Past fire shaping future fuel: influence of recent fire history on forest foliage chemistry
Margot A. Schneider



A
Abstract
Knowledge of how fire regimes influence flammability, and its role in shaping future fire regimes, exists mostly at species composition and ecosystem levels. The effect of fire regimes on fuel at the chemical level is poorly known.
We aimed to empirically investigate the association between recent fire history and forest foliage chemistry; and to explore potential implications for forest fuel flammability.
Using an orthogonal study design, we investigated the effects of fire frequency and time since fire on the chemical composition of Eucalyptus pilularis leaves from forest canopies and surface litter in south-eastern Australia.
We found high fire frequency (fire-return interval 10–13 years) was associated with higher C:N, C:P, and C:K ratios in E. pilularis surface litter, and higher C:P in canopy leaves.
Nutrients including nitrogen and phosphorous reduce flammability of plant tissues, and hence, the observed differences in leaf nutrients between high and low fire frequency sites could have important implications for future flammability of these forests.
Our study has demonstrated a relationship between fire frequency and leaf nutrient stoichiometry, which may act as a mechanism through which repeated fires could increase fuel flammability. This warrants further investigation in other environments.
Keywords: canopy fuel, Eucalyptus pilularis, fire frequency, fire regimes, foliar nutrients, leaf litter, low-nutrient environment, nutrient resorption, soil.
Introduction
Many parts of the world are experiencing changing fire regimes; extreme weather events are becoming more common, fuel loads are changing, ignition rates are increasing, and as a result, fires are becoming more frequent and sometimes burning more intensely (Cary et al. 2012; Deb et al. 2020; Duane et al. 2021; Jones et al. 2022). Frequent, high-severity fires can have adverse ecological, social, and economic effects (Borchers Arriagada et al. 2020), and therefore, understanding the factors influencing fire behaviour is critically important. Bushfire behaviour is influenced by three main elements: topography, weather, and fuel (Countryman 1972). The effects of topography and weather are well characterised, however the influence of fuel on fire behaviour is complex, due to diverse mechanistic influences. Fuel load is the most well understood variable, and has historically dominated fuel characterisation for fire modelling (McCaw et al. 2012) and management (Eburn and Cary 2017). However, other fuel properties also influence flammability, including: fuel moisture content, leaf morphology, plant architecture, and chemistry (Scarff and Westoby 2006; Tumino et al. 2019). There are limited studies on how fuel properties vary across landscapes (Varner et al. 2015; Pausas et al. 2017).
Variation in landscape-level factors and fire history can cause differences in the flammability of live and dead vegetation (Bowman et al. 2014; Pausas et al. 2017). Popović et al. (2021) concluded that leaf flammability variation was related to the leaf traits response to environmental variables, or site fire history. For example, plants in fire-prone areas have been found to be more flammable than in areas less prone to fire (Pausas and Moreira 2012; Cui et al. 2020). Further, Cui et al. (2020) and Pausas et al. (2012) demonstrated that plants can exhibit intraspecific variation in flammability via changes in leaf chemistry, leaf morphology, moisture content and plant architecture. For example, a decrease in carbon to nitrogen ratio (C:N) and/or carbon to phosphorus ratio (C:P) in leaves can decrease their flammability (DeBano et al. 1998; Drysdale 2011; Scarff et al. 2012; Grootemaat et al. 2015). Repeated fires can increase carbon to nutrient ratios in ecosystems (Beadle 1954; Butler et al. 2019). However, the relationship between foliar nutrient content and past fires has rarely been studied in forest vegetation (Toberman et al. 2014; Archibald et al. 2018). Indeed, foliar nutrient content is generally left out of fire behaviour studies because it is assumed this influence will be negligible at the ecosystem level (Fernandes and Cruz 2012). This may be because other fuel characteristics including structure have been found to have larger effects on flammability than fuel nutrients (Grootemaat et al. 2015). However, small changes in the flammability of vegetation may significantly impact total area burned (Varner et al. 2015; Grootemaat et al. 2017; Archibald et al. 2018). Thus, there is a need to better understand how fuel characteristics vary across landscapes, and in relation to past fire.
Effects of fire on leaf nutrient content are not well characterised, but may occur via indirect pathways whereby fire alters soil nutrient levels and availability (Lagerström et al. 2009), subsequently influencing foliar nutrient content (Butler et al. 2018b). The relationship between fire, soil nutrients and foliar nutrients can be highly variable depending on characteristics of fire events such as fire severity, frequency, and time since last fire, as well as environmental conditions (Cui et al. 2010; Pellegrini et al. 2015; Zhang et al. 2015). Fire can also have direct impacts on foliar nutrients via damage to leaves, stem and root systems of plants (Bär et al. 2019). The indirect and direct pathways (and interactions), through which fire can alter leaf chemistry, make predicting the effects of fire on leaf nutrients challenging. Short-term, low-severity fires may decrease soil nitrogen and increase phosphorus lability (Butler et al. 2017). Immediately following fire, ash deposition can cause an increase in available phosphorus (Butler et al. 2018a). Long-term, soil nitrogen can re-establish via plant nitrogen fixation, increasing nutrient availability post-fire (Adams and Attiwill 1984). However, in sandy soils, labile nutrients (e.g. nitrate) are lost to leaching (Hinojosa et al. 2021). High-severity fires can combust organic matter, causing nutrient loss via volatilisation, and high fire frequency can exacerbate this (Beadle 1954). Finally, long term exclusion of fire can decrease phosphorus lability as nutrients become increasingly sequestered by recalcitrant plant species (Butler et al. 2018a).
In this study, we used an orthogonal study design to investigate effects of fire frequency and time since fire on the chemical composition of Eucalyptus leaves from forest canopies and associated surface litter in a south-eastern Australian forest. We focused on a single species, Eucalyptus pilularis (Smith), the dominant tree species in the study area.
Methods
Study area
This study was conducted in Booderee National Park (35°40′S, 150°40′E), a 6300 ha reserve in south-eastern Australia, which is owned and co-managed by the Wreck Bay Aboriginal Community. Booderee National Park is on a coastal peninsula, with a temperate maritime climate, and receives on average 1240 mm of rain per annum (Lindenmayer et al. 2014). Sandstone, siltstone, and marine conglomerates, topped by dunes and sandy soils, form the geological foundation of landscapes (Lindenmayer et al. 2014). The park is dominated by dry sclerophyll forests (Taws 1997). The spatial distribution of vegetation types within the area is primarily influenced by soil with fire influencing structure and composition within community types (Beadle 1954; Keith 2004; Foster et al. 2017; Bowd et al. 2022).
