A review of viral and parasitic infections in wild deer in Australia with relevance to livestock and human health
Jose L. Huaman A , Karla J. Helbig A , Teresa G. Carvalho A , Mark Doyle B , Jordan Hampton



A Department of Microbiology, Anatomy, Physiology and Pharmacology, School of Agriculture, Biomedicine and Environment, La Trobe University, Melbourne, Vic., Australia.
B South East Local Land Services, Bega, NSW, Australia.
C Faculty of Science, University of Melbourne, Parkville, Vic., Australia.
D Vertebrate Pest Research Unit, New South Wales Department of Primary Industries, Orange Agricultural Institute, Orange, NSW, Australia.
E Department of Agriculture and Fisheries, Invasive Plants and Animals Research, Biosecurity Queensland, Ecosciences Precinct, Brisbane, Qld, Australia.
F Department of Environment, Land, Water and Planning, Arthur Rylah Institute for Environmental Research, Melbourne, Vic., Australia.
G Environmental and Conservation Sciences, Murdoch University, Perth, WA, Australia.
Wildlife Research 50(9) 593-602 https://doi.org/10.1071/WR22118
Submitted: 30 June 2022 Accepted: 28 April 2023 Published: 11 July 2023
© 2023 The Author(s) (or their employer(s)). Published by CSIRO Publishing. This is an open access article distributed under the Creative Commons Attribution-NonCommercial-NoDerivatives 4.0 International License (CC BY-NC-ND)
Abstract
Wild animals harbour a diverse range of pathogens. In Europe and North America, cervids (Family Cervidae) can act as reservoirs for viral, prion, bacterial, and parasitic infections. Wild deer often inhabit agricultural land, therefore representing a biosecurity risk due to their potential ability to transmit diseases to livestock. Multiple studies have investigated the infection status of wild deer in Australia, mostly during the 1970s and 1980s, and deer populations have increased greatly in abundance and distribution since then. Those studies provide an important baseline for the pathogens carried by wild deer in Australia but are limited by small sample size, the small number of deer species studied, and the disease detection methods used. Recent investigations using ELISA (Enzyme-Linked Immunosorbent Assay), PCR-based assays, and next-generation sequencing have substantially increased our understanding of viral and parasitic infections in Australian deer. These studies indicate that deer may act as reservoirs for pathogens such as Pestivirus, Neospora caninum and Entamoeba bovis. The use of next-generation sequencing has led to the discovery of novel viruses such as Picobirnavirus and a novel species of the genus Bopivirus, both of which pose transmission risks for domestic animals. Recent research confirms that wild deer could be a future source of viral and parasitic infections for domestic livestock and other wildlife species.
Keywords: chital deer, fallow deer, genetics, infectious disease, invasive species, pest control, rusa deer, sambar deer, wildlife diseases.
Introduction
Multiple infectious diseases, including avian influenza virus, African swine fever virus and coronaviruses have emerged or re-emerged in recent decades, raising questions about their pathogenesis and epidemiology (Rhyan and Spraker 2010; Barroso et al. 2021; McClymont et al. 2022). Wildlife populations are the most significant source of emergent infectious diseases that impact human health, biodiversity, and agriculture (Morner et al. 2002). As a result, pathogen transmission between wildlife and livestock is a global concern (Rhyan and Spraker 2010). Of particular importance in wildlife health are pathogens that do not exhibit host specificity or can infect host species across different taxa. In this context, it is estimated that 77% of pathogens detected in livestock can infect multiple wild and domestic species (Woolhouse et al. 2001), and can therefore be acquired by other species. Wild animals typically carry more pathogens than are present in domestic animals (Walker et al. 2017), and some of these pathogens do not need to persist for an extended period within a wildlife population for transmission to livestock to occur (Morgan et al. 2006).
Due to altered landscapes or the introduction of non-native species, changes in wildlife density and habitat use lead to new interfaces between livestock and wildlife, potentially exacerbating processes that promote pathogen transmission (Rhyan and Spraker 2010; Miller et al. 2013). Because cervids (Family Cervidae) are ruminants and closely related to economically important livestock species such as cattle, sheep, and goats, it is not surprising that they share many pathogens, including several of major agricultural significance. Here, we focus on the role of wild deer as wildlife hosts of viral and parasitic pathogens in Australia. The role of wild deer as a source of infection for livestock has been widely studied in Europe and North America, where wildlife–livestock interactions have increased (Böhm et al. 2007; Conner et al. 2008; Martin et al. 2011; Ruiz-Fons et al. 2014).
Deer are not native to Australia and were first introduced in the early 19th century from Europe and Asia (Bentley 1998; Hall and Gill 2005). Six species – chital (Axis axis), fallow (Dama dama), hog (Axis porcinus), red (Cervus elaphus), rusa (Cervus timorensis), and sambar (Cervus unicolor) deer – have established self-sustaining wild herds in Australian habitats (Bentley 1998; Moriarty 2004a). Wild deer are present in all Australian states and territories, but are most widespread and abundant in Tasmania, Victoria, New South Wales, and the Australian Capital Territory (Forsyth et al. 2015; Davis et al. 2016; Forsyth et al. 2016; Crittle and Millynn 2020). For approximate current and potential distributions of the six species of wild deer in Australia, see fig. 2 in Davis et al. (2016). Wild deer populations continue to increase in size and distribution in mainland Australia and Tasmania (Moriarty 2004a; Davis et al. 2016; Cunningham et al. 2022). Wild deer are particularly widespread and abundant in south-eastern Australia, where population densities of up to 39 per km2 occur in agricultural landscapes (Bengsen et al. 2022). Bioclimatic modelling, which matches animal species’ requirements to suitable environments, suggests that deer currently occupy a small proportion of their potential distribution in Australia (Moriarty 2004a; Davis et al. 2016).
Australia is currently free of many animal pathogens detected elsewhere in the world, such as those causing Foot and Mouth Disease or Lumpy Skin Disease, and exotic diseases remain a major threat to Australia’s livestock industry as well as to human and wildlife health. Therefore, ongoing monitoring to determine the presence of pathogens in wildlife is crucial to identifying potential reservoirs of infectious diseases and preventing future disease outbreaks. Wild deer populations currently pose a disease risk (Fig. 1), and further increases in size and distribution could increase that risk. However, data about the infection status of Australian wild deer populations are sparse, with only six studies reported before 2014, all of them identified in localised surveys conducted 41–56 years ago (Munday 1966; Munday 1972; Presidente and Westbury 1979; Slee and Presidente 1981; English 1982; McKenzie et al. 1985). More recently, however, at least 11 further surveys have been reported, many of them our own work (Davies 2014; Koehler et al. 2016; Panozzo 2018; Huaman et al. 2020, 2021a, 2021b, 2021c, 2022a, 2022b; Jenkins et al. 2020; Lamb et al. 2021).
