The 1986 Annaburroo experimental grassland fires: data
James S. Gould A , Miguel G. Cruz

A
Abstract
In 1986, CSIRO conducted a large program of experimental fires in grassland at Annaburroo Station, Northern Territory, Australia, with the objective of quantifying the effect of fuel condition (load and height) on fire behaviour.
This paper provides the data collected during this program, representing a unique set of observations and measurements of large, free-burning experimental fires conducted in a multi-factor experimental design.
Data are collated by experimental burn plot, providing detailed measurements of weather (wind speed, air temperature, relative humidity), fuel state (load, height, moisture content, curing) and fire behaviour (rate of spread, flame depth, flame height, head fire width), as well as processed information (e.g. steady-state rate of spread).
The data are made available for free download on the CSIRO Data Access Portal (https://data.csiro.au/collection/csiro:58746) and include detailed metadata descriptions of the data and their structure, also provided in this article.
We have made the data available for fire behaviour researchers around the world to use in their research under the Creative Commons Attributions licence. It is hoped they will analyse these data and extract new and innovative insights to help improve our understanding of wildland fires burning in grass fuels.
Keywords: Experimental fires, fire behaviour, fuel state, grassland fire.
Introduction
Our current understanding of wildland fire behaviour dynamics is underpinned by empirical evidence (e.g. Van Wagner 1977; Cheney et al. 1993; Cheney and Gould 1995a, 1995b; Fernandes et al. 2008; McCaw et al. 2012; Finney et al. 2018). Empirical data, the basis of an evidence-based scientific approach, provide insights into the physical processes and mechanisms driving a phenomenon and ensure theories and hypothesis can be objectively evaluated. As fire is a scale-dependent phenomenon (Fons et al. 1963; Byram 1966), the study of wildland fire requires field-based experiments at a scale (e.g. size, intensity) that ensures the key processes governing behaviour and spread are captured. The planning and safe execution of well-thought-out experimental fire research projects that adequately test hypotheses (e.g. Cheney et al. 1993; Stocks et al. 2004; Gould et al. 2007; McCaw et al. 2012; Finney et al. 2018; Katurji et al. 2023), particularly of high-intensity wildfire propagation, are often constrained by legal, operational and safety regulations. This largely explains why the field of wildland fire behaviour is data-poor. The open sharing of experimental fire data is a necessary component for the advancement of fire behaviour science.
The CSIRO (Commonwealth Scientific and Industrial Research Organisation) conducted a large experimental burning program during the dry season of July and August 1986 at Annaburroo Station, 100 km south-east of Darwin, in the Northern Territory, Australia, in collaboration with a significant number of Australian State and Territory rural fire and land management agencies (Cheney et al. 1989). These experiments were initially intended to evaluate the effect of grass fuel attributes (such as fuel load, fuel bed height, fuel particle size, moisture content) on the forward rate of fire spread.
The major direct outcomes of this research were the separation of the calculation of fire rate of spread from that of fire danger in the McArthur (1973) Grassland Fire Danger Meter (Cheney and Gould 1995a) and development of new fire spread models (CSIRO 1997; Cheney et al. 1998; Cheney and Sullivan 2008) to supplant that of McArthur for fires in grasslands. These models are currently the recommended models for use in grasslands in Australia for fire behaviour prediction (Cruz et al. 2015; Plucinski et al. 2017) and recently have also been implemented in the new Australian Fire Danger Rating System (Hollis et al. 2024). Other outcomes included analysis of the effect of fuel load on fire spread and flame characteristics (Cheney et al. 1993), the effect of fireline width on fire growth and acceleration (Cheney and Gould 1995b, 1997), the relevance of non-dimensional convection numbers on grassland fire dynamics (Sullivan 2007), and future grassland fire management under the impacts of climate change (Sullivan 2010). This dataset was also used to parameterise grassland fire spread models in the Canadian Fire Behaviour Prediction System (Forestry Canada Fire Danger Group 1992). A small subset of the dataset, comprising detailed fire propagation isochrones and associated burning conditions, has been made available to a number of researchers to calibrate and test physical based models, such as the Wildland–Urban Interface Fire Dynamics Simulator (Mell et al. 2007; Moinuddin et al. 2018), FIRETEC (Dupuy et al. 2011) and FIRESTAR (Morvan et al. 2009; Morvan 2011). More recently, Khanmohammadi et al. (2022) used the full dataset to train different machine learning algorithms and evaluate their suitability to predict the spread rate of grassfires.
The aim of this paper is to make the Annaburroo experimental burn program data available to the international fire behaviour research community, and to foster its use by fire behaviour modellers. Use and analysis of this dataset should be preceded by a careful reading of Cheney et al. (1989, 1993, 1998), Cheney and Gould (1995b), Durre and Beer (1989) and Gould (1991).
