Feral cat control: improving Eradicat® bait efficiency and effectiveness for fauna conservation in the Southern Jarrah Forest, Western Australia
Adrian F. Wayne
A
Abstract
Toxic meat baits are the most effective broadscale method used for reducing the densities and impacts of feral cats (Felis catus) on vulnerable Australian native fauna when alternative prey is minimal.
Our aim was to assess the efficiency (proportion of baits removed by target animals) and effectiveness (proportion of target animals removed) of Eradicat® baits and to identify how their use may be improved in Southern Jarrah Forest ecosystems of Western Australia. We sought to determine how, when, and where best to deploy baits using the current Eradicat® bait prescriptions to maximise the reduction of feral cats.
Eradicat® uptake trials were conducted over a 15-month period using remote sensor cameras (RSCs) to observe animals interacting with the baits at 40 sites. Ten successive baiting trials were conducted, each involving four randomly selected sites (two replicates for each of two bait deployment methods: clusters and transects).
The fate of 5658 Eradicat® baits at 2000 bait locations was recorded during 54,361 camera trap nights. Despite occupancy rates being high for cat and fox (Vulpes vulpes), (92% and 84%, respectively), the efficiency and effectiveness of Eradicat® baits was low for both introduced predators (cat: 0.1% and 10–12%, respectively; and fox: <0.6% and 8–20%, respectively). There were no major differences in baiting efficiency in relation to bait deployment method or time of year. More than half (56–58%) of the baits were removed by non-target animals prior to an introduced predator being observed on camera at the bait location. Along transects, there were more cat and fox visits closer to tracks and surface water features. Foxes were also more likely to visit bait locations closer to private property. Younger cats appeared to be more interested and more likely to eat a bait than older cats.
Substantial improvements could be made by increasing bait availability (reducing non-target interference), detectability and attractiveness to cats.
Additional introduced predator threat abatement methods may be needed for the conservation and recovery of many threatened native mammals in the Southern Jarrah Forests and elsewhere in Australia. Feral cat baiting should be conducted within an integrated and holistic invasive animal management system.
Keywords: 1080, bait, control, feral cat, introduced predator, invasive species, sodium fluoroacetate, threatened species.
Introduction
Introduced predators, particularly feral cats (Felis catus), are considered the principal driver in most of Australia’s mammal extinctions and ongoing declines since settlement by Europeans (e.g. Abbott et al. 2014; Woinarski et al. 2014; Doherty et al. 2017; Radford et al. 2018; Woinarski et al. 2019). Effective mitigation of the impact of introduced predators is, therefore, critical to the conservation of much of Australia’s native terrestrial vertebrate fauna.
Toxic meat baits have been the most extensive and effective broadscale method for reducing the densities and impacts of the introduced red fox (Vulpes vulpes) on vulnerable Australian native fauna (Orell 2004; Reddiex et al. 2006; Saunders and McLeod 2007; Robley et al. 2014; Marlow et al. 2015a). The toxin used (sodium fluoroacetate, FCH2CO2Na, branded ‘1080’) is particularly effective for targeting introduced vertebrates in southwestern Australia where the native fauna has a naturally evolved tolerance (e.g. Twigg et al. 2003).
While broadscale control of feral cats has been more challenging than fox control, baiting is used extensively (Woinarski et al. 2019; Lohr and Algar 2020), being considered the most effective broadscale method when alternative prey is minimal (Christensen et al. 2013; Moseby et al. 2021). The Eradicat® bait (4.5 mg 1080 in 70% kangaroo meat; 20% chicken fat; 10% digest and flavour enhancers; 20 g wet weight dried to 15 g) is a toxic meat sausage designed to target feral cats (Algar and Burrows 2004; Algar et al. 2007). The average efficacy of Eradicat® to control feral cat numbers across several sites has been estimated as 55% (34–77%) of cats killed (Garrard et al. 2017) (not including the results of Short et al. (1997) which included other bait types), but have been reported as high as 96% (Algar and Burrows 2004) and 100% (Moseby et al. 2021). However, baiting has not always been effective and/or consistent (e.g. Moseby and Hill 2011; Comer et al. 2018; Lohr and Algar 2020; Doherty et al. 2022). The timing and environmental conditions of Eradicat® deployment can be particularly important in optimising its effectiveness to coincide with the time when cats are more hungry and motivated to eat baits (Algar and Burrows 2004; Algar et al. 2007; Christensen et al. 2013). In the rangelands of Western Australia, baiting has been most effective during winter when conditions are cool and dry. Prey, such as reptiles and small mammals, are relatively scarce in winter and the milder conditions reduce the rates of bait degradation due to moisture, ants and hot temperatures (Algar and Burrows 2004). In areas with a Mediterranean-type climate and where rabbits are the primary prey, the optimum baiting may be in autumn/winter before the winter rains and young prey become abundant (Algar et al. 2007), or where rabbit abundance is low (Moseby et al. 2021).
The Western Australian Government Department of Biodiversity, Conservation and Attractions (DBCA) is aiming to integrate Eradicat® into the existing broadscale fox baiting conducted as part of the Western Shield program to assist with the management of feral cat numbers within DBCA managed lands. Western Shield aims to recover and sustain wild populations of native fauna threatened by foxes and feral cats principally through the control of introduced predators and the reintroduction of native species through translocations (Department of Parks and Wildlife 2017). A co-ordinated and integrated control of the feral cat and red fox is also necessary to reduce the risk of a mesopredator release of feral cats in areas where foxes are effectively controlled (e.g. Wysong 2016; Molsher et al. 2017).
The jarrah (Eucalyptus marginata) forest in southwestern Australia is within a global terrestrial biodiversity hotspot (Myers et al. 2000). It supports some of the largest remnant indigenous populations of native fauna that previously had distributions across much of Australia, but since the introduction of the cat and red fox, have substantially contracted (National Land and Water Resources Audit 2002). These species include the critically endangered woylie (Bettongia penicillata) and ngwayir (western ringtail possum, Pseudocheirus occidentalis), endangered numbat (Myrmecobius fasciatus), vulnerable chuditch (Dasyurus geoffroii) and quokka (Setonix brachyurus), and other conservation priority species also listed under the Western Australian Wildlife Conservation Act 1950, such as quenda (Isoodon fusciventor), wambenger (Phascogale tapoatafa wambenger), tammar wallaby (Notamacropus eugenii) and western brush wallaby (Notamacropus irma), among other species. Some of these species are considered important ecosystem engineers and keystone species. Their populations help to maintain the health of the jarrah forest and its resilience to withstand pressures such as climate change, fire and other anthropogenic disturbances (e.g. Fleming et al. 2014).
The Upper Warren area within the Southern Jarrah Forest (SJF), constitutes ‘the jewel in the crown’ for mammal conservation in southwestern Australia, supporting an abundance of threatened mammals and an almost complete suite of mammal species known to be present at the time of European settlement (Wayne and Moore 2011). Since the mid-1990s; however, seven native mammal species have undergone successive catastrophic declines resulting in the elevation of the conservation status of two species to Critically Endangered (woylie and ngwayir) (Wayne et al. 2017a, 2017b). Introduced predators (feral cats and foxes) are common and widespread throughout the region (Geary et al. 2023) and are considered the principal common driver to most of these declines (Wayne et al. 2015, 2017a, 2017b). Effective threat abatement of feral cats in the SJF is, therefore, important to the conservation of many threatened native species.
This study specifically explored how Eradicat® could be efficiently (proportion of baits removed by target animals) and effectively (proportion of target animals removed, assuming these bait removal events were lethal) used within the SJF to reduce the predation impact of feral cats, with the aim of delivering better wildlife conservation outcomes. We sought to determine how, when, and where to deploy Eradicat® to maximise the reduction of feral cats and minimise the potential risks to non-target native species. With a focus on the baits and target species’ behaviour around the baits, the specific research questions investigated here (Fig. 1) were: does (1) Eradicat® deployment factors (method/spatial-arrangement, time of year and habitat type) at the site level; and (2) landscape features (proximity to vehicle tracks, surface water features or private property) at the bait location level, affect:
introduced predator visits at bait locations,
Eradicat® availability when bait locations are visited by introduced predators, and
Eradicat® consumption rates by introduced predators?
And finally, how do introduced predators interact with the Eradicat® baits? Specifically, do they detect baits? And, if so, how do they respond?
An illustrative overview of this study’s objective to determine how, when, and where to deploy Eradicat® baits that would maximise the reduction of feral cats. The specific research questions investigated relate to how (1) deployment factors at the site level and (2) proximity to landscape features at bait locations affected (i) introduced predator visits at bait locations, (ii) bait availability when bait locations are visited by introduced predators, and (iii) bait consumption rates by introduced predators. Information relating to the statistical tests used are provided in square brackets.