A farm paddock in Victoria, Australia. Note the presence of deer scats in the foreground and domestic animals (horses) in the background.
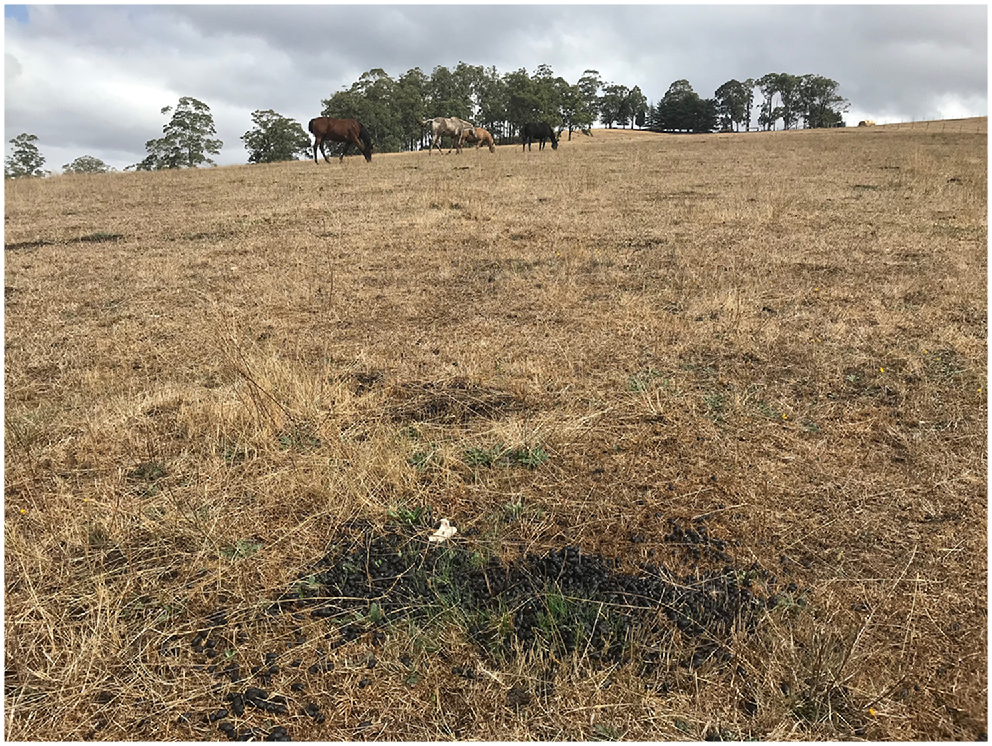
Here, we provide an updated assessment of the prevalence of both viral and parasitic infections in wild deer in Australia. We conducted this assessment by searching the PubMed database using the keywords ‘Deer’, ‘Australia’ and ‘Disease’; ‘Deer’, ‘Australia’ and ‘Pathogen’, or ‘Deer’, ‘Australia’, and ‘Parasite’ (in the title and abstract) and inspecting the references in the publications identified in this literature search. Our search was conducted on 02 December 2022. We also highlight how advanced genomic techniques have increased our understanding of wild deer diseases. In this present review we aimed to: (1) review current knowledge of deer infection in Australia; (2) examine how this compares to deer overseas; and (3) assess the implications for wild and domestic animal populations and humans.
Viral diseases in deer
Viral infections of deer have been examined across multiple decades in Australia. All six deer species have been assessed for various viral infections; however, most data are available for fallow deer. The first study of diseases in Australia’s wild deer was published in 1966 (Munday 1966) and assessed fallow deer in Tasmania for the presence of Bovine Herpesvirus (BoHV-1) and Pestivirus. BoHV-1 causes two clinical diseases in cattle: infectious bovine rhinotracheitis and infectious pustular vulvovaginitis. The prevalence of antibodies to BoHV-1 in adult cattle in Australia is typically 25–40%, with the virus occurring across the continent but being particularly associated with feedlots (Gu and Kirkland 2008). No BoHV-1 was detected in the survey of Munday (1966), or in other surveys of small areas and a more recent study across large areas of eastern Australia (Table 1). These findings suggest that Australian deer are not carriers of BoHV-1, despite the moderate prevalence levels in livestock and the extensive sharing of pastoral habitats by cattle and wild deer across Australia (Gu and Kirkland 2008). Multiple deer species have been experimentally infected with BoHV-1 (Chow and Davis 1964; Mollema et al. 2005). Bovine Herpesvirus has also been detected serologically in deer in Poland (<5% prevalence) and Hungary (12–47% prevalence via PCR detection and subsequent sequencing) (Kalman and Egyed 2005; Fabisiak et al. 2018). All studies involving Australian deer were conducted using serological tests, and it may be that this detection method is less sensitive than PCR, but further work is needed to confirm this.
