Camera traps show foxes are the major predator of flatback turtle nests at the most important mainland western Australian rookery
J. King A B , S. D. Whiting A , P. J. Adams


A Marine Science Program, Department of Biodiversity, Conservation and Attractions, 17 Dick Perry Avenue, Kensington, WA 6151, Australia.
B Terrestrial Ecosystem Science and Sustainability, Harry Butler Institute, Murdoch University, 90 South Street, Murdoch, WA 6150, Australia.
C Present address: Department of Primary Industries and Regional Development, 3 Baron-Hay Court, South Perth, WA 6151, Australia.
D Behavioural Ecology Lab., School of Molecular and Life Sciences, Curtin University, Kent Street, Bentley, WA, Australia.
Abstract
Quantifying marine turtle-nest depredation by daily observer monitoring requires substantial labour.
To quantify nest depredation of the Vulnerable flatback turtle (Natator depressus) at one of its largest rookeries and to compare effectiveness of different monitoring methods.
We used daily observer monitoring and passive infrared-camera traps separately or in combination to record nest depredation, and identified impacts on remaining eggs and hatchlings in depredated nests.
More than a quarter (28%) of the 69 monitored nests were confirmed as depredated, although this figure is an underestimate of total losses because camera traps detected twice as many depredation events (39%) as did direct observation (17%) (P = 0.012). Cameras also provided important behavioural data and identified predators. Although reptile and bird predators were also recorded, the introduced red fox (Vulpes vulpes) was the primary predator identified, digging into 26% of the monitored nests. More than half of the depredation events by foxes (58% or 11/19 nests) occurred late term, between turtles hatching and emerging from the nest, which therefore means that losses calculated through counts of eggshell left in the nest are underestimates because predation of hatchlings is not included by the eggshell count method. Furthermore, almost half (42%) of all depredated nests were depredated more than once, with some nests opened up to five times, potentially exacerbating clutch losses due to environmental exposure.
Egg losses for confirmed depredated nests (27 ± 37%, range 0–100%) were three times the background levels (i.e. 9.3% of eggs that failed to hatch as a result of embryo death during development).
The results of this study strongly warrant the implementation of ongoing fox predator monitoring and mitigation strategies to protect nests at this nationally, and internationally, conservation-significant population of flatback turtles.
Keywords: conservation management, depredation, hatchling, invasive species, monitoring, monitoring methodology, Mundabullangana rookery, red fox.
Introduction
Nest depredation is recognised as a key threat for many marine turtle species populations (Commonwealth of Australia 2017). While native predators exert a degree of damage to marine turtle nests, depredation by invasive terrestrial species introduces additional pressure on hatchling production rates (Table 1). The red fox (Vulpes vulpes) is a major predator of marine turtle nests within its native range (MacDonald et al. 1994; Brown and Macdonald 1995; Yerli et al. 1997). For example, MacDonald et al. (1994) found that 25 of 28 surveyed loggerhead turtle (Caretta caretta) nests were raided by foxes at Dalian Beach in Turkey. Depredation by red foxes has also become a significant problem since their introduction to Australia about 150 years ago. For example, at Ningaloo Station, 70% of loggerhead turtle nests were depredated by foxes prior to fox control (Butcher and Hattingh 2013). At Wreck Rock in Queensland, an estimated 90–95% of marine turtle nests were lost to foxes; following fox population control, the nest depredation rate was reduced to near zero (Limpus 2007).
Species | Location | Predator | Impact (number of nests monitored) | Reference | |
---|---|---|---|---|---|
Natator depressus | Mundabullangana Rookery, Western Australia | Red fox (Vulpes vulpes)A, Gould’s goannas (Varanusgouldii) | 27 ± 37%, range 0–100% of 69 nests depredated; 17% of eggs monitored lost plus unmeasurable loss of hatchlings | Present study | |
Jardine River, Queensland, Australia | Pig (Sus scrofa)A | 90% | Limpus et al. (1993) | ||
Cape York Peninsula, Queensland, Australia | Dingo (Canis familiaris), yellow-spotted goanna (Varanus panoptes), pigA | 22.2% (117 nests) | Nordberg et al. (2019) | ||
Cape Domett, Western Australia | Dingo | Predated minimum 1 clutch per night | Whiting et al. (2009) | ||
Natator depressus, Eretmochelys imbricata, Lepidochelys olivacea | Cape York Peninsula, Queensland, Australia | PigA | 33.5% (161 nests) | Whytlaw et al. (2013) | |
Lepidochelys olivacea | Cape York Peninsula, Queensland, Australia | Dingo, goanna (Varanus panoptes), pigA | 58.4% (243 nests)B | Nordberg et al. (2019) | |
Gahirmatha, India | Feral dog (Canis familiaris)A, pigA, golden jackal (Canis aureus), hyena (Hyaena hyaena), water monitor (Varanus salvator) | 38% (1223 nests) | Behera and Kaiser (2020) | ||
Eretmochelys imbricata | Barbados | Asian mongoose (Herpestes javanicus)A | 27% (551 nests) | Leighton et al. (2011) | |
Caretta caretta, Eretmochelys imbricata and Lepidochelys olivacea | Brazil | Crab-eating fox (Cerdocyon thous) | 22.8% (635 nests) | Longo et al. (2009) | |
Chelonia mydas | Costa Rica | Dog, coati (Nasua narica), black vulture (Coragyps atratus), turkey vulture (Cathartes aura) | 38% (350 nests) | Fowler (1979) | |
Chelonia mydas | Turkey | Red fox, golden jackal | 67.7% (93 nests) | Brown and Macdonald (1995) | |
Caretta caretta and Chelonia mydas | Florida | Raccoon (Procyon lotor) | ~22% (~20 000 nests) | Welicky et al. (2012) | |
Caretta caretta | Wreck Point, Queensland, Australia | Red foxA | 90–95% between 1976–1982 | Limpus (2007) | |
Lace monitor (Varanus varius) and yellow-spotted goanna | 2 seasons: 57.7% (52 nests); 17.4% (46 nests) | Lei and Booth (2017) | |||
45.8% (24 nests) | Madden Hof et al. (2020) | ||||
Florida | Raccoon, grey fox (Urocyon cinereoargenteus) | 26% (901 nests) | Mroziak (1997) | ||
South Carolina | Coyote (Canis latrans) | 33% (66 nests) | Lamarre-Dejesus and Griffin (2013) | ||
Georgia | PigA, raccoon | 10 years (2009–2018) (19 158 nests) | Butler et al. (2020) | ||
Turkey | Red fox | 89% (28 nests) | MacDonald et al. (1994) | ||
Turkey | Red fox | 62.5% (113 nests) | Yerli et al. (1997) |
AIntroduced predator species.
BHalf were meshed.
In addition to direct predation impacts, predators such as foxes excavate turtle chambers in the process of removing eggs or hatchlings, and therefore indirectly damage the remaining eggs or leave chambers exposed (e.g. MacDonald et al. 1994; Nordberg et al. 2019), resulting in embryonic mortality through changed temperatures, or can kill the embryos by rotating the eggs (Limpus 2007). Foxes raid flatback turtle nests, eating eggs and hatchlings at all phases of the incubation period, and often damage more eggs than they eat (Limpus 1971). Fox disturbance potentially increases subsurface mortality, where deceased hatchlings may become an obstruction to hatchlings below, preventing them from digging their way out of the chamber to the surface, such that they die when their energy resources are expended (Limpus 1971). Egg chambers excavated by foxes can also attract other predators such as reptiles (e.g. goannas, Varanus spp.) and crabs (Blamires and Guinea 1998; Lei and Booth 2018) and mammalian omnivores (e.g. bandicoots, Peramelemorphia) (Welicky et al. 2012) and allows them easier access.
In addition to predation of eggs from nests, predators such as foxes also predate on hatchlings as they travel from the nest to the water’s edge (Limpus 1971; Brown and Macdonald 1995). Compounding the effect of fox depredation on marine turtle nests and hatchlings is the fox’s propensity to cache food, including turtle eggs (Limpus 1971; MacDonald et al. 1994). This caching behaviour would result in a greater number of eggs or hatchlings being taken than if predation were only to satiation.