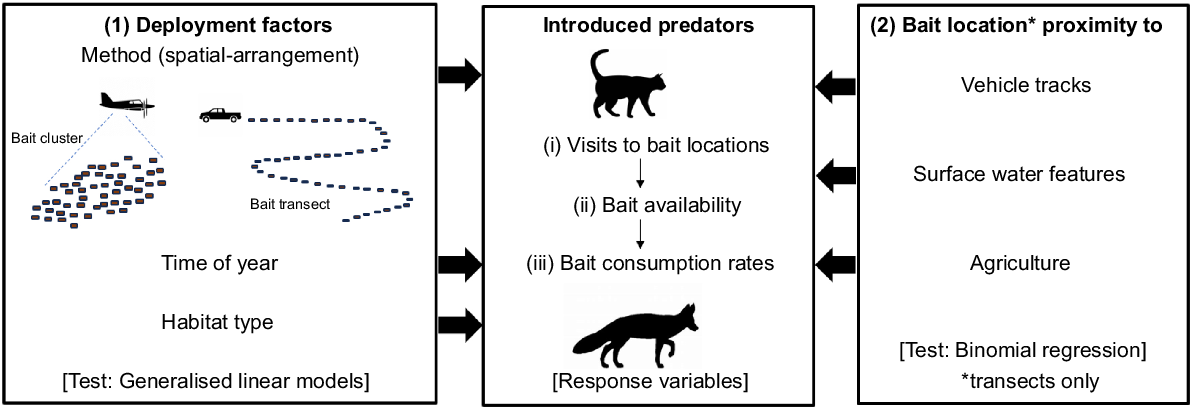
Materials and methods
This study is the first step to investigating the suitability of using Eradicat® baits in the SJF (see Supplementary material 1 for details). This study was not an assessment of an operational-scale deployment of Eradicat®. Rather, this was a research program at a precautionary, smaller, and less intensive scale to inform how Eradicat® might best be used safely at an operational scale in this ecosystem.
Study area
This study focused on the core area (456,000 ha) of SJF managed by DBCA. The north-west extent (115.8°E, 33.9°S) of the study was south of the Blackwood River and east the town of Nannup and the south-east extent (117.1°E, 34.7°S) was west of the Kent River, Western Australia (Figs 2 and 3).
Study sites
Eradicat® uptake trials were conducted over 15 months using remote sensor cameras (RSCs) to observe animals interacting with the baits. A stratified-random selection of study areas was used to comparatively test the effectiveness of Eradicat® baits according to bait deployment method and time of year. The study area was partitioned into 5 km × 5 km grid cells, and those cells with >75% of the area managed by DBCA with mostly SJF ecosystems that were subject to broad-scale fox baiting under the Western Shield program, were identified. To ensure spatial independence between treatments, every second cell in every second row was selected for inclusion. A small study site involving 50 bait locations (arranged either in a cluster to mimic a single aerial bait drop or along a transect; described below) was located as close to the centre of the selected cells as possible, to maximise spatial separation from other selected treatment sites (8.4 km mean minimum distance between bait/camera locations on nearest neighbour sites; s.d. = 1.5, range = 5.3–11.8 km) (Fig. 2). Note that 5 km spatial separation between cat bait treatments has been used in other studies (Lohr and Algar 2020 and references within); however, long-distance movements by feral cats can occur (e.g. Jansen et al. 2021). Table 1 summarises how, in each of 10 successive sessions, four randomly assigned sites provided two replicates of the two Eradicat® deployment methods (cluster and transect).
Session | Period of camera and bait deployment | Cluster 1 | Cluster 2 | Transect 1 | Transect 2 | |
---|---|---|---|---|---|---|
1 | October–November 2016 | 39 | 23 | 8 | 6 | |
2 | November–December 2016 | 16 | 11 | 19 | 9 | |
3 | January–February 2017 | 29 | 4 | 20 | 17 | |
4 | February–March 2017 | 38 | 10 | 31 | 12 | |
5 | March–April 2017 | 26 | 21 | 40 | 5 | |
6 | May–June 2017 | 7 | 33 | 24 | 36 | |
7 | June–July 2017 | 18 | 32 | 2 | 37 | |
8 | July–August 2017 | 14 | 28 | 22 | 1 | |
9 | September–October 2017 | 15 | 35 | 25 | 27 | |
10 | October–November 2017 | 30 | 3 | 13 | 34 |
Note each deployment method had two replicates per session.
Deployment method and site layouts
The deployment of Eradicat® baits in clusters or along transects (regarded henceforth as the two ‘treatments’) was done in accordance with the government prescription (Department of Parks and Wildlife 2015) and approval conditions, except with respect to the placement of baits along transects in relation to prevailing winds (see below). Sites with a cluster involved a 200 m × 40 m plot, >100 m from trafficable tracks and >250 m from main public roads, to comply with directions of use for aerial 1080 baiting (Department of Agriculture and Food Western Australia 2016). Each cluster site had 50 bait stations roughly evenly spaced throughout the plot to resemble the expected bait scatter pattern had an aircraft deployed the baits according to prescription. Note that the spatial separation between cluster sites in this study was much greater than a large-scale operational deployment of Eradicat® whereby 50 baits are deployed every square kilometre. This difference in the density of baits between this study and a large-scale operational baiting event affects how the results for the introduced predators can be interpreted. For example, the likelihood of detecting a bait consumption by an introduced predator during the trials may be higher than if the baits were deployed at operational densities, because individuals in these trials had no opportunity for a lethal encounter with an Eradicat® bait elsewhere within 5–10 km of the study site. The scale of the baiting trials is expected to have no or negligible effect on non-target removal of baits given that most native non-target consumers of baits have home ranges that are smaller than the 1 km spacing between clusters, and they are unlikely to die from the baits they consume because of their higher natural tolerances to 1080 (e.g. Twigg et al. 2003).
The transect treatment involved a 5 km section of an unsealed vehicle track that was closed to unauthorised vehicles during the trials at that site (~2 months) to reduce the risk to humans and their animal companions, and to reduce the risk of interference or theft of cameras. Tracks frequently used by vehicles were avoided to minimise the disruption caused by the temporary road closures. Bait stations were at 100 m intervals along the transect. The Parks and Wildlife Service, Western Australia 1080 Bait Risk Assessment and Approval process for the project stipulated that the baits be placed 5–20 m off track. The bait stations were also located to reduce the visibility of the associated RSC from the track. It was not practical nor meaningful to routinely locate baits on the prevailing windward side of the track (to improve detection by target species) as described in the baiting prescriptions (Department of Parks and Wildlife 2015). This was because wind directions in the SJF are highly variable both within and between days, and often there is no discernible breeze at ground level.
Bait stations
All intended bait locations had a dummy RSC (i.e. a camera case, with no internal parts, identical to the RSC models being used) deployed for at least four weeks prior to the start of the bait trials. This was to allow time for the wildlife to become accustomed to the presence of novel objects in their environment to minimise behavioural responses of animals to the RSCs during the trials (i.e. reduce the observer effect). A real RSC (a randomly selected Reconyx HC600 or PC900 model) replaced the dummy on the day of deployment of a toxic Eradicat® bait 1.5 m in front of the RSC and at the centre of the field of view (verified by use of test images taken by the RSC in position). The RSC set-up was informed by the experience of other researchers (e.g. Meek et al. 2012; Jansen et al. 2014) and refined during our pilot trial, which was designed to optimise the effectiveness of the RSCs to record wildlife and particularly animal interactions with the baits (Baraud 2016; A. Wayne unpubl. data). RSCs were oriented south between south-west and south-east and with the lens about 20–30 cm above ground depending on ground slope. The RSCs were concealed as much as practically possible to reduce detection (i.e. within or adjacent to existing natural structures such as vegetation, logs or debris) but adjacent to and focused on an open area in which the bait could be placed and monitored by the camera. Some selective light pruning of the vegetation in front of the camera and around the bait location was done where required to improve camera surveillance and standardise the minimum size of the field of view in front of camera. Test images were used to standardise and confirm the camera was centrally focused on the bait location 1.5 m in front of camera. The walk test function of the RSCs was used to improve the sensitivity of the cameras to detect animals around the bait.
RSC settings included 10 images per trigger, no time delay between triggers, high sensitivity, and motion sensor on. A total of 243 Reconyx RSCs (73 HC600 and 170 PC900 models) were used during the study (200 per trial). While the hardware specifications of the HC600 and PC900 are identical, wherever possible the priority was to use PC900 units, which have greater programming capabilities, enabling time lapse images to be taken while in motion sensor mode. The proportion of PC900 RSCs increased from ~50% to 75% during the trials. The HC600 units were spread evenly across the four treatment sites in each session. The PC900 models were initially programmed to take a time lapse image at 00:00 hours and 12:00 hours to record the status of the Eradicat® bait. The frequency of time lapse photos was doubled in Session 4–10 to include 06:00 hours and 18:00 hours to better discriminate the timing of unknown bait-removal events. The location of the RSC and bait were not visibly marked during the trials. GPS co-ordinates of each camera were recorded.
The Eradicat® baits were stored frozen and prepared immediately prior to deployment in accordance with DBCA prescriptions (Department of Parks and Wildlife 2015), including being sweated and sprayed with an ant deterrent compound (Coopex) at a concentration of 12.5 g L−1 prior to deployment. The amount of bait available during the trials was estimated from the imagery recorded by the RSCs and verified by observers, where possible, during bait replenishment visits (transects only) and at the end of the trails at each site. The amount of bait remaining after partial consumptions was estimated to the nearest decile.
Timing of Eradicat® uptake trials
Within each session the trials were conducted concurrently, except that the start of the baiting at each of the four sites (deployment of baits and replacement of dummy cameras with RSCs) was staggered over four (on one occasion five) successive days. To avoid differences between sites within a session the start of some sessions was delayed to avoid significant weather events, such as rainfall and storms, anticipated during the first 5 days. Each session ran for at least 25 days (except for the two cluster sites in the first session that ran for 20–21 days). Cluster sites received 50 Eradicat® baits deployed on a single day. The transects had baits replenished or replaced on four subsequent occasions at 4–7-day intervals and to a maximum of 50 baits in total per linear km, in accordance with the prescriptions (Department of Parks and Wildlife 2015).