Pathogen | Sample size | Prevalence (%) | Deer species | Diagnostic method | State or territory | Reference |
---|---|---|---|---|---|---|
Akabane virus | 396 | 13.0 | Red | VNT | Qld | McKenzie et al. (1985) |
ND | ND | Rusa | ND (antibodies) | NSW | Moriarty (2004b) | |
10 | 0.0 | Fallow | PCR | ACT | Huaman et al. (2020) | |
60 | 0.0 | Fallow | NSW | |||
7 | 0.0 | Rusa | ||||
43 | 0.0 | Chital | Qld | |||
2 | 0.0 | Fallow | Vic. | |||
22 | 0.0 | Sambar | ||||
Bovine Ephemeral Fever Virus | 432 | 43.0 | Red | VNT | Qld | McKenzie et al. (1985) |
ND | ND | Rusa | ND (antibodies) | NSW | Moriarty (2004b) | |
10 | 0.0 | Fallow | PCR | ACT | Huaman et al. (2020) | |
60 | 0.0 | Fallow | NSW | |||
7 | 0.0 | Rusa | ||||
43 | 0.0 | Chital | Qld | |||
2 | 0.0 | Fallow | Vic. | |||
22 | 0.0 | Sambar | ||||
Bopivirus | 59 | 5.0 | Fallow | PCR | NSW | Huaman et al. (2021b) |
3 | 0.0 | Sambar | ||||
6 | 1.0 | Red | ||||
12 | 1.0 | Fallow | Vic. | |||
11 | 0.0 | Sambar | ||||
0 | 0.0 | Red | ||||
Bovine herpesvirus | 31 | 0.0 | Fallow | VNT | Tas. | Munday (1966, 1972) |
ND | 0.0 | Fallow, rusa | ND (antibodies) | Vic. | Presidente and Westbury (1979) | |
86 | 0.0 | Fallow | VNT | NSW | English (1982) | |
405 | 0.0 | Red | VNT | Qld | McKenzie et al. (1985) | |
34 | 0.0 | Fallow | ELISA | ACT | Huaman et al. (2020) | |
164 | 0.0 | Fallow | NSW | |||
80 | 0.0 | Rusa | ||||
110 | 0.0 | Chital | Qld | |||
2 | 0.0 | Fallow | Vic. | |||
42 | 0.0 | Sambar | ||||
Bluetongue | 396 | 13.0 | Red | VNT | Qld | McKenzie et al. (1985) |
Epizoootic Hemorrhagic Disease Virus | 432 | 19.0–50.0 | Red | VNT | Qld | McKenzie et al. (1985) |
10 | 0.0 | Fallow | PCR | ACT | Huaman et al. (2020) | |
60 | 0.0 | Fallow | NSW | |||
7 | 0.0 | Rusa | ||||
43 | 0.0 | Chital | Qld | |||
2 | 0.0 | Fallow | Vic. | |||
22 | 0.0 | Sambar | ||||
Palyam group | 432 | 86.0 | Red | VNT | Qld | McKenzie et al. (1985) |
Parainfluenza-3 | 10 | 0.0 | Fallow | VNT | Tas. | Munday (1972) |
Pestivirus | 76 | 14.5 | Fallow | VNT | Tas. | Munday (1966, 1972) |
ND | 1.0 | Sambar | VNT | Vic. | Slee and Presidente (1981) | |
86 | 1.2 | Fallow | VNT | NSW | English (1982) | |
405 | 4.0 | Red | VNT | Qld | McKenzie et al. (1985) | |
34 | 7.7 | Fallow | ELISA | ACT | Huaman et al. (2020) | |
164 | 6.1 | Fallow | NSW | |||
80 | 2.5 | Rusa | ||||
110 | 0.0 | Chital | Qld | |||
2 | 0.0 | Fallow | Vic. | |||
42 | 0.0 | Sambar | ||||
Picobirnavirus | 60 | 53.3 | Fallow | PCR | NSW | Huaman et al. (2021c) |
11 | 36.4 | Sambar | Vic. | |||
Other arboviruses | ND | ND | Fallow, rusa, sambar | VNT | Vic., NSW | English (1982), Presidente and Westbury (1979), Slee and Presidente (1981) |
ND, no data; Qld, Queensland; NSW, New South Wales; ACT, Australian Capital Territory; Vic., Victoria; Tas., Tasmania; VNT, Virus neutralisation test; NGS, next-generation sequencing; ELISA, Enzyme-Linked Immunosorbent Assay.
Pestivirus infection is widespread in Australian cattle, causing the clinical disease Bovine Viral Diarrhoea (Reichel 2000; Scharnbock et al. 2018). Studies of Pestivirus exposure in deer species in Australia have been conducted across small geographical areas, with more recent studies encompassing larger land areas (Table 1). All these studies have found variable but low prevalence. Seropositive fallow deer were first reported in a small study in Tasmania with a prevalence of 14.5% (11/76 deer) (Munday 1966), and a decade later in another small study in NSW with a prevalence of 1.2% (1/86) (English 1982).
A larger study assessing Pestivirus prevalence in red deer sourced from 20 localities in eastern Australia in 1985 reported 4% prevalence (McKenzie et al. 1985). Recently, a study sourcing five deer species from locations in eastern Australia reported a similar prevalence of 3% (13/432) for Pestivirus antibodies (Huaman et al. 2020). These findings are consistent with the low seroprevalence of deer species globally for Pestivirus (reviewed in Passler et al. 2016). In addition, Australian cattle exhibit high seropositive rates (53%) (Scharnbock et al. 2018), but deer experimentally infected with Pestivirus have a low ability to shed the virus or display clinical symptoms (reviewed in (Passler et al. 2016)). Therefore, deer seem to be a spill-over end-host for Pestivirus and seldom able to transmit the virus to cattle.
Many significant viral infections of Australian livestock are carried by mosquitoes, termed arboviruses. Because arboviruses are vector-borne viruses, their occurrence is mainly driven by the effects of temperature and rainfall. There are distinct seasonal geographical changes in the incidence of important livestock-associated arboviruses (Geoghegan et al. 2014). Four agriculturally significant arboviruses have been detected in Australian deer: Bluetongue virus; Akabane virus; BEFV (Bovine Ephemeral Fever Virus); and EHDV (Epizoootic Hemorrhagic Disease Virus) (Table 1). Most of these observations have come from one large study of wild deer conducted across Queensland in 1985, in which 43% of deer were seropositive for BEFV, 19−50% seropositive for one of five strains of EHDV, and 13% seropositive for Akabane virus (McKenzie et al. 1985). Similar results were reported in a small study conducted in NSW in 2004, with rusa deer seropositive for Akabane virus and EHDV. More recently, a large-scale PCR-based screening study of deer blood samples collected from eastern Australia revealed no infection for a range of vector-borne diseases including BEFV, EHDV and Akabane (Huaman et al. 2020, 2021a). However, considering (1) endemicity of these pathogens in eastern Australia, (2) the presence of suitable vectors, and (3) expected increases in the distribution and abundance of deer (in the absence of substantial control efforts) (Davis et al. 2016; Cunningham et al. 2022), the possibility that deer species could be a future source of infection of these pathogens cannot be ruled out.