The act of quantifying nest predation can itself increase the risk that predators will locate and depredate the eggs. Studying marine turtles often includes removing and counting eggs, then re-covering the nest (Miller 1999). This activity may have the effect of attracting foxes to a newly laid nest by changing scents and visual look of the nest. Although a number of studies have examined the sensory cues used by foxes during foraging (Spencer 2002; Dawson et al. 2014), the potential role of people covering nests acting as a visual or olfactory cue is largely unexplored (Dawson et al. 2014). Furthermore, the presence of markers used to relocate nests for follow-up assessment could also attract unwanted attention, although most studies testing this have demonstrated that it is the soil disturbance itself that is attractive, not artificial markers (Tuberville and Burke 1994; Burke et al. 2005; Strickland et al. 2010). The monitoring method used is also important for interpretation of results, each method having benefits and drawbacks. Physical examination of nest survival provides important information about the fate of eggs within nests, whereas camera trapping and spoor analysis have the advantage of identifying the nest predators (e.g. Lei and Booth 2017). It is therefore valuable to compare different options for monitoring the fate of turtle nests and test whether the monitoring methods themselves could influence depredation results.
This study investigated depredation on flatback turtle (Natator depressus) nests at one of the largest flatback rookeries in Western Australia (WA). Flatback turtles are listed as Vulnerable under the Australian Environment Protection and Biodiversity Conservation (EPBC) Act 1999. Genetic evidence indicates natal philopatry in the choice of breeding locations for males as well as females for the species (FitzSimmons et al. 2020), and therefore substantial nest predation can cause long-term reduction in their population numbers. We used daily observation and passive infrared remote cameras to record the fate of flatback nests at Mundabullangana Station (‘Munda rookery’) to
quantify the (a) direct and (b) potential indirect effects of depredation on flatback turtle nests,
identify what potential cues nest predators might have used to locate nests,
compare the number of depredation events detected by direct observation or camera trap, and
identify temporal patterns in nest depredation events by foxes.
Methods
This project was approved by the Murdoch University (N2701/14) and Department of Biodiversity, Conservation and Attractions (2013-2t) Animal Ethics Committees.
Study species
Flatback turtles are an Australian endemic species, nesting only on Australian beaches from Exmouth Gulf, WA, to Bundaberg, Queensland (Qld) (Limpus et al. 1988; Limpus 2007). Flatback turtles on the WA Pilbara coast nest during the austral spring and summer, with females laying an average of ~50 eggs per clutch, and have an incubation period of ~39–48 days (Pendoley et al. 2014).
Site description
This study was conducted across three beaches adjacent to Mundabullangana Station (−20.42°S, 118.05°E), a pastoral lease 70 km south of Port Hedland in the Pilbara region, north-western WA (Fig. 1). The Munda rookery consists of the following three beaches: Cowrie Beach ~3 km in length, Victory Beach ~500 m in length, and Munda Beach ~11 km in length, of which nesting occurs on the eastern 6 km. This is one of the largest flatback rookeries in Western Australia, with an estimated 1800 nesting females per year (Pendoley et al. 2014), and is the only large mainland rookery for the north-western shelf of the continent, between Exmouth Gulf and Port Hedland (FitzSimmons et al. 2020).
(a) Location map of Mundabullangana Station, Western Australia. (b) Map of beaches showing camera sites and flatback turtle (Natator depressus) nesting density of beaches.
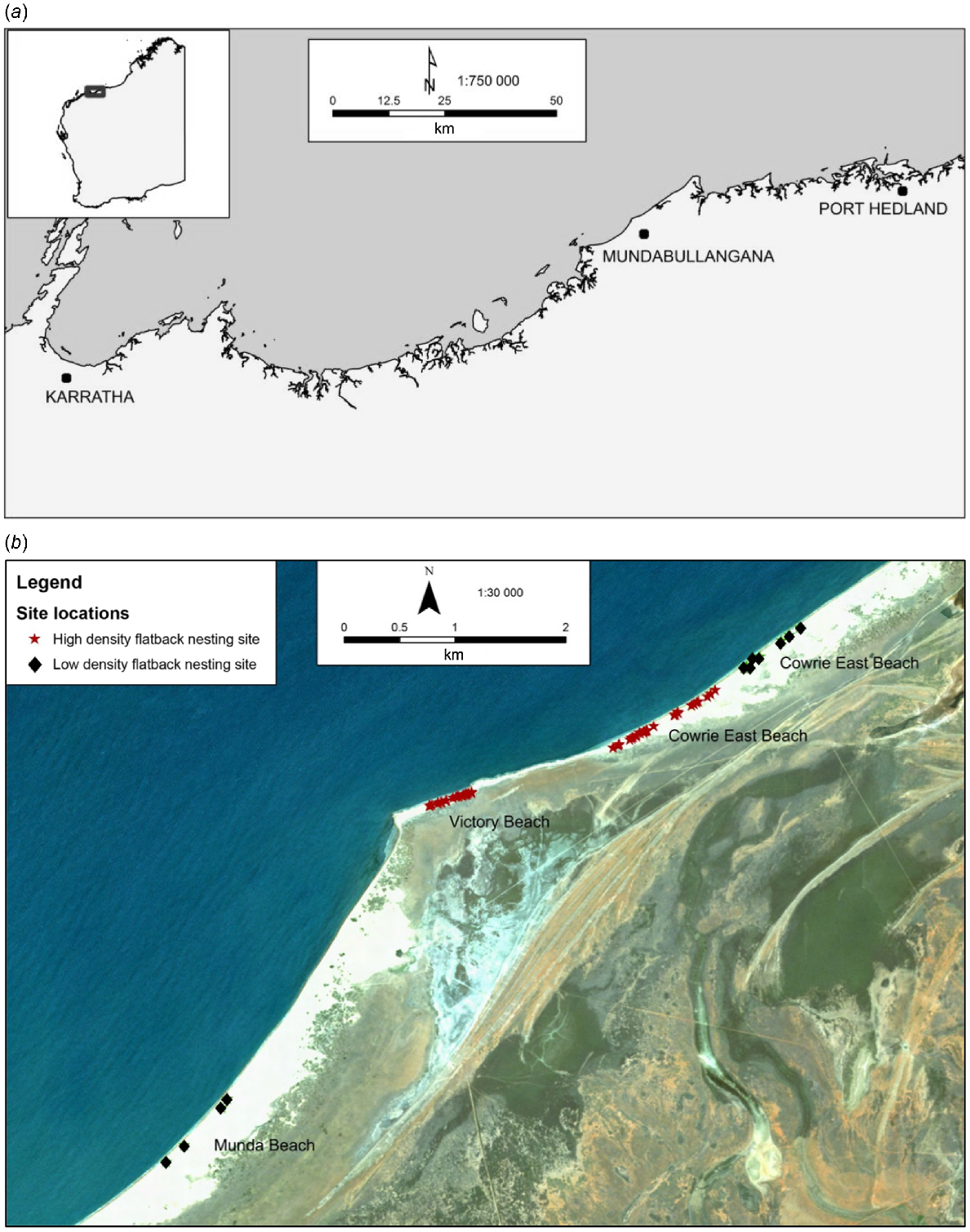
The Munda rookery is flanked by river systems at the north-eastern end of Cowrie Beach and the south-western end of Munda Beach. The coastal zone is characterised by sheltered low wave-energy sandy beaches, with predominately high secondary dunes interspersed with rocky platform shelves and sparse coastal vegetation. The area has a semi-arid climate, with summer maximum temperatures averaging 36°C and often exceeding 40°C (Bureau of Meteorology Climate Statistics, Port Hedland Airport). The region experiences strong winds through the nesting season, as well as cyclonic activity, that can lead to significant beach erosion (causing termination of Year 1 data collection).
The threat of fox depredation on flatback nests was recognised at Munda in the late 1990s, and 1080 toxin baiting by the Department of Biodiversity, Conservation and Attractions was undertaken on an ad hoc basis from 2002 until 2010 on Unallocated Crown Land along the beachfront adjacent to the pastoral property. Continuation of such control was contingent on monitoring data showing the extent and spatial distribution of turtle nest depredation. Mundabullangana Station staff undertook opportunistic control of foxes on the pastoral lease through ground shooting, and 1080 baiting around water points for dingoes (Canis familiaris; Jackson et al. 2017) up to the 2013/14 season (station staff, pers. comm.). Baits for dingoes have a dosage that is lethal for foxes and therefore may result in a reduction of foxes; however, it is undetermined whether any incidental uptake by foxes has occurred during dingo baiting efforts.
Experimental approach
Two camera-trap studies were undertaken in consecutive years at Cowrie, Victory and Munda beaches at the Munda Rookery. In both years, sites were accessed using a quad bike (all terrain vehicle) to move equipment and cover distances effectively; the bike was driven below the high tide mark and not used during the highest tide peaks. The first year was a pilot study (Year 1) to determine predator species, depredation levels, and whether camera monitoring would be feasible. The second year (Year 2) expanded on Year 1 and included modifications to the experimental design and improved camera set-up to reduce the number of false triggers that are likely to be due to capturing moving sand in the distance, wave action, and sun glare (Fig. 2).
