Assessing target and non-target species interactions with buried non-toxic meat baits across fire mosaics
Rachel T. Mason


A
B
Abstract
Lethal 1080 baiting to control invasive predators, or to reduce livestock predation by canids, is a widespread management tool used throughout Australia and New Zealand. Techniques to reduce the impact of 1080 baiting on non-target species exist, but their efficacy, including effects of environmental variation and disturbance on baiting outcomes, remains poorly understood.
We aimed to experimentally quantify the extent to which target and non-target species dig up and consume buried baits, and to examine how habitat variation and fire affect such interactions.
Remote cameras were deployed for 70 days to monitor the fate of non-toxic baits in the semi-arid Big Desert-Wyperfeld region of southeastern Australia. Species digging up or eating baits (collectively ‘bait interactions’) were identified, and the effects of environmental factors on bait interaction rates were assessed.
Non-target species accounted for 128 (88%) of 146 total bait interactions, primarily native mice species. Target species interacted with fewer baits and took longer to record bait interactions, with foxes (Vulpes vulpes) averaging 41 days for 17 bait interactions and one dingo (Canis familiaris/Canis dingo) taking 60 days, whereas native mice interactions occurred after ~13 days. At sites where foxes and dingoes were detected, both target species almost always interacted with baits (83%), whereas non-target species interacted with baits at less than half of the sites they were detected (42%). Areas with greater variation in fire frequency (pyrodiversity) and mid-successional vegetation were associated with more native mice-bait interactions.
Non-target species interacted with baits more often and sooner than target species, suggesting efforts to reduce predator populations could have inadvertent effects on other species. The influence of fire and vegetation variables on bait interaction rates also highlights the importance of accounting for landscape features when designing lethal control programs.
Predator control can benefit native wildlife, and help to reduce livestock loss; however, managers must account for environmental factors that may influence which species are most likely to be affected by toxic baits, and by extension possible broader impacts on ecosystems. Monitoring to assess baiting outcomes and limit the negative consequences for non-target species constitutes best practice.
Keywords: Dingo, European red fox, fire management, invasive species control, integrated ecosystem management, lethal predator control, non-target impacts, pyrodiversity, sodium fluoroacetate (1080).
Introduction
Lethal control of animal populations through poison baiting is a widespread tool used in threatened species conservation, pest management, and for reducing human-wildlife conflict (reviewed in Taggart et al. 2023). Invasive and native predators can have detrimental effects on agricultural production of crops and livestock and/or on native wildlife and ecosystems (Saunders et al. 2010; Capizzi et al. 2014; Doherty et al. 2016). Predator management often centres on lethal control with poison baiting for the protection of agricultural production values and threatened species (Reddiex et al. 2006; Philip 2019; Taggart et al. 2023). Various substances have been trialled for use in lethal baiting, but sodium fluoroacetate (hereafter: 1080) remains one of the most frequently used toxins for lethal control programs (Taggart et al. 2023), especially in Australia and New Zealand.
In Australia, lethal control of animals with poison baiting is common on both private and public lands (Philip 2019). Australia has the most extensive use of poison baiting globally, the majority of which is carried out with 1080 (Taggart et al. 2023), primarily targeting dingoes (Canis familiaris/Canis dingo) and European red foxes (Vulpes vulpes). Governments and private landholders invest tens of millions of dollars in this management action annually (Bomford and Hart 2002; McLeod 2004). The widespread use of 1080 is in part due to the natural occurrence of fluoroacetate in Gastrolobium plant species in Australia, primarily in the south-west of the country. The coevolution of Gastrolobium and native herbivorous and omnivorous species from this region means native wildlife typically have a relatively high tolerance to this chemical compound (Mead et al. 1985). Additionally, the lower basal metabolic rate of marsupial species compared to eutherians (Dawson and Hulbert 1970) may contribute to a higher innate tolerance for the toxin due to a more gradual impact on mitochondrial functioning (Twigg and King 1991). Hence, many introduced and invasive species have a much higher sensitivity to 1080, and as such, 1080 is generally considered moderately target-specific when used to control eutherian species such as foxes, feral cats (Felis catus), European rabbits (Oryctolagus cuniculus), and dingoes (Calver et al. 1989).
There are a range of concerns surrounding the ethics (Littin et al. 2014; Wallach et al. 2015), efficacy (Dundas et al. 2014; Newsome et al. 2014; Allsop et al. 2017; Kreplins et al. 2018) and outcomes (Wallach et al. 2009; Marlow et al. 2015; Campbell et al. 2019; Rees et al. 2023) of poison baiting with 1080. In particular, the impact of 1080 baiting on non-target species has been investigated as a cause for concern around its continued use (APVMA 2008). Non-target bait interference is reported in nearly 30% of control program outcomes (Taggart et al. 2023). However, monitoring and quantifying the impact of poison baiting on non-target individuals is not routine and can be practically and logistically challenging (Glen et al. 2007).
Risks to non-target species from poison baiting remain insufficiently understood (Glen et al. 2007; Ross and Eason 2022). Some species, such as the spotted-tailed quoll (Dasyurus maculatus) and eastern quoll (Dasyurus viverrinus), have been the focus of substantial research to determine their risk of unintentional poisoning (Mcilroy 1981; Belcher 1998; Claridge et al. 2006). While individual mortalities of quolls exposed to baiting have been recorded, no negative impacts have been demonstrated at a population level (Körtner et al. 2003; Murray and Poore 2004; Körtner and Watson 2005; Claridge et al. 2021). Most other native species are assumed to be low risk, either due to their body size, sensitivity to 1080, or the relative risk of individual animals encountering and consuming baits when compared to the population-level risk posed by targeted predator species (APVMA 2008).
Despite the presumed low risk of baiting to most non-target species, a number of techniques are recommended or legislated in different jurisdictions across Australia aimed at minimising non-target harm. These ‘best practice’ baiting methods include – reducing palatability of the bait matrix to non-target species, by using dried, cooked or manufactured meat rather than fresh meat (Allen et al. 1989; Calver et al. 1989; Saunders et al. 1995); injecting blue or green dye into baits to reduce visual appeal for non-target species (particularly birds; Cowan and Crowell 2017); deploying baits in late autumn or early spring, to align with lower activity periods for varanid species (Thomson 1986; Robley et al. 2009); and reducing the ability of non-target species to access baits by burying them, either below ground or within a mound of sand/earth to a depth of 10 cm (Allen et al. 1989; Thomson and Kok 2002; Saunders and McLeod 2007; Moseby et al. 2011). Bait burial 8–10 cm below the surface is a requirement in eastern Australian states including Victoria due to concerns about non-target harm from surface-laid baits (Saunders and McLeod 2007).
How these ‘best practice’ methods affect the outcomes of baiting programs is not well-established, and when implementing methods like bait burial, reported bait take rates for target species can vary significantly. Reported uptake of buried baits by foxes fluctuates widely, between 1% (Dundas et al. 2014) and 35% (Thompson and Fleming 1994; Saunders et al. 1999). A similarly broad range of uptake rates has been reported for dingoes across previous studies of buried baits, from <1% (Glen and Dickman 2003a; Kreplins et al. 2018) to 17% (Allen et al. 1989). Non-target species are often reported as relatively unimpacted when baits are buried (Allen et al. 1989; Fleming 1996), but in addition to quolls (Belcher 1998; Glen and Dickman 2003a), other species that have been reported as capable of digging up baits buried to a depth of 10 cm include corvids (Allen et al. 1989; Dexter and Meek 1998), yellow-footed antechinus (Antechinus flavipes; Fairbridge et al. 2003), brush-tailed phascogales (Phascogale tapoatafa; Fairbridge et al. 2003), swamp rats (Rattus lutreolus; Dexter and Meek 1998), and bush rats (Rattus fuscipes; Fairbridge et al. 2000). Despite being suggested or required in many Australian states, the efficacy of these baiting methods for actually preventing negative impacts on non-target species is not well understood.
An often-overlooked aspect that may affect baiting outcomes is the influence of environmental factors, including fire history and habitat variation. Many environmental factors have been shown to alter predator behaviour and habitat use, including pyrodiversity (Bliege Bird et al. 2018), time since fire (McGregor et al. 2014; Bliege Bird et al. 2018; Geary et al. 2018; Doherty et al. 2023), and vegetation complexity (McGregor et al. 2014; Hradsky et al. 2017a; Stobo-Wilson et al. 2020). In turn, these factors may also influence and regulate prey populations (Griffiths and Brook 2014; Bennison et al. 2018; Nimmo et al. 2019; Sukma et al. 2019). Despite these effects on predators and prey, there has been limited investigation into the relationship between environmental variation and predator control outcomes for target and non-target species. Lethal predator control is regularly recommended or implemented in response to bushfires and planned burns (Robley et al. 2012; DENR 2017; Hradsky 2020), but how fire (and subsequent changes to vegetation) could be altering the outcomes of predator control programs is poorly understood.
We therefore aimed to evaluate the potential for non-target species to be impacted by ‘best practice’ 1080 bait deployment in a semi-arid mallee ecosystem, through monitoring interactions with replica (non-toxic) meat baits in a simulated canid-control program. We aimed to determine how fire history and vegetation characteristics influence bait uptake rates by target and non-target species. Specifically, we aimed to determine:
Which target and non-target species interact with buried baits.
Whether the relative ratio of interactions with baits compared to detections at bait sites would differ between target and non-target species.
How long after bait deployment target and non-target species would first interact with baits.
How environmental factors (time since fire, pyrodiversity, and vegetation cover) influence target and non-target species interacting with baits.
Based on previously reported target and non-target bait uptake rates, we predicted that foxes (Thompson and Fleming 1994) and dingoes (Allen et al. 1989) would primarily interact with baits, and that cats (Moseby et al. 2011), corvids (Dexter and Meek 1998), varanids (Moseby et al. 2011), and small mammals (Moseby et al. 2011) would also interact with buried baits, but to a lesser extent. We also predicted that target species would interact with baits in less time (Fleming 1996), and with greater frequency relative to the number of times they were detected (Thompson and Fleming 1994) than non-target species. Based on observed relationships between environmental variables and animal abundance and behaviour, we also predicted that target species would be more likely to interact with baits in recently burned areas with reduced vegetation cover (Robley et al. 2012; Geary et al. 2018), and that non-target species may be more likely to interact with baits in long-unburned (Griffiths and Brook 2014), pyrodiverse (Nimmo et al. 2019) landscapes with greater vegetation cover (Geary et al. 2018; Sukma et al. 2019).
Materials and methods
Study area
Our study was located in the Big Desert State Forest, Big Desert Wilderness Area, and Wyperfeld National Park (hereafter called the BDW complex), which cumulatively make up ~7000 km2 of protected semi-arid mallee and heathland habitat in north-western Victoria on Wotjobaluk Country (Fig. 1). The BDW complex receives an annual average of ~350 mm of rain (Australian Bureau of Meteorology, http://www.bom.gov.au/), but with high variability influenced by El Niño/Southern Oscillation (ENSO) phases. The area received lower than average rainfall in 2021, with ~260 mm, but a much greater than average ~550 mm across 2022, during a strong La Niña ENSO phase (Australian Bureau of Meteorology).