Operational deployments of Pro-baits targeting foxes (3 mg 1080 in a sausage 70% kangaroo meat; 20% animal fat (pork or chicken); 10% canine ‘digest’ and hardening agent; about 80–85 g wet weight, heated and dried to about 40 g) (Marlow et al. 2015b), occurred throughout the study area during the trials. Generally, there was a substantial temporal separation at the site level between the deployment of Pro-baits and the Eradicat® trials. Pro-baits were deployed by air on average 59 days (1–171 days) prior to the Eradicat® trials commencing at each site, and Pro-baits were deployed along transects near some sites on average 104 days (4–243 days) prior to the Eradicat® trials. Some sites may have been influenced by the deployment of Pro-baits immediately prior to (within 10 days of the start of the Eradicat® trials at two sites) or during the Eradicat® trials (five sites; see Supplementary material 2). The frequency of fox visits was significantly lower on these sites during these periods compared to the other sites and periods (mean number of fox visits per site per day was 0.076 (s.e. = 0.0318) vs 0.145 (s.e. = 0.0164), respectively; P = 0.028, one tailed t-Test assuming unequal variances). Consequently, the fox models reported here omitted data for those sites affected by Pro-bait deployment activities either entirely (Sites 9 and 40) or partially (Sites 5, 21, 26, 30 and 34). Nonetheless, the results for the fox models did not fundamentally change with the removal of this data. There was no significant difference in the frequency of cat visits at the sites and periods potentially influenced by Pro-baits deployment compared to other sites and periods (mean number of cat visits per site per day was 0.115 (s.e. = 0.0372) vs 0.856 (s.e. = 0.0125), respectively; P = 0.225, one tailed t-Test assuming unequal variances). Additionally, because cat numbers are not expected to be significantly affected by Pro-baits (e.g. de Tores et al. 2011; Dundas et al. 2014), all data was used in the cat models.
Data management
All 40 sites were originally classified into eight habitat types, based on the Landscape Conservation Unit (LCU) classification system (scale resolution and positional accuracy 1:250,000), which is based on climate, landform, vegetation structure and vegetation composition (Mattiske and Havel 2002; Fig. 3). However, three LCUs were represented by one or two sites that were on or very close to the boundaries with adjacent LCUs and so were aggregated (sites 15 and 20 in ‘Frankland Unicup Muir Complex’ were aggregated with ‘Yornup Wilgarup Perup’ (YWP; n = 14), site 37 (Southern Karri) and site 40 (Redmond Siltstone Plain) were aggregated with ‘Southern Hilly Terrain’ (SHT; n = 7), and site 33 (Southern Karri) was aggregated into ‘South Eastern Upland’ (SEU; n = 4)). The other LCUs represented were ‘Northern Karri’ (NK; n = 9) and ‘Strachan Cattaminup Jigsaw’ (SCJ; n = 6).
For each RSC the distance to road (transect deployments only), orientation (compass bearing) and height above ground was recorded. The time of bait deployment and the type and number of ants visible within a metre of the bait was also recorded. The assessment of baits at subsequent site visits (i.e. rebaiting events for transects and at the completion of the trials at both cluster and transect sites), included presence/absence of the bait, sign of damage, bait proportion remaining and condition, whether the bait had moved and number and type of ants on the bait and within 1 m radius of the bait.
All images from the cameras were managed in the CPW Photo Warehouse database, Ver. 4 (Newkirk 2016). All distinguishable taxa on camera were recorded, as well as animal interactions with baits and bait status (e.g. estimates of the proportion of bait remaining, when the bait was moved or removed and by what species or the period in which the bait disappeared). Independent visits by species were defined as >60-min intervals between records of a given species or when individuals could be clearly distinguished. This conservative approach made no difference to the number of feral cat visits because individuals within the 60-min intervals were readily distinguished. It also made little difference to the number of fox visits (i.e. 154 vs 156, 156, and 163 events based on 60, 30, 10, and 2 min intervals respectively). Individuals (particularly cats) were distinguished where possible by multiple observers, based on distinctive features and variations in pelt patterns (e.g. Bengsen et al. 2011; Hohnen et al. 2013). Cat and fox behaviour recorded in the images were subjectively assessed in relation to the individual’s interaction with the RSC using a similar approach to Meek et al. (2016). Behaviour classifications were negative (e.g. avoidance, runs away), positive (curious, investigates camera), neutral (looks at camera but shows no overt signs of behaviour change), unaware (no evidence of camera detection), or indeterminate (not enough information to determine behaviour). When available, the animal’s interactions with the bait were also recorded: none, positive evidence of bait detection (i.e. looked at the bait) either >1 or ≤1 body length from the bait and then left, remote investigation of the bait either >1 or ≤1 body length from the bait but then left (e.g. prolonged looking and/or approach toward the bait without contacting the bait), contact with the bait but then left, ate the bait or took the bait away (out of the field of view).
Data analysis
The age and accumulated rainfall of the baits taken or not, were compared using a one-tailed t-Test (assuming unequal variances), given the a priori expectation that baits removed by cat or fox would be younger or drier. Given the low number of baits removed by both cats and foxes, logistic regression was unsuitable, so Fisher’s exact test was used to test treatment differences in bait efficiency (Vittinghoff et al. 2005).
The effects of treatment, session and LCU on Eradicat® baiting in the SJF was investigated using Generalised Linear Models (GLMs) on two response variables for both cats and foxes: number of visits (Ʃ independent cat or fox visits at the 50 locations where baits were deployed at a site) and baiting opportunities (i.e. when an introduced predator was present and a bait was available; Ʃ independent cat or fox visits where a whole or partial bait is present at a site). Due to the presence of slight over-dispersion (variance being greater than the mean), negative binomial models were fitted, and the number of survey days (RSC deployment period) at each site was used as an offset term. Seven models were used (all additive combinations of the three deployment factors included as factors singularly, as pairs and all three together, and not including interaction terms). Model selection was based on Akaike Information Criterion (AIC) whereby models with a difference in AIC from the best fitting model of less than 2 are considered equivalent (Burnham and Anderson 2002).
We used binomial regression models to investigate bait locations along transects only, in relation to proximity to vehicle tracks, surface water features (i.e. mostly ephemeral creeks and swamps) and private property with respect to two response variables for both cats and foxes (i) whether the bait location was visited, and (ii) when a cat or fox did visit a bait location, whether a bait was available (i.e. baiting opportunity). Bait locations with less than 10 trap nights (i.e. faulty cameras) were removed from the dataset (12 out of 1000 bait locations). The number of survey days at each bait location was included as a fixed effect to account for variation in sampling effort. The distances to each of the landscape features were scaled for comparative purposes. For (i) visits by a cat or fox, zero inflated binomial models were used with site (n = 20) included as a random effect. For (ii) baiting opportunities, standard binomial regression models were used with bait location included as a random effect. The best of seven models (combinations of the three landscape features) was selected using AIC.
Analyses were conducted in Program R (v. 4.1.0, R Core Team 2021) and procedures included ‘stats’, ‘MASS’, ‘pscl’, ‘AER’, emmeans’, ‘car’ and ‘rcompanion’ (https://cran.r-project.org/web/packages/).
Ethics approval
This study was conducted in compliance with the conditions of the DBCA Animal Ethics Committee (approval number 2016/04) and with the approval of the DBCA Feral Cat Baiting Technical Committee. It was conducted in accordance with the conditions specified by the DBCA 1080 Baiting Risk Assessment and Approval process (FEM 053 Approval numbers: BWD 5/2017, BWD6/2017, BWD7/2017, DON1/2017, DON 2/2017, DON 3/2017 and DON 4/2017).
Results
The bait uptake trial sessions ran for an average of 25.7 (s.d. = 3.1) days for cluster sites and 28.6 days (s.d. = 1.4) for transects. A total of 54,361 camera trap nights at 2000 bait locations recorded 1.98 million images, including 1.19 million images of fauna. Of the 5658 Eradicat® baits deployed, 72% (4061 baits) and 1% (85 baits) were removed entirely or partially by fauna, respectively (assuming all bait disappearances were fauna related). The species was identified in 81% and 74% of the whole and partial bait removals, respectively (the remaining bait removals were either not attributable to a species or were partially consumed by insects). A total of 63% (n = 2542) of the whole baits removed had a high level of confidence in the identification of the species responsible, including removal by woylie (28%), koomal (Trichosurus vulpecula hypoleucus; 24%), bush rat (Rattus fuscipes; 10%), chuditch (9%), goanna (Varanus rosenbergi; 6%), raven (Corvus coronoides; 5%), currawong (Strepera versicolor; 3%), mardo (Antechinus flavipes; 2%), black rat (Rattus rattus; 2%), quenda (2%) and quokka (2%). Species that each removed less than 1% of the whole baits in descending order included, bobtail (Tiliqua rugosa), emu (Dromaius novaehollandiae), wambenger, western brush wallaby, feral pig (Sus scrofa), king skink (Egernia kingii), kookaburra (Dacelo novaeguineae), dunnart (Sminthopsis spp.), southwestern crevice skink (Egernia napoleonis), tammar, and magpie (Cracticus tibicen dorsalis).
While it is possible that cats and foxes might have been involved in the proportion of events whereby baits were removed and the species responsible could not be identified, our unpublished data suggests that the RSC set up used in this study rarely if ever missed cat or fox detections close to the baits. This was verified by independent evidence from multiple cameras of various models and/or footprints on purpose-built sand pads used in the same study area but not during these trials. The ‘unknown’ cases in our study are likely mostly due to small reptiles such as the crevice skink on drier sites or small rodents on mesic sites. Evidence includes most of the unknown removals on drier sites occurred during the day in the hotter seasons, and there are records of these lizards removing the baits (especially from time-lapse images) and observations from the cameras of the lizards being present around the baits when the bait trials were being conducted. Rodents on warmer season evenings were likely responsible for most of the unknown removals on the mesic sites based on evidence like that described for skinks.