(a) Year 1 and (b) Year 2 camera set-up to monitor flatback turtle (Natator depressus) nests at Mundabullangana Station rookery, Western Australia. (a) In Year 1, cameras were attached to a vertical metal stake, ~0.4 m above the sand surface and situated 3 m from the centre of the egg chamber. Vegetation from the field of view had to be removed. (b) In Year 2, cameras were attached near the top of an angled metal stake, ~1.3 m above the sand surface. Minimal vegetation removal was therefore required and there was little sky in most frames, which eliminated false triggers owing to sunrise and sunset. Cameras were orientated in a southerly direction ±45° to reduce glare and wave action (which can cause false triggers).
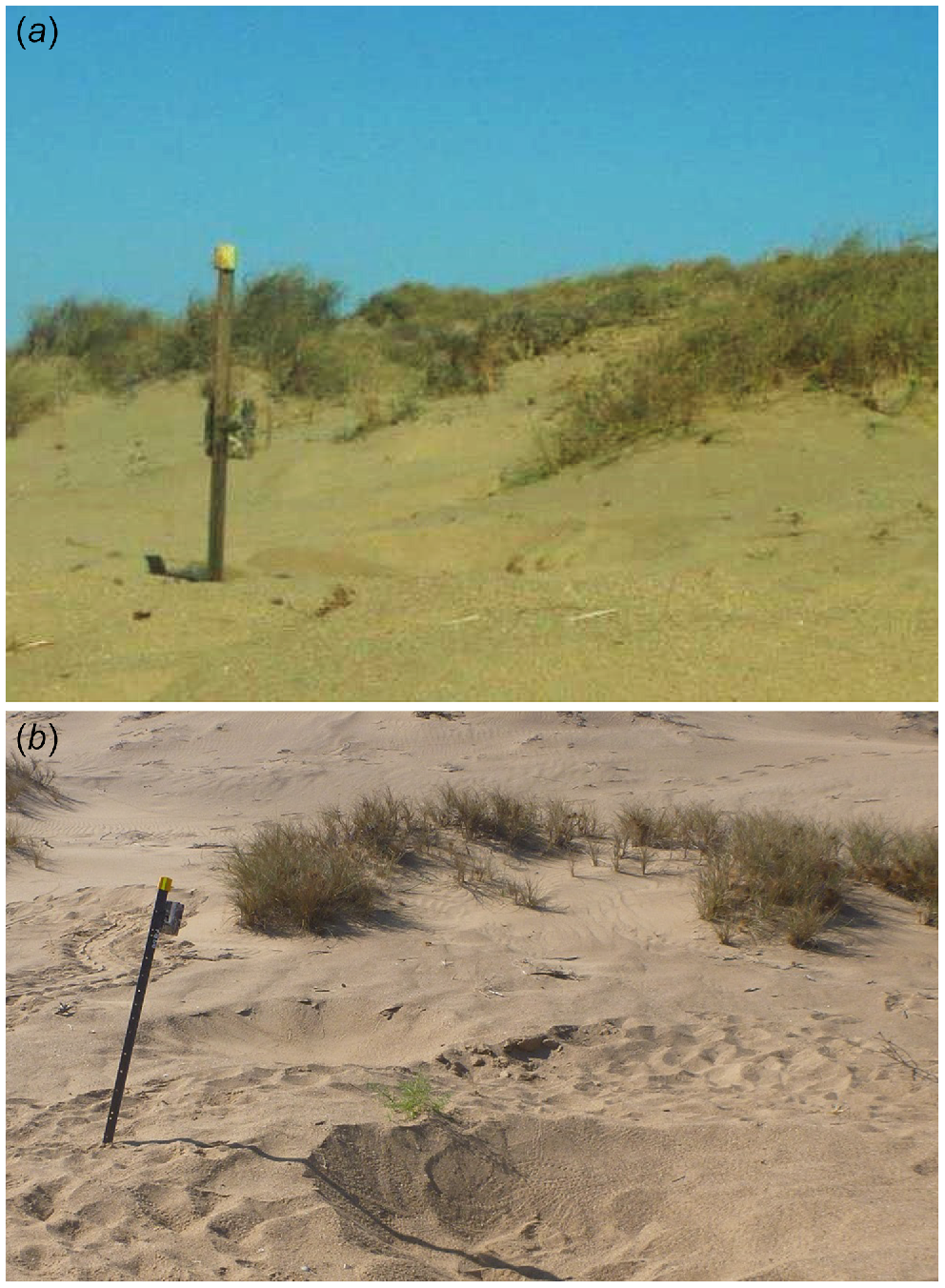
In the first field season, 34 nests above the highest tide mark were monitored by camera trap, with nests chosen at random across the three beaches (numbers shown in Table 2). Date, time and location of each nesting was recorded. Within 2 h of oviposition, eggs were counted for 15 of the 34 nests (randomly chosen). The clutch was uncovered, eggs removed, counted and returned to the chamber with a small piece of plastic tape at the bottom of the chamber (to confirm nest at exhumation) and then re-covered (Limpus and McLachlan 1979). The area above the clutch was temporarily marked (e.g. with a piece of coral or piece of wood) to assist with orientation of a camera trap; the marker was removed once the camera was installed. Passive infrared (PIR) motion-sensing cameras (Reconyx Hyperfire 600) were attached to metal stakes situated 3 m from the centre of the egg chamber (Fig. 2a) and were set to take three consecutive photos per trigger event with no delay between triggers. Cameras were serviced every 2 weeks, when screens were cleaned, battery life and cards checked, and replaced if necessary.
Item | Beach ID | Beach nesting density | Total monitored sites | Monitored | Egg count | Observations | ||||
---|---|---|---|---|---|---|---|---|---|---|
Camera | No camera | Video (natural nest re-cover) | Exhumation (human nest re-cover) | DailyA | WeeklyB | |||||
Year 1 (2013) 15 Nov. to 28 Dec. 2013 | Cowrie | 11 | 11 | 0 | Y | |||||
Victory | 14 | 14 | 0 | Y | ||||||
Munda | 9 | 9 | 0 | Y | ||||||
Total | 34 | 34 | 0 | |||||||
Year 2 (2014) 10 Nov. 2014 to 7 Jan. 2015 | Cowrie East | Low | 8 | 4 | 4 | 0 | 8 | Y | Y | |
Munda | Low | 4 | 2 | 2 | 0 | 4 | NC | Y | ||
Cowrie West | High | 31 | 14 | 5 | 9 | Y | Y | |||
17 | 6 | 11 | Y | Y | ||||||
Victory | High | 26 | 11 | 5 | 6 | Y | Y | |||
15 | 5 | 10 | Y | Y | ||||||
Total | 69 | 31 | 38 | 21 | 48 |
ADaily observations for predation were conducted up to 23 December 2014 during early morning, walking along the beach.
BAdditional physical checks for predation were completed on all sites every 7–10 days when cameras were cleaned, and batteries and cards checked.
CMunda beach was not included in the daily observations owing to logistical access constraints.
A separate turtle-tagging study was in progress on the same beaches as this study (dates shown in Table 2), where researchers surveyed Cowrie and Victory beaches on foot for at least 2–3 h each side of the high tide. This had the potential to disturb any predators during this time.
A cyclone towards the end of the season in Year 1 meant that the cameras were retrieved prematurely. Data from Year 1 has therefore been used only where cameras monitored nests for the full duration of incubation, so that comparable estimates can be made. Unless Year 1 (2013) is mentioned, the results and discussion refer to data collected during 2014.
In the second field season, 69 nests were monitored, 31 by camera and 38 without camera (Table 2). Monitored nests were deployed across the high nesting-density Cowrie West and Victory beaches (>2 nests per 10 m, identified through beach survey counts conducted at the time of the study) and low nesting-density Munda and Cowrie East beaches (≤2 nests per 10 m; Fig. 1b). Markers were installed at each nest with cameras attached to a steel stake located 3 m from the nest (Fig. 2b), facing slightly downward toward the nest. ‘Non-camera’ nests were marked with a wooden stake located 1 m from the egg chamber.
Toward objective (2), to identify what potential cues nest predators might have used to locate nests, we compared depredation rates between nests covered naturally by turtles and those disturbed and covered by researchers (human handling), counting eggs at each of the 69 nests, as follows:
For 48 nests, the clutch was uncovered after the female had left the nest; eggs were removed, counted, and returned to the chamber with a small piece of flagging tape at the bottom of the chamber to confirm nest identification at exhumation after incubation was completed, and the nest was re-covered.