(a) Locations of 48 cameras deployed within the Big Desert-Wyperfeld complex (south-eastern Australia) to monitor target and non-target species interactions with non-toxic liver baits. Camera and bait locations are denoted by circles. Areas marked with red indicate where lethal control with toxic 1080 baits has been conducted by land managers within the 5 years prior to this study. Tracks marked with black lines delineate public-private land boundaries where lethal control has not been conducted by land managers but may have been conducted by private landholders. Internal unbaited park tracks are marked with grey lines. (b) Set-up of wildlife camera and burial method of non-toxic liver bait at each camera deployment site.
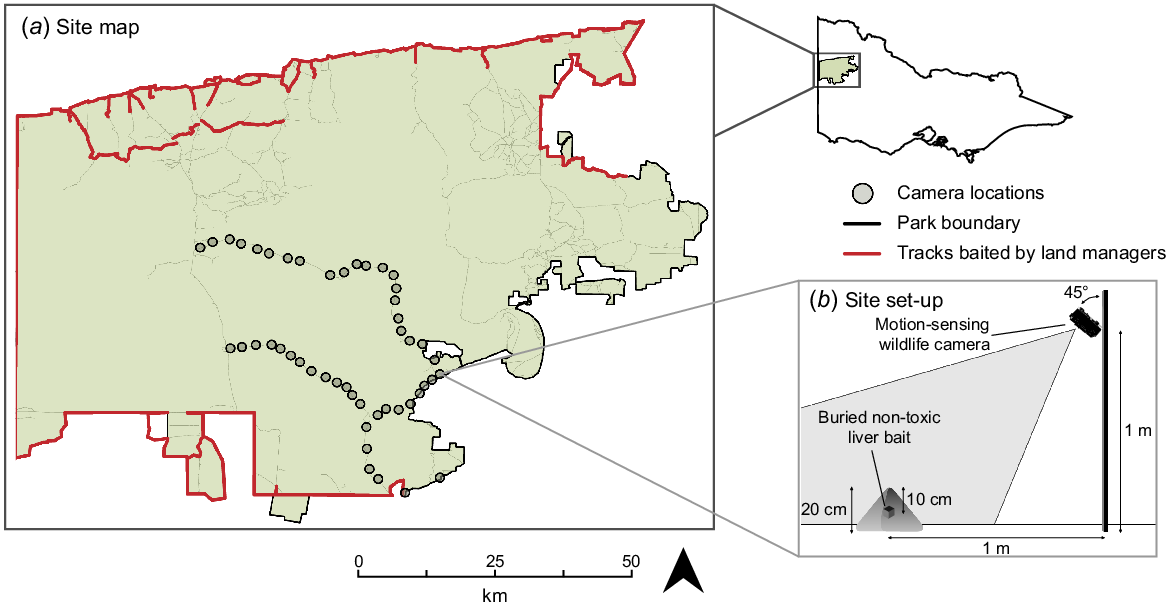
Fire is an important driver in the BDW complex (Clarke et al. 2021), with large scale bushfires occurring at ~15-year intervals, and >2% of the BDW complex burned annually (Pausas and Bradstock 2007). The intense flammability of mallee and heath vegetation, combined with strong horizontal and vertical continuity in vegetation structure, has often resulted in bushfires spreading across a large extent of the park complex, resulting in homogeneity in the growth stages of recovering vegetation (Clarke et al. 2021).
Predator management has been conducted by management organisations within the BDW complex for at least the last 20 years (Robley and Choquenot 2002), although intermittent lethal control of dingoes (also known as ‘Wilkerr’ in Wergaia language) has been ongoing since early British colonisation of the area in the 1860–1870s (Alpin et al. 1987). Previously, baiting for foxes and dingoes was conducted yearly by Parks Victoria and the Victorian Department of Energy, Environment and Climate Change (DEECA) park management staff. Baiting was conducted through the deployment of 100–200 g cubes of boiled liver injected with aqueous 1080, with the concentration for fox baits deployed in Victoria 3 mg/bait, and 6 mg/bait for dingoes (APVMA 2008). Predator baiting in the BDW complex was performed to reduce the potential predation impact on vulnerable species such as the malleefowl (Leipoa ocellata) and livestock on neighbouring private properties. Fox baiting has been primarily conducted throughout the north of the BDW complex (Fig. 1). Dingo baiting was conducted within a three kilometre ‘buffer zone’ which extends from the public-private land interface and into the BDW complex (Fig. 1), under the Dingo Unprotection Order of Victoria’s Wildlife Act 1975 (S McLean, pers. comm. 2021), up until March 2024 when the Unprotection Order in this region ceased. Previously, both dingo and fox control were widely carried out on private property bordering the BDW complex, but this is no longer permitted for dingoes on private land as of March 2024.
Bait and camera deployment
To determine the rate of interaction of target and non-target species with liver baits deployed in the BDW complex, cameras were deployed to monitor buried baits. Monitoring of sand-pads for footprints and digging signs is commonly used to assess bait uptake (e.g. Allen et al. 1989; Dexter and Meek 1998; Thomson and Kok 2002), but there are examples where sand-pad monitoring has resulted in overestimations of the bait uptake rate by target species (Robley et al. 2009; Robley et al. 2011), and differences between bait take rates reported by sand-pad monitoring and cameras (Glen and Dickman 2003b; Robley et al. 2011; Kreplins et al. 2018). Additionally, wet weather and extensive digging by foxes are known to obscure signs of other species at bait stations (Belcher 1998; Fairbridge et al. 2000; Glen et al. 2007). Hence, to ensure accurate identification of species at bait sites, motion activated wildlife cameras were used in our study.
A total of 48 sites were selected along a gradient from the boundary to the interior of the BDW complex, which is correlated with distance from areas where lethal baits had been deployed in the 5 years prior to our study (Fig. 1). Sites were a minimum of 1.5 km apart and 50 m (±10 m) adjacent to tracks in vegetated areas. Baits were deployed to mirror ‘best practice’ methods for reducing non-target uptake in Victoria – non-toxic Foxoff™ free-feed cooked liver baits (Animal Control Technologies Pty Ltd), which had been dyed blue, were buried to a depth of 10 cm into a mound of sand 20 cm high in mid-late autumn (Fig. 1). This aligned with the timing and methodology of lethal predator control conducted annually in the BDW complex by DEECA (S McLean, pers. comm. 2021). Immediately adjacent to the buried liver baits, one metre from the centre of the sand mound, a Swift Enduro wildlife monitoring camera was attached to a wooden stake one metre above the ground. Cameras were mounted facing south, to minimise solar glare, at a 45-degree angle with the sand mound at the centre of the camera field of view (Fig. 1). Camera sensitivity was set to high, with no quiet period between triggers, and five images were captured one second apart for every trigger. Cameras were deployed at the same time baits were buried and were retrieved 70 days later. This deployment length was selected to capture the entire period over which buried lethal baits likely retain a 1080 dose higher than the median lethal dose (LD50) for an adult fox under typical environmental conditions (Saunders et al. 2000; Allsop et al. 2017). All cameras were still functioning when retrieved.
Image labelling
A total of 36,129 images were captured over 3360 camera trap nights. Images were imported into open-source image-labelling program Camelot (Hendry and Mann 2018). Animals detected in camera images were identified to species-level wherever possible, and two behaviours were classified: ‘digging’, where a species was actively digging into the centre of the mound of sand containing the buried liver; and ‘eating’, where species were seen to be consuming some or all of the bait.
Digging and eating behaviours of small mammal species were difficult to differentiate accurately and consistently, particularly in infrared images taken at night, so consequently ‘digging’ and ‘eating’ behaviours were grouped and collectively labelled as ‘bait interactions’. This allowed us to investigate instances where species directly consumed baits, but also instances where species contributed to making a buried bait available (exposing it or bringing it to the sand surface) for potential consumption by other animals. Detections and bait interactions were defined as independent when there was greater than 20 min between successive images of the same species at a site. This independence threshold accommodated species that were more likely to linger in front of cameras for extended periods, such as large herbivores, but reduced the likelihood of incorrectly filtering out valid observations of smaller, fast-moving species such as rodents (Rendall et al. 2014; Meek et al. 2014; Iannarilli et al. 2021).
Environmental variables
Five environmental variables were measured across the BDW complex to investigate variation in species’ interactions with baits: park boundary distance, vegetation cover, fire frequency, pyrodiversity, and time since fire. Variables were either measured as straight-line distance (park boundary distance) or calculated within a 750 m radius around each camera location (vegetation cover, fire frequency, pyrodiversity, and time since fire). This buffer was chosen to capture contrast in environmental variables over a meaningful area for both larger and wide-ranging species (e.g. dingoes, foxes and kangaroos) and more range-restricted species (e.g. rodents; Nimmo et al. 2019). Buffers around sites that bordered agricultural land excluded farmland from the calculations of environmental variable values. As we were measuring bait interactions within a remnant semi-arid mallee ecosystem, environmental conditions in adjacent farmland were excluded as not reflective of conditions inside the park.
To capture variation in species’ response based on distance to adjacent farmland (i.e. ‘edge effects’; Yahner 1988; May and Norton 1996), a measure of the straight-line distance in metres from the bait deployment location to the nearest boundary of the park complex was recorded for each site.
Vegetation cover was determined with a rasterised layer containing overlapping projected foliage cover of indigenous plant lifeforms occurring within the study system, including all native species of trees, shrubs, chenopods, tussock grasses, hummock grasses, and forbs (M White, pers. comm. 2024). This vegetation cover layer was generated from a subset of plant lifeform distributions mapped as part of a separate project (M White, pers. comm. 2024), where weighted modelling of more than 57,000 field measured vegetation plots resulted in spatial models of ‘benchmark’ (‘pre−1750’) lifeform cover across south-eastern Australia. Percent cover of the perennial life-form types occurring in Victorian mallee dune field ecosystems were summed to generate a total vegetation cover score and clipped by the extent of remnant native vegetation landcover (to account for areas which have been cleared for agriculture or infrastructure post−1750). As mallee ecosystems are generally sparsely vegetated, cover scores for 40 × 40 metre grid cells ranged from ~10% to 29%. Mean vegetation cover was calculated across buffers surrounding each site.