The total number of independent visits by cat and fox was 100 and 154, respectively. Supplementary material 3 provides further detail on these visits at the site level. Individuals could be confidently discriminated in 90% of the cat visits based on pelage and physical characteristics and assuming spatial independence between sites. This resulted in 51–61 cat individuals being identified (the upper limit including the sum of cat records that could not be distinguished or attributed to identifiable individuals). Fox individuals were more difficult to identify from physical characteristics, but in some cases, they could be discriminated by old injuries such as torn ears, coat condition, distinct markings, size, and/or sex. Additional individuals could be deduced from spatio-temporal evidence (e.g. some individuals could be inferred by the movement pattern of a similar-looking fox recorded on nearby cameras within a limited period). This resulted in an estimated 51–112 fox individuals being encountered across the study.
A minimum of 92% (s.e. = 9.4%) and 84% (s.e. = 8.8%) of the sites were predicted to be occupied by cat and fox, respectively at the time of the trials based on occupancy modelling (Supplementary material 4). As expected, this is higher than the observed naïve occupancy rates (68% for both species, Supplementary material 3), because of the low probabilities of detection.
Introduced predator interactions with the Eradicat® baits
Whole baits were definitely available in 42% (n = 42/100) and 44% (n = 67/154) of the independent visits of a cat or fox, respectively (Table 2). Three and 13 whole baits were definitely consumed or removed by a cat or fox, respectively (Tables 2 and 3). There was also a partial bait definitely consumed by a cat (~20% of the bait consumed by a young animal <2 kg) and another by an adult fox (~70% of the bait by a female <7 kg). In both these cases the consumption of the partial bait was probably lethal, assuming the toxin was evenly distributed throughout the bait (based on 4.5 mg 1080 toxin per bait, cat LD50 = 0.28 mg/kg or LD90 = 0.35 mg/kg and fox LD50 = 0.13 mg/kg) (Mcilroy and King 1990; Eason and Frampton 1991). There were an additional two cases for both cat and fox when a bait was probably removed (i.e. good but not definitive evidence) (Tables 2 and 3).
Cat (n) | Fox (n) | ||
---|---|---|---|
Number of independent introduced predator visits | 100 | 154 | |
Whole bait available | 42 | 67 | |
Partial bait available | 4 | 3 | |
Bait present but an unknown amount of bait available | 1 | 2 | |
Bait definitely not present at the time of visit | 50 | 77 | |
Unknown whether bait was present at the time of visit (bait not visible) | 3 | 5 | |
Cases studied of potential animal interactions with a baitA | 47 | 72 | |
Animal did not get within a body length of the bait and showed no interest in it | 10 | 18 | |
Animal came within <1 body length of the bait but showed no interest in it | 20 | 13 | |
Animal investigated the bait from >1 body length away but came no closer | 0 | 20 | |
Animal came within <1 body length of the bait, investigated it remotely then left | 5 | 5 | |
Animal contacted the bait but did not take it | 6 | 0 | |
Animal took the baitB | 6 | 16 | |
Whole bait definitely eaten | 3 | 8 | |
Whole bait definitely taken away | 0 | 5 | |
Partial bait definitely eaten | 1 | 1 | |
Whole bait probably eaten | 1 | 0 | |
Unknown amount of bait probably taken | 1 | 2 |
Animal | Site | Treatment | When | Bait interaction | Confidence | Comments | |
---|---|---|---|---|---|---|---|
Cat 1 | 12 | Transect | February | Ate partial (20%) | Definite | Young animal, black and white | |
Cat 2 | 31 | Transect | February | Ate whole | Definite | Young animal, dark, mostly uniform, slight tabby | |
Cat 3 | 26 | Cluster | April | Ate whole | Definite | Older animal, dark tabby, classic blotched pattern | |
Cat 4 | 5 | Transect | April | Ate unknown amount | Probable | Older animal, black | |
Cat 5 | 6 | Transect | November | Ate whole | Definite | Young animal, black | |
Cat 6 | 6 | Transect | November | Ate whole | Probable | Older animal, dark, mostly uniform, tail stripes | |
Fox 1 | 20 | Transect | January | Ate whole | Definite | Young animal; indistinct, ate bait 5 days before Fox 2 | |
Fox 2 | 20 | Transect | January | Took whole | Definite | Young animal; indistinct from Fox 1 | |
Fox 3 | 17 | Transect | January | Ate whole | Definite | Distinct poor coat, injuries | |
Fox 4 | 17 | Transect | January | Ate whole | Definite | Distinct coat, skinny male | |
Fox 5 | 17 | Transect | January | Took whole + ate whole | Both definite | Poor condition female; bait interactions 9 min apart | |
Fox 6 | 17 | Transect | January | Ate one unknown amount | Probable | Distinct grey coat, older male | |
Fox 7 | 12 | Transect | February | Took whole | Definite | Indistinguishable | |
Fox 8 | 26 | Cluster | April | Took two wholes | Both definite | Bait interactions 5 days apart; distinct ears | |
Fox 9 | 7 | Cluster | May | Ate whole | Definite | Good condition | |
Fox 10 | 36 | Transect | June | Ate whole | Definite | Distinct injuries, eye damage, poor coat | |
Fox 11 | 36 | Transect | July | Ate unknown amount + one whole | Probable + Definite | Distinct coat, good condition; bait interactions 4 min apart | |
Fox 12 | 6 | Transect | October | Ate partial (70%) | Definite | Distinct ear marks, female | |
Fox 13 | 6 | Transect | November | Ate whole | Definite | Complete ears |
Note: more baiting occurred in October–November (trial sessions 1, 9 and 10) than in other months.
Of the 47 independent visits of cats potentially interacting with a bait (including 42 whole baits, four partial baits and one unknown quantity of bait), 63.8% had no evidence that the individual showed any interest in the bait, 10.6% actively investigated it from a distance, 12.8% contacted the bait but did not take it (smelled or licked the baits), and finally 8.5% definitely – and 4.3% probably – ate the bait (Table 2).
Younger cats appeared to be more interested and more likely to eat a bait than older cats. Of the eight visits involving five younger/smaller cats in the presence of a bait, 75% of the events and all five individuals demonstrated an interest in the bait, which resulted in three young cats eating a bait. Of the 39 cases of older/larger cats being recorded in the presence of a bait, 28% demonstrated an interest in the bait, including one cat that definitely ate a bait and two cats that probably ate a bait. In summary, these trials probably removed 10–12% of the 51–61 individual cats identified, assuming the cats that ate baits died.
Of the 72 cases investigated of foxes potentially interacting with baits (including 67 whole baits, three partial baits and two unknown quantities of bait), 43.1% had no evidence that the individual showed any interest in the bait, 27.8% apparently detected the bait but did not get close to it, 6.9% actively investigated it within close proximity, and the 22.2% that contacted the bait also took it (Table 2). Of the 67 whole baits definitely available when a fox was present, 13 (19%) were definitely taken by a fox. In five of these cases, the whole bait was removed by the fox outside the camera’s field of view and then the fox returned 13–109 s later without the bait (i.e. bait possibly eaten or cached).
A total of 13 fox individuals removed 16 baits, given that three individuals took two baits each (Tables 2 and 3). One individual removed a bait (possibly eaten or cached out of sight) from a cluster site and then five days later was confidently identified removing another bait, after which it was not recorded again. Another individual removed one bait from a transect and then 9 min later was confidently identified eating another bait 300 m away, after which it was not recorded again. In a third case a fox probably ate a bait from a transect and then 4 min later definitely ate a second bait 100 m away, and was not identified again. Overall, there were 9–10 likely lethal baiting events of fox individuals based on enough bait being eaten and recorded on camera (i.e. at least 8–20% of fox individuals encountered in this study). Another three individuals removed the baits outside the field of view of the camera, for which the fate of the bait was unknown and therefore the fate of the fox cannot be deduced (Table 3).
The mean age of the six baits definitely or probably eaten by a cat was 4.4 days (s.d. = 3.9, max = 10.7 days), which was not significantly different from the six that were contacted but not eaten (mean = 9.1 days, s.d. = 9.7, max = 25.1 days; P = 0.15), nor all baits present but not eaten (mean = 4.7, s.d. = 5.8, max = 25.1 days, n = 41; P = 0.43). The mean age of the baits eaten by a fox was 5.6 days (s.d. = 4.0, max = 12.3 days, n = 11 includes partial and probable bait consumptions), which was not significantly different from the five that were taken away (mean = 6.3 days, s.d. = 5.3, max = 14.6 days; P = 0.40), nor all baits present but not removed (i.e. not eaten or taken away; mean = 6.5 days, s.d. = 6.4, max = 24.5, n = 56; P = 0.28). Eight out of 47 cat baiting opportunities and 15 out of 72 fox baiting opportunities had more than 5 mm cumulative rain on the bait at the time of the bait interaction. Six and 11 of these potentially wet baits that were available to a cat and fox respectively, were greater than 5 days old at the time. None and three of the potentially wet baits were eaten by a cat or fox, respectively. There was no significant difference in the mean accumulated rainfall on the six baits that were contacted by a cat but not eaten from the six that were eaten (22.3 mm (s.e. = 16.5) and 0.4 mm (s.e. = 0.4), respectively; P = 0.1208). There was; however, a significant difference between the 6 eaten baits and the 41 that were available but not eaten by a cat (mean = 11.0 mm (s.e. = 4.5); P = 0.0115). There was no significant difference in the mean accumulated rainfall on the baits that were and were not removed by a fox (n = 16, mean = 4.3 mm (s.e. = 2.2) and n = 56, mean = 7.2 mm (s.e. = 2.4), respectively; P = 0.1859). Note that the small sample sizes available in many of these tests are expected to be limiting the statistical power to detect a significant difference.