Fire history
Prescribed and unplanned fires have been well documented in Booderee National Park since 1957 (Foster et al. 2017). Between 1957 and 2012, there were 230 individual fires recorded (average of 4.2 fires per year), with most areas of the park experiencing one to five fires during this time (Foster et al. 2017). Fires ranged from small, low intensity, to moderate, high intensity, with six large bushfires (>5 ha) having occurred in 1962, 1972 (×2), 2002, 2003, and 2017 (Lindenmayer et al. 2014; Foster et al. 2017) (Fig. 1). The fire return interval of forested vegetation in the park ranges from 5 to >20 years, with most areas experiencing return intervals of >10 years (Foster et al. 2017).
Map of Booderee National Park, showing the mosaic of fire frequencies (1957–2019) within forested areas of the park and time since fire, in years. Black circles indicate site locations. Top left shows location of Booderee National Park (red circle) in south-eastern Australia. Non-forested areas are blocked out in grey. Data sources: Booderee National Park Fire History Database and Taws (1997).
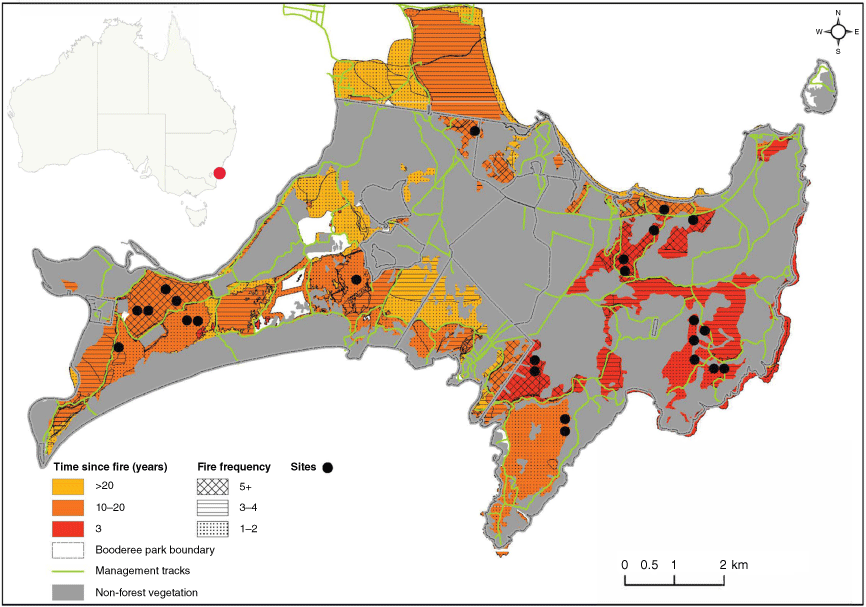
Eucalyptus pilularis forests
Eucalyptus pilularis (Blackbutt), has a wide yet discontinuous distribution along the eastern Australian coastline from the New South Wales/Victorian border to K’gari (formerly Fraser Island) in Queensland (Maiden 1909; Florence 2004; Shepherd and Raymond 2010). The species occurs in open dry sclerophyll and tall, open wet sclerophyll forests, and is associated with soils high in silica (Florence 2004). Eucalyptus pilularis is a fire resistant species, with buds in the stem, producing epicormic growth after canopy fire (Florence 2004). The fire return interval of Booderee National Park of 5–20 years is tolerable for E. pilularis, and typical for dry sclerophyll forests (Bradstock and Kenny 2003; Kenny et al. 2004; Murphy et al. 2013; Foster et al. 2017).
Study design
Study sites were selected in 2018, as part of a larger investigation, using the following approach: A 200 × 200 m grid of points was overlaid on the mapped areas of E. pilularis forest and sites assigned to one of six fire history treatments (low, moderate or high fire frequency; crossed with time since fire: 3 years, or 10–20 years). Six sites were randomly selected for study from each treatment combination, so that time since fire (TSF) and fire frequency (FF) are not correlated (Fig. 1) (for full details see Bowd et al. 2022). From these 36 sites, we selected 24 sites to include in our study; the 12 high fire frequency and 12 low fire frequency sites. Sites with a high fire frequency were subjected to more than five fires in 60 years, representing an average inter-fire interval of 10–13 years. Sites with a low fire frequency were subjected to one or two fires in 60 years, representing an inter-fire interval of approximately 25–30 years. The high fire frequency category of this study is within the tolerable range for dry sclerophyll forests (Bradstock and Kenny 2003; Kenny et al. 2004), and may be viewed as ‘moderate’ in the regional context of fire ecology research. Each of the 24 sites had a slope <20°, northerly aspects (bearing <135° or >225°) and ranged between 7 and 97 m above sea level. At each site we sampled canopy and litter leaves of up to five trees within an 80 m radius of the centre of a previously established 10 × 10 m quadrat (Bowd et al. 2022, 2023).
Leaf sample collection (fresh and litter)
We sampled canopy and surface Eucalyptus litter leaves for nutrient analysis from 117 E. pilularis trees in August 2020 (Australian winter). To do this, five E. pilularis trees closest to the central point of the site were selected. To sample canopy leaves, we removed canopy branches from each selected tree using an arborist throw line launcher, throw line, and a weight bag (340 g) and collected 5–10 fresh leaves in paper bags (Youngentob et al. 2016). Directly below the canopy of the same tree, we collected 5–10 intact, undamaged E. pilularis leaves, from the surface of the litter layer. This approach was used to maximise the likelihood that we were collecting mature, naturally senesced leaves with minimal decomposition from the same individual tree. If there were less than five adult E. pilularis trees located within 80 m of the centre of the site, or if these could not all be sampled safely, we sampled from fewer trees. This occurred at two sites (minimum of three trees sampled per site). Sampled trees were located up to 75.9 m from the centre of each site, with an average of 28.8 m (±1.1 m). The location and diameter at breast height (DBH) were recorded for all trees. After collection, leaves were air dried and stored for up to 8 weeks in brown paper bags in the laboratory (22°C) until processing. We visually inspected all leaves prior to analysis to ensure they were intact, and observed no visible signs of decomposition during this storage period. Leaf samples were dried in metal drying tins for 24 h at 60°C, following methods outlined in Reuter and Robinson (1997). Once dried, stems were removed, and samples finely ground. Total carbon (C) and nitrogen (N) (%) were determined by weighing dried and ground leaf samples of 0.200 g (±0.1 g) into ceramic crucibles, and C and N concentrations were measured with a CN analyser (VarioMax CNS macro-element analyser, Elementar Analytical Systems GmbH, Hanau, Germany). Due to budgetary limitations, three of the five trees per site were analysed for total phosphorus (P) and potassium (K). Total P and K (%) were determined for fresh and litter samples by dry ashing the samples, followed by wet digestion using nitric-perchloric acid (methodology based on Kalra (1998)). Following digestion, concentrations of P and K were determined using inductively coupled plasma–atomic emission spectroscopy (ICP-AES; Varian VistaPro, axial view).