The data
We present here the base environmental and fire behaviour data associated with the free-burning experimental fires carried out in open grasslands at Annaburroo Station (the Annaburroo Grassland Experimental Fire dataset). Data are provided at different spatial and temporal scales: at the original sample scale (e.g. 2-m wind speed at 5-s intervals, fuel load at the sample quadrat location), observation period (average per burning observation period within each fire) and plot (i.e. averaged for each experimental fire). Data are made available for free download from the CSIRO Data Access Portal (DAP, https://data.csiro.au/collection/csiro:58746). Fig. 1 illustrates the structure of the data files in the repository. Under the main root folder are two files that collate fire and environmental data by burn observation period (Burn_Period_Data.csv) and by fire experiment (Plot_Average_Data.csv). Burn observation period is defined by the timing of oblique aerial photography taken during each experimental fire at approximately 20–50 s intervals (see Fig. 2 for an example) – continuous observation of fire behaviour was not feasible at the time of the experiments. Plot average is the average value for all variables for each experimental fire. An additional file, AWS_Data.csv, provides the meteorological data for each burn day.
Annaburroo Experimental Grassland Fire Data file structure and naming convention. FirePlotData consists of 120 folders, one for each fire plot encoded with the fire plot ID given as one letter and three digits, i.e. annn. Each FirePlotData folder consists of wind speed measured at 2 m at each plot corner, a series of oblique aerial photographs illustrating the spread of the fire at semi-regular periods, defining the spread interval and annotated in the filename with the time of the photo in hhmmss (hour, minutes, seconds) format, a map of the fire isochrones at each spread interval, and a map of the fuel attributes as measured across each plot prior to ignition. The folder ‘Site and block maps’ contains maps of the location of the experimental site including the location of the automatic weather station (AWS), as well as detailed maps of the layout of each experimental block. AWS_Data.csv consists of meteorological data collected at the AWS for each burn day during the burn program. Burn_Period_Data.csv collates the data for each fire plot by each burn period (interval) while Plot_Average_Data.csv provides the average of these values for each fire plot. Numerical data are provided as comma-separated values in ASCII text files (csv file format) whereas images (photos and isopleth maps) are provided as JPEG format files.
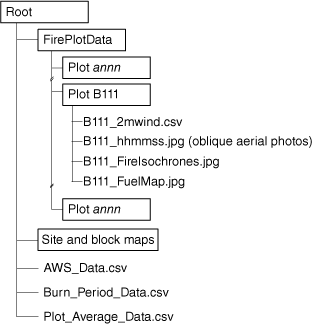
Example series of oblique aerial photographs (a–e) showing growth of experimental fire B111 and a composite map of fire perimeter isochrones for experimental fire B111 after rectification to planar view (f). Ignition took place at 13:26:10 hours. Photograph file names indicate plot number (as annn) and time photograph was taken (as hhmmss: hour, minutes, seconds).
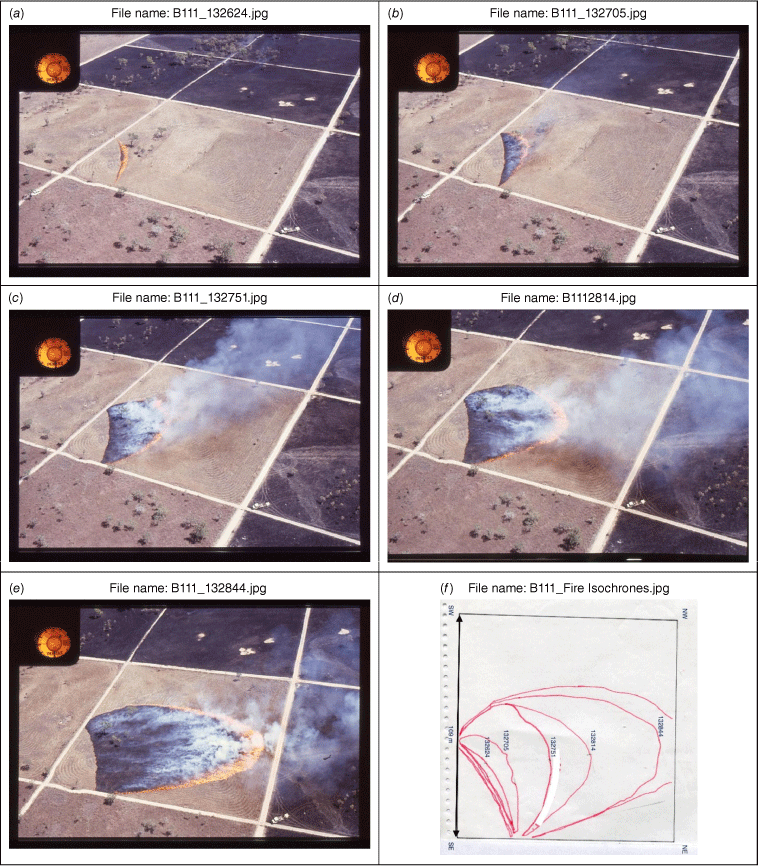
The Site and block maps folder contains, as PNG image files, maps of the experimental site as well as individual maps of each experimental block within the site. Each experimental block was further subdivided into experimental plots.