For 21 nests, the eggs dropping into the egg chamber were recorded during oviposition by video recording (Lumix, Panasonic Corporation) and the number of eggs viewed on video were later counted (twice).
Daily physical observations for nest depredation were conducted during early morning at Cowrie East, Cowrie West and Victory beaches until 23 December (the four Munda Beach nests were monitored only via camera trap because of logistical access issues), and additional physical checks for depredation were completed during camera servicing at all four beach sites (Table 2). Any depredation or hatchling activity was recorded and the surface of the sand to a radius of 1 m was brushed clean with a broom to remove existing animal tracks. Turtle tagging for a separate research study was undertaken on Cowrie East, Cowrie West and Victory beaches up until the 17 November 2014 (approximately the first week of nest monitoring for the present study).
Nest depredation was defined as an attempt to enter the nest, regardless of the attempt being successful or not, and was calculated as a percentage of nests monitored. Incubation age was calculated for each depredation event. At about 49 days after oviposition, at the end of the incubation period, nests were exhumed and findings recorded, as follows − hatched eggs (empty shells >50% intact); live hatchlings in nest; dead hatchlings in nest; undeveloped eggs; and unhatched: early-stage embryos, mid-stage embryos, and full-term embryos (Miller 1999).
Data handling
Camera-trap photos were individually viewed as jpeg files and data managed using Microsoft Excel 2010. A photo-capture event was defined as a series of images with a time interval of 30 min between the previous and next group of images of the same species (Dinata et al. 2008). Fox behaviour was categorised as ‘walking through’ (no behaviour directed towards the nest location), ‘sniffing/investigating’, ‘scent-marking’ (urinating or defecating), and ‘depredation’ (digging or consuming hatchlings).
Toward objective (1a), to investigate direct effects of depredation on flatback turtle nests, we analysed the egg fate for 61 ‘known-relocated’ nests monitored in Year 2 (total losses could not be calculated for Year 1 because of incomplete monitoring for the season) that were confirmed by exhuming the marker placed at the nest during laying. We compared nest losses (number of eggs/eggshells counted after hatching as a proportion of the recorded number of eggs laid) for 14 depredated nests (depredation recorded either through direct observation or camera trapping) and 47 intact nests (those where no depredation was recorded) using Mann–Whitney–Wilcoxon Test in R (R Core Team 2018). Toward objective (1b), to investigate potential indirect effects of exposure of the nest chamber and potential thermal change on egg development, we compared proportion of undeveloped/unhatched eggs between depredated and intact nests using a Mann–Whitney–Wilcoxon test. Similalry, to test whether there was an impact of the potential inclusion of debris on hatchling survival, we compared the dead hatchlings (as a proportion of the original eggs laid) between depredated and intact nests using a Mann–Whitney–Wilcoxon test.
Toward objective (2), to identify what potential cues nest predators might have used to locate nests, for 69 nests monitored in Year 2, we used separate Pearson’s χ2 tests (calculated in Microsoft Excel) to compare the fate of nests (depredated or intact) by (2a) nest density (high or low; comparing n = 12 nests on low nesting-density beaches, and n = 57 nests on high nesting-density beaches), (2b) human handling (yes or no; comparing n = 21 nests covered by turtles, and n = 48 nests exhumed and re-covered by the experimenters), or (2c) marking method, comparing a subset of n = 62 nests that could be monitored via daily observation, with n = 26 nests being also monitored by camera (camera) and n = 36 nests that had only a wooden stake positioned near them (no camera). Note that we did not have confidence in recording the positions of known nests without using some physical marking method. For all three tests, expected values were calculated assuming that an equal proportion of nests was depredated by category tested.
Toward objective (3), to compare the number of depredation events detected by direct observation or camera trap, we compared the total numbers for 26 nests monitored using both methods by χ2 test. For this test, expected values were calculated assuming that an equal proportion of nests was identified as depredated by each monitoring method.
Toward objective (4), to identify temporal patterns in nest-depredation events by foxes, we compared the number of fox depredations for the incubation phase when nests would have contained eggs only (0–37 days post-laying; Pendoley et al. 2014), and for older nests likely to contain hatchlings (38–47 days post-laying) by χ2 test, with expected values calculated assuming an equal proportion of depredations per day of monitoring. We also compared fox activity (camera-trap capture rate per 24-h period as the dependent variable), with the day of the field season, year of study, and the presence of people monitoring turtle nesting on the beach during the night (yes or no) as independent factors, by using the glm function in the ‘stats’ package in R.
Statistical significance was accepted at α < 0.05. Values are given as means ±1 s.d. throughout.
Results
In the first year of study (2013), 34 nests were monitored by camera, producing 13 306 photo images over 1437 trap nights, but early retrieval of the cameras because of a cyclone meant that the data for nest depredation were incomplete. In the second year of study (2014), 69 nests (~3427 eggs) were monitored, 31 by camera, producing 13 433 camera-trap images over 1594 camera-trap nights, and another 38 nests were monitored without camera.
Images of other flatback turtles nesting on the beach as well as multiple potential predator species were recorded on camera traps (Table 3). Four mammal species were identified from camera-trap images. Foxes (Fig. 3a, b) were most commonly observed in both years. One dingo and four cats (Felis catus) were observed in Year 1, although neither was observed depredating nests. Varanids were observed over both years, with markedly more records in Year 2. Seven bird species were identified from camera-trap images, of which four species were observed taking hatchlings that had emerged onto the surface: nankeen night herons (Nycticorax caledonicus; Fig. 3c), silver gulls (Chroicocephalus novaehollandiae), crows (Corvus sp.) and whistling kites (Haliastur sphenurus; Fig. 3d). After a nest was depredated, hermit crabs (Fig. 3a) and silver gulls were recorded cleaning up remains of eggs and hatchlings. No ghost crabs were observed taking hatchlings, but camera angles and the passive infrared trigger mechanism of the cameras was likely to limit detections of crabs.
Species | Common name | Year 1 (2013) | Year 2 (2014) | |||
---|---|---|---|---|---|---|
Capture events | Number of cameras | Capture events | Number of cameras | |||
Turtles: | ||||||
Natator depressus | Flatback turtle adult | 37 | 17 | 99 | 26 | |
Predators: | ||||||
Vulpes vulpes | Red fox | 94 | 24 | 235 | 31 | |
Canis familiaris | Dingo | 1 | 1 | 0 | 0 | |
Felis catus | Domestic cat | 4 | 4 | 0 | 0 | |
Varanus gouldii | Goanna | 6 | 5 | 41 | 13 | |
Macropus sp. | Kangaroo | 2 | 2 | 7 | 5 | |
Haliastur sphenurus | Whistling kite | 3 | 1 | 9 | 6 | |
Chroicocephalus novaehollandiae | Silver gull | 4 | 1 | 40 | 17 | |
Corvus sp. | Crow | 12 | 9 | 23 | 12 | |
Nycticorax caledonicus | Nankeen night heron | 9 | 3 | 6 | 4 | |
Aquila audax | Wedge-tailed eagle | 1 | 1 | 0 | 0 | |
Haliaeetus leucogaster | White-bellied sea eagle | 3 | 3 | 1 | 1 | |
Anthus novaeseelandiae | Richard’s pipit | 0 | 0 | 2 | 2 | |
Total | 13 species | 176 | 34 | 463 | 31 |
Capture event is defined as a minimum 30-min interval between the last image and the next.
Predators observed at Mundabullangana Station flatback turtle (Natator depressus) rookery. (a) Red fox (Vulpes vulpes) and hermit crabs (Coenobita variabilis) eating flatback turtle remains. (b) Image of fox about to collect a fourth hatchling before departing the area (c) Nankeen night herons (Nycticorax caledonicus) catching hatchlings as they are walking across beach, and (d) whistling kite (Haliastur sphenurus) predation on emerging hatchlings.