Three fire history variables were calculated across site buffers. All fire history variables included both planned burns and bushfires, as most fires in mallee ecosystems are stand-replacing (Haslem et al. 2011) and as such, there is similar fire intensity and ecosystem outcomes for both. All fires in the recorded history of the BDW complex between 1930 and March 2022 were included in the analysis. The three variables included: fire frequency, pyrodiversity, and time since fire. Fire frequency represented the number of times each site had previously burned. 40 × 40 metre grid cells across the entire park were assigned a value based on the number of burns overlapping each cell, and the mean frequency of burns was calculated for each site. Pyrodiversity represented the variation in fire frequency surrounding each site. Previous research, including within the same study system (Geary et al. 2018), has identified that variability in fire age classes within a defined area (i.e. ‘pyrodiversity’) can be beneficial to and increase the abundance of some species, particularly species that benefit from variation in the level of vegetation cover (e.g. silky mice (Pseudomys apodemoides) in the BDW complex; see Geary et al. 2018). The pyrodiversity score for each site was generated by taking the standard deviation of fire frequency. Time since fire represented the proportion of each site that was recently burned (fire < 11 years ago), of a mid-successional fire age (fire 11–35 years ago), and long-unburned (fire > 35 years ago; fire intervals adapted from Geary et al. 2018). To reduce potential correlation in predictor variables, a Principal Component Analysis (PCA) was conducted on the three proportions. Two Principal Components (PCs) that explained 100% of the variance in the three categories were generated (Fig. S1). The first component (hereafter ‘mid to late successional’ PC; Eigenvalue = 1.95) represented a gradient from mid-successional time since fire (low values) to areas that are long-unburned (high values). The second component (hereafter ‘recently burned’ PC; Eigenvalue = 1.05) described a gradient from recently burned areas (low values) to areas associated with either mid-successional or long-unburned fire ages (high values). PC scores were approximately equally weighted across the original recently burned, mid-successional, and long-unburned categories (Fig. S1).
Statistical approach and analysis
To determine whether the number of detections of each species was proportional to the number of bait interactions, and whether this detection–bait interaction ratio differed between target and non-target species, detections and bait interactions by all species that recorded bait interactions (excluding unidentifiable animals) were separately totalled. The relative proportion of total detections and bait interactions attributable to each species was then calculated.
To quantify whether target species found and interacted with baits more quickly than non-target species, we compared the time taken for the first bait interaction by each species at a site. First bait interaction events by species at sites were blocked into five 14-day periods: 0–14 days post deployment, 15–28 days, 29–42 days, 43–56 days, and 57–70 days. A Chi-square Test of Independence with Markov Chain Monte Carlo simulation was run to compare the likelihood of a species interacting with a bait within each of the five two-week blocks. Standardised residuals with the greatest magnitude difference from the mean (±2 in this study) were identified as strong contributors and reported accordingly.
The impact of environmental variables on the likelihood of species to interact with baits was investigated through two pathways: with an all-species model, including every species who had recorded at least one bait interaction, and with individual species models, for species with sufficient detections and bait interactions to model independently. For all models, environmental variables were scaled and centred (except PC scores). Correlation between all covariates was tested and variables with a correlation coefficient > 0.7 were inspected (Fig. S2). Fire frequency and mid to late successional PC were negatively correlated (rp = −0.79; Fig. S2). As time since fire is a key predictor of community composition in this ecosystem, and there is less understood about the effects of fire frequency on animal populations (Clarke et al. 2021), we excluded fire frequency from further analysis. All remaining covariates had correlation coefficients < 0.4 (Fig. S2).
There were no a priori expectations for the impact of each environmental variable on the likelihood of target and non-target species interacting with baits. For the all-species model, initial global generalised linear mixed effects models (GLMMs) included all uncorrelated environmental variables and their interaction with ‘target’ versus ‘non-target’ species (a two-level categorical variable). Site was included as a random effect to account for instances where multiple species were detected or interacted with a bait at the same site. For individual species models, initial global generalised linear models (GLMs) included all uncorrelated environmental variables. Due to sample size constraints, we limited the number of covariate combinations in model selection in the all-species model to six, and for individual species models to four, to reduce potential overparameterization.
The all-species model and individual species models were run with a binomial distribution to avoid issues with potential non-independence of detections or bait interactions (including individual sites with many bait interactions by single species). Global models were validated to ensure model assumptions were met with diagnostic plots. Residuals vs Leverage was graphed to identify the presence of any significant outliers (defined as having a cook’s D statistic >1). Global models with identified outliers were tested with and without the outliers present to determine their impact on overall result, and where overall trends did change, results with and without the outlier are reported. Resulting models were ranked using Akaike Information Criterion with correction for small sample sizes (AICC) and AICC weights (ωi). All models with ΔAICC > 2, or models ranked below the null model, were not considered further. Where there was model selection uncertainty, covariates that appeared in all models with a delta AIC < 2 are reported. The confidence level for result reporting was selected at 85% confidence intervals (CI) to reduce the likelihood of including uninformative parameters in result interpretations (Arnold 2010; Leroux 2019).
Results
Detections and bait interactions
A total of 716 independent observations of animals were recorded on cameras at bait sites (Supplementary material; Table S1). This included 558 mammal detections from 12 identified species, with the most common being Mitchell’s hopping-mice (Notomys mitchellii; 20% of observations), silky mice (16%), European rabbit (9%) and western grey kangaroo (Macropus fuliginosus; 8%) (Table S1). Birds were detected 127 times across 18 species, with chestnut quail-thrush (Cinclosoma castanotum; 4%), grey shrike-thrush (Colluricincla harmonica; 3%) and pied currawong (Strepera graculina; 2%) detected most often (Table S1). Reptiles from three species groups were detected 25 times, including painted dragons (Ctenophorus pictus; 1%) and central bearded dragons (Pogona vitticeps; <1%) (Table S1). Six detections were not able to be assigned to a species group (Table S1).
A total of 145 bait interactions were recorded for nine mammal species (Table S1). No birds or reptiles were recorded interacting with baits, they were only recorded passing through the camera area or perching/basking on the sand mound, without exhibiting any digging behaviour.
Both target species – foxes and dingoes – were detected at bait sites and interacted with baits. Foxes were observed at 17 sites (35%), and 32 bait interactions were recorded across 14 sites (Table 1). Dingoes were detected once with a single detection and bait interaction at only one site (2%) (Table 1). Dingoes are known to occur in the area surrounding the locations of these cameras and have previously been detected within 3.5 km of multiple bait sites (R.T. Mason, unpubl. data).
Species | Sites detected | Sites with bait interactions | Detected sites with bait interactions (%) | |
---|---|---|---|---|
Mitchell’s hopping-mouse (Notomys mitchellii) | 26 | 16 | 61.5 | |
Silky mouse (Pseudomys apodemoides) | 24 | 7 | 29.2 | |
Western grey kangaroo (Macropus fuliginosus) | 20 | 6 | 30.0 | |
Fox (Vulpes vulpes) | 17 | 14 | 82.4 | |
House mouse (Mus musculus) | 13 | 6 | 46.2 | |
European rabbit (Oryctolagus cuniculus) | 8 | 3 | 37.5 | |
European hare (Lepus europaeus) | 4 | 2 | 50.0 | |
Short-beaked echidna (Tachyglossus aculeatus) | 4 | 2 | 50.0 | |
Dingo (Canis dingo) | 1 | 1 | 100.0 |
Seven non-target mammal species were detected at bait sites and were recorded interacting with baits. Mitchell’s hopping-mice were recorded at the highest number of camera sites (54% of sites) and interacted with baits at the most sites (33%; Table 1). In total, Mitchell’s hopping-mice were detected 146 times, and recorded 43 bait interactions. Silky mice were also frequently detected (n = 116 detections) at a high number of sites (n = 50% of sites; Table 1). Silky mice recorded the highest number of bait interactions of any species (n = 54 bait interactions), but this was constrained to a small number of sites (n = 15%), with 46 of these bait interactions happening at a single site. Three mammal species that were detected on cameras were not recorded interacting with buried bait mounds: feral cats (Felis catus, n = 7 detections), common dunnarts (Sminthopsis murina, n = 4 detections) and swamp wallabies (Wallabia bicolor, n = 1 detection).
Digging and eating behaviours could not always be accurately differentiated from camera images, however four mammal species were recorded partially consuming baits on camera on at least one occasion: foxes, western grey kangaroos, silky mice, and Mitchell’s hopping-mice.
Target species accounted for more recorded bait interactions relative to their detection numbers, and when detected, were more likely to interact with baits compared to non-target species. Target species, primarily foxes, accounted for 7% of all species detections and 12% of all bait interactions, with only a single detection and bait interaction recorded by dingoes (Fig. 2). The seven non-target species that recorded bait interactions accounted for 93% of detection records and 88% of bait interactions. Two non-target species, silky mice and short-beaked echidnas (Tachyglossus aculeatus), frequently interacted with baits when detected at sites and consequently accounted for a greater proportion of bait interactions relative to their detection rate. The five remaining non-target species had fewer bait interactions relative to the number of times they were detected (Fig. 2).
Clustered bar chart depicting the percentage of detections (darker coloured bars) and bait interactions (lighter coloured bars) by (a) each target (orange) and non-target (blue) species and (b) all target and non-target species combined. A temporal filter of 20 min between successive images of a species was used to determine independence of detections (n = 474 detections) and bait interactions (n = 145 bait interactions). Bait interactions were defined as records of an identifiable species actively digging into a buried bait sand mound or otherwise interacting with the bait. Detections of species which did not record bait interactions (n = 170) and detections and bait interactions by unidentifiable mammals (n = 72 detections, 1 bait interaction), have not been included in this figure.
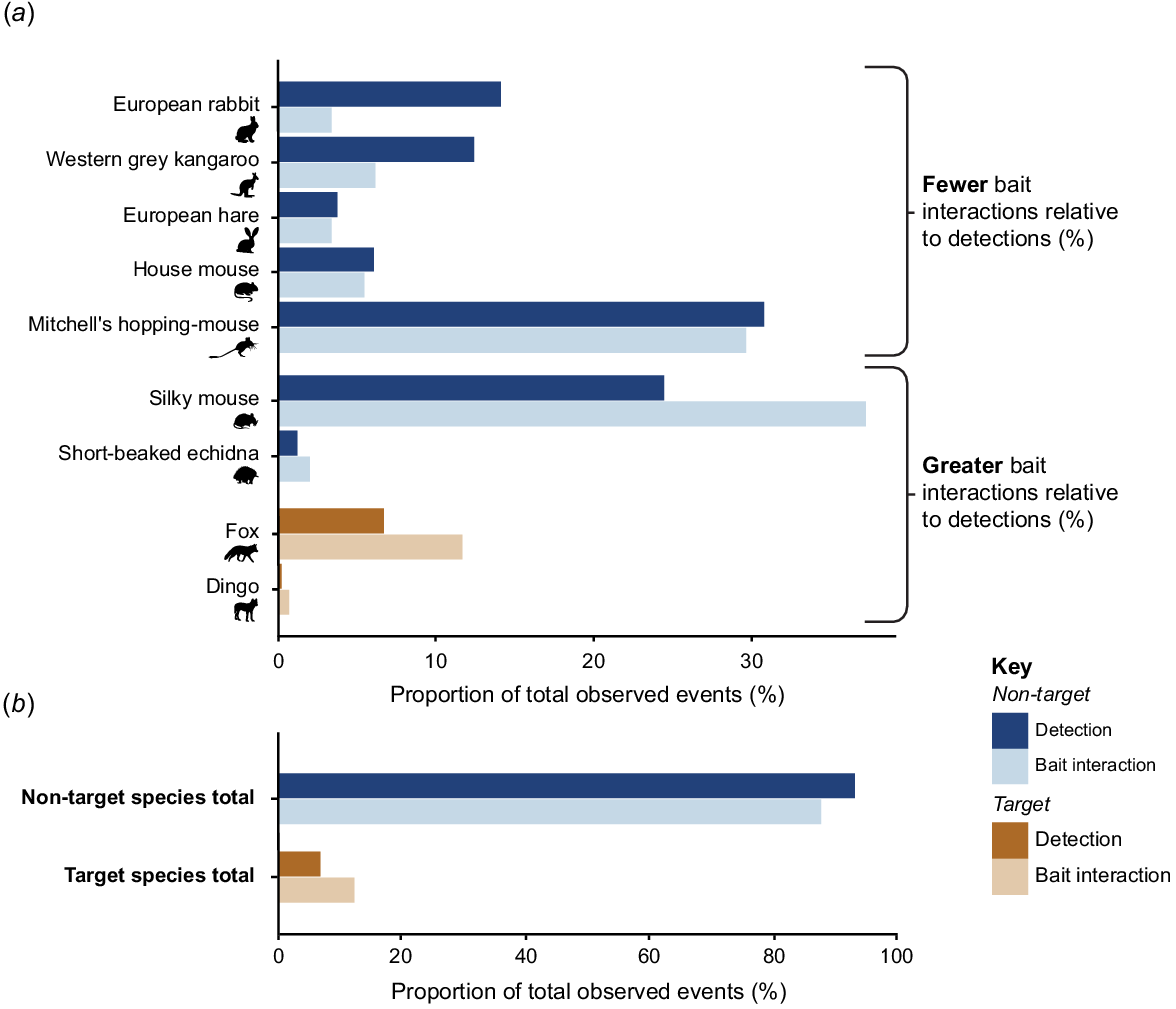
Timing of bait interactions by species
There was a significant difference in the time taken for each species to first interact with baits (χ2 = 50.52, P = 0.011). Foxes and dingoes took the greatest number of days to first interact with buried baits (median time to first bait interaction, foxes: 42 days, dingoes: 60 days). A single fox interacted with a buried bait within 14 h of bait deployment, but the next interaction was after 19 days (Fig. 3). There were significantly fewer bait interactions in the 0–14-day period than expected (χ2 standardised residual = −2.78; Fig. 3). Bait interactions by foxes were greater than expected 42–56 days after deployment (χ2 standardised residual = 2.69; Fig. 3). The single dingo that recorded a bait interaction did so after 60 days, which was greater than expected for the 56–72-day period (χ2 standardised residual = 2.50; Fig. 3).