The FirePlotData folder consists of 120 folders, one for each experimental fire encoded with the fire plot ID given as one letter and three digits (annn). Each of these folders contains a number of files with the basic data for each experimental fire, namely: (1) the annn_2mwind.csv file with the wind speed measured at 2 m at each plot corner; (2) the series of oblique aerial photographs illustrating the spread of the fire at semi-regular periods defining the burn period observation and spread interval and annotated in the filename with the time of the photo in hhmmss format (e.g. Fig. 2a–e; annn_hhmmss.jpg); (3) a geo-rectified map of the fire isochrones at each spread interval (Fig. 2f; annn_FireIsochrones.jpg); and (4) a map of the transects of fuel height and load samples (annn_FuelMap.jpg) as measured in each plot prior to ignition. Each aerial photograph defines the start or the end of a burn observation period used in file Burn_Period_Data.csv. In this file, data on environmental and fire behaviour variables have been processed and averaged per identified burn observation period for each individual experimental fire. Plot-level data that aggregates each experiment burn observation period data into a single plot level average are provided in the Plot_Average_Data.csv file.
Numerical data are provided as comma-separated values in ASCII text files (csv file format) while images (photos and isopleth maps) are provided as JPEG image format files. Table 1 lists the variables provided in the burn observation period and plot average data files (Burn_Period_Data.csv and Plot_Average_Data.csv). Table 2 lists the variables provided in the site AWS and plot wind data files (AWS_Data.csv and annn_2mwind.csv).
Variable | Description | Burn period data | Plot average data | |
---|---|---|---|---|
FireID | Individual fire plot identification | ✓ | ✓ | |
Date.yymmdd | Date of experimental fire, yy, year; mm, month; dd, day | ✓ | ||
IgnTime.hhmmss | Time of ignition, hh, hour; mm, minutes; ss, seconds | ✓ | − | |
IgnLngth.m | Ignition line length (m), 0 = point ignition fires | ✓ | ✓ | |
2mWind.ms | 2-m wind speed (m s−1); average of the four anemometers located on the corners of the experimental plot | ✓ | ||
MidFlWind.ms | Mid-flame wind speed calculated from 2-m average wind speed and the wind speed profile at the time of the measurement (m s−1) | ✓ | ||
10mWind.ms | Wind speed at 10 m in the open as an extrapolation of the 2-m average wind and the wind speed profile at the time of the measurement (m s−1) | ✓ | ||
NE2mWind.ms | 2-m wind speed (m s−1) measured at the NE corner of the experimental plot | ✓ | ||
NW2mWind.ms | 2-m wind speed (m s−1) measured at the NW corner of the experimental plot | ✓ | ||
SW2mWind.ms | 2-m wind speed (m s−1) measured at the SW corner of the experimental plot | ✓ | ||
SE2mWind.ms | 2-m wind speed (m s−1) measured at the SE corner of the experimental plot | ✓ | ||
Temp.C | Air temperature (°C) at 1.4 m during fire from the AWS weather tower | ✓ | ✓ | |
RH.% | Relative humidity (%) at 1.4 m during the fire from the AWS weather tower | ✓ | ✓ | |
CloudCov.8 | Cloud cover (visual okta estimates), e.g. 2/8 represents 2 oktas (two-eighths cloud cover) | ✓ | ||
FuelType | Fuel type: ER, Eriachne sp.; TH, Themeda triandra | ✓ | ✓ | |
FuelTreat | Fuel treatment | ✓ | ✓ | |
E1 = Eriachne sp., natural/undisturbed | ||||
E2 = Eriachne sp., grass cut at 50% of natural height and left on site | ||||
E3 = Eriachne sp., grass cut at 50% of natural height and removed from site | ||||
T1 = Themeda triandra, natural undisturbed | ||||
T2 = Themeda triandra, grass cut at 50–25% of natural height and left on site | ||||
T3 = Themeda triandra, grass cut at 50–25% of natural height and removed from site | ||||
HeadFShape | Head fire shape | ✓ | ||
1 = Pointed shape | ||||
2 = Parabolic shape | ||||
FuelHt.m | Fuel height (m) | ✓ | ✓ | |
FuelLoad.kgm2 | Fuel load dry mass (kg m−2) | ✓ | ✓ | |
SAVR.cm | Surface area-to-volume ratio (cm−1) | ✓ | ||
Cure.% | Degree of grass curing (%) visual estimate | ✓ | ✓ | |
DFMC.% | Dead fuel moisture content (%) measured | ✓ | ✓ | |
BurnPeriodEnd.hhmmss | Time of isopleth fire perimeter, hh, hour; mm, minutes; ss, seconds | ✓ | ||
CumDist.m | Distance head fire travel from ignition (m) | ✓ | ||
IncDist.m | Increment distance between fire perimeter isopleths (m) | ✓ | ||
FlameDpthAir.m | Flame depth interpreted from oblique aerial photographs (m) | ✓ | ||
FlameDpthGnd.m | Flame depth (m) from ground observations | ✓ | ||
FlameHt.m | Flame height (m) from ground observations | ✓ | ||
FlameAng.deg | Flame angle (°) from ground observations | ✓ | ||
FireDir.deg | Bearing of head fire travel (°) | ✓ | ||
TimeSinceIgn | Fire perimeter time since ignition (s) | ✓ | ||
MidTime | Mid-point time between fire spread intervals (s) | ✓ | ||
ROS.ms | Forward rate of spread (m s−1) | ✓ | ✓ | |
EffHFWidth.m | Effective head fire width (m) calculated as per Cheney and Gould (1995b) | ✓ | ||
RSS.ms | Steady-state rate of fire spread calculated from observed rate of fire spread and effective width of the fire front (EffHFWidth.m) (m s−1); see Cheney and Gould (1995b) | ✓ |
Plot average data are generally the average of all the burn period data for a given plot. In some cases, specific burn periods were omitted from the plot average value owing to missing or non-representative data.