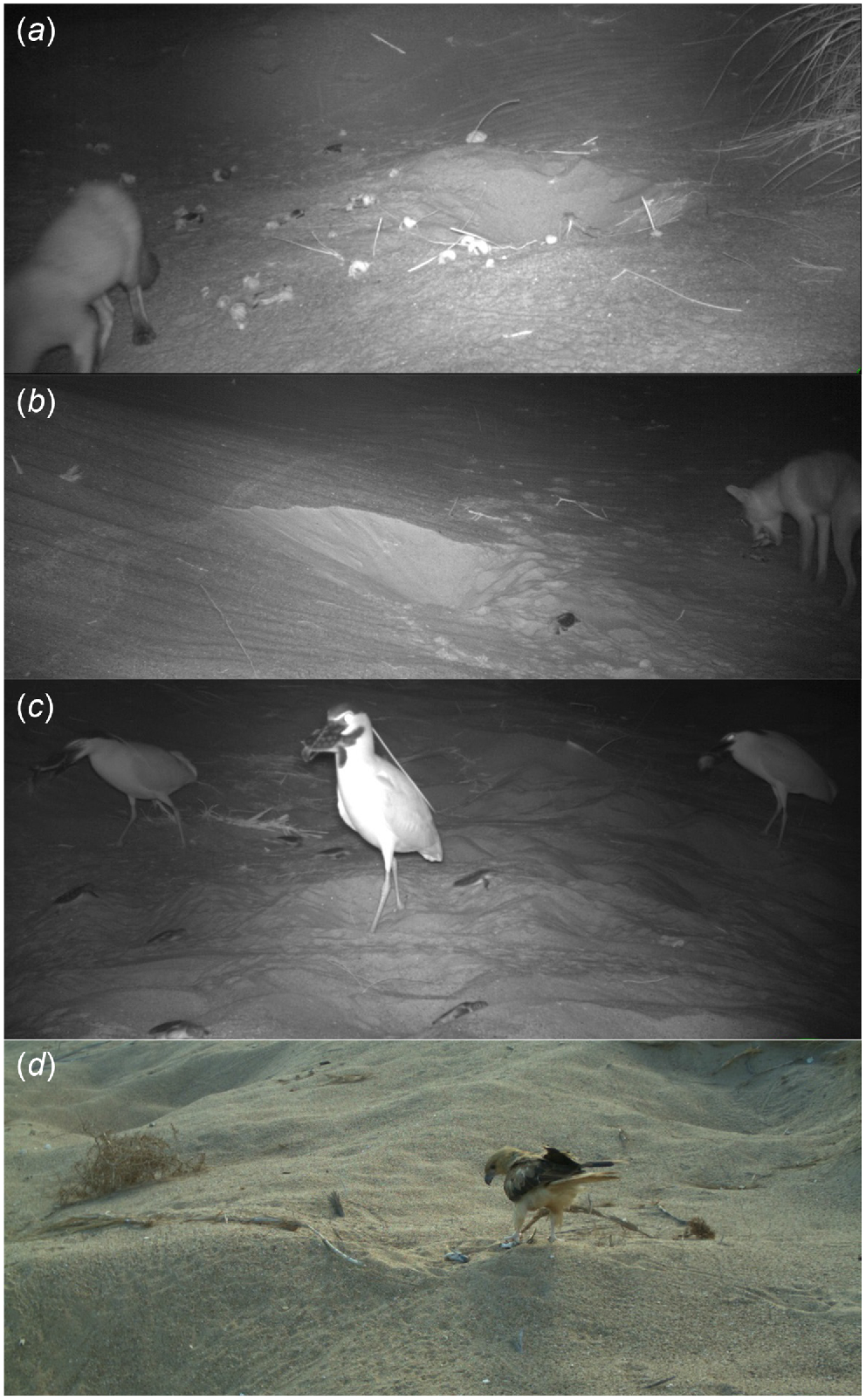
(1a) Direct and (1b) indirect effects of depredation on flatback turtle nests monitored for the complete nesting period in Year 2
Over a quarter (28%, 19 of 69) of the monitored nests were depredated. All predation events were attributable to a species on the basis of visual assessment. Of the 19 nest depredations, 17 were initially depredated by foxes and two by Gould’s goannas (Varanus gouldii; Table 4). Multiple depredation events were observed for eight depredated nests (42% of the 19), with some nests being re-visited up to five times. There were two re-visits by goannas on nests that had initially been opened by foxes. A fox revisited one nest that was initially opened by a goanna. There was no record of multiple depredation events for the non-camera nests.
Species | Predation attempt | |||||
---|---|---|---|---|---|---|
Initial | Second | Third | Fourth | Fifth | ||
Fox | 17 | 6 | 3 | 2 | 2 | |
Goanna | 2 | 2 |
Direct predation losses. Turtles laid an average of 50 ± 7 (range 33–71) eggs, with only 10 of the 3427 eggs being unyolked. Overall average egg losses in Year 2 for 61 ‘known-relocated’ nests were 14 ± 25%. Although losses were more than twice the value for 14 depredated nests (27 ± 37%, range 0–100%) than for 47 intact nests (11 ± 18%, range 0–100%), the difference was not statistically significant (W = 241.5, P = 0.134). Of the 14 depredated nests, predators had removed all eggs from two of these nests, and a third nest had all but one egg removed (i.e. a single hatched egg). However, nest depredation rate recorded through egg remains undoubtedly underestimated total losses, with four of the depredated nests showing no difference between egg (on laying) and eggshell (when exhumed) counts, and two of these nests had images of foxes digging into the nest and eating hatchlings. Additionally, some ‘intact’ nests also showed losses, suggesting they had also been depredated, although this was not observed.
Indirect predation losses. There was no evidence of indirect impacts of nest depredation for 61 ‘known-relocated’ nests, with similar proportions of undeveloped or unhatched eggs (9.3 ± 10.7, range 0–51.0% of eggs laid; W = 337.5, P = 0.890) and dead hatchlings (8.4 ± 17.5, range 0–91.3% of eggs laid; W = 361.5, P = 0.553) present for depredated or intact nests.
(2) Potential cues that nest predators could use to locate nests monitored in Year 2
(2a) Nest density did not increase depredation risk because there was no significant difference in the proportion of depredated nests (, P = 0.228) between high-density (14 depredation events of 57 nests; 24%) and low-density (five depredation events of 12 nests; 41%) nesting beaches in Year 2 (monitored for the complete nesting period). (2b) Although it did not reach statistical significance (, P = 0.103), human handling at the time of laying could increase the risk of predators finding nests, with 33% of nests that were human-covered being later depredated (16 of 48 monitored nests depredated) compared with 14% of turtle-covered nests (3 of 21 monitored nests where egg counts were determined from video recordings depredated). (2c) The presence of a camera did not increase depredation risk, because there was no significant difference (, P = 0.698) in the proportion of depredation events recorded through daily observation for nests that were also monitored with camera (6 of n = 31 camera nests depredated; 19%) or not (6 of n = 38 non-camera nests depredated; 16%; Table 5).
Item | Number of nests monitored | Method of detecting depredation events | Number of depredation events detected | |
---|---|---|---|---|
Both monitoring methods | 31 | Camera trap | 12 (39%) | |
Daily observation | 6A (19%) | |||
Daily observation only | 38 | Daily observation | 6 (16%) | |
Total | 69 | 19 (28%) |
A5 of the 6 predation events were also detected through camera-trap monitoring.
(3) Number of depredation events detected by direct observation or camera trap in Year 2
For the n = 31 nests monitored simultaneously using both methods, camera traps showed twice the depredation events (39%) compared with 19% of nest predation events detected through daily observation (, P = 0.012). Cameras and observation both detected depredation events on five occasions, and cameras recorded a further seven depredation events that were not detected by observation (Table 3). One nest depredation was detected through observation but was not detected by camera. Camera failures also occurred with cameras missing fox activity on at least 11 instances (across nine cameras), with fox footprints evident in subsequent images (when other animals triggered the cameras).
(4) Temporal patterns in nest depredation events by foxes
The red fox was the most common vertebrate predator caught on camera across both years, with 235 camera-trap capture events in Year 2, 55% of which showed the animal simply walking past the camera trap, 38% showed sniffing/investigation, 1% showed urination/defacation, and 6% of which showed nest depredation. Foxes were more active in the first half of the night, with peak activity times from 20:00 hours to 00:00 hours (Fig. 4a); most of their activity was therefore likely to be hours old by the time that nest observers commenced their daily monitoring during daylight the following morning. However, foxes were still active on the beach for close to 1.5 h after sunrise, with two capture of foxes occurring in the middle of the day.
Timing of flatback turtle (Natator depressus) nest depredation at Mundabullangana Station rookery. (a) Circadian pattern of red fox activity captured on camera traps during Year 2 field season and displayed in a 24-h time format. (b) Incubation age for the 19 depredated nests; only initial depredation data by goanna (Varanus spp.) and red fox (Vulpes vulpes) are shown. Egg phase is the time from when the eggs have been laid to hatching, up to and including Day 37. Hatchling phase is from when the first hatchlings have begun to emerge from the eggs, but are still within the sand, from Day 37 onward. (c) Scatterplot of camera-trap rate for red foxes against day of the field season (the field season was cut short in Year 1 because of a cyclone; nest-fate data from Year 1 are included in analyses if the nest incubation period for that nest was complete). Lines and ribbons represent the line of best fit and 95% confidence intervals.