Box plot and dot plot depicting the time taken (in days) to record the first bait interaction by non-target (blue) and target (orange) species at camera sites in the Big Desert-Wyperfeld complex. Thick lines indicate median time for each species, boxes show the interquartile range (IQR), and whiskers depict 1.5 × IQR. Grey arrows correspond to significant results of a Chi-square with Markov Chain Monte Carlo simulation (χ2 = 50.52, P = 0.018). Up arrows indicate standardised residuals with a strong positive magnitude (>2), denoting periods where a species interacted with baits more than expected, and down arrows indicate a strong negative magnitude (<−2), denoting periods where species interacted with baits less than expected.
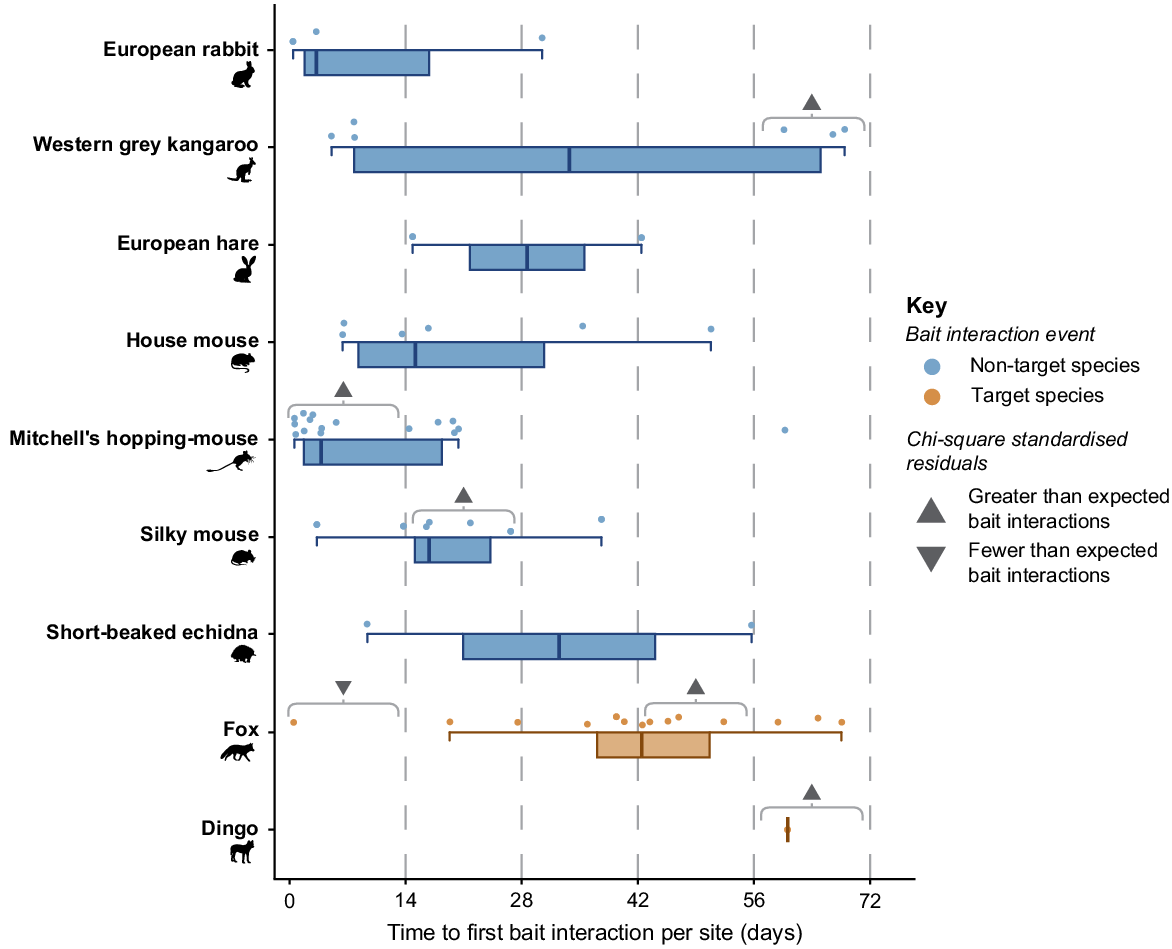
Three non-target species had greater than expected bait interactions at different periods in the bait deployment. Mitchell’s hopping-mice had a higher-than-expected number of bait interactions in the 0–14-day period (χ2 standardised residual = 2.32; Fig. 3), interacting with baits at ten sites in the first 6 days. Within the 14–28-day period, there were greater than expected bait interactions by silky mice (χ2 standardised residual = 2.31; Fig. 3). Comparatively, western grey kangaroos bait interactions were greater than expected in the last period, 56–72 days after bait deployment (χ2 standardised residual = 2.68; Fig. 3).
Impact of environmental variables on bait interactions
A total of 117 records from the nine mammal species that interacted with baits were included in this analysis, with a minimum of one record from each camera site. 18 records were from target species (overwhelmingly represented by foxes; n = 17) and 99 records were from non-target species.
There was some model selection uncertainty. The best-supported model included pyrodiversity, the mid to late successional PC, and species (target/non-target) status (AICCωi = 0.044). Additional variables were supported in models with ΔAICC < 2, but these variables all met the criteria for uninformative parameters (Leroux 2019; Table S2) and were, therefore, not interpreted further. Target species, primarily represented by foxes, were twice as likely (83%) to interact with baits (estimated marginal means (EMM) = 0.83, 85% CI = 0.66 to 0.93) when compared to non-target species (42%; EMM = 0.42, 85% CI = 0.35 to 0.50). Bait interactions were more likely to be recorded at sites with greater pyrodiversity across the surrounding landscape (β = 1.43, 85% CI = 0.24 to 2.63). There were also more bait interactions at sites with a higher proportion of mid-successional time since fire and a lower proportion of long-unburned areas (β = −0.24, 85% CI = −0.45 to −0.03).
Four mammal species were detected at more than 15 sites, allowing for further investigation into variables that may be driving responses in each species: foxes (n = 17 sites; Table 1), western grey kangaroos (n = 20 sites; Table 1), Mitchell’s hopping-mice (n = 26 sites; Table 1) and silky mice (n = 24 sites; Table 1).
The best-supported model for the bait interaction response of foxes (Table S3) included park boundary distance and vegetation cover (AICc ωi = 0.085). There were no additional supported variables in models ranked above the null model (ΔAICc = 0.27). Greater distance from park boundaries (β = 3.44, 85% CI = −0.62 to 7.49) and increased vegetation cover (β = 13.98, 85% CI = −1.93 to 28.891) generally increased the likelihood for foxes to record bait interactions, however confidence intervals for both coefficients overlapped zero (Fig. 4).
Forest plot depicting the influence of environmental variables on the likelihood of species interacting with buried meat baits at camera sites in the Big Desert-Wyperfeld complex. Estimates ±85% confidence intervals for variables included in the best-fit binary generalised linear model (GLM) outputs for target species (orange; foxes (Vulpes vulpes), n = 17 sites) and non-target species (blue; Mitchell’s hopping-mice (Notomys mitchellii), n = 26 sites; and silky mice (Pseudomys apodemoides), n = 24 sites) are shown. Confidence interval brackets that do not overlap zero (dashed grey line) are considered significant.
