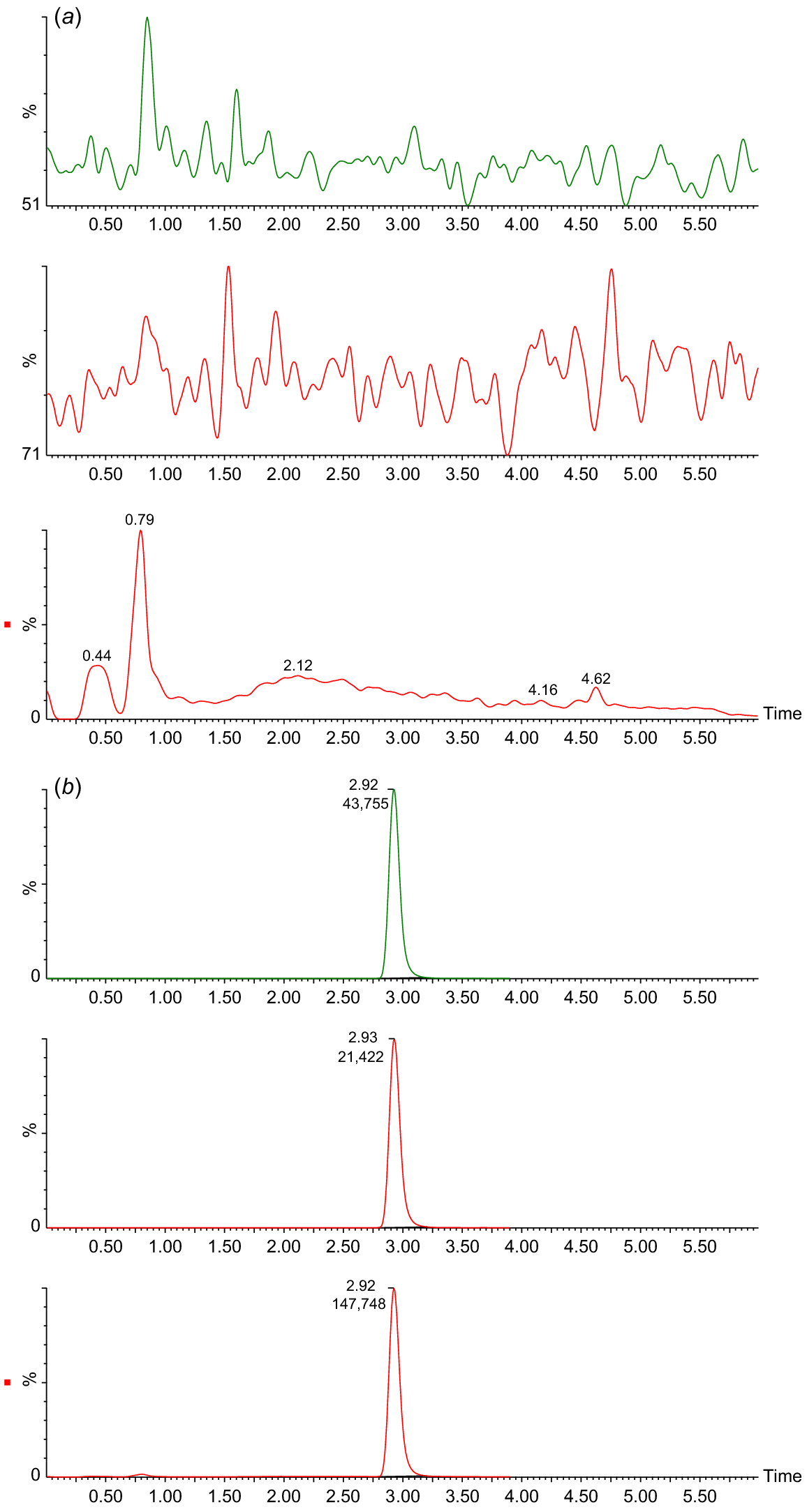
Fig. 1b shows a clear peak in all SIM and MRM chromatograms at retention time 2.92 min, identifying the presence of Et-IPA. The highest concentration of serum Et-IPA was observed for TD3 (15,152 ng/mL) on day 1 after ingestion. This was high enough to require dilution of the serum extracts with the Blank extract, to maintain the appropriate matrix effect and allow the sample into the calibration range. Serum Et-IPA was detected in all eight devils the day following bait offering. Quantifiable levels of serum Et-IPA remained in TD1 to TD5 at the final blood collection time-point of 6 months. For TD6 to TD8, serum Et-IPA was detected up until the second last time-point of 2 months (Fig. 2). Serum Et-IPA declined in all eight devils over time. This showed as a distinct linear pattern when simple linear regression analysis was applied to the log-transformed serum Et-IPA concentration values (Fig. 2, Table S2). At three different time-points (days 1, 56 and 180–206), simple linear regression analysis was performed on the Et-IPA mg/kg body weight dose given to each devil, and the resultant log-transformed serum Et-IPA concentration (Fig. 3, Table S3).