Variable | Description | AWS_Data.csv | annn_2mwind.csv | |
---|---|---|---|---|
AWSDate.yymmdd | Date, yy, year; mm, month; dd, day | ✓ | ||
AWSTime.hhmmss | Time, hh, hour; mm, minutes; ss, seconds | ✓ | ||
2mAWSWind.ms | Automatic weather station 2-m wind speed (m s−1) | ✓ | ||
10mAWSWind.ms | Automatic weather station 10-m wind speed (m s−1) | ✓ | ||
10mAWSDir.deg | Automatic weather station 10-m wind direction (°) | ✓ | ||
Temp.C | Air temperature (°C) at 1.4 m during fire from the AWS weather tower | ✓ | ||
10mTemp.C | Air temperature (°C) measured at the AWS at 10-m height | ✓ | ||
RH.% | Relative humidity (%) at 1.4 m during the fire from the AWS weather tower | ✓ | ||
Insol.kW | Insolation (kW m−2) measured at the AWS at 1.4 m height | ✓ | ||
Time.hhmmss | Time, hh, hour; mm, minutes; ss, seconds | ✓ | ||
ExpPeriod | Timing of measurement relative to experiment. Pre, measurement prior to ignition; fire, measurement during the experiment; post, measurement after experiment concluded | ✓ | ||
NW2mWind.ms | 2-m wind speed (m s−1) measured at the NW corner of the experimental plot | ✓ | ||
NE2mWind.ms | 2-m wind speed (m s−1) measured at the NE corner of the experimental plot | ✓ | ||
SE2mWind.ms | 2-m wind speed (m s−1) measured at the SE corner of the experimental plot | ✓ | ||
SW2mWind.ms | 2-m wind speed (m s−1) measured at the SW corner of the experimental plot | ✓ |
Weather data in AWS_Data.csv were recorded at 1-min intervals. Wind speed data in annn_2mwind.csv were recorded at 5-s intervals. Wind speed data are the average value recorded over the sampling period.
Experimental design
The experiments took place at Annaburroo station, NT, Australia (12°54′25″S, 131°40′02″E, Fig. 3). The site is a broad flat area in the flood plain of the Mary River. The area was divided in 10 main blocks (A–J in Fig. 3), and then subdivided into 170 plots. Of these, 121 fitted the requirements of the study (plots with trees or inadequate fuel distribution were excluded). The experimental plots had two sizes: 100 × 100 m and 200 × 200 m. Fuel breaks of bare mineral earth 1.5 or 3 m wide separated the plots. The experiments were carried out during July and August 1986. This period is characteristically the middle of the dry season at the location. Grasses are normally fully cured, and prevailing weather patterns ensure a predominance of warm and dry easterly winds over the area. Rain events during this period are infrequent. A wide buffer area was burnt around the perimeter of the experimental area to reduce the risk of escape of experimental fires even under heightened fire danger conditions. Table 3 summarises the means and ranges of weather, fuel and fire behaviour data collected during the experimental program. Table A1 lists all the experimental fires by date.
Map of the layout of the experimental blocks (A–J) and 200 × 200 m experimental plots within each block at Annaburroo Station. Some plots were further divided into 100 × 100 m plots. Each main plot was defined by a 3-m wide graded bare-earth break. Inset map indicates the location (star) of the experimental site in the Northern Territory, Australia.