Data analysis
We conducted statistical analysis in RStudio Version (4.3.1) (R Core Team. 2020). We analysed leaf nutrients N, P, and K as ratios to C content, as an indication of nutrient bioavailability (Yuan and Chen 2015), and to be comparable with earlier studies (Archibald et al. 2019). All leaf nutrient ratios were natural log transformed prior to analysis. We excluded five samples (two litter and three fresh) from analyses of leaf P and K due to machine error returning unrealistically low values in adjacent samples. Four additional litter P sample values were below the detection limit of the methodology, and we replaced these values with the detection limit of 0.01%.
The effect of fire history variables and their interaction on leaf chemistry was tested using generalised linear mixed models. The full model for this analysis included fixed effects of leaf type (fresh or litter), fire frequency (FF) and time since fire (TSF), and their interaction. Each model included site elevation (scaled, continuous variable) as a covariate to account for its potential influence on soil nutrient availability. In Booderee National Park, pronounced nutrient leaching can occur at higher elevations, which can lead to low soil nutrient availability at higher elevation sites (Beadle 1954; George et al. 1995). We also included the diameter at breast height (DBH) (scaled, continuous variable) of each tree as a proxy for tree age, which can influence foliar nutrient levels (Whitford 2002; Koch et al. 2008; Turner and Lambert 2008). We included two nested random effects (trees nested within sites) to account for spatial clustering of samples. Models were fitted using the ‘glmmTMB’ function in R with a Gaussian distribution (Brooks et al. 2017). Residuals were plotted using the DHARMa package to inspect the model fit (Hartig 2020). Model selection, based on Akaike’s Information Criterion, corrected for small sample size (AICc) (Burnham and Anderson 2002), was performed using the dredge function in the MuMIn package (Barton and Barton 2015). All possible subsets of the full model were ranked according to AICc, and the most parsimonious model selected. Full model selection tables are presented in Supplementary Tables S1–S3. We used the ‘emmeans’ and the ‘ggplot2’ package (Searle et al. 1980; Wickham et al. 2016) to visualise estimated model means and 95% confidence intervals for the most parsimonious model.
Results
Fire and leaf chemistry
Model selection revealed that fire history was associated with nutrient content of fresh leaves and litter (Table 1, Fig. 2). The most parsimonious models for leaf C:N, C:P, and C:K all included leaf type and fire frequency, while only the C:N and C:K models included the fire frequency by leaf type interaction (Table 1). The variable ‘time since fire’ was not included in any of the most parsimonious models (Table 1). Elevation was included in the most parsimonious model for C:P, and positively associated with higher C:P ratio (P < 0.001). Tree diameter at breast height (DBH) was included and positively associated for C:N (P = 0.016, Table 1).
Parsimonious models | |||||||
---|---|---|---|---|---|---|---|
Carbon:Nitrogen | Carbon:Phosphorus | Carbon:Potassium | |||||
Predictors | Estimates | P | Estimates | P | Estimates | P | |
(Intercept) | 3.974 (3.923–4.026) | <0.001 | 6.532 (6.391–6.674) | <0.001 | 5.504 (5.415–5.594) | <0.001 | |
Leaf Type [Litter] | 0.448 (0.388–0.509) | <0.001 | 0.984 (0.900–1.068) | <0.001 | 2.071 (1.944–2.198) | <0.001 | |
FF [H] | 0.056 (-0.019–0.131) | 0.147 | 0.700 (0.494–0.907) | <0.001 | 0.062 (-0.071–0.196) | 0.358 | |
DBH | 0.029 (0.005–0.053) | 0.016 | |||||
Leaf Type [Litter] × FF [H] | 0.169 (0.080–0.257) | <0.001 | 0.324 (0.136–0.511) | 0.001 | |||
Elevation | 0.214 (0.111–0.317) | <0.001 |
Leaf type = Fresh or Litter, FF = Fire Frequency (Low [L] or High [H]), DBH = tree Diameter at Breast Height. Elevation and DBH were scaled in the model. The full model additionally included TSF (time since fire) and the respective interactions with the other terms in the model. Random terms for each model were nested (1|Site/Tree). Blank cells indicate the term was not included in the most parsimonious model. Intercept indicates Fresh leaf type and Low FF.
Relationship between Fire Frequency (L = Low, H = High), and Leaf type (Fresh and Litter) for (a) Carbon:Nitrogen, (b) Carbon:Phosphorus, (c) Carbon:Potassium (fresh only), and (d) Carbon:Potassium (litter only) ratios of nutrient percentage data. Note different y-axis scales in panels C and D. Solid points are back-transformed predicted means from the most parsimonious model for each measure. Error bars are 95% CI of the predicted means. Open points are raw data points of nutrient ratios from each tree at each site.
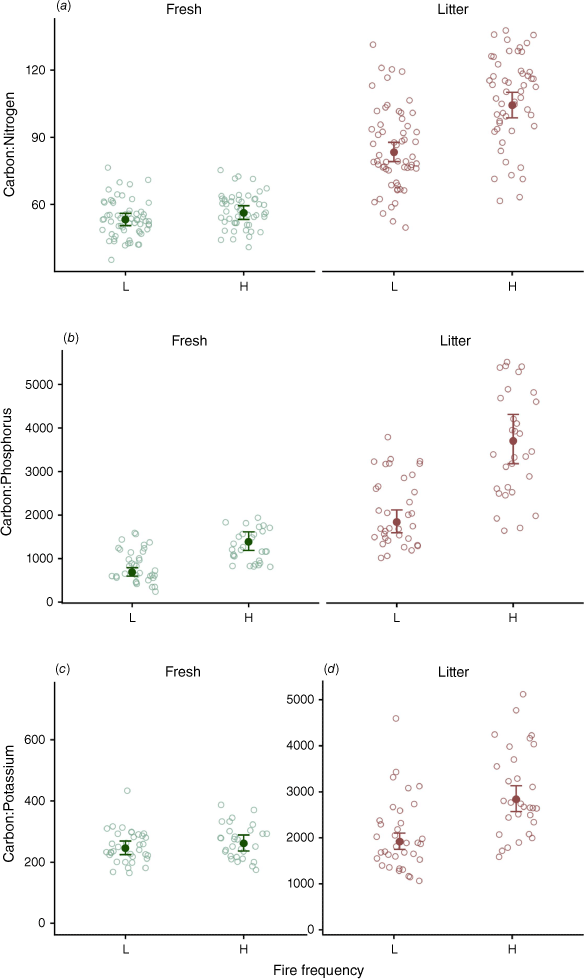
The C:P ratio of fresh leaves was higher under high fire frequency compared to low fire frequency (42% difference, P < 0.001, Table 1, Fig. 2). By contrast, the C:K and C:N ratio of fresh leaves did not differ between fire frequency treatments (P = 0.358 and 0.147 respectively, Table 1, Fig. 2).
In litter samples, C:N, C:P, and C:K ratios were higher in sites with high fire frequency than in sites with low fire frequency (23, 54 and 37% difference respectively, P ≤ 0.001, Table 1, Fig. 2). Additionally, litter C:N, C:P and C:K ratios were higher in leaf litter relative to those in fresh leaves (53, 92 and 162% difference, respectively, P < 0.001, Table 1, Fig. 2).