Differences according to deployment method, over time and over space
The best fitting models included a treatment effect whereby transects had more cat visits (Transect coefficient = 0.7797, s.e. = 0.3745) and more cat baiting opportunities (Transect coefficient = 1.3050, s.e. = 0.5388) than clusters (Table 4).
Model | Degrees of freedom | Cat | Fox | |||
---|---|---|---|---|---|---|
Visit | Baiting opportunity | Visit | Baiting opportunity | |||
Null | 2 | 2.05 | 3.41 | 3.17 | 6.6163 | |
Treatment only | 3 | 0 | 0 | 0.64 | 0 | |
LCU only | 6 | 5.44 | 10.95 | 1.75 | 13.4838 | |
Treatment + LCU | 7 | 2.43 | 7.21 | 0 | 7.4216 | |
Round only | 11 | 13.11 | 15.24 | 15.23 | 14.1428 | |
Treatment + Round | 12 | 10.16 | 11.93 | 10.59 | 7.1465 | |
Full | 16 | 9.55 | 16.71 | 2.26 | 14.3873 | |
Best model: R-squared | 0.089 | 0.145 | 0.27 | 0.29 |
Models with a delta AIC value of 0 (highlighted in bold text) are the best model.
The six cases of baits being consumed by a cat observed with a high (n = 4) or moderate level of confidence (n = 2), involved one cluster site and four transects (one site had two baits removed by different cats; Table 3). There was insufficient count data to test for a treatment difference.
The best model for fox visits included treatment and LCU (Tables 4 and 5), whereby transects had more fox visits than clusters and fox visits were greatest in the YWP LCU and least in SHT. The best model for fox baiting opportunity included a treatment effect whereby transects had more fox baiting opportunities than clusters (Transect coefficient = 1.5411, s.e. = 0.4847). Bait removal events by foxes occurred on five transects and two cluster sites (Table 3). This was insufficient data to test for treatment differences.
Estimate | s.e. | ||
---|---|---|---|
(Intercept) | −2.90714 | 0.40968 | |
LCU_SCJ | 0.07373 | 0.586 | |
LCU_SEU | 0.10848 | 0.71972 | |
LCU_SHT | −0.22203 | 0.63081 | |
LCU_YWP | 1.10406 | 0.47684 | |
Treatment_Transect | 0.71057 | 0.36537 |
While the response variables used in these GLMs are considered sound, lethal bait interactions by cat and fox individuals may have reduced the number of visits and baiting opportunities over the course of the trials at each site. However, any potential effect was relatively minor for cats at least, given that only four to six cat individuals and 9–10 foxes potentially had lethal bait interactions, constituting only 10–12% of the 51–61 cat individuals from 100 visits and 8–20% of the fox individuals from 154 visits across all sites. Furthermore, these cases are not expected to have imposed a significant bias in the response variables used in the GLMs, given there were insufficient bait removal events by cats and foxes (Table 3) to demonstrate statistically significant differences related to treatment, time of year or LCU.
Overall, cats definitely or probably ate 0.1% each of the 998 and 4660 Eradicat® baits deployed in clusters and transects. Foxes removed 0.4% of the Eradicat® baits deployed along transects and 0.1% of baits deployed in clusters (having omitted data potentially affected by Pro-bait deployment activities as described in the Methods). When considering just the first 1000 baits deployed along all of the transects (i.e. only the first 50 Eradicat® baits at 20 sites; i.e. consistent with some operational deployments of Eradicat®, whereby no bait replenishment is done), 0.1% was eaten by a cat and 0.6% were removed by a fox. Based on Fisher’s exact tests, there was no evidence to reject the Null hypotheses that there were no significant differences in Eradicat® bait efficiencies (proportion of baits removed) for either cat or fox between the five baiting events along the transects (P = 0.1177 and 0.07694 for cat and fox, respectively), or between deployment methods (transect vs cluster; either based on all five baiting events along each transect combined (P = 1 and 0.4873 for cat and fox, respectively), or based only on the first bait event on transects (P = 1 for both cat and fox; Fig. 4), noting that these baits were deployed for 7.2 days on average compared to 25.7 days for the cluster treatment). Supplementary material 3 provides a site level summary of the baiting efficiencies for cats and foxes.
Mean Eradicat® bait efficiencies (proportion of baits removed) per site with standard error bars, for cat and fox according to bait deployment method, including the five baiting events along the transects presented separately (G1–G5, number of baits per event combined across all 20 transect treatment sites = 1000, 841, 923, 955 and 941, respectively) and combined (G1-5, n = 4660 baits), and the 20 sites with baits arranged in a cluster, mimicking the aerial bait deployment method (n = 998 baits).
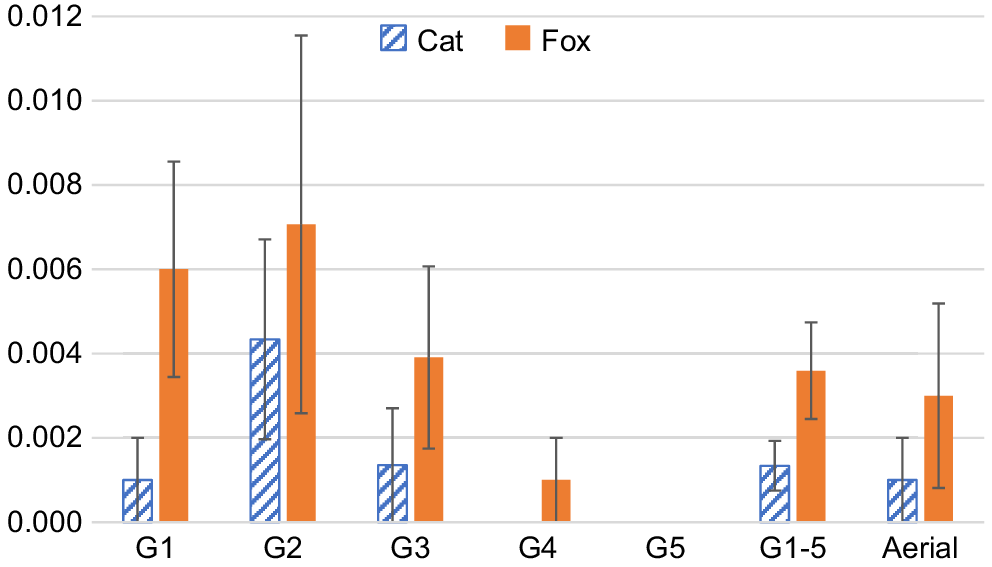
Differences according to proximity to landscape features along transects only
Cat visits to bait locations along transects increased closer to a vehicle track and a surface water feature (Table 6, Fig. 5). When a cat was present, baiting opportunities were not strongly related to proximity to the three landscape features beyond a slight positive relationship with distance from a vehicle track (although the 95% confidence intervals overlapped with zero). The second-best model (delta AIC = 0.84) indicated that proximity to surface water feature was uninformative.
Model | Degrees of freedom | Cat | Fox | |||
---|---|---|---|---|---|---|
Visit | Baiting opportunity | Visit | Baiting opportunity | |||
Private property only | 5 | 16.95 | 2.2 | 9.85 | 3.65 | |
Track only | 5 | 7.03 | 0 | 10.78 | 1.92 | |
Water only | 5 | 4.48 | 2.19 | 14.65 | 5.93 | |
Track + Private property | 6 | 9.03 | 0.99 | 2.22 | 0 | |
Track + Water | 6 | 0 | 0.84 | 8.93 | 1.93 | |
Water + Private property | 6 | 6.34 | 3.9 | 4.8 | NA | |
Full | 7 | 1.86 | 2.12 | 0 | 0.7 |
Note: the degrees of freedom for the baiting opportunity binomial regression models are one less than the zero-inflated models for visits (shown).
Models with a delta AIC value of 0 (highlighted in bold text) are the best model.
Cat (top row) and fox (bottom row) visits to bait locations that are significantly related to proximity of landscape features (left to right, vehicle track, surface water feature and private property).