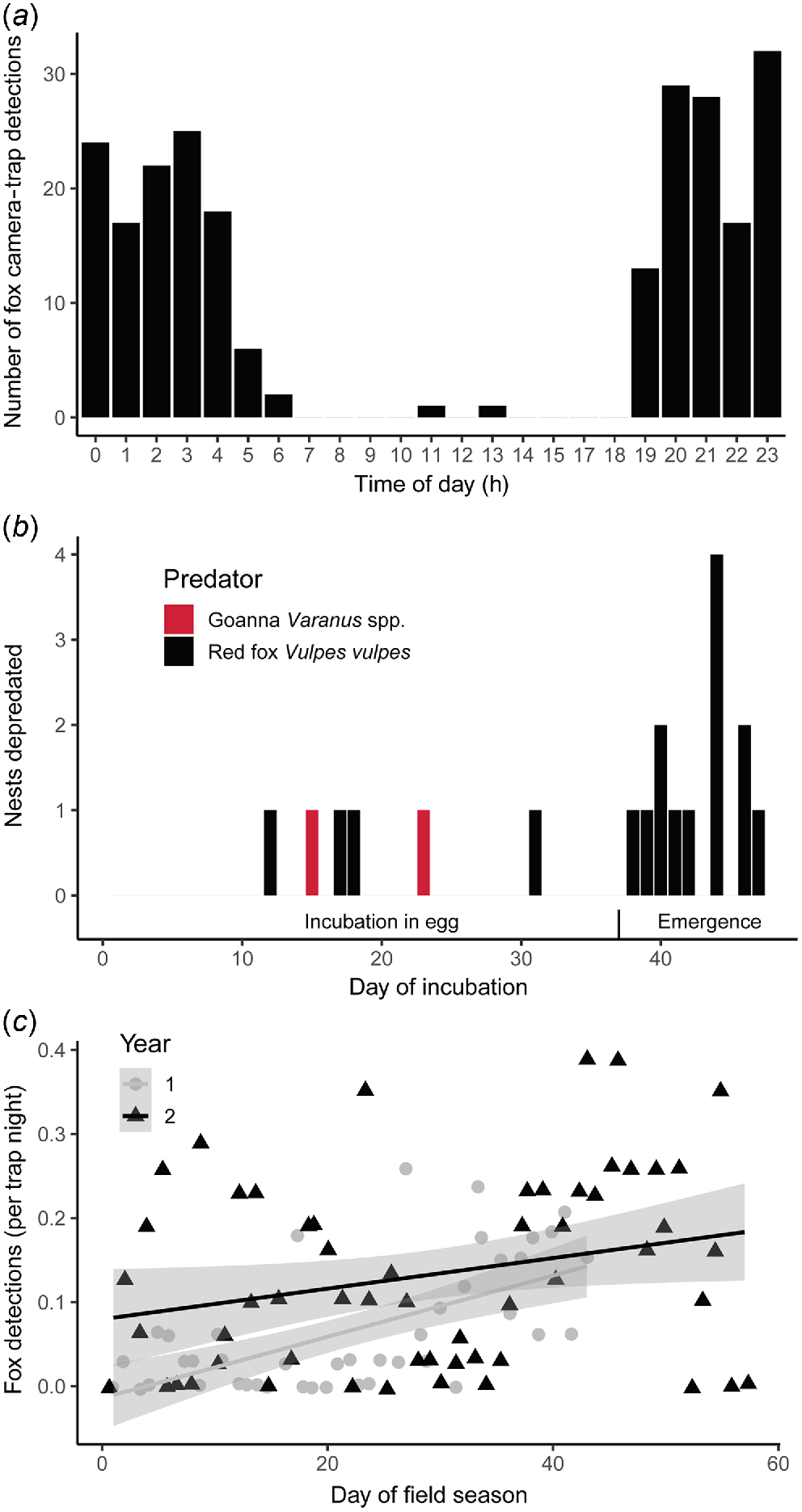
The earliest depredation event of a turtle nest, on Day 12 of incubation, was by a fox. The last depredation event on a nest (excluding re-visits) was on Day 47 after oviposition (likely to be about the time of hatching), and was also by a fox. Four depredation attempts by foxes occurred during the incubation phase (i.e. 0.1 nest depredations per incubation day), whereas 12 were for nests older than 37 days post-laying (i.e. 1.2 nest depredations per incubation day); the difference in depredation rate between these two periods (Fig. 4b) was statistically significant (, P < 0.001). There was also a significant positive relationship between fox detection rate and the date of the field season (t96 = 3.13, P = 0.002) (Fig. 4c), further suggesting that greater predation by foxes of hatchlings than eggs was likely to occur. For this multiple-regression analysis, there was also a significant effect of year of study on fox detection rate (t96 = −1.98, P = 0.050), with overall less fox activity in Year 1 than in Year 2, but no significant difference for fox activity depending on whether or not there were people present on the beaches on each night (t96 = −0.26, P = 0.795).
Images were captured on two occasions of foxes with mouths full of eggs as they walked through the frame. Cameras also captured images confirming foxes’ propensity for surplus killing on four occasions; foxes were observed to dig up multiple hatchlings in a nest, kill them as they were dug up, eat some, then gather as many as four in their mouths and depart (Fig. 3b). On this occasion, other dead hatchlings were left on the surface around the nest.
All the bird and reptile predator activity was recorded during the day; 59% of records were within 4 h of daylight and therefore would likely be detected when daily nest monitoring commenced. Three of the goanna depredations occurred during the incubation phase, with two of these events being the initial depredation of a nest, and the third occurred after a fox had already opened the nest (Fig. 4b). The longest hatchling emergence period for a single nest was 6 days, measured from the day a fox dug up hatchlings to when hatchlings on the beach surface above the same nest were predated by a whistling kite, activating the camera (Fig. 3).
Discussion
Depredation of flatback turtle nests at the Munda rookery (28%; 19 of 69 monitored nests) was somewhat lower than published records for some other rookeries in Australia and other parts of the world (Table 1, and references therein), possibly owing to the aridity of the Munda rookery site, density of predators, or publication bias (with high depredation records more likely to be published). The most common vertebrate predator at the Munda rookery was the red fox (17 of 19 nest depredations). Egg losses for confirmed depredated nests (27 ± 37%, range 0–100%) were three times the background levels of egg losses (9.3 ± 10.7%, range 0–51%), but we now know that this depredation figure omits the unmeasurable number of hatchlings that are also killed and eaten. Eggshell count does not reflect predation of hatchlings during the nest escape process, i.e. once they have left the egg but not yet reached the water, and therefore nest depredation totals derived through observation of eggshell remains are underestimates.
(1) Direct and indirect effects of depredation on flatback turtle nests
Foxes were responsible for the greatest confirmed nest depredations, supporting previous studies showing that the red fox is a significant predator of marine turtle nests across its native (MacDonald et al. 1994; Brown and Macdonald 1995; Yerli et al. 1997) and introduced (Limpus 2007; Nordberg et al. 2019) geographic range. The red fox is also a known predator of freshwater turtle nests (e.g. Burger 1977; Congdon et al. 1983; Thompson 1983; Marchand et al. 2002; Spencer 2002; Dawson et al. 2016), and has been argued to represent a significant threat to conservation-significant populations (but see Chessman 2021).
Repeated nest exhumation is likely to increase the total impact of depredation because it exposes the nest chamber and is also likely to attract other nest predators (e.g. Lei and Booth 2018). With 42% of depredated nests being re-visited a second time, and monitored nests re-opened up to five times, an already-disturbed nest may have greater visual or scent cues and therefore an increased risk of further depredation. Similarly, Welicky et al. (2012) and Yerli et al. (1997) reported greater depredation rates for loggerhead turtle nests that had already been opened by predators.
There are also indirect impacts of nest exhumation itself that could increase losses, namely, decreased hatchling emergence for partially depredated nests (Nordberg et al. 2019) and loss of eggs damaged and destroyed in the act of depredation by foxes that were not directly consumed or taken by the predator (Limpus 1971). Although egg losses for disturbed nests were twice those for intact nests, our small sample size precluded statistical significance in this study and we found no difference in egg and hatchling mortality for depredated and intact nests. We also note that the majority of nest depredations we recorded were late-term, when hatchlings were about to emerge or already emerging. Furthermore, the semiarid climate and sand composition at the Munda rookery also readily collapses back into diggings, which can also help preserve viability of remaining eggs.