Discussion
Fire history effects on leaf nutrient content
We aimed to identify how different fire histories were associated with the nutrient content of leaves of mature E. pilularis trees in Booderee National Park. We found that sites with a higher fire frequency were associated with higher C:N, C:P and C:K ratios in E. pilularis leaves. The influence of fire frequency was particularly pronounced in the C:P ratio of litter leaves, with litter from high fire frequency sites having much higher C:P ratios than litter from low fire frequency sites. Our finding that high fire frequency reduced leaf N, P, and K concentrations was broadly consistent with other studies of leaf nutrient responses to long-term changes in fire frequency (Butler et al. 2019). By contrast, we did not observe the short-term differences in nutrients after fire that are commonly reported (Certini 2005; Toberman et al. 2014; Butler et al. 2017, 2019). We discuss two non-competing hypotheses to explain how repeated fire may have reduced the nutrient content of E. pilularis leaves in our study area: (1) indirect effects of fires on soil (Bowd et al. 2022), and (2) direct effects of the fire on trees (Pressler et al. 2019; Nolan et al. 2022).
Potential indirect effects of fire on foliar nutrients
Fire can modify the chemical and physical composition of soil through processes of volatilisation, nutrient leaching and erosion (Fig. 3) (Beadle 1954; Tulau and McInnes-Clarke 2015). Fire-induced changes in soil properties, especially bioavailability, can influence plant nutrient uptake, which then influences foliar nutrient content (Pressler et al. 2019). Such interactions may explain our results of higher carbon to nutrient ratios with high-frequency fire. Carbon in our study was unaffected by fire treatments (Supplementary Table S4), and therefore differences in C:N, C:P, and C:K can be attributed to differences in nutrient content. Fire-induced losses in soil nutrients may have been accelerated in our study location by the low nutrient and high sand content of soils, which can rapidly leach nutrients compared to ecosystems where soils have higher organic matter content (Beadle 1954). In an allied, earlier investigation in the same location of our study, Bowd et al. (2022, 2023) described fire-associated differences in soils, including soil pH, sulfur, soil moisture, carbon, and potassium, but found no difference between measures of available nitrogen (Supplementary Table S6). The low soil phosphorus and potassium availability in high fire frequency sites reported by Bowd et al. (2022) may partially explain our findings of lower foliar phosphorus and potassium with high fire frequency (Supplementary Tables S4 and S6). Moreover, fire-induced changes in soil pH and moisture may also influence the bioavailability of specific soil nutrients, including soil phosphorus and potassium (Certini 2005). Fire converts the organic pool of phosphorus to orthophosphate, which can increase soil pH. A soil pH of 6.5 is optimal for plants to uptake available phosphorus (Certini 2005). However, in acidic soils, like those of Booderee (Supplementary Table S6, mean pH of 4.2), orthophosphate preferentially binds to metals (Certini 2005). While fire may have increased the pH and availability of phosphorus immediately after fire, plants may not have been able to benefit due to soil acidity inhibiting uptake, and high sand content allowing rapid nutrient leaching. Spatially and temporally matched sampling of both soils and trees would be required to determine if fire-induced shifts in soil properties underpin the causal mechanism of fire effects on foliar nutrients. We were unable to draw these conclusions from soil data collected in Bowd et al. (2022) due to sampling at different spatial and temporal scales.
Possible mechanisms for nutrient loss with frequent fire. Grey text indicates loss off nutrient from soil. (a) Defoliation, (b) bark damage/basal scarring, leading to xylem cavitation, and (c) root/mycorrhizal damage, are potential direct causes of nutrient loss from the tree after a fire event. Dotted lines indicate loss of nutrients from the ecosystem.
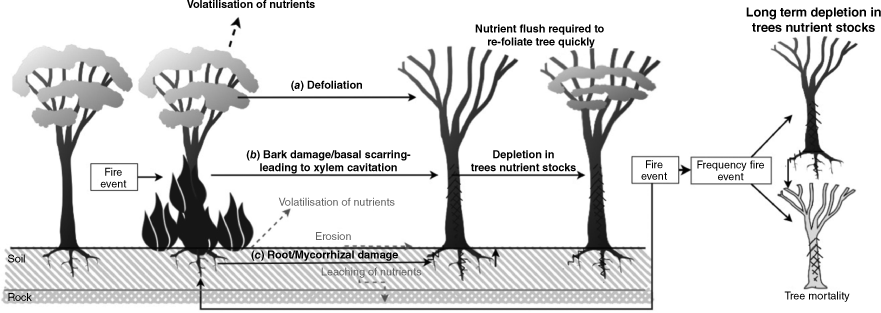
Potential direct effects of fire on trees
While fire can indirectly impact plant nutrient content through soil (Pressler et al. 2019), the direct impact of fire on trees can also result in nutrient losses (Nolan et al. 2022). Three types of direct effects of frequent fire could result in accumulated nutrient loss in trees: (1) fire induced defoliation, (2) basal scarring or bark damage, and (3) root damage (Fig. 3). Defoliation of fire-tolerant eucalypts by high-intensity fires may result in net losses of stored nutrients, including nitrogen and phosphorous (Nolan et al. 2021), with re-foliation after fire depleting nutrient reserves (Bowman and Prior 2005). Damage and consumption of bark during fire may also cause a net loss of nutrients (Varner et al. 2021). Basal scarring and xylem cavitation can compromise the structural integrity of trees, causing hydraulic limitations, leading to nutrient limitation (Nolan et al. 2021; Varner et al. 2021). Finally, damage caused by fire to the tree root systems, and their mycorrhizal symbionts (Bowd et al. 2023), may reduce uptake of water and nutrients (Resco de Dios et al. 2020). With repeated fires, the combination of these effects could result in the foliar nutrient differences we observed between high and low fire frequency treatments.