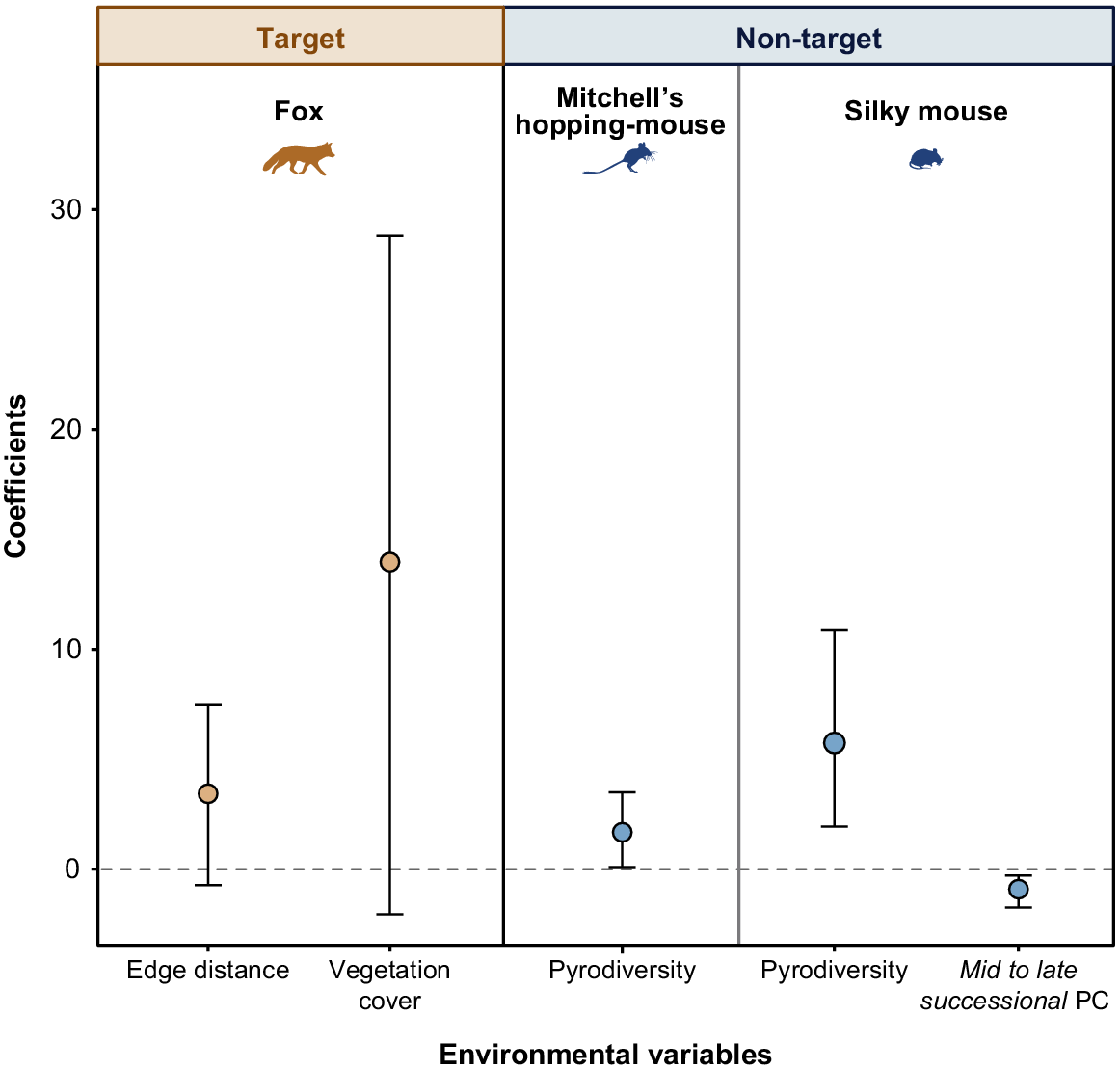
The bait interaction response of western grey kangaroos was influenced by an outlier (Cook’s D = 1.20) that altered the overall result trends when the global model was tested with and without its inclusion (Table S3). With the outlier included, the best-fit model for western grey kangaroos was the null model (AICCωi = 0.214; Table S3). With the outlier removed, the only model that ranked above the null model (ΔAICc = 1.07) included the park boundary distance and recently burned PC as covariates (AICCωi = 0.217, Table S3). When the outlier was not considered, the likelihood of western grey kangaroos to interact with baits increased with park boundary distance (β = 4.23, 85% CI = 1.42 to 8.27), and with a higher proportion of recently burned areas (β = −1.19, 85% CI = −2.31 to −0.35). As the sample size was constrained in the single species models, outliers could have strong impacts on overall model outcomes. Results excluding the outlier should be interpreted in a cautionary manner.
There was model selection uncertainty for Mitchell’s hopping-mice, as the top two models had equal weight (AICcωi = 0.132; Table S3). The top supported model included pyrodiversity, and the second-best model was the null model. As such, while there is an apparent trend towards Mitchell’s hopping-mice being more likely to interact with bait mounds at camera sites with more variation in the fire age of the surrounding vegetation (β = 1.68, 85% CI = 0.10 to 3.49; Fig. 4), this result should be interpreted with caution.
Silky mice models indicated strong support for pyrodiversity and the mid to late successional PC. All other variables (vegetation cover and park boundary distance) met the criteria for uninformative parameters and were not interpreted further (Leroux 2019; Table S3). Silky mice were more likely to interact with baits at camera sites with a higher diversity of fire age in the surrounding landscape (β = 5.74, 85% CI = 1.94 to 10.87; Fig. 4), and with a greater proportion of mid-successional time since fire relative to the proportion of long-unburned areas (β = −0.91, 85% CI = −1.73 to −0.28; Fig. 4).
Discussion
Understanding how target and non-target species may be impacted by lethal predator control is essential for achieving management goals and to limit any unintended negative consequences. In our study, using baiting methods recommended to reduce non-target bait interactions, we found that both target species, foxes and dingoes, interacted with buried non-toxic baits. However, they were typically not the first nor the most common species to interact with baits. Instead, native non-target species, particularly Mitchell’s hopping-mice and silky mice, were more likely to dig up or consume baits, and in a shorter timeframe than target predator species. The likelihood of non-target species interacting with buried baits was impacted by environmental variables including pyrodiversity, time since fire and vegetation cover. Our results reveal that lethal control programs have the potential to impact communities and hence ecosystems in unexpected ways.
Target and non-target bait interactions
Target species for lethal predator control – foxes and dingoes – did not detect and interact with buried baits more frequently than non-target species did. The proportion of bait interactions by target species recorded in this study is low in comparison to previously reported bait uptake rates for foxes and dingoes (i.e. bait uptake between <1% and 35% for similar deployment methods: Thompson and Fleming 1994; Saunders et al. 1999; Glen and Dickman 2003a; Dundas et al. 2014). Despite implementing ‘best practice’ methods for reducing non-target bait interactions, non-target species accounted for ~90% of bait interactions, nearly two-thirds of which were by Mitchell’s hopping-mice and silky mice. Bait uptake rates by native rodent species have rarely been quantified, although Moseby et al. (2011) detected spinifex hopping mouse (Notomys alexis) and greater stick-nest rats (Leporillus conditor) partially consuming surface-laid baits at 16% of bait stations.
Contrary to our predictions, all non-target species generally interacted with baits earlier in the deployment period than either foxes or dingoes. The single dingo and the majority of fox bait interactions occurred late in the bait deployment period. Due to rapid degradation of 1080 in wet conditions (Saunders et al. 2000; Allsop et al. 2017), the greater than average rainfall occurring throughout this experiment likely meant that if toxic baits had been used, only three target animals may have ingested a lethal or sublethal 1080 dosage. Despite the lower numbers and slower time for bait interactions by target species, in cases where they were detected at camera sites, foxes and dingoes were twice as likely to interact with baits than non-target species were. This suggests that target species may be more intentional in digging up and consuming buried baits, whereas non-target animals, including herbivorous or granivorous species, are potentially opportunistically digging up baits for the abundant and easily accessible source of protein.
Impact of environmental variables on bait interactions
When evaluating all nine species’ response to baiting, bait interactions were more likely to occur in areas with increased pyrodiversity and a greater proportion of mid-successional vegetation, compared to long-unburned areas. Individually, greater pyrodiversity also increased the likelihood of bait interactions by Mitchell’s hopping-mice, and both pyrodiversity and mid-successional vegetation were associated with increased bait interactions by silky mice. Many vegetation attributes that influence habitat suitability in mallee environments are strongly influenced by time since fire and pyrodiversity (Haslem et al. 2011; Kelly et al. 2012). Understory and midstory complexity peak in mid-successional time since fire areas (Haslem et al. 2011), and these densely vegetated microhabitats can provide important shelter resources to aid predator avoidance (Di Stefano et al. 2011). Previous research has shown that more open microhabitats in recently burned areas reduce the foraging time of Mitchell’s hopping-mice and other rodent species, compared to foraging in sheltered shrub-covered microhabitats (Doherty et al. 2015). Digging up and consuming baits in a cleared, bare ground area results in lengthy periods of exposure for small prey species. Access to nearby unburned mid-successional vegetation in pyrodiverse landscapes can provide rapid shelter from approaching predators (Doherty et al. 2022), explaining the influence of these factors on bait interaction rates by native mice in this study.
Foxes interacted with baits at >80% of sites where they were detected. Foxes are an opportunistic, generalist species with a broad diet (Fleming et al. 2021), meaning they’re predisposed to interacting with baits. In this study, contrary to predictions, greater distance from the park boundary and increased vegetation cover were associated with an increased likelihood of bait interactions by foxes. Lethal baiting in the BDW complex is concentrated on the northern and southern boundaries, potentially reducing the likelihood of foxes consuming baits at park edges due to selection pressure (Allsop et al. 2017) and/or a localised reduction in fox abundance after previous baiting (Carter and Luck 2013). Additionally, human-modified or fragmented landscapes including farmland and open habitat edges can be associated with greater prey density (Šálek et al. 2010), prey vulnerability (Panzacchi et al. 2009; Doherty et al. 2022) and anthropogenic food resources (Hradsky et al. 2017b). Foxes with home ranges in the continuous, highly vegetated interior of the park complex may be more likely to interact with baits due to differences in resource availability associated with these complex habitats. Future research should focus on clarifying potential differences in the response of foxes to baiting in intact and fragmented landscapes, and in relation to prey availability.
Bait interactions by western grey kangaroos tended to increase with distance from the edge of the park complex and in recently burned areas, but these trends were only apparent in models where an outlier was removed. When considered in association with the small sample size, these trends should be interpreted cautiously. Western grey kangaroos preferentially feed on grasses (Arnold et al. 1989), including pasture and crops around the BDW complex (Short and Grigg 1982), and the sclerophyllous shrub understory of denser mallee habitats may not provide nutrient-dense food resources (Short and Grigg 1982). As such, with increasing distance from the edge of the BDW complex, western grey kangaroos may be attracted to recently burned areas due to highly palatable reshooting vegetation and may be consuming meat baits as a source of protein. Previous published occurrences of western grey kangaroos scavenging carrion or consuming meat baits have all been in sandy, nutrient poor environments (Dundas et al. 2014; Hughes and Bérengier 2018), comparable to the conditions in this study.
Factors affecting efficacy of baiting programs
The low numbers and slow time frame of target species interacting with baits could be influenced by climatic conditions during this period. This study was undertaken during a period of above average rainfall (Australian Bureau of Meteorology). Increases in native small mammal populations have been linked to increased rainfall in the previous 6–12 months (Letnic and Dickman 2006; Kelly et al. 2012; Christensen et al. 2013; Bennison et al. 2018). Similarly, rabbit and kangaroo abundance can increase following rainfall events in arid and semi-arid environments (Wood 1980; Caughley et al. 1984; Cairns and Grigg 1993). These species collectively make up a high proportion of the diet of both foxes and dingoes in this park complex (Ritchie 2013; Davis et al. 2015), so increased prey abundance could be reducing the scavenging of buried baits by these species. Dietary switching with changing availability of prey species is well-demonstrated in dingoes and foxes (Doherty et al. 2019; Fleming et al. 2021) and scavenging rates can vary in response to fluctuating live prey abundance (Allen 2010; Forsyth et al. 2014). However, the direct influence of prey availability on bait uptake has thus far only been demonstrated in cats, with increasing rabbit (Algar et al. 2007) and small mammal (Christensen et al. 2013) abundance shown to substantially reduce bait uptake in semi-arid areas, and conversely, nutritional stress increasing records of bait scavenging by cats in arid areas (Paltridge et al. 1997).
This inverse relationship between prey abundance and bait scavenging rates could also explain the lack of bait interactions recorded by cats, varanids, and corvids in this study, as varanids (Moseby et al. 2011; Kreplins et al. 2018) and corvids (Dexter and Meek 1998; Kreplins et al. 2018; Hohnen et al. 2020) are frequently responsible for non-target bait uptake in monitored baiting trials, but did not interact with baits in our study.