Using next-generation sequencing (NGS), three recent studies identified and characterised novel viruses in deer serum, plasma, and faecal samples (Huaman et al. 2021b, 2021c, 2022a). These studies illustrate how novel molecular techniques can change our understanding of viral epidemiology and evolution. Picobirnaviruses (PBVs) were detected in serum and plasma from fallow, rusa, and sambar deer (Huaman et al. 2021c), with subsequent molecular screening of a range of specimens collected from wild deer and of faecal samples from farmed cattle. PBVs have been detected in several animal species worldwide, mostly in faecal samples but also in blood and respiratory tract samples (Smits et al. 2011; Malik et al. 2014). High prevalence of PBVs were recorded in deer and cattle sampled in south-eastern Australia (Huaman et al. 2021c), with a predominance of genogroup I and simultaneous genogroups I and II detection in these host species. Moreover, the detection of identical sequences in the trachea and nasal swabs of the same animal, with no amplification in the lung, suggests active replication and infection of PBVs in the upper respiratory tract, expanding our knowledge of picobirnavirus tropism.
Genomic and phylogenetic analyses of NGS data revealed the presence of a new member of the genus Bopivirus, proposed as ‘Bopivirus C’ (Huaman et al. 2021b), in samples from south-eastern Australia. Further epidemiological investigation showed a prevalence of 8.5% in fallow deer, 16.7% in red deer and 0% in sambar deer and cattle. Phylogenetic and sequence analyses of the positive samples indicated that the same genotype is circulating. To our knowledge, this study reports for the first time a deer-origin bopivirus and the presence of a member of the genus Bopivirus in Australia.
A nearly complete genome of an endogenous betaretrovirus was characterised for the first time in fallow deer (Huaman et al. 2022a). Further genomic analysis showed that this provirus, tentatively named cervid endogenous betaretrovirus 1 (CERV β1), has typical betaretroviral genome features (gag-pro-pol-env). In addition, CERV β1 pol sequences were detected by PCR in wild populations of all the six non-native deer species in Australia. Phylogenetic analyses suggested that CERV β1 endogenisation occurred between 3.3 and 5 million years ago (Huaman et al. 2022a). Although this provirus does not appear to constitute a current risk for livestock, these results provide important insights into the evolution of betaretroviruses in cervids.
Parasitic diseases in deer
Unlike viral infections, research into parasitic infections on Australian deer populations was mostly conducted in the last 20 years, the majority of which was reviewed in Cripps et al. (2019). The research has focused on detecting gastrointestinal parasites and helminths. However, exposure to Sarcocystis, Entamoeba, and Neospora caninum and four vector-borne parasites (Babesia, Theileria, Trypanosoma, and Plasmodium) was recently assessed in PCR-based assays in wild deer from eastern Australia (Table 2).
Pathogen (genera or species) | Sample size | Prevalence (%) | Deer species | Diagnostic method | State or territory | Reference |
---|---|---|---|---|---|---|
Babesia, Plasmodium, Theileria, Trypanosoma, Sarcosytis | 31 | 0.0 | Fallow | PCR | ACT | Huaman et al. (2021a) |
105 | 0.0 | Fallow | NSW | |||
63 | 0.0 | Rusa | ||||
2 | 0.0 | Fallow | Vic. | |||
38 | 0.0 | Sambar | ||||
4 | 0.0 | Chital | Qld | |||
Babesia bovis | 105 | 0.0 | Chital | IFAT | Qld | |
Cryptosporidium | 700-950 | 1.0−9.0 | Fallow, red, sambar | PCR | Vic. | Cinque et al. (2008), Koehler et al. (2016), Nolan et al. (2013) |
137 | 0.7 | ND | PCR | NSW | Ng et al. (2011) | |
Entamoeba bovis | 48 | 72.9 | Fallow | PCR | NSW | Huaman et al. (2022b) |
12 | 100.0 | Vic. | ||||
11 | 100.0 | Sambar | ||||
Fasciola hepatica | 15 | 1.0 | Red | M.E | Qld | McKenzie et al. (1985) |
17 | 53.0 | Fallow | M.E | NSW | Jenkins et al. (2020) | |
1 | 0.0 | Fallow | M.E | ACT | ||
1 | 0.0 | Sambar | M.E | Vic. | ||
79 | 45.0 | Fallow | M.E | NSW | Lamb et al. (2021) | |
ND | 15.0 | Hog | M.E | Vic. | Game Management Authority (2006) | |
Giardia | 137 | 21.2 | ND | PCR | NSW | Ng et al. (2011) |
950 | 0.0−14.0 | Fallow, red, and sambar | PCR | Vic. | Koehler et al. (2016), Nolan et al. (2013) | |
Neospora caninum | 76 | 1.3 | Fallow | ELISA | NSW | Huaman et al. (unpubl. data) |
6 | 0.0 | Red | ||||
3 | 0.0 | Sambar | ||||
21 | 0.0 | Fallow | Vic. | |||
8 | 0.0 | Red | ||||
75 | 8.0 | Sambar | ||||
Toxoplasma | 74 | 0.0 | Fallow | IFAT | Tas. | Munday (1972) |
Other gastrointestinal helminths | 22 | 2.0−18.0 | Red | M.E | Qld | McKenzie et al. (1985) |
ND | ND | Fallow, rusa | M.EI | NSW | Moriarty (2004b), Munday (1972), Mylrea et al. (1991) | |
312 | 15.0-60.0 | Fallow, sambar, hog | M.E, PCR, NGS | Vic. | Davies (2014), Panozzo (2018) |
ND, no data; Qld, Queensland; NSW, New South Wales; ACT, Australian Capital Territory; Vic., Victoria; Tas., Tasmania; VNT, Virus neutralisation test; IFAT, Immunofluorescence antibody test; M.E, microscopic examination; NGS, next-generation sequencing.
PCR-based screening of deer serum and blood samples detected no active infection (i.e. where the pathogen replicates) of Sarcocystis and a range of vector-borne infections relevant for the livestock industry (Huaman et al. 2020, 2021a). Despite this non-detection, it remains possible that deer could become infected with these parasites (and act as a source of spill-back infection) in the future, given their endemicity in livestock, the presence of suitable vectors, and the ongoing spread of deer (Davis et al. 2016). This survey represents the first large-scale molecular study of its type in Australian deer and provides important baseline information about the infection status of wild deer in eastern Australia.