(2) Potential cues nest predators could use to locate nests
We found no effect of nest density on depredation risk. Additionally, despite camera-monitored nests having additional sensory cues (i.e. infrared flash visual cues and olfactory cues owing to weekly camera servicing), there was no significant difference in depredation rate (detected through daily observations) for camera versus stake-marked nests, suggesting that the presence of cameras did not attract (or deter) foxes. This result supports previous studies that have examined nest-marking methods (Tuberville and Burke 1994; Burke et al. 2005; Strickland et al. 2010). In contrast, Mroziak et al. (2000) and Nordberg et al. (2019) found that the presence of wire cages/mesh may result in increased depredation attempts by raccoon (Procyon lotor), grey fox (Urocyon cinereoargenteus) and yellow-spotted goanna (Varanus panoptes). We could not compare our marked nests with completely unmarked nests owing to a failure to be able to relocate them, but future studies could potentially use high-accuracy GPS or buried markers (e.g. VHF transmitters) to re-find unmarked nests.
It is possible that human handling at the time of laying could increase the risk of predators finding nests, although this result did not reach statistical significance owing to our sample size. It has been shown that foxes will investigate and depredate artificial freshwater turtle nests where there has been any sign of soil disturbance (Dawson et al. 2014), which presumably acts as a cue for some predators. The likelihood of olfactory cues left by researchers is also a possibility that needs to be considered, especially when dealing wth predators that use scent to locate nests.
(3) Number of depredation events detected by direct observation or camera trap
While there was no evidence that camera traps made nests more vulnerable to being located (as indicated from visual observation data), they effectively doubled the number of depredation events detected compared with daily in-person observation, and confirmed the identity of nest and hatchling predators. Daily visitation by observer to record nest depredation events requires that the observer accurately interprets the signs of a predator, including tracks, disturbance of the ground, and egg/hatchling remains. Strong winds and the nesting actions of other marine turtles can obscure or cover predator sign when nests are visited hours later during daylight, and visitation of multiple predators within a 24-h period also cannot be documented. Quantifying the degree of nest depredation is further confounded by the removal of eggs or hatchling remains by birds or crabs, as observed in this study. Recording nest success by observation alone can therefore significantly underestimate depredation rates. Another advantage of camera traps was that it showed the identity of the predator, timing and frequency of their activity, and showed predator behaviour around the nests. Camera trap data also confirmed predation on hatchlings, which is not possible with other means of nest monitoring (e.g. daily observations, track counts in the sand, Lei and Booth 2017).
(4) Temporal patterns in nest depredation events by foxes
We found that foxes were more likely to depredate late-term nests that were more likely to contain emerging hatchlings, which may be due to noise the hatchlings make as they pip their eggs (Guinea et al. 2015) or additional olfactory cues on release of fluids from the eggs (Lei and Booth 2018). We also recorded increased fox presence on beaches late in the season (see also Lei and Booth 2017). Nest depredation records based on observer monitoring (i.e. comparing counts of eggshells with eggs laid) do not capture loss of hatchlings and will therefore underestimate total predation losses. Furthermore, we observed foxes leaving nests with eggs and hatchlings in their mouth suggesting caching behaviour (MacDonald et al. 1994). These findings could suggest that synchronous hatching, while having benefits in conserving energy (Rusli et al. 2016), may assist predators in locating the nest as the hatchlings wait to emerge from below the surface. Our findings are inconsistent with those of Limpus (1971), who claimed that depredation of flatback turtle nests by foxes occurs at all stages of incubation. Our data also differ from studies showing 90% of green turtle (Chelonia mydas) nests at Akyatan Beach in Turkey were depredated by foxes in the first 3 days (Brown and Macdonald 1995), and loggerhead turtle nests were depredated within the first 48 h of laying (MacDonald et al. 1994), when soil disturbance was likely to be an important factor in locating nests (Lei and Booth 2018).
Limitations of this study
Although camera traps proved to be a useful method of monitoring nest depredation and detecting predator activity and behaviour on the beach, they still missed some events and have their own limitations (Meek et al. 2015). Camera traps may fail to capture predation events because of the hot environment, where temperature range was between 25°C and 44°C, reducing contrast between the predator species’ body temperature and the environment. Cameras may be particularly problematic in detecting reptile predators that have a body temperature closer to ambient temperature on warm days, and are therefore likely to underestimate predation by goannas (but see Lei and Booth 2017, and Madden Hof et al. 2020 who, nevertheless, recorded substantial numbers of varanid detections on camera trap). In 11 instances, fox footprints were noticed when another source triggered the camera (e.g. sand movement from a female turtle out of field of view), suggesting fox activity that had not been detected by camera trap. The potential for false negatives means that care needs to be taken when estimating nest depredation events from camera-trap data.
Conclusions and recommendations for management
Like any sea turtle rookery, Mundabullangana will be subjected to multiple pressures. We have quantitatively shown that fox predation of eggs is relatively high at 26% of monitored nests and, with further investigation, this might be refined spatially between low- or high-density beaches (Fowler 1979), and temporally within and among seasons. Total predation rates (by all predators, including foxes, crabs, varanids and birds) would be higher if the predation of live hatchlings during transit from the nest to the water could be added. In addition, anecdotal evidence suggests further damage to nests and beaches due to inundation (e.g. Behera and Kaiser 2020) and cyclonic activity, as witnessed in the present study. All pressures combined mean that the likely total loss of eggs and hatchlings would exceed 30%. Being one the largest rookeries for the flatback turtle globally, and its use as a reference site for a major gas development at another rookery (Gorgon Gas Project), management actions to reduce nest depredation by the introduced red fox should be implemented where is it is feasible and cost effective.
We therefore suggest that long-term monitoring of egg and hatchling predation is established (frequency to be determined) and combined with invasive animal population control. The reduction of fox numbers is a highly feasible and cost-effective action if the main goal is to protect turtle eggs and hatchlings along the narrow coast strip that supports the turtle nesting beaches. Fox mitigation presents no adverse risks to turtles and furthermore provides additional ecological value to the wide area where native fauna populations are generally depleted in the presence of introduced grazing and predator species. Monitoring predation impacts (e.g. Madden Hof et al. 2020) and fox population size will inform an adaptive management approach that ensures that effective and efficient predator control continues into the future.
Acknowledgements
Thanks go to Michael Thompson for permission to access the rookery on Mundabullangana Station, station staff for assistance and information, and field volunteers Mick Davies, Clem Whittles. Thanks also go to Pendoley Environmental staff and volunteers, especially Anna Vitenbergs, Anna and Tyrone. Thanks go to Rachael Marshall, Alicia Whittington, Margie Mohring and Jo Williams for support.
References
Behera S, Kaiser H (2020) Threats to the nests of Olive Ridley Turtles (Lepidochelys olivacea Eschschholtz, 1829) in the world’s largest sea turtle rookery at Gahirmatha, India: need for a solution. Herpetology Notes 13, 435-442.
| Google Scholar |
Blamires SJ, Guinea ML (1998) Implications of nest site selection on egg predation at the sea turtle rookery at Fog Bay. In ‘Proceedings of the Marine Turtle Conservation and Management in Northern Australia Workshop, 3–4 June 1997, Darwin, NT, Australia’. (Eds R Kennett, A Webb, G Duff, ML Guinea, GJE Hill) pp. 20–24. (Centre for Indigenous and Natural Resources, Centre for Tropical Wetlands Management: Darwin, NT, Australia)
Brown L, Macdonald DW (1995) Predation on green turtle Chelonia mydas nests by wild canids at Akyatan beach, Turkey. Biological Conservation 71, 55-60.
| Crossref | Google Scholar |
Burger J (1977) Determinants of hatching success in Diamondback Terrapin, Malaclemys terrapin. American Midland Naturalist 97, 444-464.
| Crossref | Google Scholar |
Burke RL, Schneider CM, Dolinger MT (2005) Cues used by raccoons to find turtle nests: effects of flags, human scent, and diamond-backed terrapin sign. Journal of Herpetology 39, 312-315.
| Crossref | Google Scholar |
Butler ZP, Wenger SJ, Pfaller JB, Dodd MG, Ondich BL, Coleman S, Gaskin JL, Hickey N, Kitchens-Hayes K, Vance RK, Williams KL (2020) Predation of loggerhead sea turtle eggs across Georgia’s barrier islands. Global Ecology and Conservation 23, e01139.