We found that high fire frequency was associated with higher C:N, C:P, and C:K ratios in E. pilularis leaves. This fire frequency effect appeared to be stronger for C:P ratios, when compared to effects on C:N and C:K ratios, which contrasts with a number of existing studies (Toberman et al. 2014; Tulau and McInnes-Clarke 2015). Further, meta-analysis has shown that fire most often increases phosphorus availability in plants with high N:P ratios (above 20; (Dijkstra and Adams 2015)); however, we did not find this despite high N:P ratios in all fire treatments (Supplementary Table S5). Our contrasting results are likely due to the climatic and soil conditions in the forests of Booderee National Park – environmental factors which can produce large disparities in findings like these (Butler et al. 2017). In high nutrient environments, low intensity fires can decrease carbon and nitrogen more than phosphorus in E. pilularis (Toberman et al. 2014) likely due to differing volatilisation temperatures between elements. Carbon and nitrogen (volatilising at 200°C) will be more likely depleted by low intensity fire, while phosphorus and potassium (volatilising at 774°C) may be unaffected, or increase in availability post-fire, via ash deposition (Certini 2005; Liao et al. 2013; Tulau and McInnes-Clarke 2015). Fires in Booderee National Park occur as a mix of wildfires and prescribed burns with most of the large fires in the 60 years of recorded history being moderate to high severity unplanned fires (Foster et al. 2018). While in our study we did not control for fire intensity due to a paucity of these data, in low nutrient environments both high and low-intensity fires can negatively impact ecological function (Beadle 1954; Toberman et al. 2014; Tulau and McInnes-Clarke 2015). Phosphorus is often limited in dry sclerophyll forests (Bui and Henderson 2013), and sandy soils allow nutrients to be leached easily (Lehmann and Schroth 2003). In higher-nutrient, non-sandy environments, fire converts phosphorus into orthophosphate, which binds to organic matter, increasing phosphorus availability (Certini 2005; Tulau and McInnes-Clarke 2015). The acidic soils of Booderee (Supplementary Table S6) increase the likelihood that orthophosphate will preferentially bind to metals in the soil, limiting bioavailability (Certini 2005). Fire-induced damage to root systems, and mycorrhizal symbionts could also limit nutrient uptake (Taudière et al. 2017; Bowd et al. 2023), making it less likely that tree phosphorus levels recover with frequent fire. Therefore, the combination of high-intensity fires and low-nutrient, acidic, sandy soils, as well as altered plant–nutrient uptake (via root and symbiont damage) may explain why high fire frequency was associated with decreased phosphorus levels in this study.
In this study, time since fire had no association with C:N, C:P or C:K, in fresh leaves or litter samples, which is contrary to many published studies (Toberman et al. 2014; Butler et al. 2019, 2020). Two factors likely influence this outcome. Firstly, we compared only two times since fire treatments; 3 years since fire, and 10–20 years since fire. In some ecosystems, leaf nutrient re-establishment can occur more quickly than the shortest (3 years) time since fire in our study (Certini 2005). Inclusion of both more recently burned sites, and long unburned sites in the future studies may increase the chance of detecting time since fire effects. Secondly, our study was able to separately test effects of time since fire and fire frequency in a factorial design, limiting correlation between the two variables. By contrast, many studies of fire effects on leaf nutrients, fire frequency and time since fire variables remain correlated (e.g. Granged et al. (2011) and Brennan et al. (2009)). Therefore, some reported time since fire effects on nutrients may be confounded with underlying differences in fire frequency, given sites that burn more frequently may have burned more recently (Murphy and Russell-Smith 2010). Interestingly, in the same study system, and also with 3 years post-fire as the shortest time since fire treatment, Bowd et al. (2023) found predominantly time since fire effects, rather than fire frequency effects on soil nutrients. These contrasting results suggest that the post fire relationship between fire, soils, and plants is complex, requiring targeted investigation.
Differences between fresh and litter nutrients: implications for nutrient resorption
Differences in nutrient content between fresh and litter samples revealed insights into nutrient resorption capabilities of E. pilularis in this low nutrient, fire-prone environment. As expected, fresh leaves contained more nutrients than litter (Supplementary Table S4), likely a result of nutrient reabsorption and translocation before senescence (Archibald et al. 2018). However, the resorption efficiency observed is noteworthy. The average nutrient resorption efficiency – defined as the percentage difference in nutrient concentration between fresh leaves and litter, scaled by fresh leaf concentration (Turner and Lambert 2015; He et al. 2020) – was 38, 62 and 89% for nitrogen, phosphorus and potassium respectively, with greater values at high fire frequency sites compared with low fire frequency sites (Supplementary Table S5). These resorption efficiency values are consistent with reported maximum resorption for this species for nitrogen (42%) and phosphorus (56%) (Turner and Lambert 2015), but well exceeds the previously reported maximum for potassium (62%, Turner and Lambert 2015).
The difference between fresh and litter nutrient content was greater in high fire frequency sites, compared with low fire frequency sites, which could indicate fire-induced stoichiometric shifts in E. pilularis at Booderee National Park. Trees in high fire frequency sites may be increasing nutrient resorption before senescence to minimise nutrient loss. This could indicate that trees are investing substantial resources in maintaining leaf nutrient levels. If fires become more frequent and intense (Deb et al. 2020), increasing C:N, C:P, and C:K ratios could drive plant communities to become dominated by species with even more conservative nutrient use strategies, or there may be risk of ecosystem decline (Carbone and Aguilar 2016).
Potential implications for forest flammability
In this study, we observed significant differences between high and low fire frequency sites in the nutrient content of leaves, particularly in the litter layer, which could have implications for the flammability of fuel in these forests. Nutrients can reduce the flammability of vegetation by promoting the formation of char during depolymerisation, which produces a competing reaction with oxygen and limits oxygen availability for flame consumption, reducing combustibility (Grootemaat et al. 2015). Grootemaat et al. (2015) found that higher measures of nitrogen and phosphorus in Eucalyptus leaves can reduce flame duration in fresh and senesced leaves and, in combination with leaf dry mass, these measures can explain a high proportion of variation in flame duration (sustainability) in senesced leaves. Higher C:N, C:P, and C:K ratios can also increase the combustibility of plant biomass (King and Vines 1969; Scarff and Westoby 2008; Grootemaat et al. 2015), and hence a cumulative reduction in nutrient content can significantly increase both combustibility of fuel and flammability (King and Vines 1969; Scarff et al. 2012; Grootemaat et al. 2015). However, leaf size has been shown to be the dominant fuel trait influencing surface litter bed flammability (Scarff and Westoby 2006). Therefore, it is possible that other fuel traits, than those studied here, may have a greater influence on flammability (Scarff et al. 2012) than nutrient content. Future studies of relationships between fire history, multiple leaf traits, and flammability (including burn tests) would be needed to disentangle such effects.
We found that differences in leaf nutrient content between low and high fire frequency sites were greatest in surface litter leaves. This is important given the significance of the surface fuel bed (in combination with leaf dry mass) (Grootemaat et al. 2015) for the initial rate of spread of a fire. In addition to the potential direct effects of nutrient content on leaf flammability, the observed differences in litter nutrients could act to slow litter decomposition rates (due to higher carbon to nutrient ratios (Krishna and Mohan 2017)), which could increase rates of fuel accumulation (Raison et al. 1986). All else being equal, greater litter fuel accumulation in combination with increased fuel flammability could shorten inter-fire intervals, increase fire intensity and reduce longevity of hazard reduction burning effectiveness. Therefore, the relationship between fire frequency and fuel flammability warrants further research attention.