Following a similar research project detecting N. caninum parasites in wild dogs (Canis familiaris) (Davidson et al. 2022), and given the current gaps in knowledge about the sylvatic life cycle of this parasite, the seroprevalence of N. caninum was investigated in wild deer (unpublished data). Using a competitive ELISA (cELISA) test, a seroprevalence of 4% was detected in deer serum samples from fallow, red, and sambar deer collected in south-eastern Australia. These results suggest that wild deer contribute to the sylvatic cycle of this parasite in Australia. To our knowledge, this is the first reported detection of N. caninum antibodies in Australian wild deer and highlights the usefulness of cELISA for assessing serological assays in wildlife populations.
The intestinal parasite Entamoeba bovis has been previously detected in farm and wild ruminants worldwide, but its epidemiology and distribution in wild ruminants remain largely unexplored (Stensvold et al. 2010). E. bovis has also been detected in Australia, including in feral goats (Capra hircus) from Western Australia (Al-Habsi et al. 2017) and wild deer from eastern Australia (Huaman et al. 2022b). The cross-species infection between deer and livestock species was evaluated by estimating the time to a most recent common ancestor (TMCRA) of E. bovis sequences of wild deer and cattle origin (Huaman et al. 2022b). The TMCRA in this study was estimated to be >200 years ago (i.e. before cattle and deer were introduced to Australia), providing no evidence of E. bovis transmission between wild deer and cattle in Australia. This finding is surprising, but it is possible that wild deer populations have until recently been at a too-low density to play an important role in the transmission of these parasites.
Panozzo (2018) focused on interspecific transmission of gastro-intestinal parasites between livestock and wild deer (C. unicolor, D. dama and A. porcinus). She showed that the prevalence of parasites typically associated with cattle (e.g. Monieza spp.) was significantly higher in deer samples collected close to farmland, suggesting (but not proving) inter-species transmission. This study reported a high number of parasite species from deer samples that are relevant to the livestock industry and established that about a quarter of deer samples were positive for Fasciola hepatica and gastro-intestinal parasites, including O. ostertagi, O. leptospicularis, Haemonchus spp., O. radiatum, Trichostrongylus spp., and C. oncophora. The high prevalence of F. hepatica is consistent with the findings of Jenkins et al. (2020) and Lamb et al. (2021). Both studies focused on identifying the risk that deer pose to maintaining the infection of F. hepatica in livestock and established that approximately 50% of the samples were positive for this important parasite. These results led Jenkins et al. (2020) to conclude that deer are likely to represent a major source of environmental contamination. The presence of these parasites reduces livestock growth rates, which delays animals reaching slaughter weight (Roeber et al. 2013; Mazeri et al. 2017).
Current and future directions
This review suggests a low risk of transmission from wild deer to livestock in Australia. However, in Europe and North America wild deer were considered an important source of infection for livestock, and several publications focused on their local consequences and implications (Böhm et al. 2007; Conner et al. 2008; Martin et al. 2011; Ruiz-Fons et al. 2014). This discrepancy likely reflects differences between Australia and Europe/North America in climate, farming practices, and potential wildlife hosts. Changes in weather patterns in Australia due to climate change can alter the distributions, movements, and dispersal rates of invertebrate vectors (Bryan et al. 1996). Climate change could also influence the distributions and abundances of wildlife hosts. Thus, climate patterns can significantly impact pathogen transmission and interactions between wildlife hosts, vectors, and humans. Indeed, climate change is increasingly recognised as playing a major role in the emergence of vector-borne diseases in humans and wildlife (Fouque and Reeder 2019; Rocklov and Dubrow 2020). Therefore, long-term changes, especially warming temperatures, could significantly alter the distribution and prevalence of vector-borne diseases in wildlife populations (Fouque and Reeder 2019).
Climate change may pose a similar risk when considering the distribution of deer, and the potential for the spread of wild deer to new parts of Australia could result in new epidemiological challenges. The social behaviour of hosts is also likely to be an important factor influencing the epidemiology of some pathogens. Each of the six deer species in Australia congregates at preferred feeding areas, increasing the probability of disease spread among individuals. Male deer may further contribute to the risk of disease transmission through contact with multiple females during the breeding season.
Advances in high-throughput sequencing and bioinformatics have greatly increased our understanding and capacity to identify novel microorganisms. For example, in the last decade, a novel virus (Schmallenberg virus – SBV) was described in European cattle, constituting an emerging threat to the livestock industry in Europe and worldwide (Hoffmann et al. 2012; Endalew et al. 2019). Since its description in 2011, high seroprevalence of SBV has been detected in wild deer in Europe, indicating that deer could act as a source of this virus for livestock (Mouchantat et al. 2015; Garcia-Bocanegra et al. 2017; Jimenez-Ruiz et al. 2021). As mentioned above, next-generation sequencing was instrumental in identifying a novel Bopivirus in fallow and rusa deer from south-eastern Australia (Huaman et al. 2021b). The authors hypothesised that this virus, like other enteric picornaviruses, is transmitted via the faecal–oral route. However, further work is required to determine its distribution in other deer species (e.g. chital, rusa and hog deer), and livestock species other than cattle, to increase our understanding of the potential for cross-transmission between wild deer and livestock.
As discussed above, deer are infected by and susceptible to many diseases, some of which are zoonotic, meaning that they can also affect humans. Increasing the focus on wildlife disease surveillance to detect emerging infectious diseases and integrate wildlife and environmental health into One Health policies (Cunningham et al. 2017) is crucial to prepare Australia to better recognise and manage the adverse impacts of zoonotic diseases. Indeed, high wild deer densities (e.g. 39 per km2; Bengsen et al. 2022) may cause concern for human health via the transmission of infectious agents through direct contact, the consumption of venison, or contamination of the environment (particularly water) with faeces or urine.
Several zoonotic diseases occur in wild deer in Australia, including Q fever, leptospirosis, fasciolosis, cryptosporidiosis, and giardiasis. Recently, attention has turned to the potential role of deer as reservoirs of the SARS-CoV-2 virus, which causes COVID-19 in humans. There was high seroprevalence of SARS-CoV-2 in free-ranging white-tailed deer (Odocoileus virginianus) in the USA, revealing high susceptibility to infection of this deer species and active deer-to-deer transmission (Murphy and Ly 2021; Palmer et al. 2021; Hale et al. 2022). Moreover, the identification of SARS-CoV-2 variants suggests human-to-deer infections and that wild deer can sustain transmission (Hale et al. 2022). In contrast, no evidence of SARS-CoV-2 infection was found in common European deer species (Holding et al. 2022; Moreira-Soto et al. 2022). These studies indicate that deer should be considered when identifying potential reservoirs and intermediate hosts of emerging zoonotic diseases.