Serum concentration of Et-IPA over 6 months after ingestion. Serum concentrations were measured in ng/mL and shown on log10 scale. The colour of the symbol indicates the dose of Et-IPA per devil. Black, 50 mg; grey, 20 mg; red, 5 mg; blue, 1 mg. Devil details, including dose of Et-IPA in mg per kg of devil body weight, are: TD1, 4 year old male, 4.5 mg/kg; TD2, 5 year old female, 7.1 mg/kg; TD3, 4 year old female, 7.3 mg/kg; TD4, 4 year old male, 2.2 mg/kg; TD5, 4 year old female, 0.8 mg/kg; TD6, 3 year old male, 0.5 mg/kg; TD7, 4 year old female, 0.1 mg/kg; TD8, 3 year old male, 0.1 mg/kg. Simple linear regression analysis was performed on the log-transformed data for each individual and the lines of best fit are shown on the graph. See Table S2 for R2 values and line equations.
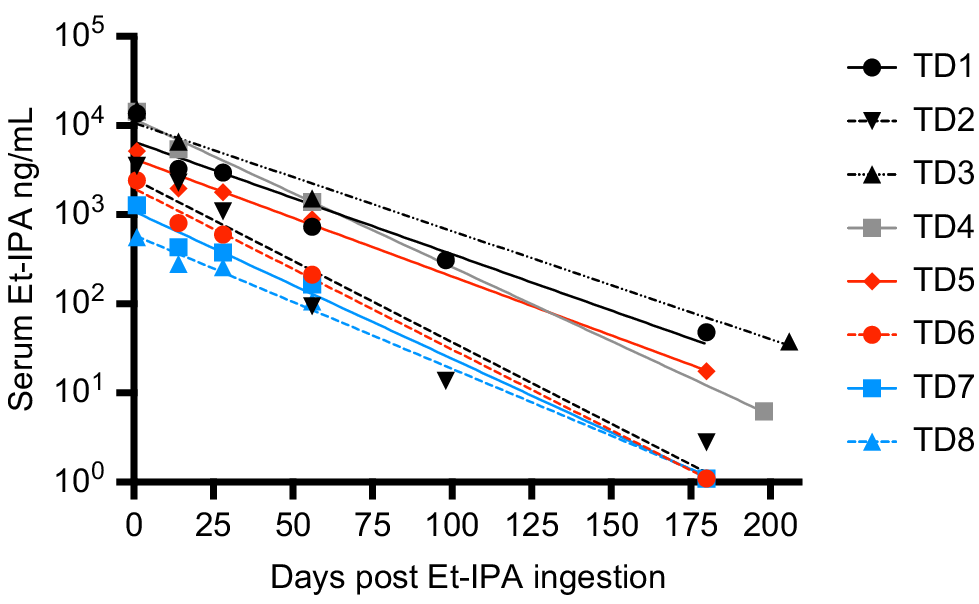
Et-IPA dose and resultant serum Et-IPA concentration for each devil at three different time points. Serum concentrations were measured in ng/mL and shown on log10 scale. Simple linear regression analysis was performed on the log-transformed data for each time-point and the lines of best fit are shown on the graph. See Table S3 for R2 values and line equations.