While we detected an association between fire frequency and foliar nutrient content that may have potential implications for forest fuel flammability, conclusions regarding the relative importance of this association in a landscape context cannot be made from the available data. This is because the relationship between fuel traits and flammability is complex and the major components of flammability (ignitibility, combustibility, and sustainability (Anderson 1970) are mechanistically different (Grootemaat et al. 2015). For example, a leaf with low ignitibility, from a thick cuticle, could still be highly combustible once alight due to the presence of volatile oils (Grootemaat et al. 2015). Further, while leaf nutrient ratios differed with fire frequency, nutrient concentrations we report in this study were extremely low across all sites. This might suggest that fire frequency effects on leaf nutrients would ultimately have a small impact on forest flammability relative to other traits, which are not all positively correlated, especially at the forest level (Montgomery and Cheo 1971; Grootemaat et al. 2015). Aside from fuel, topography and climate are strong drivers of future fire patterns (Countryman 1972), and may override observed differences in fuel chemistry in influencing patterns of landscape fire. While our study design controlled for elevation, aspect and slope, it was correlative, not experimental, and hence it is possible that high fire frequency sites lacked nutrients due to unmeasured landscape factors that also drive higher fire frequency at these sites (e.g. wind exposure, geology). While these caveats highlight the need for caution in interpreting our findings, it cannot necessarily be assumed that the observed relationship between fire frequency and leaf nutrients will have negligible effects on forest fuel flammability at a landscape scale. The relationship between flammability and fuel elements in fire behaviour is not linear and small changes in flammability can result in significant differences in total area burned (Archibald et al. 2018).
Our study has highlighted a potential mechanism through which repeated fires could alter fuel flammability in a coastal Eucalyptus forest. To fully understand how fire regimes might influence flammability through changing nutrient concentrations, burn tests to quantify effects of leaf nutrient concentrations on flammability are essential, ideally in combination with other leaf traits. Complementary investigations of the relationship between fire and soil, leaf and litter nutrients, and how these vary across landscapes would reveal to what extent variation in leaf nutrient content should be considered in bushfire behaviour modelling and management.
Data availability
Data that support this study will be shared upon reasonable request to the corresponding author.
Conflicts of interest
Geoffrey Cary was an Associate Editor of International Journal of Wildland Fire. To mitigate this potential conflicts of interest he had no editor-level access to this manuscript during peer review. The authors declare no other conflicts of interest.
Declaration of funding
This research was funded by the Australian Research Council, Parks Australia, and the Australian Department of Defence as part of an ARC Linkage Project (LP170100152). M.A.S. was supported by a scholarship from the National Parks Association of the ACT.
Acknowledgements
We acknowledge the Wreck Bay Aboriginal Community, the owners and traditional custodians of the land on which our research was conducted. We thank the Wreck Bay Aboriginal Community Council and Parks Australia for supporting this project. The authors would also like to thank Chris MacGregor for assisting with data collection, Wade Blanchard for data analysis advice and James Latimer and Andras Keszei for providing advice on chemical analyses.
References
Adams M, Attiwill P (1984) Role of Acacia spp. in nutrient balance and cycling in regenerating Eucalyptus regnans F. Muell. forests. I. Temporal changes in biomass and nutrient content. Australian Journal of Botany 32(2), 205-215.
| Crossref | Google Scholar |
Anderson HE (1970) Forest fuel ignitibility. Fire Technology 6(4), 312-319.
| Crossref | Google Scholar |
Archibald S, Lehmann CE, Belcher CM, Bond WJ, Bradstock RA, Daniau A, Dexter K, Forrestel E, Greve M, He T (2018) Biological and geophysical feedbacks with fire in the Earth system. Environmental Research Letters 13(3), 033003.
| Crossref | Google Scholar |
Archibald S, Hempson GP, Lehmann C (2019) A unified framework for plant life-history strategies shaped by fire and herbivory. New Phytologist 224(4), 1490-1503.
| Crossref | Google Scholar | PubMed |
Bär A, Michaletz ST, Mayr S (2019) Fire effects on tree physiology. New Phytologist 223(4), 1728-1741.
| Crossref | Google Scholar | PubMed |
Barton K, Barton MK (2015) Package ‘MuMIn’. Version, 1(18), 439.
| Crossref | Google Scholar |
Beadle N (1954) Soil phosphate and the delimitation of plant communities in eastern Australia. Ecology 35(3), 370-375.
| Crossref | Google Scholar |
Borchers Arriagada N, Palmer AJ, Bowman DM, Morgan GG, Jalaludin BB, Johnston FH (2020) Unprecedented smoke-related health burden associated with the 2019-20 bushfires in eastern Australia. Med J Aust 213(6), 282-283.
| Crossref | Google Scholar | PubMed |
Bowd EJ, Egidi E, Lindenmayer DB, Wardle DA, Kardol P, Cary GJ, Foster C (2022) Direct and indirect effects of fire on microbial communities in a pyrodiverse dry-sclerophyll forest. Journal of Ecology 110(7a), 1687-1703.
| Crossref | Google Scholar |
Bowd EJ, Egidi E, Lindenmayer DB, Wardle DA, Kardol P, Foster C (2023) Temporal dynamics of soil fungi in a pyrodiverse dry‐sclerophyll forest. Molecular Ecology 32(15), 4181-4198.
| Crossref | Google Scholar | PubMed |
Bowman DMJS, Prior LD (2005) Why do evergreen trees dominate the Australian seasonal tropics? Australian Journal of Botany 53(5), 379-399.
| Crossref | Google Scholar |
Bowman DMJS, Murphy BP, Neyland DLJ, Williamson GJ, Prior LD (2014) Abrupt fire regime change may cause landscape-wide loss of mature obligate seeder forests. Global Change Biology 20(3), 1008-1015.
| Crossref | Google Scholar | PubMed |
Bradstock R, Kenny B (2003) An application of plant functional types to fire management in a conservation reserve in southeastern Australia. Journal of Vegetation Science 14(3), 345-354.
| Crossref | Google Scholar |
Brennan KEC, Christie FJ, York A (2009) Global climate change and litter decomposition: more frequent fire slows decomposition and increases the functional importance of invertebrates. Global Change Biology 15(12), 2958-2971.
| Crossref | Google Scholar |
Brooks ME, Kristensen K, Van , Benthem KJ, Magnusson A, Berg CW, Nielsen A, Skaug HJ, Machler M, Bolker BM (2017) glmmTMB balances speed and flexibility among packages for zero-inflated generalized linear mixed modeling. The R journal 9(2), 378-400.
| Crossref | Google Scholar |
Bui EN, Henderson BL (2013) C:N:P stoichiometry in Australian soils with respect to vegetation and environmental factors. Plant and Soil 373(1), 553-568.
| Crossref | Google Scholar |
Burnham KP, Anderson DR (2002) ‘Model selection and multimodel inference: A practical information-theoretic approach’. 2nd edn. (Springer: New York, NY, USA) 10.1007/b97636
Butler OM, Lewis T, Chen C (2017) Fire alters soil labile stoichiometry and litter nutrients in Australian eucalypt forests. International Journal of Wildland Fire 26(9), 783-788.
| Crossref | Google Scholar |
Butler OM, Elser JJ, Lewis T, Mackey B, Chen C (2018a) The phosphorus-rich signature of fire in the soil–plant system: a global meta-analysis. Ecology Letters 21(3), 335-344.