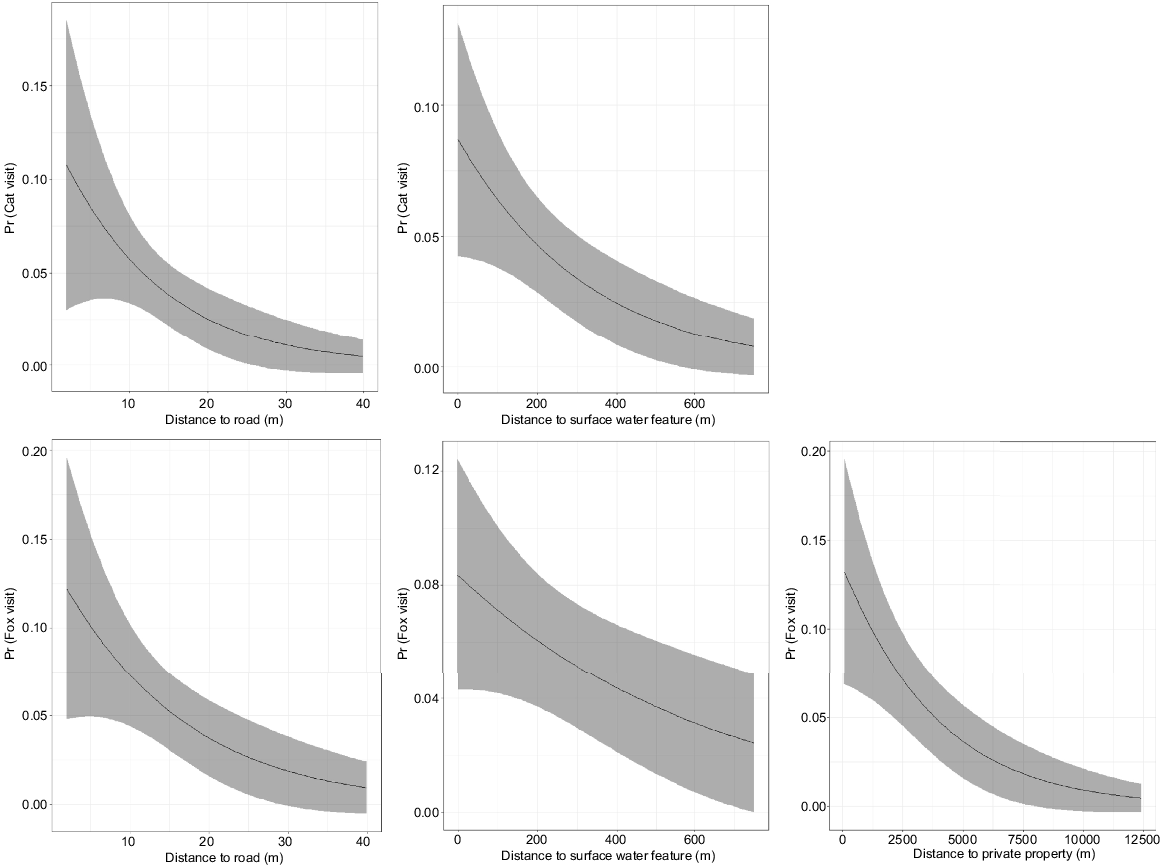
Fox visits to bait locations along transects increased closer to a vehicle track, a surface water feature and private property (Fig. 5). When a fox was present, baiting opportunities were more likely closer to a vehicle track (Fig. 6) but all models with a delta AIC less than 2 indicated that any possible effects of distance to private and surface water features were insignificant. There was insufficient data to relate bait take events by cats or foxes when baits were available along transects (n = 5 and 13, respectively) to their proximity to these landscape features.
Introduced predator interactions with cameras
Of the 100 independent visits of a cat, it was assessed that the individual’s response to the camera was 16% negative, 24% positive, 23% neutral, 28% unaware and 9% indeterminate. During 11 occasions when a cat was present and a part or whole bait was available (n = 47; including three cases of a cat showing a prior interest in the bait), the cat appeared to have a negative response to the camera. In these cases, the camera may have compromised the potential for the cat to detect or interact with the bait. On three occasions when a cat ate a bait it also appeared to have a positive interaction with the camera.
Of the 154 independent visits of a fox, it was assessed that the individual’s response to the camera was 40% negative, 9% positive, 23% neutral, 23% unaware and 5% indeterminate. During 32 occasions when a part or whole bait was available (n = 72; including 19 cases of the fox showing a prior interest in the bait), the fox appeared to have a negative response to the camera. On five occasions when a fox ate a bait it also appeared to have a positive interaction with the camera.
There were several aspects of this study that mean the results may differ from what might have occurred in a fully operational deployment of Eradicat®. This includes bait detectability by target and non-target species may have improved with the placement of baits within relatively open areas (to allow RSCs to record bait condition and animal interactions). Conversely, the RSCs were sometimes detected by cats and foxes and in some cases had an apparent negative effect that may have reduced the number of bait consumption events. Finally, visits and bait consumptions by target species might have been greater had the approvals to use the baits been closer than 5–20 m from the edge of tracks along the transects. Nonetheless, this study provides a practical and informative indication of the effectiveness of Eradicat® baits in the SJF.
Discussion
The efficiency and effectiveness of Eradicat® deployed to mimic the current baiting prescriptions in the Southern Jarrah Forests (SJF) were low for feral cats and slightly better for foxes. This is despite high occupancy rates for both species. There were no clear differences in baiting efficiency and effectiveness between baits deployed in clusters compared with either the initial bait deployment along road-based transects or the subsequent bait replenishments. Our results suggest that baiting for cats and foxes along transects could be improved by placing baits closer to tracks and surface water features. Also, there were more fox visits closer to private property. Substantial improvements could be made by increasing bait availability (i.e. reduce non-target bait interference), detectability and attractiveness to cats. Additional introduced predator threat abatement methods may be needed for the conservation and recovery of many threatened native mammals in the SJFs and similar environments in Australia. Feral cat baiting and other control methods should be used within an integrated and holistic invasive animal management system.
Eradicat® deployment factors
Visits to a bait location by a cat or fox were up to three times greater where baits were deployed along road-based transects rather than in clusters. This is likely to be a function of two spatial factors. Firstly, both cats and foxes can prefer using tracks in some habitats (e.g. Read et al. 2015a; Towerton et al. 2016; Raiter et al. 2018; Geyle et al. 2020; Wysong et al. 2020a, 2020b). Secondly, the spatial array of 50 points along a 5 km transect increases the likelihood of intersecting the home-ranges of more individuals than a 40 m × 200 m area within which a cluster of 50 baits is typically deployed by an aircraft. Unfortunately, the same principle also applies to there being a higher likelihood of more non-target animals being intercepted along a transect, which may also result in more non-target removals of baits.
The rationale for the aerial baiting protocols deploying clusters of 50 baits every kilometre is that the density of baits within the cluster should be greater than the combined appetite of non-target species, thus improving the chances that some baits might be available if/when a cat visits the area. However, the results of this study suggest that bait replenishment (up to 50 baits per linear kilometre at 100 m intervals within a baiting session) may compensate for the lower bait:animal density along transects to provide more cat and fox baiting opportunities than an aerial deployment of baits in clusters.
Our study also found that the frequency of fox visits varied across the region. Fox visits were greatest within the YWP LCU, the predominant habitat in the Upper Warren region (northeast SJF). This area also supports the highest richness, abundance, and activity rates of native mammals (Wayne et al. 2017a, 2019), thereby representing the greatest abundance of prey. Elsewhere, seasonal lows in prey availability are used to exploit hungrier cats more interested in scavenging (e.g. Algar and Burrows 2004; Algar et al. 2007). However, in the SJF there were no clear temporal differences in the visits, baiting opportunities or removal events for cats or foxes during our study. The lack of an apparent ‘sweet spot’ in the timing of cats or foxes consuming baits is most likely a function of sparse data and the characteristics and availability of prey in the SJF. The SJF supports a high abundance and diversity of mammals throughout the year, reptile activity peaks in the warmer drier months and amphibian activity peaks in the cooler, wetter months. This means that prey are plentiful year round and there is little opportunity to exploit hunger driven scavenging behaviours in feral cats (Christensen et al. 2013; Woinarski et al. 2019).
Bait location proximity to landscape features
Landscape context is important in determining baiting success. Our study demonstrates that targeting areas closer to linear features such as tracks and riparian areas can significantly increase both cat and fox visits. Bait locations closer to private property (agriculture or plantation forestry) may also be visited more frequently by foxes. Our observations are consistent with the habitat use by cats and foxes in the SJF (A. Wayne unpubl. data) and elsewhere (e.g. Towerton et al. 2016; Wysong 2016). The higher fox encounters closer to private property may indicate that fox densities (or at least activity) are greater closer to private property. There is also evidence that bait availability for foxes may be greater closer to roads.
Bait interactions and efficiency
Baiting efficiency was low with only 0.1% of the baits being consumed by a cat, at both transects and clusters. Fox baiting efficiency was also low (0.4% of the Eradicat® baits deployed along transects and 0.1% of baits deployed in clusters). This is due to several factors including: high non-target bait interference and low bait detection, interest, and consumption rates by introduced predators. Reduced bait availability due to high levels of non-target bait interference is a significant and widespread problem (e.g. Moseby et al. 2011; Dundas et al. 2014; Doherty and Algar 2015; Fancourt et al. 2022a). Even when Eradicat® baits were available to a cat, relatively few were detected, investigated, and ultimately eaten. Most cats (64%) within proximity of a bait showed no evidence of detection or interest in the bait and only half of those that contacted the bait went on to eat the bait. Younger cats appeared more interested and more likely to eat a bait than older cats, although the sample size was small. This potential age bias is consistent with the findings of others elsewhere (e.g. Lohr and Algar 2020) and could exacerbate management issues if these hard to bait older and larger cats are also disproportionately predating vulnerable fauna (Moseby et al. 2015).
The time the bait has been in the field and exposure to rainfall are also important factors worth further investigation given that we found the baits that were eaten tended to be ‘younger’ and had been exposed to less rain than those that were contacted by a cat but not eaten, although these differences were not statistically significant due to small sample size. Foxes were apparently less fussy than cats with no discernible ‘age’ or accumulated rainfall difference between baits that were removed from those that were not. Furthermore, all foxes that contacted the bait removed the bait, resulting in foxes proportionately removing about twice as many of the available baits than were removed by cats (22.2% vs 12.8%, respectively). Bait age and ambient conditions may also affect bait toxicity (e.g. Kirkpatrick 1999). For example, a limited analysis found that Eradicat® bait toxicity in field conditions (near Rockhampton, Queensland) may be reduced by 25% after 7 days and 35% by 14 days (Fancourt et al. 2022b).