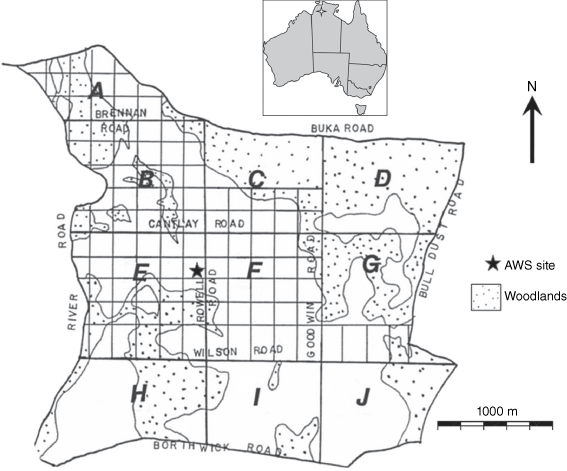
Variable | Mean | Minimum | Maximum | |
---|---|---|---|---|
Weather | ||||
Air temperature (°C) | 30.2 | 23.0 | 38.0 | |
Relative humidity (%) | 29.7 | 13.0 | 55.0 | |
NE 2-m wind speed (m s−1) | 3.80 | 0.0 | 8.3 | |
NW 2-m wind speed (m s−1) | 4.15 | 0.3 | 10.0 | |
SW 2-m wind speed (m s−1) | 4.14 | 0.0 | 11.0 | |
SE 2-m wind speed (m s−1) | 3.70 | 0.0 | 7.7 | |
Fuel | ||||
Degree curing (%) | 91.5 | 80 | 100 | |
Fuel bed height (m) | 0.29 | 0.07 | 0.59 | |
Fuel Load (kg m−2) | 0.35 | 0.12 | 0.61 | |
Surface area-to-volume ratio | ER = 97.7 cm−1 | |||
TH = 122.4 cm−1 | ||||
Dead fuel moisture content (%) | 6.80 | 2.7 | 12.1 | |
Fire behaviour | ||||
Ignition line length (m) | 65.9 | 0 | 200 | |
Rate of fire spread (m s−1) | 0.87 | 0.02 | 4.88 | |
Flame height (m) | 2.2 | 0.3 | 6.0 | |
Flame angle (°) | 47.9 | 10 | 120 | |
Flame depth (m) | 5.2 | 0.1 | 12.0 |
For a detailed breakdown of fuel bed variables with fuel treatment, please see Table 3 in Cheney et al. (1993).
Fuel condition and sampling
The selected site contained two distinct grass species, Eriachne burkittii Jansen (commonly kerosene grass) and Themeda triandra Forrsk. (previously known as Themeda australis R.Br, commonly kangaroo grass) that provided fuel beds of dissimilar characteristics. Further manipulation of the fuel bed was achieved by controlling grass height (by cutting at 50 and 25% of the undisturbed height) and fuel quantity (by removing or leaving the cut fuel). This yielded six different fuel bed treatments (see Treatment in Table 1). Treatments were randomly assigned to each plot.
Surface-area-to-volume ratio for the two major species was measured on selected plants. The diameter of stalks and thickness of leaves were measured with a micrometer and converted to a surface-area-to-volume ratio following Brown (1970). The mean surface-area-to-volume of a plant was estimated as the average value of leaves and stalk component, weighted by their length (Gould 1991).
Fuel bed structure at an experimental plot level was sampled through a 16-point grid located within the plot (Fig. 4). At each sample point, the mean height of the surrounding grass sward was measured and a destructive sample (0.3 × 0.6 m quadrat) of all standing and matted fuel collected. Each fuel sample was oven-dried at a nominal temperature of 104°C for 24 h to determine its dry weight, expressed as the mass per unit area in units of kilograms per square metre. Plots with large fuel heterogeneity or discontinuous fuel cover were excluded from burning or from analysis.
Weather data
An automatic meteorological station (AWS) was located at a fixed site within the experimental area for the duration of the experimental program (see Fig. 3). At this AWS, measurements of wind speed were made at 10 and 2 m above ground. Wind speed measurements used UNIDATA anemometers that were calibrated in the CSIRO Division of Atmospheric Research wind tunnel in Aspendale, Victoria. Wind direction was measured at the 10-m height using a UNIDATA wind vane. Wind speed and direction data were recorded as 1-min averages.
Air temperature, relative humidity and solar radiation at the AWS were measured at 1.4-m height. Air temperature was measured using a solid-state temperature probe (UNIDATA AD537) that was shielded and aspirated with an encapsulated UNIDATA 2B57 pre-amplifier. Relative humidity was measured with a Philips Humidicap sensor. Solar radiation was measured with a pyranometer (make and model unknown), calibrated using the maximum recorded value on cloudless days. The temperature probe was calibrated in a temperature-controlled oil bath over the range 20–40°C. The relative humidity sensor was calibrated against field readings using an Assman psychrometer. Air temperature, relative humidity and solar radiation were measured and recorded in UNIDATA dataloggers at 1-min intervals.
On selected days, vertical temperature measurements were taken every 150 m from the ground up to ~1400 m using a helicopter with either an Assman psychrometer or the helicopter’s temperature probe. On most days, the data revealed a super adiabatic layer near the ground up to 150 m, a well-mixed layer aloft to ~800 m and an inversion above that (Durre and Beer 1989).