| Crossref | Google Scholar |
Chessman BC (2021) Introduced red foxes (Vulpes vulpes) driving Australian freshwater turtles to extinction? A critical evaluation of the evidence. Pacific Conservation Biology 28(6), 462-471.
| Crossref | Google Scholar |
Congdon JD, Tinkle DW, Breitenbach GL, Van Loben Sels RC (1983) Nesting ecology and hatching success in the turtle Emydoidea blandingi. Herpetologica 39, 417-429.
| Google Scholar |
Dawson SJ, Adams PJ, Huston RM, Fleming PA (2014) Environmental factors influence nest excavation by foxes. Journal of Zoology 294, 104-113.
| Crossref | Google Scholar |
Dawson SJ, Crawford HM, Huston RM, Adams PJ, Fleming PA (2016) How to catch red foxes red handed: identifying predation of freshwater turtles and nests. Wildlife Research 43, 615-622.
| Crossref | Google Scholar |
Dinata Y, Nugroho A, Achmad Haidir I, Linkie M (2008) Camera trapping rare and threatened avifauna in west-central Sumatra. Bird Conservation International 18, 30-37.
| Crossref | Google Scholar |
FitzSimmons NN, Pittard SD, McIntyre N, Jensen MP, Guinea M, Hamann M, Kennett R, Leis B, Limpus CJ, Limpus DJ, McCann MJ, MacDonald AJ, McFarlane G, Parmenter CJ, Pendoley K, Prince RT, Scheltinga L, Theissinger K, Tucker AD, Waayers D, Whiting A, Whiting S (2020) Phylogeography, genetic stocks, and conservation implications for an Australian endemic marine turtle. Aquatic Conservation: Marine and Freshwater Ecosystems 30, 440-460.
| Crossref | Google Scholar |
Fowler LE (1979) Hatching success and nest predation in the green sea turtle, Chelonia mydas, at Tortuguero, Costa Rica. Ecology 60, 946-955.
| Crossref | Google Scholar |
Guinea M, Giuliano C, Wright D, Raith A (2015) Sounds emitted by flatback (Natator depressus) and olive ridley (Lepidochelys olivacea) sea turtle hatchlings. In ‘Proceedings of the Second Australian and Second Western Australian Marine Turtle Symposia, 25–27 August 2014, Perth, WA, Australia’. (Eds SD Whiting, A Tucker) pp. 37–39. (Department of Parks and Wildlife)
Jackson SM, Groves CP, Fleming PJS, Aplin KP, Eldridge MDB, Gonzalez A, Helgen KM (2017) The Wayward dog: is the Australian native dog or Dingo a distinct species? Zootaxa 4317, 201-224.
| Crossref | Google Scholar |
Lamarre-Dejesus AS, Griffin CR (2013) Use of habanero pepper powder to reduce depredation of loggerhead sea turtle nests. Chelonian Conservation & Biology 12, 262-267.
| Crossref | Google Scholar |
Lei J, Booth DT (2017) Who are the important predators of sea turtle nests at Wreck Rock beach? PeerJ 5, e3515.
| Crossref | Google Scholar |
Lei J, Booth DT (2018) How do goannas find sea turtle nests? Austral Ecology 43, 309-315.
| Crossref | Google Scholar |
Leighton PA, Horrocks JA, Kramer DL (2011) Predicting nest survival in sea turtles: when and where are eggs most vulnerable to predation? Animal Conservation 14, 186-195.
| Crossref | Google Scholar |
Limpus CJ (1971) The flatback turtle, Chelonia depressa Garman in Southeast Queensland, Australia. Herpetologica 27, 431-446 Available at https://www.jstor.org/stable/3891281.
| Google Scholar |
Limpus CJ, McLachlan C (1979) Observations on the leatherback turtle, Dermochelys coriacea (L.), in Australia. Wildlife Research 6, 105-116.
| Crossref | Google Scholar |
Limpus CJ, Gyuris E, Miller JD (1988) Reassessment of the taxonomic status of the sea turtle genus Natator McCulloch, 1908, with a redescription of the genus and species. Transactions of the Royal Society of South Australia 112, 1-9.
| Google Scholar |
Limpus CJ, Couper PJ, Couper KLD (1993) Crab Island revisited: reassessment of the world’s largest flatback turtle rookery after twelve years. Memoirs of the Queensland Museum 33.1, 277-289 Available at https://eurekamag.com/research/037/331/037331913.php.
| Google Scholar |
Longo G, Pazeto FD, de Abreu JAG, Floeter SR (2009) Flags reduce sea turtle nest predation by foxes in NE Brazil. Marine Turtle Newsletter 125, 1-3.
| Google Scholar |
MacDonald DW, Brown L, Yerli S, Canbolat A-F (1994) Behavior of red foxes, Vulpes vulpes, caching eggs of loggerhead turtles, Caretta caretta. Journal of Mammalogy 75, 985-988.
| Crossref | Google Scholar |
Madden Hof CA, Shuster G, Mclachlan N, Mclachlan B, Giudice S, Limpus C, Eguchi T (2020) Protecting nests of the Critically Endangered South Pacific loggerhead turtle Caretta caretta from goanna Varanus spp. predation. Oryx 54, 323-331.
| Crossref | Google Scholar |
Marchand MN, Litvaitis JA, Maier TJ, DeGraaf RM (2002) Use of artificial nests to investigate predation on freshwater turtle nests. Wildlife Society Bulletin 30, 1092-1098.
| Google Scholar |
Meek PD, Ballard G-A, Fleming PJS (2015) The pitfalls of wildlife camera trapping as a survey tool in Australia. Australian Mammalogy 37, 13-22.
| Crossref | Google Scholar |
Mroziak ML, Salmon M, Rusenko K (2000) Do wire cages protect sea turtles from foot traffic and mammalian predators? Chelonian Conservation and Biology 3, 693-698.
| Google Scholar |
Nordberg EJ, Macdonald S, Zimny G, Hoskins A, Zimny A, Somaweera R, Ferguson J, Perry J (2019) An evaluation of nest predator impacts and the efficacy of plastic meshing on marine turtle nests on the western Cape York Peninsula, Australia. Biological Conservation 238, 108201.
| Crossref | Google Scholar |
Pendoley KL, Bell CD, McCracken R, Ball KR, Sherborne J, Oates JE, Becker P, Vitenbergs A, Whittock PA (2014) Reproductive biology of the flatback turtle Natator depressus in Western Australia. Endangered Species Research 23, 115-123.
| Crossref | Google Scholar |
Rusli MU, Booth DT, Joseph J (2016) Synchronous activity lowers the energetic cost of nest escape for sea turtle hatchlings. Journal of Experimental Biology 219, 1505-1513.
| Crossref | Google Scholar |
Spencer R-J (2002) Experimentally testing nest site selection: fitness trade-offs and predation risk in turtle. Ecology 83, 2136-2144.
| Crossref | Google Scholar |
Strickland J, Colbert P, Janzen FJ (2010) Experimental analysis of effects of markers and habitat structure on predation of turtle nests. Journal of Herpetology 44, 467-470.
| Crossref | Google Scholar |
Thompson MB (1983) Populations of the Murray River Tortoise, Emydura (Chelodina): the effect of egg predation by the red fox, Vulpes vulpes. Wildlife Research 10, 363-371.
| Crossref | Google Scholar |
Tuberville TD, Burke VJ (1994) Do flag markers attract turtle nest predators? Journal of Herpetology 28, 514-516.
| Google Scholar |
Welicky RL, Wyneken J, Noonburg EG (2012) A retrospective analysis of sea turtle nest depredation patterns. Journal of Wildlife Management 76, 278-284.
| Crossref | Google Scholar |
Whiting AU, Thomson A, Chaloupka M, Limpus CJ (2009) Seasonality, abundance and breeding biology of one of the largest populations of nesting flatback turtles, Natator depressus: Cape Domett, Western Australia. Australian Journal of Zoology 56, 297-303.
| Crossref | Google Scholar |
Whytlaw PA, Edwards W, Congdon BC (2013) Marine turtle nest depredation by feral pigs (Sus scrofa) on the Western Cape York Peninsula, Australia: implications for management. Wildlife Research 40, 377-384.
| Crossref | Google Scholar |
Yerli S, Canbolat AF, Brown LJ, Macdonald DW (1997) Mesh grids protect loggerhead turtle Caretta caretta nests from red fox Vulpes vulpes predation. Biological Conservation 82, 109-111.
| Crossref | Google Scholar |