The low baiting efficiency rates observed in our study are consistent with results from many larger Eradicat® baiting programs elsewhere being typically <1% (e.g. Algar et al. 2010; Moseby and Hill 2011; Algar et al. 2020). While baiting efficiency is not a meaningful indicator of baiting success, reducing the number of non-target bait interactions should increase the opportunities for a cat to eat a bait, reduce costs and/or reduce possible risks to potentially vulnerable non-target fauna. Ways to reduce non-target bait interference may include altering the spatial and temporal distribution of baits, detectability and attractiveness of the bait, access (e.g. use of gantries or other animal excluding devices), and size and palatability of the bait.
Bait efficacy
The effectiveness of the Eradicat® baiting trials was low in our study, with about 10–12% of cat individuals and 8–20% of the fox individuals encountered, having consumed enough Eradicat® bait to be considered lethal. Because of the nature of these baiting trials, it is not possible to directly relate these results to what proportion of the population might be removed under a full-scale operational baiting program. However, these results do indicate that operational efficacy rates are likely to be relatively low, because of the reduced bait availability (high-non-target uptake), low detection rates, low interest in the baits, and ultimately, the low consumption rates. For control methods to be effective at substantially reducing cat and fox populations, annual removal rates of more than 20–60% are probably needed (e.g. Hone et al. 2010; Venning et al. 2021), depending on factors such as spatial scale, demographics, population densities, carrying capacity and compensatory feedbacks (e.g. immigration, fecundity and survivorship) (e.g. Hone et al. 2015; Lieury et al. 2015; Porteus et al. 2018). Some of the options available to help achieve higher cull rates, may include increasing baiting frequency or density, increasing the efficacy of baiting, and/or integrating baiting with other measures, such as live trapping (Lohr and Algar 2020).
The high abundance and diversity of native mammals is likely the greatest challenge to overcome to improve the efficacy of Eradicat® baiting in the SJF. Indeed, others have found baiting efficacy to be inversely related to prey availability (e.g. Short et al. 1997; Christensen et al. 2013). We may also have a ‘wicked’ problem whereby as introduced predator numbers are reduced and native animals correspondingly increase, the effectiveness of food-related control methods are likely to decrease due to increased non-target bait interference and lower motivation by cats to take the fewer baits available when prey are abundant. This may be especially problematic for the conservation of some vulnerable species. Differing prey susceptibilities, prey preferences and the risks of hyper-predation and prey switching mean that some species are at greater risk of local extinction even when other species are abundant. Evidence that this may be occurring in the SJF includes the catastrophic declines of seven native prey species while others have increased (Wayne et al. 2017a).
Increasing bait consumption rates by cats by improving the detectability and attractiveness of the baits to cats is also important to improving baiting efficacy. The challenge here is doing so while also reducing the consumption rates by non-target species, thereby increasing bait availability for cats. Reducing non-target consumption rates is also important for reducing the risks to potentially vulnerable native populations through individuals having access to multiple baits and potentially exceeding their natural dosage tolerances to the toxins used in the baits. For example, the risks to potentially vulnerable non-targets can be reduced by deploying the baits along transects, which is at a lower density (1 bait per 100 m) to clusters of 50 baits deployed by aircraft (50 baits within < 1 ha).
Beyond improvements to the efficacy of Eradicat® baiting programs, more effective introduced predator management may be achieved through: (i) the use of other cull methods (e.g. trapping, shooting, biological control, guardian dogs, Felixer grooming traps, and/or toxic collars and implants in prey species) (Woinarski et al. 2019; Venning et al. 2021), especially those less reliant on food attractants; (ii) integration with the control of other invasive species (e.g. Woinarski et al. 2019); (iii) managing introduced predators across the landscape and therefore across tenure; (iv) reducing the resources that limit population growth (e.g. alternative food sources) and immigration; (v) appropriate habitat management to improve the natural physical and chemical defences provided by the habitat for the vulnerable native prey (e.g. Gastrolobium thickets) (Short et al. 2005; Read et al. 2015b); (vi) increasing the area of core habitat by consolidating and expanding areas principally used for wildlife conservation; and (vii) integration with other management activities (e.g. timber harvesting, prescribed burning, roads), recreation and other sources of disturbance (e.g. Doherty et al. 2015). A good understanding of the biology, ecology and behaviour of the target and priority non-target species and a robust scientific approach are also fundamentally important to effective introduced predator control for native species conservation. Whatever the combination of strategies and methods, an holistic, integrated and adaptive management approach is necessary to optimise threat abatement for vulnerable native species because of the myriad and dynamism of the interactions between the various constituents within the ecosystems (e.g. Doherty et al. 2017; Woinarski et al. 2019; Lohr and Algar 2020).
Declaration of funding
The work was conducted as part of the South West Threatened Fauna Recovery Project, in consultation and collaboration with these and other related projects. Funding was received from the Australian Government’s National Landcare Program and the Western Australian Department of Biodiversity, Conservation and Attractions.
Acknowledgements
We acknowledge the Nyoongar people as the Traditional Custodians of the lands and animals on which this study was conducted. We thank the large number of volunteers that helped with the field work and image management, and our colleagues for their advice and support including Matthew Williams, Sarah Comer, Dave Algar, and their teams. Joanne Potts and Billy Geary helped with the statistical modelling. Michelle Drew, Sarah Comer, Ashley Millar and Tim Doherty provided comments on the manuscript. Thank you also to the editors and reviewers for their help to improve the paper.
References
Algar D, Burrows ND (2004) Feral cat control research: Western Shield review-February 2003. Conservation Science Western Australia 5(2), 131-163.
| Google Scholar |
Algar D, Angus GJ, Williams MR, Mellican AE (2007) Influence of bait type, weather and prey abundance on bait uptake by feral cats (Felis catus) on Peron Peninsula, Western Australia. Conservation Science Western Australia 6(1), 109-149.
| Google Scholar |
Algar D, Angus GJ, Brazell RI, Gilbert C, Withnell GB (2010) Eradication of feral cats on Faure Island, Western Australia. Journal of the Royal Society of Western Australia 93(3), 133-140.
| Google Scholar |
Algar D, Johnston M, Tiller C, Onus M, Fletcher J, Desmond G, Hamilton N, Speldewinde P (2020) Feral cat eradication on Dirk Hartog Island, Western Australia. Biological Invasions 22(3), 1037-1054.
| Crossref | Google Scholar |
Bengsen A, Butler J, Masters P (2011) Estimating and indexing feral cat population abundances using camera traps. Wildlife Research 38(8), 732-739.
| Crossref | Google Scholar |
Christensen PES, Ward BG, Sims C (2013) Predicting bait uptake by feral cats, Felis catus, in semi-arid environments. Ecological Management & Restoration 14(1), 47-53.
| Crossref | Google Scholar |
Comer S, Speldewinde P, Tiller C, Clausen L, Pinder J, Cowen S, Algar D (2018) Evaluating the efficacy of a landscape scale feral cat control program using camera traps and occupancy models. Scientific Reports 8(1), 5335.
| Crossref | Google Scholar | PubMed |
de Tores PJ, Sutherland DR, Clarke JR, Hill RF, Garretson SW, Bloomfield L, Strümpher L, Glen AS, Cruz J (2011) Assessment of risks to non-target species from an encapsulated toxin in a bait proposed for control of feral cats. Wildlife Research 38(1), 39-50.
| Crossref | Google Scholar |
Doherty TS, Algar D (2015) Response of feral cats to a track-based baiting programme using Eradicat® baits. Ecological Management & Restoration 16(2), 124-130.
| Crossref | Google Scholar |
Doherty TS, Dickman CR, Nimmo DG, Ritchie EG (2015) Multiple threats, or multiplying the threats? Interactions between invasive predators and other ecological disturbances. Biological Conservation 190, 60-68.
| Crossref | Google Scholar |
Doherty TS, Dickman CR, Johnson CN, Legge SM, Ritchie EG, Woinarski JCZ (2017) Impacts and management of feral cats Felis catus in Australia. Mammal Review 47(2), 83-97.
| Crossref | Google Scholar |
Doherty TS, Hall ML, Parkhurst B, Westcott V (2022) Experimentally testing the response of feral cats and their prey to poison baiting. Wildlife Research 49(2), 137-146.
| Crossref | Google Scholar |
Dundas SJ, Adams PJ, Fleming PA (2014) First in, first served: uptake of 1080 poison fox baits in south-west Western Australia. Wildlife Research 41(2), 117-126.
| Crossref | Google Scholar |
Eason CT, Frampton CM (1991) Acute toxicity of sodium monofluoroacetate (1080) baits to feral cats. Wildlife Research 18(4), 445-449.
| Crossref | Google Scholar |
Fancourt BA, Zirbel C, Cremasco P, Elsworth P, Harry G, Gentle MN (2022a) Field assessment of the risk of feral cat baits to nontarget species in eastern Australia. Integrated Environmental Assessment and Management 18(1), 224-244.
| Crossref | Google Scholar | PubMed |
Fancourt BA, Harry G, Speed J, Gentle MN (2022b) Efficacy and safety of Eradicat® feral cat baits in eastern Australia: population impacts of baiting programmes on feral cats and non-target mammals and birds. Journal of Pest Science 95(1), 505-522.
| Crossref | Google Scholar |
Fleming PA, Anderson H, Prendergast AS, Bretz MR, Valentine LE, Hardy GES (2014) Is the loss of Australian digging mammals contributing to a deterioration in ecosystem function? Mammal Review 44(2), 94-108.
| Crossref | Google Scholar |
Geary WL, Wayne AF, Tulloch AIT, Ritchie EG, Maxwell MA, Doherty TS (2023) Fox and cat responses to fox baiting intensity, rainfall and prey abundance in the Upper Warren, Western Australia. Wildlife Research 50, 201-211.