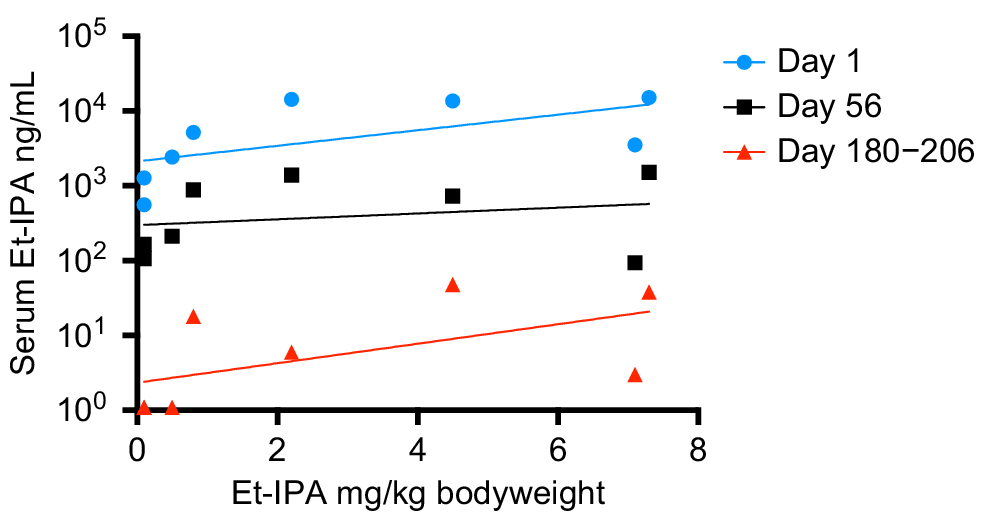
Discussion
This study is the first to demonstrate that Et-IPA is a useful biomarker in a marsupial species. We used the LC-MS/MS method to directly quantify Et-IPA in the serum of Tasmanian devils for up to 6 months following oral administration of between 0.1 and 7.3 mg/kg Et-IPA in a bait. In previous studies, serum iodine was not detectable in two other marsupial species, swamp wallabies and brushtail possums, 7 days after Et-IPA ingestion despite administration of relatively high doses of Et-IPA (up to 30 mg/kg for wallabies). It was hypothesised the short window of iodine detection was due to a difference in Et-IPA metabolism between marsupial and eutherian mammals (Fisher and Marks 1997). Those studies used the indirect method of serum iodine measurement to determine the duration of Et-IPA as a biomarker in contrast to the more sensitive LC-MS/MS method described here.
Tasmanian devils are carnivorous, in contrast to the herbivorous wallabies and omnivorous possums, which may have some effect on Et-IPA metabolism. It is possible that the LC-MS/MS method used here would be more sensitive than serum iodine measurement in detecting Et-IPA in these other marsupial species. The LC-MS/MS method described here used a similar instrumental approach to that described previously (Berentsen et al. 2019) but we used a different experimental protocol, most notably with our approach to quantitation. The previous study (Berentsen et al. 2019) detected and differentiated between Et-IPA and another IPA derivative, methyl-IPA, in small Indian mongoose serum samples. That study used external calibration in a water matrix and then corrected for recovery and matrix effect by the use of methyl-IPA as the internal standard. We were unable to find a commercial supplier of methyl-IPA, and so our approach was to use a fully matrix-matched external calibration of Et-IPA in devil serum. This showed no Et-IPA in the negative controls but a clear signal in serum containing the Et-IPA spike and serum from devils consuming Et-IPA.