Conservation and management implications
Lethal control with 1080 is a widespread method of predator management in Australia and New Zealand, and can be an effective conservation tool (e.g. Morris et al. 2003; Dexter and Murray 2009; Kinnear et al. 2010; Kirkwood et al. 2014; Robley et al. 2014; Nichols et al. 2021). However, the impacts of 1080 baiting on non-target species, including when ‘best practice’ baiting techniques are used, are often unquantified (Glen et al. 2007). Here, we have reported that bait burial to 10 cm, reduced bait palatability, and targeted timing of bait deployment did not subsequently result in a lack of non-target bait interactions. The low level of bait interactions recorded by foxes and dingoes in this study, and high levels recorded by native non-target animals, imply that poison baiting may be less effective at controlling predators in this region than expected. Our study has also identified a relationship between increased non-target bait interactions and fire history variables, which is often overlooked in the design of lethal predator control programs. Baiting programs that do not consider the influence of fire history on non-target bait interactions may deploy baits in areas where non-target species are more likely to dig up and consume them, and target species are less likely to. This will not only reduce the efficacy for target species, but may also harm non-target populations.
There are two possible outcomes of non-target species digging up baits, both of which have implications for conservation and management outcomes of lethal control programs. The first is that a non-target animal consumes the bait, and the alternative is that the bait becomes available in the environment after a non-target animal digs it up but does not consume it.
The consequence of consumption of some or all of a lethal bait by a non-target animal will vary based on the sensitivity and susceptibility of that species to 1080 ingestion. Some native mammals are reported to be highly tolerant to 1080, especially if their distribution overlaps vegetation with high levels of naturally occurring fluoroacetate (reviewed in Twigg and King 1991). Western grey kangaroos, for example, have demonstrated a high tolerance (LD50 20–60 mg kg−1; Mcilroy 1982a) for 1080 across their entire distribution (Oliver et al. 1979), and are unlikely to be affected even by consumption of multiple toxic baits. However, some species have demonstrated reduced 1080 tolerance in populations with increasing geographic separation from fluoroacetate-rich plants (Calver et al. 1989; Twigg et al. 2003). Mitchell’s hopping-mouse populations range from an LD50 of 51 mg kg−1 (Twigg et al. 2003), to 14 mg kg−1 (Calver et al. 1989) with increasing distance from naturally occurring fluoroacetate. Mitchell’s hopping-mice in the BDW complex, at the easternmost extent of their distribution, may have a much lower tolerance than previously tested populations. The sensitivity of silky mice to 1080 poison has never been experimentally tested, but adult silky mice in the BDW complex have been reported dying after exposure to 1080-poisoned oat baits (Cockburn 1981; Mcilroy 1982b). Populations of the most closely related species, the ash-grey mouse (Pseudomys albocinereus) that do not overlap with fluoroacetate-rich plants, are highly sensitive to 1080, with an LD50 of 1.57 mg kg−1 (Twigg et al. 2003). Silky mice, with no evolutionary exposure to fluoroacetate, would likely be equally or more sensitive to 1080.
To consume a potentially lethal 1080 dose, adult Mitchell’s hopping-mice, weighing ~52 grams (Menkhorst 2011) and assuming an LD50 of 14 mg kg−1, would need to consume ~25% of a fox bait or 12% of a dingo bait (containing 3 mg and 6 mg of 1080, respectively). Mitchell’s hopping-mice have been recorded consuming up to eight grams of food daily (Calver et al. 1991) and could therefore consume a lethal or sublethal 1080 dose of dingo or fox bait in a single day. Similar estimates cannot be directly made for silky mice, but if they are as sensitive as the closely related ash-grey mice, a silky mouse weighing 22 grams would need to consume just 0.3 grams of meat from a 60-gram dingo bait to receive a potentially lethal 1080 dose. While some rodent species have been shown to reduce their consumption of 1080 injected food items (Calver et al. 1989; O’Connor et al. 2005), neither of these native species has previously demonstrated 1080 avoidance in lab or field settings (Cockburn 1981; Mcilroy 1982b; Calver et al. 1989). As we have recorded both species readily locating, digging up, and gnawing buried non-toxic baits deployed in the field, we can assume that both Mitchell’s hopping-mice and silky mice are at risk of sublethal or lethal impacts of 1080 baiting programs conducted in this environment.
Even when not directly consuming baits, non-target species digging up baits will increase their availability to other species. Unburied baits are taken more often by corvids (Allen et al. 1989; Thomson and Kok 2002; Moseby et al. 2011), raptors (Moseby et al. 2011), varanids (Allen et al. 1989; Thomson and Kok 2002), and dasyurids (Mooney et al. 2005) than buried baits. Particularly concerning is the risk of increased bait availability to vulnerable dasyurids present in this study area, the fat-tailed dunnart (Sminthopsis crassicaudata) and the common dunnart (Sminthopsis murina). While dunnart species including fat-tailed dunnarts are generally considered highly sensitive to 1080 (Mcilroy 1981), common dunnart susceptibility has not been experimentally tested. Bait excavation by other non-target animals has the potential to impact these vulnerable species.
Conclusions
In the BDW complex, we have shown that ongoing landscape-scale baiting using ‘best practice’ baiting methods has the potential to negatively impact non-target native animals. More research into both the uptake of baits by non-target species, particularly native rodents, and the relationship between environmental variables and baiting in both target and non-target species, is needed across a variety of Australian landscapes to quantify the scope and severity of this problem. Simultaneously, efforts to monitor and reduce any non-target uptake of baits need to be considered and implemented where appropriate. Future research should also focus on quantifying the susceptibility of populations of non-target species across a range of geographic and ecological contexts. If the use of predator baiting as a conservation tool is to continue, it needs to be targeted, efficient and beneficial to the ecosystem. In the BDW complex, deploying baits as a direct response to conditions that will result in greater target uptake and reduced non-target interactions, such as immediately post-fire and in periods of low prey availability (Allen 2010; Forsyth et al. 2014), will both increase the efficiency of predator control and reduce the potential harm to native non-target animals. Increasing bait uptake must also be considered carefully in the context of the resident dingo population, estimated to number fewer than 60 individuals (E.G. Ritchie, unpubl. data). Although our study recorded only one dingo at a bait station, any bait taken by dingoes in this area is likely to have a significantly detrimental impact on this small population.
Data availability
The data that support this study will be shared upon reasonable request to the corresponding author.
Declaration of funding
This study was funded by the State of Victoria through the Department of Energy, Environment and Climate Action (previously the Department of Environment, Land, Water and Planning) Biodiversity Knowledge Framework. R. T Mason also received funding from a Deakin University Research Training Program Scholarship.
Acknowledgements
We pay our respects to the Traditional Owners of the land on which research was conducted: the Wotjobaluk, Jaadwa, Jadawadjali, Wergaia and Jupagulk Nations of the Southern Mallee. We particularly thank Matt White of the Arthur Rylah Institute for Environmental Research for providing advice and assistance with mapping vegetation cover across the study region. We thank all the Parks Victoria and the Department of Energy, Environment and Climate Action staff who assisted with the fieldwork and method development for this project, particularly Scott McLean, Hamish Hollamby and Justin Oakley. We also thank Ellisha Martion, Cort Wynen and Kim Bannister for their assistance with fieldwork.
References
Algar D, Angus GJ, Williams MR, Mellican AE (2007) Influence of bait type, weather and prey abundance on bait uptake by feral cats (Felis catus) on Peron Peninsula, Western Australia. Conservation Science Western Australia 6, 109-149.
| Google Scholar |
Allen BL (2010) Skin and bone: observations of dingo scavenging during a chronic food shortage. Australian Mammalogy 32, 207-208.
| Crossref | Google Scholar |
Allen LR, Fleming PJS, Thompson JA, Strong K (1989) Effect of presentation on the attractiveness and palatability to wild dogs and other wildlife of 2 unpoisoned wild-dog bait types. Australian Wildlife Research 16, 593-598.
| Crossref | Google Scholar |
Allsop SE, Dundas SJ, Adams PJ, Kreplins TL, Bateman PW, Fleming PA (2017) Reduced efficacy of baiting programs for invasive species: Some mechanisms and management implications. Pacific Conservation Biology 23, 240-257.
| Crossref | Google Scholar |
Arnold TW (2010) Uninformative parameters and model selection using Akaike’s Information Criterion. The Journal of Wildlife Management 74, 1175-1178.
| Crossref | Google Scholar |
Arnold GW, Steven DE, Weeldenburg JR (1989) The use of surrounding farmland by western grey kangaroos living in a remnant of wandoo woodland and their impact on crop production. Australian Wildlife Research 16, 85-93.
| Crossref | Google Scholar |
Belcher CA (1998) Susceptibility of the tiger quoll, Dasyurus maculatus, and the eastern quoll, D. viverrinus, to 1080-poisoned baits in control programmes for vertebrate pests in eastern Australia. Wildlife Research 25, 33-40.
| Crossref | Google Scholar |
Bennison K, Godfree R, Dickman CR (2018) Synchronous boom–bust cycles in central Australian rodents and marsupials in response to rainfall and fire. Journal of Mammalogy 99, 1137-1148.
| Crossref | Google Scholar |
Bliege Bird R, Bird DW, Fernandez LE, Taylor N, Taylor W, Nimmo D (2018) Aboriginal burning promotes fine-scale pyrodiversity and native predators in Australia’s Western Desert. Biological Conservation 219, 110-118.
| Crossref | Google Scholar |
Bomford M, Hart Q (2002) Non-indigenous vertebrates in Australia. In ‘Biological invasions – economic and environmental costs of alien plant, animal and microbe species’. (Ed. D Pimentel) pp. 25–44. (CRC Press: New York, NY, USA) 10.1201/9781420041668.ch3
Cairns SC, Grigg GC (1993) Population dynamics of red kangaroos (Macropus rufus) in relation to rainfall in the South Australian pastoral zone. The Journal of Applied Ecology 30, 444-458.
| Crossref | Google Scholar |
Calver MC, King DR, Bradley JS, Gardner JL, Martin G (1989) An assessment of the potential target specificity of 1080 predator baiting in Western-Australia. Australian Wildlife Research 16, 625-638.
| Crossref | Google Scholar |
Calver MC, King DR, Gardner JL, Martin GR (1991) Total food consumption of some native Australian small mammals in the laboratory. Australian Mammalogy 14, 139-142.
| Crossref | Google Scholar |
Campbell G, Coffey A, Miller H, Read JL, Brook A, Fleming PJS, Bird P, Eldridge S, Allen BL (2019) Dingo baiting did not reduce fetal/calf loss in beef cattle in northern South Australia. Animal Production Science 59, 319-330.
| Crossref | Google Scholar |
Capizzi D, Bertolino S, Mortelliti A (2014) Rating the rat: global patterns and research priorities in impacts and management of rodent pests. Mammal Review 44, 148-162.
| Crossref | Google Scholar |
Carter A, Luck GW (2013) Fox baiting in agricultural landscapes: preliminary findings on the importance of bait-site selection. Wildlife Research 40, 184-195.