Finally, we recommend developing a passive surveillance system through the Australian deer hunter community. Deer hunters harvest large numbers of deer (Moloney et al. 2022). Training in identifying, recording macroscopic lesions, and collecting specimens could be provided to hunters as part of the licensing process, and hunters could help monitor the presence and distribution of infectious diseases. The investigations conducted in the last 5 years provide vital baseline data for future research. Monitoring changes in the disease status would offer a more comprehensive view of the dynamics of infectious diseases, which is important if the risks of those disease to humans, livestock, and wildlife are to be minimised. These findings extended our knowledge of known and novel viruses and parasites associated with Australian deer.
Data availability
This manuscript reviewed published literature; as such, no new data were generated in this study.
Conflicts of interest
David Forsyth and Anthony Pople were guest Associate Editors for this special issue. Despite this relationship, they did not at any stage have editor-level access to this manuscript while in peer review, as is the standard practice when handling manuscripts submitted by an editor to this journal. Wildlife Research encourages its editors to publish in the journal, and they are kept separate from their manuscripts’ decision-making process. The authors have no further conflicts of interest to declare.
References
Al-Habsi K, Yang R, Ryan U, Jacobson C, Miller DW (2017) Morphological and molecular characterization of an uninucleated cyst-producing Entamoeba spp. in captured Rangeland goats in Western Australia. Veterinary Parasitology 235, 41-46.
| Crossref | Google Scholar |
Barroso P, Acevedo P, Vicente J (2021) The importance of long-term studies on wildlife diseases and their interfaces with humans and domestic animals: a review. Transboundary and Emerging Diseases 68, 1895-1909.
| Crossref | Google Scholar |
Bengsen AJ, Forsyth DM, Pople A, Brennan M, Amos M, Leeson M, Cox TE, Gray B, Orgill O, Hampton JO, Crittle T, Haebich K (2022) Effectiveness and costs of helicopter-based shooting of deer. Wildlife Research
| Crossref | Google Scholar |
Bryan JH, Foley DH, Sutherst RW (1996) Malaria transmission and climate change in Australia. Medical Journal of Australia 164, 345-347.
| Crossref | Google Scholar |
Böhm M, White PCL, Chambers J, Smith L, Hutchings MR (2007) Wild deer as a source of infection for livestock and humans in the UK. The Veterinary Journal 174, 260-276.
| Crossref | Google Scholar |
Chow TL, Davis RW (1964) The susceptibility of mule deer to infectious bovine rhinotracheitis. American Journal of Veterinary Research 25, 518-519.
| Google Scholar |
Cinque K, Stevens MA, Haydon SR, Jex AR, Gasser RB, Campbell BE (2008) Investigating public health impacts of deer in a protected drinking water supply watershed. Water Science and Technology 58, 127-132.
| Crossref | Google Scholar |
Conner MM, Ebinger MR, Blanchong JA, Cross PC (2008) Infectious disease in cervids of North America: data, models, and management challenges. Annals of the New York Academy of Sciences 1134, 146-172.
| Crossref | Google Scholar |
Cripps JK, Pacioni C, Scroggie MP, Woolnough AP, Ramsey DS (2019) Introduced deer and their potential role in disease transmission to livestock in Australia. Mammal Review 49, 60-77.
| Crossref | Google Scholar |
Cunningham AA, Daszak P, Wood JLN (2017) One Health, emerging infectious diseases and wildlife: two decades of progress? Philosophical Transactions of the Royal Society B: Biological Sciences 372, 20160167.
| Crossref | Google Scholar |
Cunningham CX, Perry GLW, Bowman DMJS, Forsyth DM, Driessen MM, Appleby M, Brook BW, Hocking G, Buettel JC, French BJ, Hamer R, Bryant SL, Taylor M, Gardiner R, Proft K, Scoleri VP, Chiu-Werner A, Travers T, Thompson L, Guy T, Johnson CN (2022) Dynamics and predicted distribution of an irrupting ‘sleeper’ population: fallow deer in Tasmania. Biological Invasions 24, 1131-1147.
| Crossref | Google Scholar |
Davidson MJ, Huaman JL, Pacioni C, Stephens D, Hitchen Y, Carvalho TG (2022) Active shedding of Neospora caninum detected in Australian wild canids in a nonexperimental context. Transboundary and Emerging Diseases 69, 1862-1871.
| Crossref | Google Scholar |
Davis NE, Bennett A, Forsyth DM, Bowman DMJS, Lefroy EC, Wood SW, Woolnough AP, West P, Hampton JO, Johnson CN (2016) A systematic review of the impacts and management of introduced deer (family Cervidae) in Australia. Wildlife Research 43, 515-532.
| Crossref | Google Scholar |
Endalew AD, Faburay B, Wilson WC, Richt JA (2019) Schmallenberg disease – a newly emerged culicoides-borne viral disease of ruminants. Viruses 11, 1065.
| Crossref | Google Scholar |
English A (1982) Serological survey of wild fallow deer (Dama dama) in New South Wales, Australia. Veterinary Record 110, 153-154.
| Google Scholar |
Fabisiak M, Sałamaszyńska A, Stadejek T (2018) Detection of seroconversion to bovine herpesvirus 1 related alphaherpesvirus and bovine viral diarrhea virus in polish free-living deer. Polish Journal of Veterinary Sciences 21, 437-440.
| Crossref | Google Scholar |
Fouque F, Reeder JC (2019) Impact of past and on-going changes on climate and weather on vector-borne diseases transmission: a look at the evidence. Infectious Diseases of Poverty 8, 51.
| Crossref | Google Scholar |
Garcia-Bocanegra I, Cano-Terriza D, Vidal G, Rosell R, Paniagua J, Jimenez-Ruiz S, Exposito C, Rivero-Juarez A, Arenas A, Pujols J (2017) Monitoring of schmallenberg virus in spanish wild artiodactyls, 2006-2015. PLoS ONE 12, e0182212.
| Crossref | Google Scholar |
Geoghegan JL, Walker PJ, Duchemin J-B, Jeanne I, Holmes EC (2014) Seasonal drivers of the epidemiology of arthropod-borne viruses in Australia. PLoS Neglected Tropical Diseases 8, e3325.