| Crossref | Google Scholar |
Butler OM, Rezaei Rashti M, Lewis T, Elser JJ, Chen C (2018b) High-frequency fire alters soil and plant chemistry but does not lead to nitrogen-limited growth of Eucalyptus pilularis seedlings. Plant and Soil 432(1), 191-205.
| Crossref | Google Scholar |
Butler OM, Lewis T, Rezaei Rashti M, Maunsell SC, Elser JJ, Chen C (2019) The stoichiometric legacy of fire regime regulates the roles of micro-organisms and invertebrates in decomposition. Ecology 100(7), e02732.
| Crossref | Google Scholar | PubMed |
Butler OM, Lewis T, Rezaei Rashti M, Chen C (2020) Long-term fire regime modifies carbon and nutrient dynamics in decomposing Eucalyptus pilularis leaf litter. Frontiers in Forests and Global Change 3, 22.
| Crossref | Google Scholar |
Carbone LM, Aguilar R (2016) Contrasting effects of fire frequency on plant traits of three dominant perennial herbs from Chaco Serrano. Austral Ecology 41(7), 778-790.
| Crossref | Google Scholar |
Certini G (2005) Effects of fire on properties of forest soils: a review. Oecologia 143(1), 1-10.
| Crossref | Google Scholar | PubMed |
Cui Q, Lü X-T, Wang Q-B, Han X-G (2010) Nitrogen fertilization and fire act independently on foliar stoichiometry in a temperate steppe. Plant and Soil 334(1), 209-219.
| Crossref | Google Scholar |
Cui X, Paterson AM, Wyse SV, Alam MA, Maurin KJL, Pieper R, Padullés Cubino J, O’Connell DM, Donkers D, Bréda J, Buckley HL, Perry GLW, Curran TJ (2020) Shoot flammability of vascular plants is phylogenetically conserved and related to habitat fire-proneness and growth form. Nature Plants 6(4), 355-359.
| Crossref | Google Scholar | PubMed |
Deb P, Moradkhani H, Abbaszadeh P, Kiem AS, Engström J, Keellings D, Sharma A (2020) Causes of the widespread 2019–2020 Australian bushfire season. Earth’s Future, 8(11), e2020EF001671.
| Crossref | Google Scholar |
Dijkstra FA, Adams MA (2015) Fire eases imbalances of nitrogen and phosphorus in woody plants. Ecosystems 18(5), 769-779.
| Crossref | Google Scholar |
Duane A, Castellnou M, Brotons L (2021) Towards a comprehensive look at global drivers of novel extreme wildfire events. Climatic Change 165(3), 43.
| Crossref | Google Scholar |
Eburn M, Cary GJ (2017) You own the fuel, but who owns the fire? International Journal of Wildland Fire 26(12), 999-1008.
| Crossref | Google Scholar |
Fernandes PM, Cruz MG (2012) Plant flammability experiments offer limited insight into vegetation–fire dynamics interactions. New Phytologist 194(3), 606-609.
| Crossref | Google Scholar | PubMed |
Foster CN, Barton PS, Robinson NM, MacGregor CI, Lindenmayer DB (2017) Effects of a large wildfire on vegetation structure in a variable fire mosaic. Ecological Applications 27(8), 2369-2381.
| Crossref | Google Scholar | PubMed |
Foster CN, Barton PS, MacGregor CI, Catford JA, Blanchard W, Lindenmayer DB (2018) Effects of fire regime on plant species richness and composition differ among forest, woodland and heath vegetation. Applied Vegetation Science 21(1), 132-143.
| Crossref | Google Scholar |
Granged AJP, Jordán A, Zavala LM, Muñoz-Rojas M, Mataix-Solera J (2011) Short-term effects of experimental fire for a soil under eucalyptus forest (SE Australia). Geoderma 167–168, 125-134.
| Crossref | Google Scholar |
Grootemaat S, Wright IJ, van Bodegom PM, Cornelissen JHC, Cornwell WK (2015) Burn or rot: leaf traits explain why flammability and decomposability are decoupled across species. Functional Ecology 29(11), 1486-1497.
| Crossref | Google Scholar |
Grootemaat S, Wright IJ, van Bodegom PM, Cornelissen JHC (2017) Scaling up flammability from individual leaves to fuel beds. Oikos 126(10), 1428-1438.
| Crossref | Google Scholar |
Hartig F (2020) ‘DHARMa: Residual Diagnostics for Hierarchical (Multi-Level/Mixed) Regression Models.’ R package version 0.3.0. 10.32614/CRAN.package.DHARMa
He M, Yan Z, Cui X, Gong Y, Li K, Han W (2020) Scaling the leaf nutrient resorption efficiency: nitrogen vs phosphorus in global plants. Science of The Total Environment 729, 138920.
| Crossref | Google Scholar | PubMed |
Hinojosa MB, Albert-Belda E, Gómez-Muñoz B, Moreno JM (2021) High fire frequency reduces soil fertility underneath woody plant canopies of Mediterranean ecosystems. Science of The Total Environment 752, 141877.
| Crossref | Google Scholar | PubMed |
Jones MW, Abatzoglou JT, Veraverbeke S, Andela N, Lasslop G, Forkel M, Smith AJ, Burton C, Betts RA, van der Werf GR (2022) Global and regional trends and drivers of fire under climate change. Reviews of Geophysics 60(3), e2020RG000726.
| Crossref | Google Scholar |
Koch AJ, Driscoll DA, Kirkpatrick JB (2008) Estimating the accuracy of tree ageing methods in mature Eucalyptus obliqua forest, Tasmania. Australian Forestry 71(2), 147-159.
| Crossref | Google Scholar |
Krishna MP, Mohan M (2017) Litter decomposition in forest ecosystems: a review. Energy, Ecology and Environment 2(4), 236-249.
| Crossref | Google Scholar |
Lagerström A, Esberg C, Wardle DA, Giesler R (2009) Soil phosphorus and microbial response to a long-term wildfire chronosequence in northern Sweden. Biogeochemistry 95(2), 199-213.
| Crossref | Google Scholar |
Liao X, Inglett PW, Inglett KS (2013) Fire effects on nitrogen cycling in native and restored calcareous wetlands. Fire Ecology 9(1), 6-20.
| Crossref | Google Scholar |
McCaw LW, Gould JS, Phillip Cheney N, Ellis PFM, Anderson WR (2012) Changes in behaviour of fire in dry eucalypt forest as fuel increases with age. Forest Ecology and Management 271, 170-181.
| Crossref | Google Scholar |
Montgomery KR, Cheo P (1971) Effect of leaf thickness on ignitibility. Forest Science 17(4), 475-478.
| Google Scholar |
Murphy BP, Russell-Smith J (2010) Fire severity in a northern Australian savanna landscape: the importance of time since previous fire. International Journal of Wildland Fire 19(1), 46-51.