Of note in this cohort of devils was that the mg/kg body weight dose of Et-IPA was not predictive of the level of serum Et-IPA detected. This suggests detection of serum Et-IPA in devils will best serve as a binary marker for whether ingestion of Et-IPA-laden baits occurred in the preceeding 2 months, rather than allowing for an estimate of the number of baits ingested. However, it was evident from this study that serum Et-IPA declines in devils over time. This was demonstrated as a linear relationship between the log-transformed serum Et-IPA data and time. Therefore, if individual devils were sampled at more than one time-point in the 2 month period after bait drop, and an increased serum Et-IPA level was detected at the later time-points, this would indicate consumption of additional bait(s) between sample collections. This is relevant because of the possibility that vaccine regimens that more faithfully mimic the temporal dynamics of natural infections could provide better protection against disease. It is plausible that consumption of multiple oral bait vaccines within a 2 month period will trigger tiered escalation of immune responses that yield superior protection to single bolus regimens.
Methyl-IPA has been incorporated into field trials that require distinction between the timing and/or location of bait uptake (Berentsen et al. 2020). Rhodamine B has been trialled in Tasmanian devils (Bell et al. 2020) and was detectable in whiskers when tested a minimum of 2 weeks after ingestion. If a current commercial supplier of methyl-IPA can be identified, and/or if the previously mentioned limitations of rhodamine B can be overcome, either could be used in combination with Et-IPA for temporal and spatial bait uptake field studies. Future oral DFTD bait vaccination trials for Tasmanian devils that include an immune priming component followed by an immune boosting component could benefit from validation of multiple serum biomarkers.
Ethics
Ethical approval for this study was provided by the University of Tasmania’s Animal Ethics Committee, 23220 and A0017550.
Data availability
The data used in this study will be accessible as supplementary material. A preprint version of this article is available at https://www.biorxiv.org/content/10.1101/2021.12.14.472710v1.
Declaration of funding
This research was supported by the University of Tasmania Foundation through funds raised by the Save the Tasmanian Devil Appeal; a Charitable organisation from the Principality of Liechtenstein; a Select Foundation Senior Research Fellowship; and a Wildcare Tasmanian Nature Conservation Fund Grant.
Acknowledgements
We thank Ginny Ralph and Zoodoo for the care of the devils used in this study; Are Berentsen and Shylo Johnson for their technical advice; and Linton Staples and Animal Control Technologies for provision of placebo baits.
References
Baer GM (1976) The oral rabies immunization of foxes and dogs with sausage baits. Developments in Biological Standardisation 33, 417-423.
| Google Scholar |
Ballesteros C, Sage M, Fisher P, Massei G, Mateo R, De La Fuente J, Rossi S, Gortázar C (2013) Iophenoxic acid as a bait marker for wild mammals: efficacy and safety considerations. Mammal Review 43, 156-166.
| Crossref | Google Scholar |
Bell O, Jones ME, Ruiz-Aravena M, Hamede RK, Bearhop S, McDonald RA (2020) Age-related variation in the trophic characteristics of a marsupial carnivore, the Tasmanian devil Sarcophilus harrisii. Ecology and Evolution 10, 7861-7871.
| Crossref | Google Scholar | PubMed |
Berentsen AR, Sugihara RT, Payne CG, Leinbach I, Volker SF, Vos A, Ortmann S, Gilbert AT (2019) Analysis of iophenoxic acid analogues in small Indian mongoose (Herpestes auropunctatus) sera for use as an oral rabies vaccination biological marker. Journal of Visualized Experiments 147, e59373.
| Crossref | Google Scholar |
Berentsen AR, Chipman RB, Nelson KM, Gruver KS, Boyd F, Volker SF, Davis AJ, Vos A, Ortmann S, Gilbert AT (2020) Placebo oral rabies vaccine bait uptake by small Indian mongooses (Herpestes auropunctatus) in southwestern Puerto Rico. Journal of Wildlife Diseases 56, 452-45.
| Crossref | Google Scholar | PubMed |
Bruce SJ, Tavazzi I, Parisod V, Rezzi S, Kochhar S, Guy PA (2009) Investigation of human blood plasma sample preparation for performing metabolomics using ultrahigh performance liquid chromatography/mass spectrometry. Analytical Chemistry 81, 3285-3296.