| Crossref | Google Scholar |
Caughley J, Bayliss P, Giles J (1984) Trends in kangaroo numbers in western New South Wales and their relation to rainfall. Australian Wildlife Research 11, 415-422.
| Crossref | Google Scholar |
Christensen PES, Ward BG, Sims C (2013) Predicting bait uptake by feral cats, Felis catus, in semi-arid environments. Ecological Management & Restoration 14, 47-53.
| Crossref | Google Scholar |
Claridge AW, Murray AJ, Dawson J, Poore R, Mifsud G, Saxon MJ (2006) The propensity of spotted-tailed quolls (Dasyurus maculatus) to encounter and consume non-toxic meat baits in a simulated canid-control program. Wildlife Research 33, 85-91.
| Crossref | Google Scholar |
Claridge AW, Ballard G, Körtner G, Fleming PJS, Forge T, Hine A (2021) Lethal control of eutherian predators via aerial baiting does not negatively affect female spotted-tailed quolls (Dasyurus maculatus maculatus) and their pouch young. Wildlife Research 48, 273-288.
| Crossref | Google Scholar |
Clarke MF, Kelly LT, Avitabile SC, Benshemesh J, Callister KE, Driscoll DA, Ewin P, Giljohann K, Haslem A, Kenny SA, Leonard S, Ritchie EG, Nimmo DG, Schedvin N, Schneider K, Watson SJ, Westbrooke M, White M, Wouters MA, Bennett AF (2021) Fire and its interactions with other drivers shape a distinctive, semi-arid ‘mallee’ ecosystem. Frontiers in Ecology and Evolution 9, 647557.
| Crossref | Google Scholar |
Cockburn A (1981) Diet and habitat preference of the silky desert mouse, Pseudomys apodemoides (rodentia). Australian Wildlife Research 8, 475-497.
| Crossref | Google Scholar |
Cowan P, Crowell M (2017) Visual and taste cues for minimising native bird interactions with toxic 1080 baits – a review of current practices. New Zealand Journal of Ecology 41, 178-185.
| Crossref | Google Scholar |
Davis NE, Forsyth DM, Triggs B, Pascoe C, Benshemesh J, Robley A, Lawrence J, Ritchie EG, Nimmo DG, Lumsden LF (2015) Interspecific and geographic variation in the diets of sympatric carnivores: dingoes/wild dogs and red foxes in south-eastern Australia. PLoS ONE 10, e0120975.
| Crossref | Google Scholar | PubMed |
Dawson TJ, Hulbert AJ (1970) Standard metabolism, body temperature, and surface areas of Australian marsupials. American Journal of Physiology 218, 1233-1238.
| Crossref | Google Scholar | PubMed |
Dexter N, Meek P (1998) An analysis of bait-take and non-target impacts during a fox-control exercise. Wildlife Research 25, 147-155.
| Crossref | Google Scholar |
Dexter N, Murray A (2009) The impact of fox control on the relative abundance of forest mammals in East Gippsland, Victoria. Wildlife Research 36, 252-261.
| Crossref | Google Scholar |
Di Stefano J, Owen L, Morris R, Duff T, York A (2011) Fire, landscape change and models of small mammal habitat suitability at multiple spatial scales. Austral Ecology 36, 638-649.
| Crossref | Google Scholar |
Doherty TS, Davis RA, van Etten EJB (2015) A game of cat-and-mouse: microhabitat influences rodent foraging in recently burnt but not long unburnt shrublands. Journal of Mammalogy 96, 324-331.
| Crossref | Google Scholar |
Doherty TS, Glen AS, Nimmo DG, Ritchie EG, Dickman CR (2016) Invasive predators and global biodiversity loss. Proceedings of the National Academy of Sciences 113, 11261-11265.
| Crossref | Google Scholar |
Doherty TS, Davis NE, Dickman CR, Forsyth DM, Letnic M, Nimmo DG, Palmer R, Ritchie EG, Benshemesh J, Edwards G, Lawrence J, Lumsden L, Pascoe C, Sharp A, Stokeld D, Myers C, Story G, Story P, Triggs B, Venosta M, Wysong M, Newsome TM (2019) Continental patterns in the diet of a top predator: Australia’s dingo. Mammal Review 49, 31-44.
| Crossref | Google Scholar |
Doherty TS, Geary WL, Jolly CJ, Macdonald KJ, Miritis V, Watchorn DJ, Cherry MJ, Conner LM, González TM, Legge SM, Ritchie EG, Stawski C, Dickman CR (2022) Fire as a driver and mediator of predator–prey interactions. Biological Reviews 97, 1539-1558.
| Crossref | Google Scholar | PubMed |
Doherty TS, Watchorn DJ, Miritis V, Pestell AJL, Geary WL (2023) Cats, foxes and fire: quantitative review reveals that invasive predator activity is most likely to increase shortly after fire. Fire Ecology 19, 22.
| Crossref | Google Scholar |
Dundas SJ, Adams PJ, Fleming PA (2014) First in, first served: uptake of 1080 poison fox baits in south-west Western Australia. Wildlife Research 41, 117-126.
| Crossref | Google Scholar |
Fairbridge D, Fisher P, Busana F, Pontin K, Edwards A (2000) Observations of the behaviour of free living bush Rat, Rattus Fuscipes and Southern Brown Bandicoot, Isoodon Obesulus at Buried Bait Stations. Australian Mammalogy 22, 125-127.
| Crossref | Google Scholar |
Fairbridge D, Anderson R, Wilkes T, Pell G (2003) Bait uptake by free living brush-tailed phascogales Phascogale tapoatafa and other non-target mammals during simulated buried fox baiting. Australian Mammalogy 25, 31-45.
| Crossref | Google Scholar |
Fleming PJS (1996) Ground-placed baits for the control of wild dogs: evaluation of a replacement-baiting strategy in North-Eastern New South Wales. Wildlife Research 23, 729-740.
| Crossref | Google Scholar |
Fleming PA, Crawford HM, Stobo-Wilson AM, Dawson SJ, Dickman CR, Dundas SJ, Gentle MN, Newsome TM, O’Connor J, Palmer R, Riley J, Ritchie EG, Speed J, Saunders G, Stuart JMD, Thompson E, Turpin JM, Woinarski JCZ (2021) Diet of the introduced red fox Vulpes vulpes in Australia: analysis of temporal and spatial patterns. Mammal Review 51, 508-527.
| Crossref | Google Scholar |
Forsyth DM, Woodford L, Moloney PD, Hampton JO, Woolnough AP, Tucker M (2014) How does a carnivore guild utilise a substantial but unpredictable anthropogenic food source? Scavenging on hunter-shot ungulate carcasses by wild dogs/dingoes, red foxes and feral cats in south-eastern Australia revealed by camera traps. PLoS ONE 9, e97937.
| Crossref | Google Scholar |
Geary WL, Ritchie EG, Lawton JA, Healey TR, Nimmo DG (2018) Incorporating disturbance into trophic ecology: fire history shapes mesopredator suppression by an apex predator. Journal of Applied Ecology 55, 1594-1603.
| Crossref | Google Scholar |
Glen AS, Dickman CR (2003a) Effects of bait-station design on the uptake of baits by non-target animals during control programmes for foxes and wild dogs. Wildlife Research 30, 147-149.
| Crossref | Google Scholar |
Glen AS, Dickman CR (2003b) Monitoring bait removal in vertebrate pest control: a comparison using track identification and remote photography. Wildlife Research 30, 29-33.
| Crossref | Google Scholar |
Glen AS, Gentle MN, Dickman CR (2007) Non-target impacts of poison baiting for predator control in Australia. Mammal Review 37, 191-205.
| Crossref | Google Scholar |
Griffiths AD, Brook BW (2014) Effect of fire on small mammals: a systematic review. International Journal of Wildland Fire 23, 1034-1043.
| Crossref | Google Scholar |
Haslem A, Kelly LT, Nimmo DG, Watson SJ, Kenny SA, Taylor RS, Avitabile SC, Callister KE, Spence-Bailey LM, Clarke MF, Bennett AF (2011) Habitat or fuel? Implications of long-term, post-fire dynamics for the development of key resources for fauna and fire. Journal of Applied Ecology 48, 247-256.
| Crossref | Google Scholar |
Hendry H, Mann C (2018) Camelot—intuitive software for camera-trap data management. Oryx 52, 15.
| Crossref | Google Scholar |
Hohnen R, Murphy BP, Legge SM, Dickman CR, Woinarski JCZ (2020) Uptake of ‘Eradicat’ feral cat baits by non-target species on Kangaroo Island. Wildlife Research 47, 547-556.
| Crossref | Google Scholar |
Hradsky BA (2020) Conserving Australia’s threatened native mammals in predator-invaded, fire-prone landscapes. Wildlife Research 47, 1-15.
| Crossref | Google Scholar |
Hradsky BA, Mildwaters C, Ritchie EG, Christie F, Di Stefano J (2017a) Responses of invasive predators and native prey to a prescribed forest fire. Journal of Mammalogy 98, 835-847.
| Crossref | Google Scholar |
Hradsky BA, Robley A, Alexander R, Ritchie EG, York A, Di Stefano J (2017b) Human-modified habitats facilitate forest-dwelling populations of an invasive predator, Vulpes vulpes. Scientific Reports 7, 12291.
| Crossref | Google Scholar | PubMed |
Hughes M, Bérengier V (2018) Are there conservation implications for kangaroos feeding on sea birds? Pacific Conservation Biology 24, 98-99.
| Crossref | Google Scholar |
Iannarilli F, Erb J, Arnold TW, Fieberg JR (2021) Evaluating species-specific responses to camera-trap survey designs. Wildlife Biology 2021(1),.
| Crossref | Google Scholar |
Kelly LT, Nimmo DG, Spence-Bailey LM, Taylor RS, Watson SJ, Clarke MF, Bennett AF (2012) Managing fire mosaics for small mammal conservation: a landscape perspective. Journal of Applied Ecology 49, 412-421.
| Crossref | Google Scholar |
Kinnear JE, Krebs CJ, Pentland C, Orell P, Holme C, Karvinen R (2010) Predator-baiting experiments for the conservation of rock-wallabies in Western Australia: a 25-year review with recent advances. Wildlife Research 37, 57-67.
| Crossref | Google Scholar |
Kirkwood R, Sutherland DR, Murphy S, Dann P (2014) Lessons from long-term predator control: a case study with the red fox. Wildlife Research 41, 222-232.
| Crossref | Google Scholar |
Körtner G, Watson P (2005) The immediate impact of 1080 aerial baiting to control wild dogs on a spotted-tailed quoll population. Wildlife Research 32, 673-680.
| Crossref | Google Scholar |
Körtner G, Gresser S, Harden B (2003) Does fox baiting threaten the spotted-tailed quoll, Dasyurus maculatus? Wildlife Research 30, 111-118.
| Crossref | Google Scholar |
Kreplins TL, Kennedy MS, Adams PJ, Bateman PW, Dundas SD, Fleming PA (2018) Fate of dried meat baits aimed at wild dog (Canis familiaris) control. Wildlife Research 45, 528-538.