| Crossref | Google Scholar |
Murphy BP, Bradstock RA, Boer MM, Carter J, Cary GJ, Cochrane MA, Fensham RJ, Russell-Smith J, Williamson GJ, Bowman DMJS (2013) Fire regimes of Australia: a pyrogeographic model system. Journal of Biogeography 40(6), 1048-1058.
| Crossref | Google Scholar |
Nolan RH, Collins L, Leigh A, Ooi MKJ, Curran TJ, Fairman TA, Resco de Dios V, Bradstock R (2021) Limits to post-fire vegetation recovery under climate change. Plant, Cell & Environment 44(11), 3471-3489.
| Crossref | Google Scholar | PubMed |
Nolan RH, Foster B, Griebel A, Choat B, Medlyn BE, Yebra M, Younes N, Boer MM (2022) Drought-related leaf functional traits control spatial and temporal dynamics of live fuel moisture content. Agricultural and Forest Meteorology 319, 108941.
| Crossref | Google Scholar |
Pausas JG, Moreira B (2012) Flammability as a biological concept. New Phytologist 194(3), 610-613.
| Crossref | Google Scholar | PubMed |
Pausas JG, Alessio GA, Moreira B, Corcobado G (2012) Fires enhance flammability in Ulex parviflorus. New Phytologist 193(1), 18-23.
| Crossref | Google Scholar | PubMed |
Pausas JG, Keeley JE, Schwilk DW (2017) Flammability as an ecological and evolutionary driver. Journal of Ecology 105(2), 289-297.
| Crossref | Google Scholar |
Pellegrini AFA, Hedin LO, Staver AC, Govender N (2015) Fire alters ecosystem carbon and nutrients but not plant nutrient stoichiometry or composition in tropical savanna. Ecology 96(5), 1275-1285.
| Crossref | Google Scholar | PubMed |
Popović Z, Bojović S, Marković M, Cerdà A (2021) Tree species flammability based on plant traits: a synthesis. Science of the Total Environment 800, 149625.
| Crossref | Google Scholar | PubMed |
Pressler Y, Moore JC, Cotrufo MF (2019) Belowground community responses to fire: meta-analysis reveals contrasting responses of soil microorganisms and mesofauna. Oikos 128(3), 309-327.
| Crossref | Google Scholar |
Raison RJ, Woods PV, Khanna PK (1986) Decomposition and accumulation of litter after fire in sub-alpine eucalypt forests. Australian Journal of Ecology 11(1), 9-19.
| Crossref | Google Scholar |
Resco de Dios V, Arteaga C, Peguero-Pina JJ, Sancho-Knapik D, Qin H, Zveushe OK, Sun W, Williams DG, Boer MM, Voltas J, Moreno JM, Tissue DT, Gil-Pelegrín E (2020) Hydraulic and photosynthetic limitations prevail over root non-structural carbohydrate reserves as drivers of resprouting in two Mediterranean oaks. Plant, Cell & Environment 43(8), 1944-1957.
| Crossref | Google Scholar | PubMed |
Scarff FR, Westoby M (2006) Leaf litter flammability in some semi-arid Australian woodlands. Functional Ecology 20(5), 745-752.
| Crossref | Google Scholar |
Scarff FR, Westoby M (2008) The influence of tissue phosphate on plant flammability: a kinetic study. Polymer Degradation and Stability 93(10), 1930-1934.
| Crossref | Google Scholar |
Scarff FR, Gray BF, Westoby M (2012) Exploring phosphate effects on leaf flammability using a physical chemistry model. International Journal of Wildland Fire 21(8), 1042-1051.
| Crossref | Google Scholar |
Searle SR, Speed FM, Milliken GA (1980) Population marginal means in the linear model: an alternative to least squares means. The American Statistician 34(4), 216-221.
| Crossref | Google Scholar |
Shepherd M, Raymond C (2010) Species differentiation and gene flow in the Blackbutts (genus Eucalyptus subgenus Eucalyptus section Pseudophloius). Conservation Genetics 11(5), 1965-1978.
| Crossref | Google Scholar |
Taudière A, Richard F, Carcaillet C (2017) Review on fire effects on ectomycorrhizal symbiosis, an unachieved work for a scalding topic. Forest Ecology and Management 391, 446-457.
| Crossref | Google Scholar |
Toberman H, Chen C, Lewis T, Elser JJ (2014) High-frequency fire alters C: N: P stoichiometry in forest litter. Global Change Biology 20(7), 2321-2331.
| Crossref | Google Scholar | PubMed |
Tumino BJ, Duff TJ, Goodger JQD, Cawson JG (2019) Plant traits linked to field-scale flammability metrics in prescribed burns in Eucalyptus forest. PLOS One 14(8), e0221403.
| Crossref | Google Scholar | PubMed |
Turner J, Lambert MJ (2008) Nutrient cycling in age sequences of two Eucalyptus plantation species. Forest Ecology and Management 255(5), 1701-1712.
| Crossref | Google Scholar |
Turner J, Lambert MJ (2015) Analysis of nutrient use efficiency (NUE) in Eucalyptus pilularis forests. Australian Journal of Botany 62(7), 558-569.
| Crossref | Google Scholar |
Varner JM, Kane JM, Kreye JK, Engber E (2015) The flammability of forest and woodland litter: a synthesis. Current Forestry Reports 1(2), 91-99.
| Crossref | Google Scholar |
Varner JM, Hood SM, Aubrey DP, Yedinak K, Hiers JK, Jolly WM, Shearman TM, McDaniel JK, O’Brien JJ, Rowell EM (2021) Tree crown injury from wildland fires: causes, measurement and ecological and physiological consequences. New Phytologist 231(5), 1676-1685.
| Crossref | Google Scholar | PubMed |
Whitford KR (2002) Hollows in jarrah (Eucalyptus marginata) and marri (Corymbia calophylla) trees: I. Hollow sizes, tree attributes and ages. Forest Ecology and Management 160(1), 201-214.
| Crossref | Google Scholar |
Wickham H, Chang W, Wickham MH (2016) Package ‘ggplot2’. Create Elegant Data Visualisations Using the Grammar of Graphics. Version 2(1), 1-189.
| Google Scholar |
Youngentob KN, Zdenek C, van Gorsel E (2016) A simple and effective method to collect leaves and seeds from tall trees. Methods in Ecology and Evolution 7(9), 1119-1123.
| Crossref | Google Scholar |
Yuan ZY, Chen HYH (2015) Decoupling of nitrogen and phosphorus in terrestrial plants associated with global changes. Nature Climate Change 5(5), 465-469.
| Crossref | Google Scholar |
Zhang L, Liu L, Pan K, Li W, Wang Y, Deng M, Xia J, Yang X (2015) Post-wildfire soil and plant foliar nutrient ratios and soil fungi: bacterial ratios in alpine meadows on the southeastern Qinghai-Tibet Plateau. International Journal of Wildland Fire 24(7), 933-939.
| Crossref | Google Scholar |