| Crossref | Google Scholar |
Hale VL, Dennis PM, McBride DS, Nolting JM, Madden C, Huey D, Ehrlich M, Grieser J, Winston J, Lombardi D, Gibson S, Saif L, Killian ML, Lantz K, Tell RM, Torchetti M, Robbe-Austerman S, Nelson MI, Faith SA, Bowman AS (2022) SARS-CoV-2 infection in free-ranging white-tailed deer. Nature 602, 481-486.
| Crossref | Google Scholar |
Hall GP, Gill KP (2005) Management of wild deer in Australia. Journal of Wildlife Management 69, 837-844.
| Crossref | Google Scholar |
Hoffmann B, Scheuch M, Hoper D, Jungblut R, Holsteg M, Schirrmeier H, Eschbaumer M, Goller KV, Wernike K, Fischer M, Breithaupt A, Mettenleiter TC, Beer M (2012) Novel orthobunyavirus in cattle, Europe, 2011. Emerging Infectious Diseases 18, 469-472.
| Crossref | Google Scholar |
Holding M, Otter AD, Dowall S, Takumi K, Hicks B, Coleman T, Hemingway G, Royds M, Findlay-Wilson S, Curran-French M, Vipond R, Sprong H, Hewson R (2022) Screening of wild deer populations for exposure to SARS-CoV-2 in the United Kingdom, 2020–2021. Transboundary and Emerging Diseases 69, e3244-e3249.
| Crossref | Google Scholar |
Huaman JL, Pacioni C, Forsyth DM, Pople A, Hampton JO, Carvalho TG, Helbig KJ (2020) Serosurveillance and molecular investigation of wild deer in Australia reveals seroprevalence of pestivirus infection. Viruses 12, 752.
| Crossref | Google Scholar |
Huaman JL, Pacioni C, Forsyth DM, Pople A, Hampton JO, Helbig KJ, Carvalho TG (2021a) Evaluation of haemoparasite and Sarcocystis infections in Australian wild deer. International Journal for Parasitology: Parasites and Wildlife 15, 262-269.
| Crossref | Google Scholar |
Huaman JL, Pacioni C, Sarker S, Doyle M, Forsyth DM, Pople A, Carvalho TG, Helbig KJ (2021b) Novel picornavirus detected in wild deer: identification, genomic characterisation, and prevalence in Australia. Viruses 13, 2412.
| Crossref | Google Scholar |
Huaman JL, Pacioni C, Sarker S, Doyle M, Forsyth DM, Pople A, Hampton JO, Carvalho TG, Helbig KJ (2021c) Molecular epidemiology and characterization of picobirnavirus in wild deer and cattle from Australia: evidence of genogroup I and II in the upper respiratory tract. Viruses 13, 1492.
| Crossref | Google Scholar |
Huaman JL, Pacioni C, Forsyth DM, Pople A, Hampton JO, Carvalho TG, Helbig KJ (2022a) Detection and characterisation of an endogenous betaretrovirus in Australian wild deer. Viruses 14, 252.
| Crossref | Google Scholar |
Huaman JL, Pacioni C, Kenchington-Evans L, Doyle M, Helbig KJ, Carvalho TG (2022b) First evidence of Entamoeba parasites in Australian wild deer and assessment of transmission to cattle. Frontiers in Cellular and Infection Microbiology 12, 883031.
| Crossref | Google Scholar |
Jenkins DJ, Baker A, Porter M, Shamsi S, Barton DP (2020) Wild fallow deer (Dama dama) as definitive hosts of Fasciola hepatica (liver fluke) in alpine New South Wales. Australian Veterinary Journal 98, 546-549.
| Crossref | Google Scholar |
Jimenez-Ruiz S, Risalde MA, Acevedo P, Arnal MC, Gomez-Guillamon F, Prieto P, Gens MJ, Cano-Terriza D, Fernandez de Luco D, Vicente J, Garcia-Bocanegra I (2021) Serosurveillance of Schmallenberg virus in wild ruminants in Spain. Transboundary and Emerging Diseases 68, 347-354.
| Crossref | Google Scholar |
Kalman D, Egyed L (2005) PCR detection of bovine herpesviruses from nonbovine ruminants in hungary. Journal of Wildlife Diseases 41, 482-488.
| Crossref | Google Scholar |
Koehler AV, Haydon SR, Jex AR, Gasser RB (2016) Cryptosporidium and Giardia taxa in faecal samples from animals in catchments supplying the city of Melbourne with drinking water (2011 to 2015). Parasites & Vectors 9, 315.
| Crossref | Google Scholar |
Lamb J, Doyle E, Barwick J, Chambers M, Kahn L (2021) Prevalence and pathology of liver fluke (Fasciola hepatica) in fallow deer (Dama dama). Veterinary Parasitology 293, 109427.
| Crossref | Google Scholar |
Malik YS, Kumar N, Sharma K, Dhama K, Shabbir MZ, Ganesh B, Kobayashi N, Banyai K (2014) Epidemiology, phylogeny, and evolution of emerging enteric Picobirnaviruses of animal origin and their relationship to human strains. BioMed Research International 2014, 780752.
| Crossref | Google Scholar |
Martin C, Pastoret P-P, Brochier B, Humblet M-F, Saegerman C (2011) A survey of the transmission of infectious diseases/infections between wild and domestic ungulates in Europe. Veterinary Research 42, 70.
| Crossref | Google Scholar |
Mazeri S, Rydevik G, Handel I, Bronsvoort BMd, Sargison N (2017) Estimation of the impact of Fasciola hepatica infection on time taken for UK beef cattle to reach slaughter weight. Scientific Reports 7, 7319.
| Crossref | Google Scholar |
McClymont H, Bambrick H, Si X, Vardoulakis S, Hu W (2022) Future perspectives of emerging infectious diseases control: a One Health approach. One Health 14, 100371.
| Crossref | Google Scholar |
McKenzie RA, Green PE, Thornton AM, Chung YS, MacKenzie AR, Cybinski DH, George TDS (1985) Diseases of deer in south eastern Queensland. Australian Veterinary Journal 62, 424.
| Crossref | Google Scholar |
Miller RS, Farnsworth ML, Malmberg JL (2013) Diseases at the livestock-wildlife interface: status, challenges, and opportunities in the United States. Preventive Veterinary Medicine 110, 119-132.
| Crossref | Google Scholar |
Mollema L, Rijsewijk FAM, Nodelijk G, de Jong MCM (2005) Quantification of the transmission of bovine herpesvirus 1 among red deer (Cervus elaphus) under experimental conditions. Veterinary Microbiology 111, 25-34.