| Crossref | Google Scholar | PubMed |
Cunningham CX, Comte S, McCallum H, Hamilton DG, Hamede R, Storfer A, Hollings T, Ruiz-Aravena M, Kerlin DH, Brook BW, Hocking G, Jones ME (2021) Quantifying 25 years of disease-caused declines in Tasmanian devil populations: host density drives spatial pathogen spread. Ecology Letters 24, 958-969.
| Crossref | Google Scholar | PubMed |
Dempsey S, Pye RJ, Gilbert AT, Fountain-Jones NM, Moffat JM, Benson-Amram S, Smyser TJ, Flies AS (2022) Evaluation of oral baits and distribution methods for Tasmanian devils (Sarcophilus harrisii). Wildlife Research 50, 807-819.
| Crossref | Google Scholar |
Eason CT, Batcheler D, Frampton CM (1994) Comparative pharmacokinetics of iophenoxic acid in cats and brushtail possums. Wildlife Research 21, 377-380.
| Crossref | Google Scholar |
Fisher P (1999) Review of using rhodamine B as a marker for wildlife studies. Wildlife Society Bulletin 27, 318-329.
| Google Scholar |
Fisher PM, Marks CA (1997) Evaluation of iophenoxic acid as a biomarker for swamp wallabies (Wallabia bicolor). Wildlife Research 24, 97-103.
| Crossref | Google Scholar |
Flies AS, Flies EJ, Fox S, Gilbert A, Johnson SR, Liu G-S, Lyons AB, Patchett AL, Pemberton D, Pye RJ (2020) An oral bait vaccination approach for the Tasmanian devil facial tumor diseases. Expert Review of Vaccines 19, 1-10.
| Crossref | Google Scholar | PubMed |
Jacoblinnert K, Imholt C, Schenke D, Jacob J (2021) Ethyl-iophenoxic acid as a quantitative bait marker for small mammals. Integrative Zoology 17, 981-990 10.1111/1749-4877.12547.
| Google Scholar |
Lazenby BT, Tobler MW, Brown WE, Hawkins CE, Hocking GJ, Hume F, Huxtable S, Iles P, Jones ME, Lawrence C, Thalmann S, Wise P, Williams H, Fox S, Pemberton D (2018) Density trends and demographic signals uncover the long-term impact of transmissible cancer in Tasmanian devils. Journal of Applied Ecology 55, 1368-1379.
| Crossref | Google Scholar | PubMed |
Maki J, Guiot A-L, Aubert M, Brochier B, Cliquet F, Hanlon CA, King R, Oertli EH, Rupprecht CE, Schumacher C, Slate D, Yakobson B, Wohlers A, Lankau EW (2017) Oral vaccination of wildlife using a vaccinia-rabies-glycoprotein recombinant virus vaccine (RABORAL V-RG®): a global review. Veterinary Research 48(1), 57.
| Crossref | Google Scholar | PubMed |
Muller TF, Schroder R, Wysocki P, Mettenleiter TC, Freuling CM (2015) Spatio-temporal use of oral rabies vaccines in fox rabies elimination programmes in Europe. PLoS Neglected Tropical Diseases 9, e0003953.
| Crossref | Google Scholar | PubMed |
Philip J (2021) A historical review of Australian aerial vertebrate pest control, targeting dingoes and wild dogs 1946–2019. Australian Zoologist 41, 580-592.
| Crossref | Google Scholar |
Qureshi T, Drawe DL, Davis DS, Craig TM (1994) Use of bait containing triclabendazole to treat Fascioloides magna infections in free ranging white-tailed deer. Journal of Wildlife Diseases 30, 346-350.
| Crossref | Google Scholar | PubMed |
Van Brackle MD, Linhart SB, Creekmore TE, Nettles VF, Marchinton RL (1994) Oral biomarking of white-tailed deer with tetracycline. Wildlife Society Bulletin (1973–2006) 22, 483-488.
| Google Scholar |