For each experimental fire, wind speed at 2-m height was measured at each plot corner (NW, NE, SE, SW). Purpose-built three-cup sensitive anemometers (Bradley 1969) with a start-up speed of 0.05 m s−1 were used for these measurements, with the anemometers being calibrated in the wind tunnel as described above. These wind speeds were recorded on pre-synchronised UNIDATA data loggers at 5-s intervals, with the recorded value being the average of the 5-s period. Fig. 5 illustrates the variability of the wind speed measured before, during and after an experimental fire.
Fuel moisture content and curing level
Four samples of fully cured grasses were collected in, or within a few metres of, each experimental plot for determination of fuel moisture content representative of the conditions of the experimental fire. Samples (approximately 30 g each) were collected in an undisturbed area where boundary effects, such as the existence of a fire break, were not present. Two samples were collected immediately prior to ignition and two after the experimental fire was concluded. The samples were stored in a hermetically sealed container to ensure no moisture loss occurred prior to determination of wet weight. Wet weight was determined within hours of sample collection using a sensitive balance, and then the sample was oven-dried at 104°C for 24 h and reweighed. Fuel moisture was calculated as a fraction of oven-dried weight (see Matthews 2010). The average of the four measurements expressed the dead fuel moisture content of each experimental fire.
Curing level was initially not a variable in the experimental burn program as it was conducted during the dry season in uniformly fully cured grasses. However, an unseasonal rain event just prior to commencement of the burning experiments caused germination of green shoots underneath the fully cured grass midway through the burning experiment. Subsequently, curing level for each experimental plot was visually estimated by the two senior scientists on site based on guidelines developed from previous experience in determination of grass curing through destructive sampling methods. It was felt by observers that the new growth under the taller fully cured grass had minimal effect on fire behaviour as the fire propagated through the taller dead fuels.
Fire behaviour data
The database comprises experimental fires of two types of ignitions: lines (n = 121 experimental fires) and points (n = 24). Line ignitions aimed to rapidly produce a fire spreading at a quasi-steady-state rate of spread for the prevailing conditions. Point source ignitions aimed to create fires where the dynamics of fire acceleration could be studied. A line ignition was created by two igniters with drip torches who started at the mid-point of the line on the upwind edge of the plot and moved to opposite ends of the line at a speed of ~2 m s−1. As the line was oriented at right angles to the prevailing wind, the technique allowed the rapid development of a wide and deep headfire region (Fig. 2a). The length of ignition line varied with plot area. The 100 × 100 m plots generally had an ignition line of 50 m and took 15–20 s to complete, whereas the 200 × 200 m plots generally had an ignition line of 100 m and took 30–40 s. Differences in plot characteristics, fuel distribution and occasional operator error sometimes resulted in small variations in completed ignition line length. It was these variations in ignition line length that later aided identification of the importance of head fire width in fire spread (Cheney et al. 1993).
Point ignition experiments were conducted in conjunction with the line ignition experiments in the larger plots, typically when plot width was ~200 m, and utilised an unburnt area of these plots. Point source ignitions were created using a common matchstick.
Fire behaviour observations were recorded at 1-min intervals by experienced ground observers accompanying the fire progression. These observers identified features such as flame dimensions (depth, height and angle; see Fig. 6), occurrence of spot fire ignitions ahead of the main fire, wind changes and up- and down-draughts. Low-altitude, oblique aerial photographs were taken from a helicopter at 15–30 s intervals (Fig. 2a–e). The photographs were taken from a position that produced a depression angle of approximately 45° to the principal point (approximately the centre of the plot) and 60° to the base of the plot.
The oblique photographs were interpreted for actual ignition line length, location of the leading edge and rear of the flame front, fire progression, flame depth and identification of ground control points (e.g. centre points of the track intersections, other selected features) for later image rectification and analysis. A custom computer program generated a geometric transformation based on the apparent ground control points (the corners of each plot) using the measured distances between each point. This transformation was then applied in the program to each oblique fire perimeter map to convert it into a planar map. A composite map of time isopleths of fire perimeter (Fig. 2f) and flame depth was then plotted for each experimental fire. The effective head fire width was calculated as the width of the fire front that influenced the next period of head fire spread (Cheney and Gould 1995b). Rate of forward spread was taken as the maximum distance that the head fire advanced between successive fire isochrones. The original fire isochrone maps were digitally scanned and reference scale and isopleths time stamps added (e.g. Fig. 2f). Interested users can utilise state-of-the-art methods to extract relative flame front positions from the oblique aerial imagery for further analysis and perhaps generate new fire progression data.
Data interpretation and intermediate calculations
The process of interpreting fire propagation and averaging its behaviour over a burn observation period and for a single experimental fire requires some explanation.
A burn observation period was defined as the interval between two sequential oblique aerial photographs depicting the location of the flame front. From this, measurements of rate of fire spread and effective head fire width were taken. The 2-m wind speed measurements made at each of the four plot corners were then averaged over this observation period. Careful examination of the anemometer data was conducted to ensure they were representative of the wind field driving the fire. Data from individual anemometers were removed if the location of the anemometer was influenced by external factors such as the presence of trees, firefighting vehicles, or the approaching flame front. A vertical wind profile and estimate of atmospheric stability were calculated at the AWS for each experimental fire (see Durre and Beer (1989) for details). This wind profile, along with the measured flame characteristics and 2-m wind speeds, were used to calculate a representative 10-m open wind speed and a mid-flame height wind speed for each experiment.