| Crossref | Google Scholar |
Moloney PD, Gormley AM, Toop SD, Flesch JS, Forsyth DM, Ramsey DSL, Hampton JO (2022) Bayesian modelling reveals differences in long-term trends in the harvest of native and introduced species by recreational hunters in Australia. Wildlife Research 49, 673-685.
| Crossref | Google Scholar |
Moreira-Soto A, Walzer C, Czirják GÁ, Richter MH, Marino SF, Posautz A, De Yebra Rodo P, McEwen GK, Drexler JF, Greenwood AD (2022) Serological evidence that SARS-CoV-2 has not emerged in deer in Germany or Austria during the COVID-19 pandemic. Microorganisms 10, 748.
| Crossref | Google Scholar |
Morgan ER, Lundervold M, Medley GF, Shaikenov BS, Torgerson PR, Milner-Gulland EJ (2006) Assessing risks of disease transmission between wildlife and livestock: the Saiga antelope as a case study. Biological Conservation 131, 244-254.
| Crossref | Google Scholar |
Moriarty A (2004a) The liberation, distribution, abundance and management of wild deer in Australia. Wildlife Research 31, 291-299.
| Crossref | Google Scholar |
Morner T, Obendorf DL, Artois M, Woodford MH (2002) Surveillance and monitoring of wildlife diseases. Revue Scientifique et Technique 21, 67-76.
| Crossref | Google Scholar |
Mouchantat S, Wernike K, Lutz W, Hoffmann B, Ulrich RG, Borner K, Wittstatt U, Beer M (2015) A broad spectrum screening of Schmallenberg virus antibodies in wildlife animals in Germany. Veterinary Research 46, 99.
| Crossref | Google Scholar |
Munday BL (1972) A serological study of some infectious diseases of Tasmanian wildlife. Journal of Wildlife Diseases 8, 169-175.
| Crossref | Google Scholar |
Murphy HL, Ly H (2021) Understanding the prevalence of SARS-CoV-2 (COVID-19) exposure in companion, captive, wild, and farmed animals. Virulence 12, 2777-2786.
| Crossref | Google Scholar |
Mylrea GE, Mulley RC, English AW (1991) Gastrointestinal helminthosis in fallow deer (Dama dama) and their response to treatment with anthelmintics. Australian Veterinary Journal 68, 74-75.
| Crossref | Google Scholar |
Ng J, Yang R, Whiffin V, Cox P, Ryan U (2011) Identification of zoonotic Cryptosporidium and Giardia genotypes infecting animals in Sydney’s water catchments. Experimental Parasitology 128, 138-144.
| Crossref | Google Scholar |
Nolan MJ, Jex AR, Koehler AV, Haydon SR, Stevens MA, Gasser RB (2013) Molecular-based investigation of Cryptosporidium and Giardia from animals in water catchments in southeastern Australia. Water Research 47, 1726-1740.
| Crossref | Google Scholar |
Palmer MV, Martins M, Falkenberg S, Buckley A, Caserta LC, Mitchell PK, Cassmann ED, Rollins A, Zylich NC, Renshaw RW, Guarino C, Wagner B, Lager K, Diel DG (2021) Susceptibility of white-tailed deer (Odocoileus virginianus) to SARS-CoV-2. Journal of Virology 95, e00083-00021.
| Crossref | Google Scholar |
Passler T, Ditchkoff SS, Walz PH (2016) Bovine viral diarrhea virus (BVDV) in white-tailed deer (Odocoileus virginianus). Frontiers in Microbiology 7, 945.
| Crossref | Google Scholar |
Reichel MP (2000) Neospora caninum infections in Australia and New Zealand. Australian Veterinary Journal 78, 258-261.
| Crossref | Google Scholar |
Rhyan JC, Spraker TR (2010) Emergence of diseases from wildlife reservoirs. Veterinary Pathology 47, 34-39.
| Crossref | Google Scholar |
Rocklov J, Dubrow R (2020) Climate change: an enduring challenge for vector-borne disease prevention and control. Nature Immunology 21, 479-483.
| Crossref | Google Scholar |
Roeber F, Jex AR, Gasser RB (2013) Impact of gastrointestinal parasitic nematodes of sheep, and the role of advanced molecular tools for exploring epidemiology and drug resistance – an Australian perspective. Parasites & Vectors 6, 153.
| Crossref | Google Scholar |
Ruiz-Fons F, Sanchez-Matamoros A, Gortazar C, Sanchez-Vizcaino JM (2014) The role of wildlife in bluetongue virus maintenance in Europe: lessons learned after the natural infection in Spain. Virus Research 182, 50-58.
| Crossref | Google Scholar |
Scharnbock B, Roch F-F, Richter V, Funke C, Firth CL, Obritzhauser W, Baumgartner W, Kasbohrer A, Pinior B (2018) A meta-analysis of bovine viral diarrhoea virus (BVDV) prevalences in the global cattle population. Scientific Reports 8, 14420.
| Crossref | Google Scholar |
Slee KJ, Presidente PJA (1981) Biological and pathological features of sambar in Victoria. Part 1. Haematology, Biochemistry and Serology Australian Deer 6, 7-14.
| Google Scholar |
Smits SL, Poon LLM, van Leeuwen M, Lau P-N, Perera HKK, Peiris JSM, Simon JH, Osterhaus ADME (2011) Genogroup I and II picobirnaviruses in respiratory tracts of pigs. Emerging Infectious Diseases 17, 2328-2330.
| Crossref | Google Scholar |
Stensvold CR, Lebbad M, Clark CG (2010) Genetic characterisation of uninucleated cyst-producing Entamoeba spp. from ruminants. International Journal for Parasitology 40, 775-778.
| Crossref | Google Scholar |
Walker JG, Plein M, Morgan ER, Vesk PA (2017) Uncertain links in host–parasite networks: lessons for parasite transmission in a multi-host system. Philosophical Transactions of the Royal Society B: Biological Sciences 372, 20160095.
| Crossref | Google Scholar |
Woolhouse MEJ, Dye C, Cleaveland S, Laurenson MK, Taylor LH (2001) Diseases of humans and their domestic mammals: pathogen characteristics, host range and the risk of emergence. Philosophical Transactions of the Royal Society of London. Series B: Biological Sciences 356, 991-999.
| Crossref | Google Scholar |