| Crossref | Google Scholar |
Geyle HM, Stevens M, Duffy R, Greenwood L, Nimmo DG, Sandow D, Thomas B, White J, Ritchie EG (2020) Evaluation of camera placement for detection of free-ranging carnivores; implications for assessing population changes. Ecological Solutions and Evidence 1(1), e12018.
| Crossref | Google Scholar |
Hohnen R, Ashby J, Tuft K, McGregor H (2013) Individual identification of northern quolls (Dasyurus hallucatus) using remote cameras. Australian Mammalogy 35(2), 131-135.
| Crossref | Google Scholar |
Hone J, Duncan RP, Forsyth DM (2010) Estimates of maximum annual population growth rates (rm) of mammals and their application in wildlife management. Journal of Applied Ecology 47(3), 507-514.
| Crossref | Google Scholar |
Hone J, Drake VA, Krebs CJ (2015) Prescriptive and empirical principles of applied ecology. Environmental Reviews 23(2), 170-176.
| Crossref | Google Scholar |
Jansen J, McGregor H, Axford G, Dean AT, Comte S, Johnson CN, Moseby KE, Brandle R, Peacock DE, Jones ME (2021) Long-distance movements of feral cats in semi-arid South Australia and implications for conservation management. Animals 11(11), 3125.
| Crossref | Google Scholar | PubMed |
Lieury N, Ruette S, Devillard S, Albaret M, Drouyer F, Baudoux B, Millon A (2015) Compensatory immigration challenges predator control: an experimental evidence-based approach improves management. The Journal of Wildlife Management 79(3), 425-434.
| Crossref | Google Scholar |
Lohr CA, Algar D (2020) Managing feral cats through an adaptive framework in an arid landscape. Science of The Total Environment 720, 137631.
| Crossref | Google Scholar |
Marlow NJ, Thomas ND, Williams AAE, Macmahon B, Lawson J, Hitchen Y, Angus J, Berry O (2015a) Lethal 1080 baiting continues to reduce European Red Fox (Vulpes vulpes) abundance after more than 25 years of continuous use in south-west Western Australia. Ecological Management & Restoration 16(2), 131-141.
| Crossref | Google Scholar |
Marlow NJ, Williams AAE, Brazell R, MacMahon B, Withnell B, Thomas N, Hamilton N, Fuller P, Asher J (2015b) The development of a toxic 1080 bait, Pro-bait, for fox (Vulpes vulpes) control in Western Australia. Conservation Science Western Australia 9(3), 249-257.
| Google Scholar |
Mcilroy JC, King DR (1990) Appropriate amounts of 1080 poison in baits to control foxes, Vulpes vulpes. Australian Wildlife Research 17, 11-13.
| Crossref | Google Scholar |
Meek P, Ballard G, Fleming P, Falzon G (2016) Are we getting the full picture? Animal responses to camera traps and implications for predator studies. Ecology and Evolution 6(10), 3216-3225.
| Crossref | Google Scholar | PubMed |
Molsher R, Newsome AE, Newsome TM, Dickman CR (2017) Mesopredator management: effects of red fox control on the abundance, diet and use of space by feral cats. PLoS ONE 12(1), e0168460.
| Crossref | Google Scholar |
Moseby KE, Hill BM (2011) The use of poison baits to control feral cats and red foxes in arid South Australia I. Aerial baiting trials. Wildlife Research 38(4), 338-349.
| Crossref | Google Scholar |
Moseby KE, Read JL, Galbraith B, Munro N, Newport J, Hill BM (2011) The use of poison baits to control feral cats and red foxes in arid South Australia II. Bait type, placement, lures and non-target uptake. Wildlife Research 38(4), 350-358.
| Crossref | Google Scholar |
Moseby KE, Peacock DE, Read JL (2015) Catastrophic cat predation: a call for predator profiling in wildlife protection programs. Biological Conservation 191, 331-340.
| Crossref | Google Scholar |
Moseby K, Hodgens P, Bannister H, Mooney P, Brandle R, Lynch C, Young C, Jansen J, Jensen M (2021) The ecological costs and benefits of a feral cat poison-baiting programme for protection of reintroduced populations of the western quoll and brushtail possum. Austral Ecology 46(8), 1366-1382.
| Crossref | Google Scholar |
Myers N, Mittermeier RA, Mittermeier CG, da Fonseca GAB, Kent J (2000) Biodiversity hotspots for conservation priorities. Nature 403(6772), 853-858.
| Crossref | Google Scholar | PubMed |
National Land and Water Resources Audit (2002) Australian terrestrial biodiversity assessment 2002. National Land and Water Resources Audit, Canberra. Available at https://catalogue.nla.gov.au/catalog/916056
Newkirk ES (2016) CPW photo warehouse. Colorado Parks and Wildlife, Fort Collins, CO, USA. Available at https://cpw.state.co.us/learn/Pages/ResearchMammalsSoftware.aspx
Orell P (2004) Fauna monitoring and staff training: Western Shield review – February 2003. Conservation Science Western Australia 5(2), 51-95.
| Google Scholar |
Porteus TA, Reynolds JC, McAllister MK (2018) Quantifying the rate of replacement by immigration during restricted-area control of red fox in different landscapes. Wildlife Biology 2018(1), 1-9.
| Crossref | Google Scholar |
Radford JQ, Woinarski JCZ, Legge S, Baseler M, Bentley J, Burbidge AA, Bode M, Copley P, Dexter N, Dickman CR, Gillespie G, Hill B, Johnson CN, Kanowski J, Latch P, Letnic M, Manning A, Menkhorst P, Mitchell N, Morris K, Moseby K, Page M, Ringma J (2018) Degrees of population-level susceptibility of Australian terrestrial non-volant mammal species to predation by the introduced red fox (Vulpes vulpes) and feral cat (Felis catus). Wildlife Research 45(7), 645-657.
| Crossref | Google Scholar |
Raiter KG, Hobbs RJ, Possingham HP, Valentine LE, Prober SM (2018) Vehicle tracks are predator highways in intact landscapes. Biological Conservation 228, 281-290.
| Crossref | Google Scholar |
Read JL, Bengsen AJ, Meek PD, Moseby KE (2015a) How to snap your cat: optimum lures and their placement for attracting mammalian predators in arid Australia. Wildlife Research 42(1), 1-12.
| Crossref | Google Scholar |
Read JL, Peacock D, Wayne AF, Moseby KE (2015b) Toxic Trojans: can feral cat predation be mitigated by making their prey poisonous? Wildlife Research 42, 689-696.
| Crossref | Google Scholar |
Reddiex B, Forsyth DM, McDonald-Madden E, Einoder LD, Griffioen PA, Chick RR, Robley AJ (2006) Control of pest mammals for biodiversity protection in Australia. I. Patterns of control and monitoring. Wildlife Research 33(8), 691-709.
| Crossref | Google Scholar |
Robley A, Gormley AM, Forsyth DM, Triggs B (2014) Long-term and large-scale control of the introduced red fox increases native mammal occupancy in Australian forests. Biological Conservation 180, 262-269.
| Crossref | Google Scholar |
Short J, Turner B, Risbey DA, Carnamah R (1997) Control of feral cats for nature conservation. II. Population reduction by poisoning. Wildlife Research 24(6), 703-714.
| Crossref | Google Scholar |
Towerton AL, Kavanagh RP, Penman TD, Dickman CR (2016) Ranging behaviour and movements of the red fox in remnant forest habitats. Wildlife Research 43(6), 492-506.
| Crossref | Google Scholar |
Twigg LE, Martin GR, Eastman AF, King the late DR, Kirkpatrick WE (2003) Sensitivity of some Australian animals to sodium fluoroacetate (1080): additional species and populations, and some ecological considerations. Australian Journal of Zoology 51(5), 515-531.
| Crossref | Google Scholar |
Venning KRW, Saltré F, Bradshaw CJA (2021) Predicting targets and costs for feral-cat reduction on large islands using stochastic population models. Conservation Science and Practice 3(8), e448.
| Crossref | Google Scholar |
Wayne A, Moore J (2011) The jewel in the crown: Perup and the Upper Warren. Landscope 26(3), 10-17.
| Google Scholar |
Wayne AF, Maxwell MA, Ward CG, Vellios CV, Wilson I, Wayne JC, Williams MR (2015) Sudden and rapid decline of the abundant marsupial, Bettongia penicillata in Australia. Oryx 49(1), 175-185.
| Crossref | Google Scholar |
Wayne AF, Maxwell MA, Ward CG, Wayne JC, Vellios CV, Wilson IJ (2017a) Recoveries and cascading declines of native mammals associated with control of an introduced predator. Journal of Mammalogy 98(2), 489-501.
| Crossref | Google Scholar |
Wayne AF, Wilson BA, Woinarski JCZ (2017b) Falling apart? Insights and lessons from three recent studies documenting rapid and severe decline in terrestrial mammal assemblages of northern, south-eastern and south-western Australia. Wildlife Research 44(2), 114-126.
| Crossref | Google Scholar |
Wysong ML, Hradsky BA, Iacona GD, Valentine LE, Morris K, Ritchie EG (2020a) Space use and habitat selection of an invasive mesopredator and sympatric, native apex predator. Movement Ecology 8, 18.
| Crossref | Google Scholar |
Wysong ML, Iacona GD, Valentine LE, Morris K, Ritchie EG (2020b) On the right track: placement of camera traps on roads improves detection of predators and shows non-target impacts of feral cat baiting. Wildlife Research 47, 557-569.
| Crossref | Google Scholar |