The fuel sample grid was overlayed on each of the fire isochrone maps. The mean fuel load and fuel height for each fire plot was calculated from the sample points within the burnt area (Fig. 4).
Conclusions
Nearly 40 years ago, CSIRO bushfire researchers led by Phil Cheney and Jim Gould undertook the most comprehensive study of behaviour of free-burning fires in grasslands. Although the data collected during these experiments had immediate impact, namely through the development of new grassland fire spread models that now are incorporated into Australia’s fire danger rating system and fire behaviour prediction tools, the potential of this dataset for further exploring the behaviour of grassland fires has been barely touched. It is hoped that with the publication of this dataset under a Creative Commons Attribution licence, fire researchers around the world will be able to apply creative and innovative analysis techniques to generate new insights about the behaviour and spread of one of the world’s fastest burning fuel types.
Data availability
The data presented in this publication are available from: https://data.csiro.au/collection/csiro:58746.
Conflicts of interest
Andrew Sullivan is an Associate Editor of the International Journal of Wildland Fire and to mitigate this potential conflict of interest he had no editor-level access to this manuscript during peer review. The authors have no further conflicts of interest to declare.
Declaration of funding
The collation and preparation of these data for publication was funded by the CSIRO.
Acknowledgements
The publication of this dataset could not have happened without the huge efforts of all those who contributed to the execution of the experiments and helped collect the data: Phil Cheney, Peter Hutchings, Ian Knight, John Baxter, Tom Beer, Matt Dando, Mark Durré, Edwin Mak, Peter Vis, Andrew Wilson, Marion Fallon, Rob Birtles (CSIRO), Mark Dawson (South Australia Country Fire Service), Ian Smith (Tasmanian Fire Service), Bruce Ward (Western Australia Department of Conservation and Land Management), Fiona Hamilton (Victorian Department of Conservation, Forests and Lands), Chris Trevitt (Australian National University), L. Jayawardene (Sri Lanka), and the extraordinary efforts of the staff of the Bush Fires Council NT who undertook preparation of the experimental area and fire suppression, Tony Carmody (helicopter pilot) and Jim Sullivan (fuel treatment harvester).
References
Bradley EF (1969) Small sensitive anemometer system for agricultural meteorology. Agricultural Meteorology 6, 185-193.
| Crossref | Google Scholar |
Byram GM (1966) Scaling laws for modeling mass fires. Pyrodynamics 4, 271-284.
| Google Scholar |
Brown JK (1970) Ratios of surface area to volume for common fine fuels. Forest Science 16, 101-105.
| Google Scholar |
Cheney NP, Gould JS (1995a) Separating fire spread prediction and fire danger rating. CALMScience Supplement 4, 3-8.
| Google Scholar |
Cheney NP, Gould JS (1995b) Fire growth in grassland fuels. International Journal of Wildland Fire 5, 237-247.
| Crossref | Google Scholar |
Cheney NP, Gould JS (1997) Fire growth and acceleration. International Journal of Wildland Fire 7, 1-5.
| Crossref | Google Scholar |
Cheney NP, Gould JS, Catchpole WR (1993) The influence of fuel, weather and fire shape variables on fire-spread in grasslands. International Journal of Wildland Fire 3, 31-44.
| Crossref | Google Scholar |
Cheney NP, Gould JS, Catchpole WR (1998) Prediction of fire spread in grassland. International Journal of Wildland Fire 8, 1-13.
| Crossref | Google Scholar |
Cruz MG, Gould JS, Alexander ME, Sullivan AL, McCaw WL, Matthews S (2015) Empirical-based models for predicting head-fire rate of spread in Australian fuel types. Australian Forestry 78, 118-158.
| Crossref | Google Scholar |
Dupuy JL, Linn RR, Konovalov V, Pimont F, Vega JA, Jiménez E (2011) Exploring three-dimensional coupled fire–atmosphere interactions downwind of wind-driven surface fires and their influence on backfires using the HIGRAD-FIRETEC model. International Journal of Wildland Fire 20, 734-750.
| Crossref | Google Scholar |
Fernandes PM, Botelho H, Rego F, Loureiro C (2008) Using fuel and weather variables to predict the sustainability of surface fire spread in maritime pine stands. Canadian Journal of Forest Research 38, 190-201.
| Crossref | Google Scholar |
Finney M, Pearce G, Strand T, Katurji M, Clements C (2018) New Zealand prescribed fire experiments to test convective heat transfer in wildland fires. In ‘Advances in Forest Fire Research 2018. Proceedings of the VII International Conference on Forest Fire Research’. pp. 10–16. (Universidade de Coimbra: Portugal)
Fons WL, Clements HB, George PM (1963) Scale effects on propagation rate of laboratory crib fires. Symposium (International) on Combustion 9, 860-866.