| Crossref | Google Scholar |
Leroux SJ (2019) On the prevalence of uninformative parameters in statistical models applying model selection in applied ecology. PLoS ONE 14, e0206711.
| Crossref | Google Scholar | PubMed |
Letnic M, Dickman CR (2006) Boom means bust: interactions between the El Niño/Southern Oscillation (ENSO), rainfall and the processes threatening mammal species in arid Australia. Biodiversity and Conservation 15, 3847-3880.
| Crossref | Google Scholar |
Littin K, Fisher P, Beausoleil NJ, Sharp T (2014) Welfare aspects of vertebrate pest control and culling: ranking control techniques for humaneness. Revue Scientifique et Technique de l’OIE 33, 281-289.
| Crossref | Google Scholar | PubMed |
Marlow NJ, Thomas ND, Williams AAE, Macmahon B, Lawson J, Hitchen Y, Angus J, Berry O (2015) Cats (Felis catus) are more abundant and are the dominant predator of woylies (Bettongia penicillata) after sustained fox (Vulpes vulpes) control. Australian Journal of Zoology 63, 18-27.
| Crossref | Google Scholar |
May SA, Norton TW (1996) Influence of fragmentation and disturbance on the potential impact of feral predators on native fauna in Australian forest ecosystems. Wildlife Research 23, 387-400.
| Crossref | Google Scholar |
McGregor HW, Legge S, Jones ME, Johnson CN (2014) Landscape management of fire and grazing regimes alters the fine-scale habitat utilisation by feral cats. PLoS ONE 9, e109097.
| Crossref | Google Scholar | PubMed |
Mcilroy JC (1981) The sensitivity of Australian animals to 1080 poison II. Marsupial and eutherian carnivores. Australian Wildlife Research 8, 385-399.
| Crossref | Google Scholar |
Mcilroy JC (1982a) The sensitivity of Australian animals to 1080 poison III. Marsupial and eutherian herbivores. Australian Wildlife Research 9, 487-503.
| Crossref | Google Scholar |
Mcilroy JC (1982b) The sensitivity of Australian animals to 1080 poison IV. Native and introduced rodents. Australian Wildlife Research 9, 505-517.
| Crossref | Google Scholar |
Mead RJ, Oliver AJ, King DR, Hubach PH (1985) The co-evolutionary role of fluoroacetate in plant-animal interactions in Australia. Oikos 44, 55-60.
| Crossref | Google Scholar |
Meek PD, Ballard G, Claridge A, Kays R, Moseby K, O’Brien T, O’Connell A, Sanderson J, Swann DE, Tobler M, Townsend S (2014) Recommended guiding principles for reporting on camera trapping research. Biodiversity and Conservation 23, 2321-2343.
| Crossref | Google Scholar |
Mooney N, Emms C, Bloomfield T (2005) Minimising the effects of 1080 fox baiting on non-target species and vice versa while maximising the risks to foxes in Tasmania. In ‘Proceedings of the 13th Australasian Vertebrate Pest Conference’. (Eds J Parkes, M Statham and G Edwards) pp. 148–149. (Manaaki Whenua, Landcare Research: Wellington, NZ)
Morris K, Johnson B, Orell P, Gaikhorst GS, Wayne AF, Moro D (2003) Recovery of the threatened chuditch (Dasyurus geoffroii): a case study. In ‘Predators with pouches: The biology of carnivorous marsupials’. (Eds M Jones, CR Dickman, M Archer) pp. 435–451. (CSIRO Publishing: Melbourne, Vic, Australia)
Moseby KE, Read JL, Galbraith B, Munro NT, Newport J, Hill BM (2011) The use of poison baits to control feral cats and red foxes in arid South Australia II. Bait type, placement, lures and non-target uptake. Wildlife Research 38, 350-358.
| Crossref | Google Scholar |
Murray AJ, Poore RN (2004) Potential impact of aerial baiting for wild dogs on a population of spotted-tailed quolls (Dasyurus maculatus). Wildlife Research 31, 639-644.
| Crossref | Google Scholar |
Newsome TM, Crowther MS, Dickman CR (2014) Rapid recolonisation by the European red fox: how effective are uncoordinated and isolated control programs? European Journal of Wildlife Research 60, 749-757.
| Crossref | Google Scholar |
Nichols M, Nathan H, Mulgan N (2021) Dual aerial 1080 baiting operation removes predators at a large spatial scale. New Zealand Journal of Ecology 45, 3428.
| Crossref | Google Scholar |
Nimmo DG, Avitabile S, Banks SC, Bliege Bird R, Callister K, Clarke MF, Dickman CR, Doherty TS, Driscoll DA, Greenville AC, Haslem A, Kelly LT, Kenny SA, Lahoz-Monfort JJ, Lee C, Leonard S, Moore H, Newsome TM, Parr CL, Ritchie EG, Schneider K, Turner JM, Watson S, Westbrooke M, Wouters M, White M, Bennett AF (2019) Animal movements in fire-prone landscapes. Biological Reviews 94, 981-998.
| Crossref | Google Scholar |
Oliver AJ, King DR, Mead RJ (1979) Fluoroacetate tolerance, a genetic marker in some Australian mammals. Australian Journal of Zoology 27, 363-372.
| Crossref | Google Scholar |
Paltridge R, Gibson D, Edwards G (1997) Diet of the Feral Cat (Felis catus) in Central Australia. Wildlife Research 24, 67-76.
| Crossref | Google Scholar |
Panzacchi M, Linnell JDC, Odden M, Odden J, Andersen R (2009) Habitat and roe deer fawn vulnerability to red fox predation. Journal of Animal Ecology 78, 1124-1133.
| Crossref | Google Scholar | PubMed |
Pausas JG, Bradstock RA (2007) Fire persistence traits of plants along a productivity and disturbance gradient in mediterranean shrublands of south-east Australia. Global Ecology and Biogeography 16, 330-340.
| Crossref | Google Scholar |
Philip J (2019) The institutionalisation of poison: A historical review of vertebrate pest control in Australia, 1814 to 2018. Australian Zoologist 40, 129-139.
| Crossref | Google Scholar |
Reddiex B, Forsyth DM, McDonald-Madden E, Einoder LD, Griffioen PA, Chick RR, Robley AJ (2006) Control of pest mammals for biodiversity protection in Australia I. Patterns of control and monitoring. Wildlife Research 33, 691-709.
| Crossref | Google Scholar |
Rees MW, Pascoe JH, Le Pla M, Robley A, Birnbaum EK, Wintle BA, Hradsky BA (2023) Mesopredator release among invasive predators: controlling red foxes can increase feral cat density and alter their behaviour. Journal of Applied Ecology 60, 1100-1114.
| Crossref | Google Scholar |
Rendall AR, Sutherland DR, Cooke R, White J (2014) Camera trapping: A contemporary approach to monitoring invasive rodents in high conservation priority ecosystems. PLoS ONE 9, e86592.
| Crossref | Google Scholar | PubMed |
Robley A, Newell G, White M, MacDonald A, Sarre S, Triggs B, Currie J, Smith S (2012) Strategic red fox control on bushfire affected public land in Victoria. Black Saturday Victoria 2009 – Natural values fire recovery program. Victorian Department of Environment and Primary Industries, East Melbourne, VIC, Australia.
Robley A, Gormley AM, Forsyth DM, Triggs B (2014) Long-term and large-scale control of the introduced red fox increases native mammal occupancy in Australian forests. Biological Conservation 180, 262-269.
| Crossref | Google Scholar |
Šálek M, Kreisinger J, Sedláček F, Albrecht T (2010) Do prey densities determine preferences of mammalian predators for habitat edges in an agricultural landscape? Landscape and Urban Planning 98, 86-91.
| Crossref | Google Scholar |
Saunders G, Kay B, McLeod L (1999) Caching of baits by foxes (Vulpes vulpes) on agricultural lands. Wildlife Research 26, 335-340.
| Crossref | Google Scholar |
Saunders G, McLeod S, Kay B (2000) Degradation of sodium monofluoroacetate (1080) in buried fox baits. Wildlife Research 27, 129-135.
| Crossref | Google Scholar |
Saunders GR, Gentle MN, Dickman CR (2010) The impacts and management of foxes Vulpes vulpes in Australia. Mammal Review 40, 181-211.
| Crossref | Google Scholar |
Short J, Grigg GC (1982) The abundance of kangaroos in suboptimal habitats: wheat, intensive pastoral, and mallee. Wildlife Research 9, 221-227.
| Crossref | Google Scholar |
Stobo-Wilson AM, Stokeld D, Einoder LD, Davies HF, Fisher A, Hill BM, Mahney T, Murphy BP, Stevens A, Woinarski JCZ, Bawinanga Rangers, Warddeken Rangers, Gillespie GR (2020) Habitat structural complexity explains patterns of feral cat and dingo occurrence in monsoonal Australia. Diversity and Distributions 26, 832-842.
| Crossref | Google Scholar |
Sukma HT, Di Stefano J, Swan M, Sitters H (2019) Mammal functional diversity increases with vegetation structural complexity in two forest types. Forest Ecology and Management 433, 85-92.
| Crossref | Google Scholar |
Taggart PL, Taylor P, Patel KK, Noble DWA (2023) Baiting in conservation and pest management: A systematic review of its global applications in a changing world. Biological Conservation 284, 110214.
| Crossref | Google Scholar |
Thompson JA, Fleming PJS (1994) Evaluation of the efficacy of 1080 poisoning of red foxes using visitation to non-toxic baits as an index of fox abundance. Wildlife Research 21, 27-39.
| Crossref | Google Scholar |
Thomson PC (1986) The effectiveness of aerial baiting for the control of dingoes in north-western Australia. Wildlife Research 13, 165-176.
| Crossref | Google Scholar |
Thomson PC, Kok NE (2002) The fate of dried meat baits laid for fox control: the effects of bait presentation on take by foxes and non-target species, and on caching by foxes. Wildlife Research 29, 371-377.
| Crossref | Google Scholar |
Twigg LE, King DR (1991) The impact of fluoroacetate-bearing vegetation on native Australian fauna: a review. Oikos 61, 412-430.
| Crossref | Google Scholar |
Twigg LE, Martin GR, Eastman AF, King DR, Kirkpatrick WE (2003) Sensitivity of some Australian animals to sodium fluoroacetate (1080): additional species and populations, and some ecological considerations. Australian Journal of Zoology 51, 515-531.
| Crossref | Google Scholar |
Wallach AD, Ritchie EG, Read J, O’Neill AJ (2009) More than mere numbers: the impact of lethal control on the social stability of a top-order predator. PLoS ONE 4, e6861.
| Crossref | Google Scholar | PubMed |
Wallach AD, Bekoff M, Nelson MP, Ramp D (2015) Promoting predators and compassionate conservation. Conservation Biology 29, 1481-1484.
| Crossref | Google Scholar | PubMed |
Wood DH (1980) The demography of a rabbit population in an arid region of New South Wales, Australia. The Journal of Animal Ecology 49, 55-79.
| Crossref | Google Scholar |
Yahner RH (1988) Changes in wildlife communities near edges. Conservation Biology 2, 333-339.
| Crossref | Google Scholar |