| Crossref | Google Scholar |
Gould JS (1991) Validation of the Rothermel fire spread model and related fuel parameters in grassland fuels. In ‘Proceedings of Conference on Bushfire Modelling and Fire Danger Rating Systems’, Yarralumla, ACT. 11–12 July 1988, pp. 51–64. (Eds NP Cheney, AM Gill) (CSIRO Division of Forestry: Canberra, ACT)
Hollis JJ, Matthews S, Fox-Hughes P, Grootemaat S, Heemstra S, Kenny BJ, Sauvage S (2024) Introduction to the Australian Fire Danger Rating System. International Journal of Wildland Fire 33(3),.
| Crossref | Google Scholar |
Katurji M, Noonan B, Zhang J, Valencia A, Schumacher B, Kerr J, Strand T, Pearce G, Zawar-Reza P (2023) Atmospheric turbulent structures and fire sweeps during shrub fires and implications for flaming zone behaviour. International Journal of Wildland Fire 32, 43-55.
| Crossref | Google Scholar |
Khanmohammadi S, Arashpour M, Golafshani EM, Cruz MG, Rajabifard A, Bai Y (2022) Prediction of wildfire rate of spread in grasslands using machine learning methods. Environmental Modelling & Software 156, 105507.
| Crossref | Google Scholar |
Matthews S (2010) Effect of drying temperature on fuel moisture content measurements. International Journal of Wildland Fire 19, 800-802.
| Crossref | Google Scholar |
McCaw WL, Gould JS, Cheney NP, Ellis PFM, Anderson WR (2012) Changes in behaviour of fire in dry eucalypt forest as fuel increases with age. Forest Ecology and Management 271, 170-181.
| Crossref | Google Scholar |
Mell W, Jenkins MA, Gould J, Cheney P (2007) A physics-based approach to modelling grassland fires. International Journal of Wildland Fire 16, 1-22.
| Crossref | Google Scholar |
Moinuddin KAM, Sutherland D, Mell W (2018) Simulation study of grass fire using a physics-based model: striving towards numerical rigour and the effect of grass height on the rate of spread. International Journal of Wildland Fire 27, 800-814.
| Crossref | Google Scholar |
Morvan D (2011) Physical phenomena and length scales governing the behaviour of wildfires: a case for physical modelling. Fire Technology 47, 437-460.
| Crossref | Google Scholar |
Morvan D, Méradji S, Accary G (2009) Physical modelling of fire spread in grasslands. Fire Safety Journal 44, 50-61.
| Crossref | Google Scholar |
Plucinski MP, Sullivan AL, Rucinski CJ, Prakash M (2017) Improving the reliability and utility of operational bushfire behaviour predictions in Australian vegetation. Environmental Modelling & Software 91, 1-12.
| Crossref | Google Scholar |
Stocks BJ, Alexander ME, Lanoville RA (2004) Overview of the International Crown Fire Modelling Experiment (ICFME). Canadian Journal of Forest Research 34, 1543-1547.
| Crossref | Google Scholar |
Sullivan AL (2007) Convective Froude number and Byram’s energy criterion of Australian experimental grassland fires. Proceedings of the Combustion Institute 31, 2557-2564.
| Crossref | Google Scholar |
Sullivan AL (2010) Grassland fire management in future climate. Advances in Agronomy 106, 173-208.
| Crossref | Google Scholar |
Van Wagner CE (1977) Conditions for the start and spread of crown fire. Canadian Journal of Forest Research 7, 23-34.
| Crossref | Google Scholar |
Appendix
Burn date | Fire plot identifier (fire.id) | |
---|---|---|
30/7/1986 | A011, A012, A022, A052, A053, A061, A066 | |
31/7/1986 | A023, A031, A034 | |
1/8/1986 | A121 | |
4/8/1986 | A032, A033, A041, A071, A072, A073, A074, A081, A082, A083, A084, A091, A092, A141, A142, A143, A144, A151, A154 | |
5/8/1986 | A152, A153, B032, B033, B041, B042, B043, B044 | |
6/8/1986 | B091, B092, B093, B094, B101, B102, B103, B104, B111, B114 | |
14/8/1986 | E14 | |
15/8/1986 | B173, B184, B201, B202, B211, B214 | |
16/8/1986 | B212, B213, B221, B224, E04, E10 | |
17/8/1986 | E03, E05, E09, E11, E15, E16, E17, E18, E21, E22 | |
18/8/1986 | B241, B242, B243, B244, B301, B302, B303, B034, C011, C012, C013 | |
C014, C061, C062, C063, C064, C111, C112 | ||
19/8/1986 | C071, C081, E06, E12, F011, F02, F061, F071, F11, F16, F211, F212, F221, F222 | |
20/8/1986 | F03, F04, F081, F13, F14, F18, F19, F231, F232 | |
21/8/1986 | C04, C05, C09, C10, F05, F10, F12, F15, F17 |